Abstract
Heart failure with preserved ejection fraction (HFpEF) is prevalent and associated with a poor prognosis, imposing a significant burden on society. Arterial stiffness is increasingly recognized as a crucial factor in the pathophysiology of HFpEF, affecting diagnosis, management, and prognosis. As a hallmark of vascular aging, arterial stiffness contributes to increased afterload on the left ventricle (LV), leading to diastolic dysfunction, a key feature of HFpEF. Elevated arterial stiffness is linked with common cardiovascular risk factors in HFpEF, such as hypertension, diabetes and obesity, exacerbating the progression of disease. Studies have demonstrated that patients with HFpEF exhibit significantly higher levels of arterial stiffness compared to those without HFpEF, highlighting the value of arterial stiffness measurements as both diagnostic and prognostic tools. Moreover, interventions aimed at reducing arterial stiffness, whether through pharmacological therapies or lifestyle modifications, have shown potential in improving LV diastolic function and patient outcomes. Despite these advancements, the precise mechanisms by which arterial stiffness contributes to HFpEF are still not fully understood, necessitating the need for further research.
Graphical Abstract
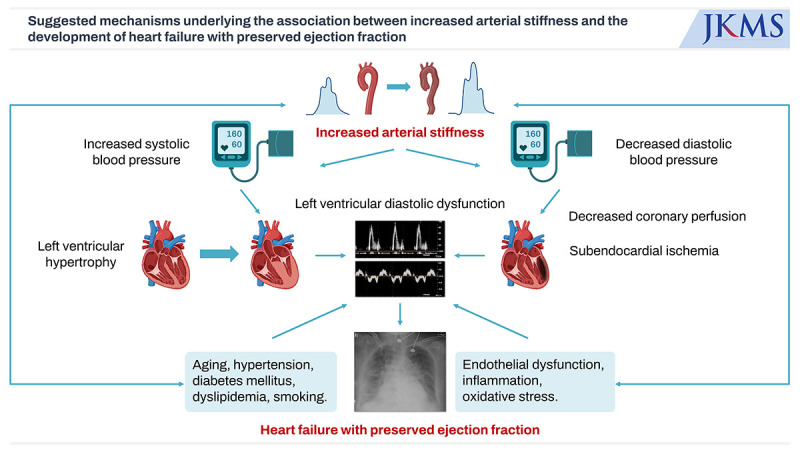
Heart failure (HF) is a condition characterized by functional or structural abnormalities of the heart resulting from various causes. These abnormalities can lead to disruptions in the ejection or filling of blood in the ventricles, manifesting as symptoms such as dyspnea, leg swelling and fatigue, as well as physical signs like lung crackles and elevated jugular venous pressure.1 As nearly all uncontrolled heart diseases ultimately progress to HF, one could describe HF as the ultimate outcome of heart-related conditions.
The prevalence and incidence of HF are steadily on the rise.2 HF is characterized by a poor prognosis, marked by high mortality and morbidity rates,2 and it stands as a significant contributor to hospitalizations and fatalities among the elderly population.3 In the United States (US), the number of HF patients aged 20 or older increased from approximately 6 million between 2015 and 2018 to around 6.7 million between 2017 and 2020. Projections indicate that by 2030, more than 8 million people in the US aged 18 years and older are expected to be afflicted with HF, marking a 46% increase in prevalence compared to 2012. The prevalence of HF patients relative to the total patient population is projected to climb from 2.4% in 2012 to 3.0% in 2030 in the US.2 According to research results from 2010, there were approximately 4 million HF patients in China.4 In 2005, the number of HF patients in Japan was estimated to be 1 million, and it is projected to increase by approximately 30% to reach 1.3 million by 2035.5 In South Korea, when analyzing data from the National Health Information Database, the prevalence of HF increased from 0.75% in 2002 to 1.53% in 2012. It is estimated to reach 3.35% in 2040, affecting approximately 1.7 million people.6 According to several registry data focusing on Koreans, the one-year mortality rate for patients with acute HF is notably high, estimated to be around 15–20%.7
HF is typically classified into two main types based on left ventricular ejection fraction (LVEF): HF with reduced ejection fraction (HFrEF), characterized by LVEF < 40%, and HF with preserved ejection fraction (HFpEF), characterized by LVEF > 50%.1 The two types of HF require distinct management strategies and medications. Additionally, patients with HFrEF and HFpEF may experience different prognoses and outcomes. Therefore, the classification of HF is vital for tailoring individualized patient care and implementing targeted treatment strategies, and it should be determined through echocardiography during the diagnosis of HF.1
