Abstract
Parathyroid hormone (PTH) and fibroblast growth factor 23 (FGF23) each play a central role in the pathogenesis of chronic kidney disease (CKD)-mineral and bone disorder. Levels of both hormones increase progressively in advanced CKD and can lead to damage in multiple organs. Secondary hyperparathyroidism (SHPT), characterized by parathyroid hyperplasia with increased PTH secretion, is associated with fractures and mortality. Emerging evidence suggests that these associations may be partially explained by PTH-induced browning of adipose tissue and increased energy expenditure. Observational studies suggest a survival benefit of PTHlowering therapy, and a recent study comparing parathyroidectomy and calcimimetics further suggests the importance of intensive PTH control. The mechanisms underlying the regulation of FGF23 secretion by osteocytes in response to phosphate load have been unclear, but recent experimental studies have identified glycerol-3-phosphate, a byproduct of glycolysis released by the kidney, as a key regulator of FGF23 production. Elevated FGF23 levels have been shown to be associated with mortality, and experimental data suggest off-target adverse effects of FGF23. However, the causal role of FGF23 in adverse outcomes in CKD patients remains to be established. Further studies are needed to determine whether intensive SHPT control improves clinical outcomes and whether treatment targeting FGF23 can improve patient outcomes.
Patients with chronic kidney disease (CKD) experience a progressive deterioration of mineral metabolism, including conditions such as hyperphosphatemia and hypocalcemia, as their kidney function declines. Parathyroid hormone (PTH) and fibroblast growth factor 23 (FGF23) play important roles in the pathophysiology of mineral disturbances and subsequent bone abnormalities [1]. Several cohort studies have shown that fracture risk increases as kidney function deteriorates [2,3], suggesting the importance of therapeutic interventions for bone abnormalities in CKD patients. Observational studies have also shown an increased risk of cardiovascular disease and mortality in this population [4-6]. This increased risk is attributable not only to traditional factors but also to CKD-specific factors, such as mineral imbalances. Consequently, the term “CKD-mineral and bone disorder” (CKD-MBD) has been proposed to describe the broader clinical syndrome involving mineral metabolism, bone abnormalities, and vascular calcification [7]. PTH and FGF23 are important components of CKD-MBD and have been the focus of extensive research over the years.
This review provides an overview of the role of PTH and FGF23 in the pathophysiology of CKD-MBD. It also discusses the current understanding of relevant adverse outcomes of CKD-MBD and the management of this condition.
CKD-MBD develops in the early stages of CKD. As kidney function declines, the number of functioning nephrons decreases and urinary phosphate excretion decreases. FGF23, which is secreted by osteocytes, increases phosphate excretion per nephron [8-10], presumably as a compensatory response. However, FGF23 suppresses the biosynthesis of 1,25-dihidryoxyvitamin D (1,25D) in proximal tubular cells, which decreases the intestinal absorption of calcium and phosphate. Consequently, PTH secretion in the parathyroid glands increases, which also increases phosphate excretion per nephron [11]. Thus, in patients with early-stage CKD, circulating levels of FGF23 and PTH increase to maintain a neutral phosphate balance by increasing urinary phosphate excretion, but as a trade-off, secondary hyperparathyroidism (SHPT) begins to progress. In patients with moderate to advanced CKD, decreased kidney expression of Klotho attenuates the ability of the kidney to excrete urinary phosphate, leading to an increase in serum phosphate. This process is accompanied by a progressive reduction in 1,25D levels through the inhibition of CYP27B1 by hyperphosphatemia [11], which further stimulates PTH secretion and causes serum calcium levels to decrease. In patients with advanced-stage CKD, the progression to kidney failure overcomes the compensatory system of FGF23 and PTH to maintain a neutral phosphate balance with a decreased nephron mass, resulting in overt hyperphosphatemia, hypocalcemia, and SHPT.
When patients reach kidney failure, the circulating levels of PTH and FGF23 increase 5- to 10-fold and 10- to 50-fold above normal, respectively. We prospectively studied the effect of hemodialysis initiation and found progressive reductions in serum PTH and FGF23 levels, with dialysis-related fluctuations [12]. We also found that the magnitude of FGF23 reduction was strongly associated with concomitant changes in serum phosphate levels, supporting the view that phosphate is a strong regulator of FGF23 production.
However, the underlying mechanism through which phosphate increases FGF23 production in osteocytes remains incompletely understood. Emerging evidence indicates that phosphate promotes glycolysis in proximal tubular cells via the type 2a sodium-dependent phosphate cotransporter. Consequently, kidney-derived glycerol-3-phosphate (G-3-P), a byproduct of glycolysis, stimulates the production of FGF23 by osteocytes [13]. The absence of an increase in G-3-P levels in the liver, heart, or skeletal muscle after phosphate loading suggests that phosphatestimulated G-3-P production is likely specific to the proximal tubules. In cases of acute kidney injury, levels of circulating G3-P derived from the injured kidney increase rapidly, thereby stimulating osteocytes to produce FGF23 [14]. However, limited information exists regarding the relationship between G-3-P and FGF23 in patients with CKD. Further studies are needed to elucidate the role of G-3-P in CKD.