HFpEF is a disease with a high prevalence and poor prognosis similar to HFrEF, posing a significant burden not only on individuals but also on our society.8910 Understanding the pathophysiology of HF is crucial for preventing HF and developing effective treatments. In HFrEF, it is well-established that the activation of the neurohormonal system plays a significant role in left ventricular (LV) remodeling and functional decline following myocardial damage caused by factors such as hypertension or myocardial infarction.11121314 Building upon this underlying pathophysiology, medications such as β-blockers (BBs), renin-angiotensin-aldosterone system (RAAS) blockers, and mineralocorticoid receptor antagonists have been developed to halt the progression of HFrEF and enhance the prognosis of affected patients.1 However, the development of HpEF is less understood and more complex than that of HFrEF. Consequently, there are limited pharmacological options available to improve the long-term prognosis of HFpEF patients.15 A pressing need exists for further research to elucidate the causes and mechanisms behind HFpEF in order to develop effective treatments that can enhance the prognosis of individuals affected by HFpEF.16
Multiple studies have established the profound impact of increased arterial stiffness on the structural and functional changes of the LV, ultimately contributing to the pathogenesis of HFpEF. This comprehensive review thoroughly examines the intricate relationship between arterial stiffness and HFpEF. By exploring how arterial stiffness affects HFpEF, this review also aims to enhance our understanding of how the disease develops. This could lead to new treatment approach and actions that focus on arterial stiffness as a modifiable risk factor in managing HFpEF.
Histologically, the arteries in our body can be categorized into two main types: elastic arteries and muscular arteries. Elastic arteries exhibit remarkable compliance, primarily attributed to the abundance of elastic fibers within their vessel walls. Notable examples of elastic arteries are the central arteries located in proximity to the heart, such as the aorta, carotid artery, pulmonary trunk, subclavian artery, and common iliac artery. As one moves away from the heart toward the peripheral regions of the circulatory system, the concentration of elastic fibers within the arterial walls diminishes, while the muscle layer becomes more prominent. These peripheral arteries are commonly referred to as muscular arteries and include well-known examples like the brachial, femoral, and mesenteric arteries.17
Arterial stiffness is a concept that encompasses the reduced ability of arteries to effectively expand and contract in response to changes in blood pressure (BP).18 This physiological characteristic plays a pivotal role in the overall health of the cardiovascular system.19 Healthy arteries are characterized by their elasticity, allowing them to stretch and accommodate the surge of blood flow from the heart during systole and then recoil during diastole to maintain continuous blood circulation. However, as individuals age or develop certain medical conditions such as hypertension, chronic inflammation or atherosclerosis, the arteries may lose their elasticity and become stiffer. This loss of compliance can be attributed to structural changes in the arterial walls, including increased collagen and reduced elastin content.20 The result is a reduced ability of the arteries to accommodate the pulsatile flow of blood, leading to higher systolic BP (SBP) and lower diastolic BP (DBP).21
Arterial stiffness is not merely a benign consequence of aging; rather, it is a significant contributor to cardiovascular diseases and associated complications. When the arteries lose their elasticity, the heart must exert greater force to pump blood efficiently, which can lead to increased cardiac workload and adverse effects on the cardiac structure and function over time.1922 Additionally, when arterial stiffness increases, the pulsatile energy from the heart cannot be adequately buffered, leading to a more direct impact on the target organs, which can result in organ damage occurring more frequently.23 Moreover, stiffened arteries can elevate SBP, potentially leading to hypertension, a major risk factor for various cardiovascular conditions, including stroke, coronary artery disease, and HF.24 Extensive research has unveiled its pivotal role as a predictor for future cardiovascular events, standing as a sentinel independent of conventional risk factors.2526 Given its crucial role in cardiovascular health, the assessment of arterial stiffness has gained significance in clinical practice.27
There are various methods available for measuring arterial stiffness, and the choice of measurement method may depend on specific research or clinical objectives, equipment availability, and the patient’s condition.18 Representative methods for assessing arterial stiffness include the following.