Patients with advanced CKD develop various skeletal manifestations as SHPT progresses. The most common skeletal manifestation of severe SHPT is high-turnover bone disease (osteitis fibrosa), which is characterized by excessive rates of bone resorption and formation, increased cortical porosity, and progressive bone loss [15,16]. A longitudinal study of patients with CKD stages 2 to 5D demonstrated that a decrease in cortical bone loss over time was associated with elevated levels of PTH and bone turnover markers [17], suggesting that SHPT-mediated cortical porosity increases bone fragility and fracture risk. Inconsistent findings have been reported regarding the association between PTH levels and fracture risk, but the international Dialysis Outcomes and Practice Patterns Study (DOPPS) [18] and the European Current Management of Secondary Hyperparathyroidism: A Multicenter Observational Study (COSMOS) [19] have shown that intact PTH levels above 800 to 900 pg/mL are independently associated with an increased risk of fragility fractures.
Importantly, several cohort studies from different regions of the world have consistently shown that SHPT is associated not only with the risk of fragility fractures, but also with mortality [20-23]. Of note, a recently published large prospective cohort study using data from the nationwide registry of Japanese dialysis patients showed that higher intact PTH levels were almost linearly associated with an increased risk of all-cause and cardiovascular mortality in a time-averaged model [23]. We and others have also reported significant associations between parathyroidectomy and improved survival [24-26]. Although these studies are observational and cannot prove causality, the associations remained significant after adjustment for potential confounders, suggesting that intensive PTH control, as seen after parathyroidectomy, may contribute to better clinical outcomes in patients with kidney failure.
To support the validity of therapy aiming to control PTH, it is important to elucidate the mechanisms by which elevated PTH might lead to poor clinical outcomes. Fractures and vascular calcification are often considered as possible mechanisms, but these cannot fully explain the association between SHPT and increased mortality. In this context, an emerging potential mechanism is wasting, which is characterized by increased energy expenditure. A previous clinical study of hemodialysis patients reported that elevated PTH levels were an independent determinant of increased energy expenditure, as measured by indirect calorimetry [27]. Moreover, the increased energy expenditure diminished significantly in all patients 6 months after parathyroidectomy, suggesting that PTH plays a key role in the pathogenesis of wasting in advanced CKD. The mechanisms underlying these observations have been elucidated through insights from cancer research. Kir et al. [28] discovered that PTH-related protein (PTHrP), a tumor-derived protein that causes hypercalcemia of malignancy, directly drives thermogenesis in adipose tissue by switching from white to brown adipocytes (a phenomenon termed adipose tissue browning), suggesting that PTHrP mediates energy wasting in adipose tissue and contributes to cancer cachexia. In collaboration with this group, we have shown that PTH also stimulates adipose tissue browning and causes cachexia in advanced CKD [29]. Furthermore, we have shown that adipocyte-specific PTH/PTHrP receptor knockout mice are resistant to adipose tissue browning and wasting even at high PTH levels induced by 5/6 nephrectomy.
These experimental data identified PTH as a driver of adipose tissue browning and wasting, but it remained unclear whether poorly controlled SHPT leads to increased energy expenditure and contributes to poor clinical outcomes. To address this, we analyzed data from the international DOPPS and found a strong linear correlation between baseline PTH levels and weight loss (a surrogate marker of wasting) over the subsequent 12 months [30]. This association remained significant after adjustment for numerous covariates and was robust regardless of recent hospitalization. Furthermore, the association between PTH levels and weight loss partially mediated the increased risk of mortality associated with elevated PTH levels. These findings suggest that this pathway may be a mediator between elevated PTH levels and mortality in patients undergoing dialysis.
Another possible mechanism linking SHPT to poor clinical outcomes may be through elevated circulating FGF23 levels. PTH is one of the main regulators of FGF23 secretion in osteocytes, and SHPT is crucial for maintaining high FGF23 levels [31]. We and others have reported that both calcimimetics and parathyroidectomy significantly decrease both circulating PTH and FGF23 levels in patients with SHPT undergoing dialysis [32,33], suggesting that the survival benefit associated with parathyroidectomy may be mediated by the reduction of both PTH and FGF23 levels. As described below, several experimental studies have demonstrated that FGF23 directly induces multiple-organ injuries. However, it remains to be determined whether FGF23 directly contributes to poor outcomes in patients with advanced CKD, and further studies are needed to confirm this possibility.