The most commonly employed method for assessing arterial stiffness in both clinical and research settings is PWV. PWV measures the speed at which the pressure wave generated by the heartbeat travels through the arteries, with a faster PWV indicating stiffer arteries.22 This measurement is typically obtained by positioning pressure sensors or cuffs at two distinct arterial locations, such as the carotid and femoral arteries, and recording the time it takes for the pressure wave to traverse this distance. Depending on the specific arterial points chosen for measurement, various types of PWVs can be determined. Among these, the two most widely used PWV measurements are carotid-femoral PWV (cfPWV) and brachial-ankle PWV (baPWV).27 cfPWV, a long-established measurement method originating from the Western medical community, has amassed extensive data and is widely regarded as the gold standard for noninvasive arterial stiffness assessment.28 Given its focus on the elastic artery segment between the carotid and femoral arteries, cfPWV theoretically provides a measurement that closely aligns with the fundamental concept of arterial stiffness. However, it is important to note that the successful measurement of cfPWV necessitates a high level of skill on the part of the examiner when positioning the probe accurately on both the carotid and femoral arteries. This procedure can cause discomfort for patients. Consequently, the test tends to be time-consuming, and individuals with obesity may encounter difficulties when undergoing this assessment.2224
Conversely, baPWV, developed in Japan as a relative newcomer compared to cfPWV, has seen its popularity steadily rise among Asian populations in recent times.27 baPWV stands out as an exceptionally straightforward measurement technique, involving the application of a BP cuff around the upper arm and ankle. Importantly, this method does not induce discomfort in patients and demands minimal expertise from the examiner.24 While it does include certain muscular artery segments between the upper arm and ankle, it boasts a strong correlation with cfPWV29 and invasive measurements,30 supported by a substantial body of clinical outcome data.25
PP and the AIx are measurements used to assess the extent of reflected waves in the arterial system, which can serve as indicators of arterial stiffness.31 PP is defined as the difference between SBP and DBP, while the AIx is determined by the augmentation pressure divided by the PP. Augmentation pressure can be measured using various non-invasive methods, including tonometry.32 In blood vessels characterized by increased arterial stiffness, peripheral arterial reflected waves travel at a higher speed and merge prematurely with the forward waves originating from the heart. Consequently, SBP increases while DBP decreases, leading to elevated PP and AIx.33 Many studies have shown that these measures are associated with an increased risk of cardiovascular events, including ischemic heart disease and stroke.34353637
Arterial compliance refers to how easily an artery expands for a given pressure. As arterial stiffness escalates, compliance diminishes, leading to inadequate stretching in response to the SBP generated by the LV during systole.38 β-stiffness index is calculated from BP and arterial dimensions using the formula: β-stiffness = ln (SBP/DBP) × D/∆D, where D represents the arterial diameter, and ∆D is the change in arterial diameter corresponding to PP.39 A higher β-stiffness index indicates decreased arterial compliance, reflecting stiffer arteries. To assess the extent of artery wall deformation based on the contraction and relaxation of the LV, imaging tests such as ultrasound, computed tomography, and magnetic resonance imaging can be employed.18 However, this approach demands image acquisition for measurement, which consumes both time and resources. Additionally, measuring compliance requires technical proficiency, making it a relatively less common method for assessing arterial stiffness. Additionally, arterial compliance and the β-stiffness index have the disadvantage of reflecting only the characteristics of specific local arteries.
PWV is affected by BP, making it difficult to maintain consistency even after measurement. To address this issue, the CAVI has been developed, based on the β-stiffness index.40 CAVI is calculated using the Bramwell-Hill equation, which corrects for BP, thus reported to be a more reproducible indicator.41 Additionally, measuring arterial stiffness through CAVI has the advantage of reflecting both the state of smooth muscle contraction and the mechanical properties of the arterial wall.42
The most precise method for assessing arterial stiffness involves the direct measurement of hemodynamic indicators by inserting a catheter into the artery.18 Several studies have reported associations between invasively measured aortic arterial stiffness indices and target organ damage as well as adverse cardiovascular events in patients undergoing invasive coronary angiography for the diagnosis and treatment of coronary artery disease.2143 However, this approach is challenging to implement in a typical medical setting due to its invasiveness and cost.
The pathophysiology of HFpEF remains incompletely understood and continues to be a subject of active research. Nevertheless, several pivotal mechanisms and contributing factors have been elucidated.