Until several years ago, vitamin D receptor activators (VDRAs) were the mainstay of treatment for SHPT. However, the clinical utility of VDRAs was limited because advanced parathyroid hyperplasia develops tolerance to these drugs due to decreased expression of the calcium-sensing receptor (CaSR) and vitamin D receptor in the parathyroid gland [34-36]. Calcimimetics, a relatively new option for the therapeutic control of SHPT, allosterically modulate the parathyroid CaSR and increase its sensitivity to extracellular calcium. Currently, calcimimetics are considered the first choice for SHPT in dialysis patients because they exert a strong inhibitory effect on PTH, leading to an effective reduction in parathyroid volume [37]. Two recent experimental studies evaluated the effects of calcimimetics on bone metabolism and showed that evocalcet [15] and etelcalcetide [36], which are novel calcimimetics, suppressed the progression of cortical porosity in severe SHPT. In the clinical setting, the Bone Histomorphometry Assessment for Dialysis Patients with Secondary Hyperparathyroidism of End-Stage Renal Disease (BONAFIDE) study showed that 6 to 12 months of treatment with cinacalcet reduced the elevated bone formation rate and improved bone histology [38]. Regarding the effect of calcimimetics on fracture risk, a subanalysis of the Evaluation of Cinacalcet Hydrochloride Therapy to Lower Cardiovascular Events (EVOLVE) trial, which found no significant effect of cinacalcet in the primary intention-to-treat analysis, showed a significant reduction in fracture risk in the cinacalcet group in the prespecified lag-censoring analysis [39]. The EVOLVE study also suggests that cinacalcet may improve survival. Although the primary analysis did not show a significant reduction in all-cause and cardiovascular mortality, the prespecified lag-censoring analysis showed a significant effect in patients treated with cinacalcet [40]. Taken together, these clinical trial data highlight the importance of controlling parathyroid function and bone turnover as a means of not only reducing fracture risk, but also improving survival.
How tightly should we control PTH in patients with kidney failure? Since parathyroidectomy lowers PTH levels more drastically than calcimimetics, it stands to reason that comparing the long-term outcomes of these treatments could shed light on this issue. Therefore, we analyzed data from the nationwide registry of Japanese dialysis patients and compared patients who underwent parathyroidectomy to those who started treatment with cinacalcet after propensity matching at a 1:3 ratio [41]. Patients in the parathyroidectomy group had lower levels of intact PTH, calcium, and phosphorus than those in the cinacalcet group. During the 6-year follow-up period, parathyroidectomy was associated with a 22% lower risk of mortality compared with cinacalcet. As an exploratory analysis, we stratified patients undergoing parathyroidectomy into tertiles based on their postoperative intact PTH levels and performed propensity score matching for each tertile. The survival benefit of parathyroidectomy over cinacalcet was most evident in patients who exhibited a sustained reduction in postoperative PTH levels, supporting the concept of “the lower, the better” for the management of SHPT [42]. There may be concerns that excessive suppression of PTH following parathyroidectomy could lead to adynamic bone disease. However, we observed no difference in the incidence of hip fractures between the parathyroidectomy and cinacalcet groups. This finding suggests that the prevailing view that low PTH levels should be avoided to preserve bone strength may need to be reevaluated.
The target range for intact PTH levels in dialysis patients varies in different regions of the world. The Japanese Society for Dialysis Therapy guideline suggests maintaining intact PTH levels in the range of 60 to 240 pg/mL [43], whereas the Kidney Disease: Improving Global Outcomes (KDIGO) guideline suggests two to nine times the upper normal limit of intact PTH (approximately 130 to 585 pg/mL) [44]. Japanese hemodialysis patients show better survival outcomes than those in other regions [45], suggesting that more intensive PTH control may lead to better clinical outcomes. Further clinical studies are needed to determine the optimal PTH target in dialysis patients.
FGF23 acts on the parathyroid glands and directly inhibits PTH secretion [46]. However, most patients with advanced CKD have high PTH levels despite markedly high FGF23 levels. To determine the mechanism underlying parathyroid resistance, we examined hyperplastic parathyroid tissue from patients undergoing parathyroidectomy [47]. We found that the expression of Klotho and its coreceptor FGF receptor 1 (FGFR1) significantly decreased, especially in glands with nodular hyperplasia (an advanced form of parathyroid hyperplasia). Other experimental studies using CKD models have also shown that FGF23 fails to suppress PTH secretion from parathyroid glands, presumably due to decreased expression of the Klotho-FGFR complex [48,49]. Of note, a recent experimental study using parathyroid-specific knockout mouse models demonstrated the importance of Klotho-CaSR interactions in PTH synthesis and parathyroid hyperplasia [50]. In that study, parathyroid-specific deletion of Klotho alone did not suppress an inhibitory effect of FGF23 on PTH secretion, but dual deletion of Klotho and CaSR led to increased PTH secretion and accelerated parathyroid hyperplasia compared to CaSR deletion alone. These findings suggest that in advanced CKD, decreased expression of Klotho and FGFR1, as well as CaSR, may induce parathyroid resistance to FGF23 and thus contribute to parathyroid hypersecretion and SHPT progression [1].
Another potential target for FGF23 is bone, as the Klotho-FGFR1 complex is expressed at low levels in osteoblasts and osteocytes [51]. Although the role of Klotho in bone cells is not fully understood, recent experimental studies suggest that FGF23 acts directly on osteocytes via the Klotho-FGFR1 complex, thereby increasing the production of FGF23 itself and inhibiting bone formation [52,53]. Since Klotho expression in bone cells decreases in advanced CKD, it might induce skeletal resistance to FGF23. Further studies are needed to determine whether skeletal resistance to FGF23 can be a therapeutic target for renal osteodystrophy [54].
As described above, FGF23 is unlikely to exert Klotho-dependent effects on classical target organs such as parathyroid glands and bone in advanced CKD due to the decreased expression of Klotho. In this setting, FGF23 might exert Klotho-independent effects on non-classical target organs [55]. Several experimental studies suggest that FGF23 has several pathogenic off-target effects, the most extensively studied of which is the possibility that FGF23 acts on cardiomyocytes via FGFR4 to induce left ventricular hypertrophy [56,57]. This finding lends biological plausibility to the observational evidence showing a strong association between high FGF23 levels and mortality [58-62].
However, there are several caveats to the hypothesis that elevated FGF23 levels are associated with mortality. First, the strength of the association between FGF23 and mortality is not consistent across the spectrum of CKD. In patients with predialysis CKD, the association between FGF23 and mortality would be expected to increase as kidney function declines [58]. However, it is progressively attenuated after the initiation of dialysis and almost disappears during long-term dialysis, despite remarkably high levels of FGF23 [59-61]. It then reappears after kidney transplantation despite a striking decrease in FGF23 [62]. Therefore, the changing pattern of FGF23-associated mortality does not parallel the trajectory of circulating FGF23 levels, which suggests that the relationship cannot be explained by a simple exposure-response mechanism. Second, cardiac hypertrophy has not been reported in disorders involving FGF23 excess, such as X-linked hypophosphatemia [63,64]. Another hypothesis is that the effects of FGF23 on the myocardium are enhanced in advanced CKD. However, this possibility seems unlikely because, as noted above, the association between FGF23 and mortality is not strengthened in dialysis patients. Finally, it should be noted that injured cardiomyocytes have the potential to produce FGF23. Experimental studies in animal models of myocardial infarction and cardiac hypertrophy showed a striking increase in FGF23 expression in cardiomyocytes [65,66]. An observational study showed a remarkable increase in circulating FGF23 levels in patients with cardiogenic shock [67]. In light of these findings, it is more plausible to suggest that cardiac injury induces an elevation in FGF23 levels rather than being a consequence of it. Although observational studies have reported an association between FGF23 and mortality, it remains unclear whether FGF23 has a direct cardiac effect. Further studies are needed to confirm this possibility [68].
The off-target effects of FGF23 are not limited to its potential to induce cardiac hypertrophy; other effects include the induction of inflammation [69], immune dysfunction [70], and anemia [71]. These toxic effects have been demonstrated in experimental data, but the clinical implications and relevance of these off-target effects are even less well understood.
Elevated levels of PTH and FGF23 play a pivotal role in the pathogenesis of CKD-MBD and are associated with several adverse outcomes, including fractures, cardiovascular disease and mortality in patients with advanced CKD (Fig. 1). Emerging evidence suggests that potential mechanisms linking SHPT and mortality may be partially explained by PTH-induced wasting. PTH-lowering therapy may improve mortality by attenuating PTH-induced wasting and other adverse effects, and our recent comparison of parathyroidectomy and cinacalcet treatment suggests the potential benefit of intensive PTH control. The target range for intact PTH levels remains a matter of debate, and further research is needed to determine the optimal PTH target in dialysis patients. Observational studies have also shown an association between elevated FGF23 and mortality, but it is not well understood whether FGF23 directly induces multiple-organ injury. Further studies are needed to determine whether intensive SHPT control and FGF23-targeted therapy improve clinical outcomes in CKD patients.
REFERENCES
1. Komaba H, Fukagawa M. FGF23-parathyroid interaction: implications in chronic kidney disease. Kidney Int. 2010; 77:292–8.