A prominent feature of HFpEF is the presence of LV diastolic dysfunction, which encompasses the relaxation and filling of the LV during the cardiac cycle.14445 Diastolic dysfunction results in heightened stiffness of the ventricular walls, diminishing their capacity to relax and accommodate blood. Consequently, this leads to elevated LV filling pressures, which can contribute significantly to the manifestation of HF symptoms.4446 Chronic pressure overload, frequently attributed to conditions such as hypertension, can precipitate LV hypertrophy and fibrosis. These structural alterations further exacerbate diastolic dysfunction and play a role in the onset of HF symptoms.4748 In HFpEF patients, the increase in LV filling pressure due to diastolic dysfunction escalates more rapidly during exercise. This escalation results in pulmonary hypertension and exacerbates dyspnea during physical exertion.45
Chronic inflammation and endothelial dysfunction are also prevalent in HFpEF. These factors can disrupt nitric oxide (NO) signaling, a crucial component for vasodilation and the regulation of blood flow. Endothelial dysfunction further contributes to vascular stiffness and diminished coronary blood flow, placing additional strain on the heart.4950
HFpEF is commonly accompanied by a range of comorbid conditions, including obesity, diabetes, chronic kidney disease, and atrial fibrillation. These comorbidities contribute to the development and progression of HFpEF through diverse mechanisms, including inflammation, oxidative stress, and metabolic disturbances.4951
Neurohormonal imbalances, marked by heightened sympathetic nervous system activity and activation of the RAAS, may also play a role in HFpEF. These neurohormonal shifts induce vasoconstriction, sodium and water retention, and an augmented workload for the heart.52
Furthermore, arterial stiffness emerges as another noteworthy risk factor for HFpEF.53 Age-related changes and comorbid conditions like hypertension and diabetes contribute to increased arterial stiffness.22 These stiffened arteries diminish the arterial system’s ability to absorb the force generated by the heart during systole, resulting in elevated afterload on the heart. This can exacerbate diastolic dysfunction and contribute significantly to the development and progression of HFpEF.54 As we move forward, further exploration of how arterial stiffness contributes to the pathogenesis of HFpEF will be instrumental in advancing our understanding of this complex condition.
Hypertension significantly contributes to the development of HFpEF by inducing LV hypertrophy, which in turn leads to LV diastolic dysfunction. The prevalence of hypertension in patients with HFpEF is approximately 60–80%.55 There is a close mutual association between arterial stiffness and hypertension.24 Sustained high BP causes pressure damage to the vessel walls, induces an increased inflammatory response or oxidative stress, activates the RAAS, and leads to endothelial dysfunction.24 Additionally, hypertension results in the destruction of elastic fibers in the arterial wall and an increase in the synthesis of collagen fibers, promoting fibrosis.36 All these factors increase arterial stiffness. Conversely, an increase in arterial stiffness leads to hypertension. In vessels with increased arterial stiffness, the loss of buffering capacity prevents the reduction of the force ejected by the heart during systole, thereby increasing systolic pressure.56 Furthermore, in vessels with increased arterial stiffness, the speed of the wave reflecting back from the periphery is accelerated, merging early with the forward wave from the heart, which raises systolic pressure and lowers diastolic pressure. Thus, ultimately, it also increases PP (systolic pressure − diastolic pressure).53
LV diastolic dysfunction is considered a primary contributing factor and a precursor to HFpEF. The relationship between arterial stiffness and LV diastolic dysfunction has been a subject of extensive research. In a study involving 48 healthy American subjects, Borlaug et al.57 reported a significant correlation between effective arterial elastance of carotid artery and cfPWV with septal e' measured by tissue Doppler of the LV. Additionally, in a study conducted with 1,929 individuals in Shanghai, China, the presence of cardiac diastolic dysfunction, as assessed by echocardiography, was associated with higher baPWV values (1,675 vs. 1,456 cm/s). In the same study, the authors observed a significant correlation between baPWV and the E/A ratio.58 A longitudinal study conducted in Europe investigated 607 community-dwelling individuals, and it found that the progression of LV diastolic dysfunction over 4.7 years was associated with a higher baseline cfPWV.59 Our research group has also conducted several studies, revealing a significant correlation between the indices of LV diastolic function and arterial stiffness in relatively healthy individuals.606162 The association between arterial stiffness and LV diastolic dysfunction has also been substantiated in patients with specific medical conditions. In a study conducted in Japan involving 100 patients diagnosed with coronary artery disease or HF, a notable correlation between the CAVI and E/e′ was established.63 Additionally, Einarsen et al.64 analyzed 260 Europeans who had experienced an acute stroke and demonstrated that cfPWV was independently linked to LV diastolic dysfunction. In patients with acute myocardial infarction, there was a significant correlation between arterial stiffness measured using the digital volume pulse stiffness index and E/e′.65 In line with these findings, our research team has demonstrated significant correlations between indices of arterial stiffness, measured both invasively and noninvasively, primarily in patients with suspected or diagnosed coronary artery disease, and indices of LV diastolic function.666768
Collectively, a significant correlation exists between increased arterial stiffness and LV diastolic dysfunction. Given that LV diastolic dysfunction represents a fundamental and crucial pathophysiological factor in HFpEF, an elevation in arterial stiffness significantly contributes to the development of HFpEF.