2. Ensrud KE, Lui LY, Taylor BC, Ishani A, Shlipak MG, Stone KL, et al. Renal function and risk of hip and vertebral fractures in older women. Arch Intern Med. 2007; 167:133–9.


3. Tentori F, McCullough K, Kilpatrick RD, Bradbury BD, Robinson BM, Kerr PG, et al. High rates of death and hospitalization follow bone fracture among hemodialysis patients. Kidney Int. 2014; 85:166–73.


4. Fischer MJ, Kimmel PL, Greene T, Gassman JJ, Wang X, Brooks DH, et al. Elevated depressive affect is associated with adverse cardiovascular outcomes among African Americans with chronic kidney disease. Kidney Int. 2011; 80:670–8.


5. Denker M, Boyle S, Anderson AH, Appel LJ, Chen J, Fink JC, et al. Chronic Renal Insufficiency Cohort Study (CRIC): overview and summary of selected findings. Clin J Am Soc Nephrol. 2015; 10:2073–83.
6. Tanaka K, Watanabe T, Takeuchi A, Ohashi Y, Nitta K, Akizawa T, et al. Cardiovascular events and death in Japanese patients with chronic kidney disease. Kidney Int. 2017; 91:227–34.


7. Kidney Disease: Improving Global Outcomes (KDIGO) CKD-MBD Work Group. KDIGO clinical practice guideline for the diagnosis, evaluation, prevention, and treatment of Chronic Kidney Disease-Mineral and Bone Disorder (CKD-MBD). Kidney Int Suppl. 2009; 113:S1–130.
8. Shigematsu T, Kazama JJ, Yamashita T, Fukumoto S, Hosoya T, Gejyo F, et al. Possible involvement of circulating fibroblast growth factor 23 in the development of secondary hyperparathyroidism associated with renal insufficiency. Am J Kidney Dis. 2004; 44:250–6.


9. Gutierrez O, Isakova T, Rhee E, Shah A, Holmes J, Collerone G, et al. Fibroblast growth factor-23 mitigates hyperphosphatemia but accentuates calcitriol deficiency in chronic kidney disease. J Am Soc Nephrol. 2005; 16:2205–15.


10. Hasegawa H, Nagano N, Urakawa I, Yamazaki Y, Iijima K, Fujita T, et al. Direct evidence for a causative role of FGF23 in the abnormal renal phosphate handling and vitamin D metabolism in rats with early-stage chronic kidney disease. Kidney Int. 2010; 78:975–80.


11. Slatopolsky E, Delmez JA. Pathogenesis of secondary hyperparathyroidism. Nephrol Dial Transplant. 1996; 11 Suppl 3:130–5.


12. Kawabata C, Komaba H, Ishida H, Nakagawa Y, Hamano N, Koizumi M, et al. Changes in fibroblast growth factor 23 and soluble klotho levels after hemodialysis initiation. Kidney Med. 2019; 2:59–67.


13. Zhou W, Simic P, Zhou IY, Caravan P, Vela Parada X, Wen D, et al. Kidney glycolysis serves as a mammalian phosphate sensor that maintains phosphate homeostasis. J Clin Invest. 2023; 133:e164610.


14. Simic P, Kim W, Zhou W, Pierce KA, Chang W, Sykes DB, et al. Glycerol-3-phosphate is an FGF23 regulator derived from the injured kidney. J Clin Invest. 2020; 130:1513–26.


15. Hasegawa T, Tokunaga S, Yamamoto T, Sakai M, Hongo H, Kawata T, et al. Evocalcet rescues secondary hyperparathyroidism-driven cortical porosity in CKD male rats. Endocrinology. 2023; 164:bqad022.


16. Swallow EA, Metzger CE, Newman CL, Chen NX, Moe SM, Allen MR. Cortical porosity development and progression is mitigated after etelcalcetide treatment in an animal model of chronic kidney disease. Bone. 2022; 157:116340.


17. Nickolas TL, Stein EM, Dworakowski E, Nishiyama KK, Komandah-Kosseh M, Zhang CA, et al. Rapid cortical bone loss in patients with chronic kidney disease. J Bone Miner Res. 2013; 28:1811–20.


18. Jadoul M, Albert JM, Akiba T, Akizawa T, Arab L, BraggGresham JL, et al. Incidence and risk factors for hip or other bone fractures among hemodialysis patients in the Dialysis Outcomes and Practice Patterns Study. Kidney Int. 2006; 70:1358–66.


19. Barrera-Baena P, Rodriguez-Garcia M, Rodriguez-Rubio E, Gonzalez-Llorente L, Ortiz A, Zoccali C, et al. Serum phosphate is associated with increased risk of bone fragility fractures in hemodialysis patients. Nephrol Dial Transplant. 2023; 39:618–26.
20. Block GA, Klassen PS, Lazarus JM, Ofsthun N, Lowrie EG, Chertow GM. Mineral metabolism, mortality, and morbidity in maintenance hemodialysis. J Am Soc Nephrol. 2004; 15:2208–18.


21. Tentori F, Blayney MJ, Albert JM, Gillespie BW, Kerr PG, Bommer J, et al. Mortality risk for dialysis patients with different levels of serum calcium, phosphorus, and PTH: the Dialysis Outcomes and Practice Patterns Study (DOPPS). Am J Kidney Dis. 2008; 52:519–30.


22. Taniguchi M, Fukagawa M, Fujii N, Hamano T, Shoji T, Yokoyama K, et al. Serum phosphate and calcium should be primarily and consistently controlled in prevalent hemodialysis patients. Ther Apher Dial. 2013; 17:221–8.


23. Goto S, Hamano T, Fujii H, Taniguchi M, Abe M, Nitta K, et al. Hypocalcemia and cardiovascular mortality in cinacalcet users. Nephrol Dial Transplant. 2024; 39:637–47.


24. Kestenbaum B, Andress DL, Schwartz SM, Gillen DL, Seliger SL, Jadav PR, et al. Survival following parathyroidectomy among United States dialysis patients. Kidney Int. 2004; 66:2010–6.


25. Komaba H, Taniguchi M, Wada A, Iseki K, Tsubakihara Y, Fukagawa M. Parathyroidectomy and survival among Japanese hemodialysis patients with secondary hyperparathyroidism. Kidney Int. 2015; 88:350–9.


26. Ivarsson KM, Akaberi S, Isaksson E, Reihner E, Rylance R, Prutz KG, et al. The effect of parathyroidectomy on patient survival in secondary hyperparathyroidism. Nephrol Dial Transplant. 2015; 30:2027–33.


27. Cuppari L, de Carvalho AB, Avesani CM, Kamimura MA, Dos Santos Lobao RR, Draibe SA. Increased resting energy expenditure in hemodialysis patients with severe hyperparathyroidism. J Am Soc Nephrol. 2004; 15:2933–9.


28. Kir S, White JP, Kleiner S, Kazak L, Cohen P, Baracos VE, et al. Tumour-derived PTH-related protein triggers adipose tissue browning and cancer cachexia. Nature. 2014; 513:100–4.


29. Kir S, Komaba H, Garcia AP, Economopoulos KP, Liu W, Lanske B, et al. PTH/PTHrP receptor mediates cachexia in models of kidney failure and cancer. Cell Metab. 2016; 23:315–23.


30. Komaba H, Zhao J, Yamamoto S, Nomura T, Fuller DS, McCullough KP, et al. Secondary hyperparathyroidism, weight loss, and longer term mortality in haemodialysis patients: results from the DOPPS. J Cachexia Sarcopenia Muscle. 2021; 12:855–65.


31. Lavi-Moshayoff V, Wasserman G, Meir T, Silver J, NavehMany T. PTH increases FGF23 gene expression and mediates the high-FGF23 levels of experimental kidney failure: a bone parathyroid feedback loop. Am J Physiol Renal Physiol. 2010; 299:F882–9.


32. Koizumi M, Komaba H, Nakanishi S, Fujimori A, Fukagawa M. Cinacalcet treatment and serum FGF23 levels in haemodialysis patients with secondary hyperparathyroidism. Nephrol Dial Transplant. 2012; 27:784–90.


33. Takahashi H, Komaba H, Takahashi Y, Sawada K, Tatsumi R, Kanai G, et al. Impact of parathyroidectomy on serum FGF23 and soluble Klotho in hemodialysis patients with severe secondary hyperparathyroidism. J Clin Endocrinol Metab. 2014; 99:E652–8.


34. Tominaga Y, Tanaka Y, Sato K, Nagasaka T, Takagi H. Histopathology, pathophysiology, and indications for surgical treatment of renal hyperparathyroidism. Semin Surg Oncol. 1997; 13:78–86.


35. Kifor O, Moore FD Jr, Wang P, Goldstein M, Vassilev P, Kifor I, et al. Reduced immunostaining for the extracellular Ca2+-sensing receptor in primary and uremic secondary hyperparathyroidism. J Clin Endocrinol Metab. 1996; 81:1598–606.


36. Fukuda N, Tanaka H, Tominaga Y, Fukagawa M, Kurokawa K, Seino Y. Decreased 1,25-dihydroxyvitamin D3 receptor density is associated with a more severe form of parathyroid hyperplasia in chronic uremic patients. J Clin Invest. 1993; 92:1436–43.


37. Komaba H, Nakanishi S, Fujimori A, Tanaka M, Shin J, Shibuya K, et al. Cinacalcet effectively reduces parathyroid hormone secretion and gland volume regardless of pretreatment gland size in patients with secondary hyperparathyroidism. Clin J Am Soc Nephrol. 2010; 5:2305–14.


38. Behets GJ, Spasovski G, Sterling LR, Goodman WG, Spiegel DM, De Broe ME, et al. Bone histomorphometry before and after long-term treatment with cinacalcet in dialysis patients with secondary hyperparathyroidism. Kidney Int. 2015; 87:846–56.


39. Moe SM, Abdalla S, Chertow GM, Parfrey PS, Block GA, Correa-Rotter R, et al. Effects of cinacalcet on fracture events in patients receiving hemodialysis: the EVOLVE Trial. J Am Soc Nephrol. 2015; 26:1466–75.
40. EVOLVE Trial Investigators, Chertow GM, Block GA, Correa-Rotter R, Drueke TB, Floege J, et al. Effect of cinacalcet on cardiovascular disease in patients undergoing dialysis. N Engl J Med. 2012; 367:2482–94.


41. Komaba H, Hamano T, Fujii N, Moriwaki K, Wada A, Masakane I, et al. Parathyroidectomy vs cinacalcet among patients undergoing hemodialysis. J Clin Endocrinol Metab. 2022; 107:2016–25.


42. Evenepoel P, Jorgensen HS. Parathyroidectomy versus calcimimetic: the lower the PTH the better? J Clin Endocrinol Metab. 2022; 107:e3532. –3.


43. Fukagawa M, Yokoyama K, Koiwa F, Taniguchi M, Shoji T, Kazama JJ, et al. Clinical practice guideline for the management of chronic kidney disease-mineral and bone disorder. Ther Apher Dial. 2013; 17:247–88.