Several studies have reported the association between arterial stiffness and HFpEF. A study conducted in Japan compared 60 HFpEF patients with 51 controls and found that baPWV was significantly higher in the HFpEF group.69 Another study in Japan showed that the CAVI value was significantly elevated in hospitalized HFpEF patients compared to the control group. Additionally, the authors reported that an increased CAVI value was independently associated with the hospitalization of HFpEF patients.70 In a study conducted on Koreans by our research team with similar findings, baPWV was significantly increased in HFpEF patients with a history of hospitalization compared to the control group (1,909 vs. 1,661 cm/sec).71 Reddy et al.72 assessed central aorta compliance by invasively obtaining radial artery waveforms in 98 patients with HFpEF and 22 patients with hypertension. The study findings indicated that HFpEF patients, when compared to hypertensive patients, exhibited reduced arterial compliance, which correlated with increased LV filling pressure. Furthermore, arterial compliance decreased even further during exercise in HFpEF patients compared to hypertensive controls.72 Similarly, a study conducted in France showed that during exercise, aortic PWV increased by 20% in HFpEF patients compared to a 7% decrease in the control group. This led to impaired adaptation of proximal ventriculoarterial coupling.73 These findings support the hypothesis that in HFpEF patients, arterial stiffness increases further during exercise, resulting in elevated LV filling pressure and subsequently more pronounced dyspnea.74 It has been reported that arterial stiffness, as measured by aortic PWV, AIx, and β-stiffness index, inversely correlates with survival in patients hospitalized and discharged with HF, with 35% being HFpEF cases.75
Tokitsu et al.76 followed 502 patients with HFpEF for approximately 3 years and found that the total cardiovascular event rate increased when the baPWV value was < 1,300 cm/s or > 1,900 cm/s. These results suggest that assessing arterial stiffness could aid in predicting the prognosis of HFpEF.
HFpEF occurs more frequently in women than in men,819 and various different factors such as genetics, anthropometry, sex hormones, and clusters of risk factor are involved in a complex manner.77 It has been suggested that a stronger association between arterial stiffness and LV diastolic function may be another important mechanism contributing to this sex difference. Shim et al.78 demonstrated that aortic PP amplification, as measured by radial artery tonometry, was significantly correlated with an indicator of LV relaxation (septal e′ velocity) only in women compared to age-matched men. Kim et al.79 conducted invasive measurements of aortic PP in elderly patients undergoing coronary angiography. Their findings revealed that the associations between septal E/e′ and aortic PP, as well as LV end-diastolic pressure, were more pronounced in women compared to men.79 Another study showed that the associations of baPWV with septal e′ velocity and septal E/e′ were significant solely in elderly women, with no significant associations found in younger women and men.80 Arterial stiffness is a key factor in understanding the sex differences observed in HFpEF prevalence, highlighting the importance of considering vascular function in the pathophysiology of HFpEF.