44. Kidney Disease: Improving Global Outcomes (KDIGO) CKD-MBD Update Work Group. KDIGO 2017 clinical practice guideline update for the diagnosis, evaluation, prevention, and treatment of chronic kidney disease-mineral and bone disorder (CKD-MBD). Kidney Int Suppl (2011). 2017; 7:1–59.
45. Yamamoto S, Karaboyas A, Komaba H, Taniguchi M, Nomura T, Bieber BA, et al. Mineral and bone disorder management in hemodialysis patients: comparing PTH control practices in Japan with Europe and North America: the Dialysis Outcomes and Practice Patterns Study (DOPPS). BMC Nephrol. 2018; 19:253.


46. Ben-Dov IZ, Galitzer H, Lavi-Moshayoff V, Goetz R, Kuroo M, Mohammadi M, et al. The parathyroid is a target organ for FGF23 in rats. J Clin Invest. 2007; 117:4003–8.


47. Komaba H, Goto S, Fujii H, Hamada Y, Kobayashi A, Shibuya K, et al. Depressed expression of Klotho and FGF receptor 1 in hyperplastic parathyroid glands from uremic patients. Kidney Int. 2010; 77:232–8.


48. Galitzer H, Ben-Dov IZ, Silver J, Naveh-Many T. Parathyroid cell resistance to fibroblast growth factor 23 in secondary hyperparathyroidism of chronic kidney disease. Kidney Int. 2010; 77:211–8.


49. Canalejo R, Canalejo A, Martinez-Moreno JM, RodriguezOrtiz ME, Estepa JC, Mendoza FJ, et al. FGF23 fails to inhibit uremic parathyroid glands. J Am Soc Nephrol. 2010; 21:1125–35.


50. Fan Y, Liu W, Bi R, Densmore MJ, Sato T, Mannstadt M, et al. Interrelated role of Klotho and calcium-sensing receptor in parathyroid hormone synthesis and parathyroid hyperplasia. Proc Natl Acad Sci U S A. 2018; 115:E3749–58.


51. Rhee Y, Bivi N, Farrow E, Lezcano V, Plotkin LI, White KE, et al. Parathyroid hormone receptor signaling in osteocytes increases the expression of fibroblast growth factor-23 in vitro and in vivo. Bone. 2011; 49:636–43.


52. Kaludjerovic J, Komaba H, Sato T, Erben RG, Baron R, Olauson H, et al. Klotho expression in long bones regulates FGF23 production during renal failure. FASEB J. 2017; 31:2050–64.


53. Komaba H, Kaludjerovic J, Hu DZ, Nagano K, Amano K, Ide N, et al. Klotho expression in osteocytes regulates bone metabolism and controls bone formation. Kidney Int. 2017; 92:599–611.


54. Komaba H, Lanske B. Role of Klotho in bone and implication for CKD. Curr Opin Nephrol Hypertens. 2018; 27:298–304.


55. Komaba H, Fukagawa M. The role of FGF23 in CKD: with or without Klotho. Nat Rev Nephrol. 2012; 8:484–90.
56. Faul C, Amaral AP, Oskouei B, Hu MC, Sloan A, Isakova T, et al. FGF23 induces left ventricular hypertrophy. J Clin Invest. 2011; 121:4393–408.


57. Grabner A, Amaral AP, Schramm K, Singh S, Sloan A, Yanucil C, et al. Activation of cardiac fibroblast growth factor receptor 4 causes left ventricular hypertrophy. Cell Metab. 2015; 22:1020–32.


58. Isakova T, Xie H, Yang W, Xie D, Anderson AH, Scialla J, et al. Fibroblast growth factor 23 and risks of mortality and end-stage renal disease in patients with chronic kidney disease. JAMA. 2011; 305:2432–9.


59. Gutierrez OM, Mannstadt M, Isakova T, Rauh-Hain JA, Tamez H, Shah A, et al. Fibroblast growth factor 23 and mortality among patients undergoing hemodialysis. N Engl J Med. 2008; 359:584–92.


60. Chonchol M, Greene T, Zhang Y, Hoofnagle AN, Cheung AK. Low vitamin D and high fibroblast growth factor 23 serum levels associate with infectious and cardiac deaths in the HEMO Study. J Am Soc Nephrol. 2016; 27:227–37.


61. Komaba H, Fuller DS, Taniguchi M, Yamamoto S, Nomura T, Zhao J, et al. Fibroblast growth factor 23 and mortality among prevalent hemodialysis patients in the Japan Dialysis Outcomes and Practice Patterns Study. Kidney Int Rep. 2020; 5:1956–64.


62. Wolf M, Molnar MZ, Amaral AP, Czira ME, Rudas A, Ujszaszi A, et al. Elevated fibroblast growth factor 23 is a risk factor for kidney transplant loss and mortality. J Am Soc Nephrol. 2011; 22:956–66.


63. Pastor-Arroyo EM, Gehring N, Krudewig C, Costantino S, Bettoni C, Knopfel T, et al. The elevation of circulating fibroblast growth factor 23 without kidney disease does not increase cardiovascular disease risk. Kidney Int. 2018; 94:49–59.


64. Takashi Y, Kinoshita Y, Hori M, Ito N, Taguchi M, Fukumoto S. Patients with FGF23-related hypophosphatemic rickets/osteomalacia do not present with left ventricular hypertrophy. Endocr Res. 2017; 42:132–7.


65. Andrukhova O, Slavic S, Odorfer KI, Erben RG. Experimental myocardial infarction upregulates circulating fibroblast growth factor-23. J Bone Miner Res. 2015; 30:1831–9.


66. Matsui I, Oka T, Kusunoki Y, Mori D, Hashimoto N, Matsumoto A, et al. Cardiac hypertrophy elevates serum levels of fibroblast growth factor 23. Kidney Int. 2018; 94:60–71.


67. Poss J, Mahfoud F, Seiler S, Heine GH, Fliser D, Bohm M, et al. FGF-23 is associated with increased disease severity and early mortality in cardiogenic shock. Eur Heart J Acute Cardiovasc Care. 2013; 2:211–8.


68. Komaba H, Fukagawa M. Jury still out on whether FGF23 is a direct contributor, a useful biomarker, or neither. Kidney Int. 2021; 100:989–93.


69. Singh S, Grabner A, Yanucil C, Schramm K, Czaya B, Krick S, et al. Fibroblast growth factor 23 directly targets hepatocytes to promote inflammation in chronic kidney disease. Kidney Int. 2016; 90:985–96.


Fig. 1.
Roles of parathyroid hormone (PTH) and fibroblast growth factor 23 (FGF23) in the pathogenesis of chronic kidney disease-mineral and bone disorder. In patients with impaired kidney function, FGF23 production by osteocytes increases. Recent experimental data have shown that glycerol-3-phosphate (G-3-P), a byproduct of glycolysis in proximal tubular cells, stimulates FGF23 production in bone. Increased FGF23 reduces 1,25-dihydroxyvitamin D (1,25D), which stimulates PTH secretion, leading to secondary hyperparathyroidism. Elevated PTH not only causes high-turnover bone disease, but also induces wasting and muscle atrophy through browning of adipose tissue, which contributes to increased mortality. Elevated FGF23 is implicated in the development of left ventricular hypertrophy, which may also lead to increased mortality. Npt2a, sodium-dependent phosphate transport protein 2A.
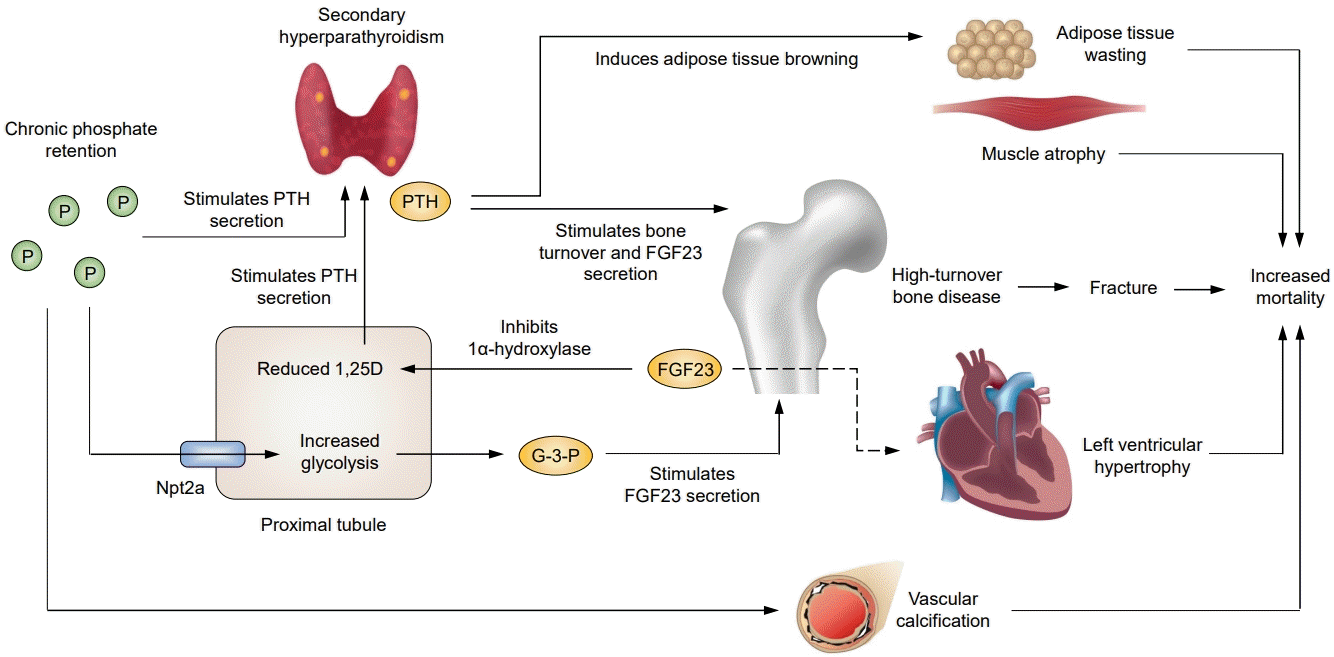