The relationship between increased arterial stiffness, LV hypertrophy, LV diastolic dysfunction, and the ultimate development of HFpEF can be elucidated as follows (Fig. 1).22535481 Arterial stiffness plays a pivotal role in this pathophysiological cascade. When blood vessels exhibit increased arterial stiffness, the speed of reflected waves originating from the periphery accelerates. These waves merge prematurely with the forward-traveling waves generated by the heart, leading to a rise in SBP and a decline in DBP.53 This alteration in pressure dynamics places a heightened afterload on the LV, instigating LV hypertrophy as a compensatory mechanism. LV hypertrophy, although initially an adaptive response, eventually compromises LV diastolic function. Simultaneously, it restricts blood flow from the epicardial coronary artery, further aggravating LV diastolic dysfunction. The diminishing diastolic pressure can also impede coronary artery perfusion,82 exacerbating the deterioration of LV diastolic function. The association between increased arterial stiffness and HFpEF is reinforced by the prevalence of cardiovascular risk factors. Aging, hypertension, hyperglycemia, endothelial dysfunction, chronic inflammation, oxidative stress, and RAAS activation are all factors commonly linked to increased arterial stiffness and LV diastolic dysfunction.22 Increased arterial stiffness is often associated with endothelial dysfunction leading to a reduction of NO production.83 As NO is crucial role for vascular relaxation, its depletion contributes to impaired relation of the arteries thereby increasing the LV load. Pro-inflammatory cytokines such as tumor necrosis factor-α, interleukin-6, and C-reactive protein (CRP) can promote vascular remodeling and fibrosis, leading to stiffer arteries.84 These inflammatory markers also affect myocardial cells, contributing to myocardial fibrosis and impaired diastolic function. Increased oxidative stress is a common feature of arterial stiffness. Reactive oxygen species can damage endothelial cells, reduce NO availability, and promote inflammatory pathways, all of which contribute to vascular and myocardial stiffness.85 Activation of RAAS, which is common in arterial stiffness, leads to increased angiotensin II and aldosterone levels. These hormones promote vascular and myocardial fibrosis and hypertrophy, contributing to both arterial stiffness and diastolic dysfunction.86 These risk factors collectively contribute to the development and progression of the complex pathophysiology underpinning HFpEF.
As previously mentioned, arterial stiffness significantly contributes to the development of HFpEF. Therefore, treatment aimed at reducing arterial stiffness has become a focal point in the management of HFpEF.
Managing cardiovascular risk factors through lifestyle interventions is essential not only for reducing arterial stiffness but also for playing a critical and foundational role in treating patients with HFpEF.
Arterial stiffness can be influenced by commonly consumed dietary factors.87 For instance, red wine is known to decrease arterial stiffness,88 while caffeine has been found to increases it.89 Among the dietary factors that elevate arterial stiffness, salt intake warrants particular attention. Increased salt consumption has been associated with heightened arterial stiffness and BP.90 Some studies have demonstrated that a low-salt diet can ameliorate arterial stiffness.91 Furthermore, adherence to a low-salt diet has been shown to improve arterial stiffness in patients with HFpEF.9293 A study involving 13 hypertensive HFpEF patients revealed that a 21-day sodium-restricted Dietary Approaches to Stop Hypertension diet significantly reduced BP, arterial stiffness, and oxidative stress, as evidenced by a decrease in urinary F2-isoprostanes,92 in addition to improvements in LV diastolic function and ventricular-arterial coupling.93
Regular aerobic exercise is known to decrease arterial stiffness by improving endothelial function, lowering BP, and reducing inflammation.94 Additionally, exercise training enhances cardiopulmonary function, exercise tolerance, and quality of life in HFpEF patients.95 HFpEF and arterial stiffness share common risk factors, including hypertension, diabetes, dyslipidemia, and obesity,22 all of which can be mitigated through regular physical activity. Although direct evidence is lacking that exercise specifically improves exercise capacity or prognosis in HFpEF patients by targeting arterial stiffness, such improvements may be inferred based on existing knowledge.
Medications known to reduce arterial stiffness span multiple classes, each targeting different aspects of cardiovascular physiology.96 Antihypertensive drugs work primarily by lowering BP and improving arterial compliance.24 A pooled analysis of 5 trials with 469 patients showed angiotensin-converting enzyme inhibitors (ACEIs) significantly reduced pulse PWV compared to placebo.97 In another analysis of 7 trials, ACEIs significantly lowered AIx when compared with BBs.97 A meta-analysis involving 3,309 participants across 53 clinical trials found significant reductions in cfPWV and baPWV with angiotensin-receptor blocker (ARB) treatment, with reductions averaging −42.5 cm/s for cfPWV and −107.0 cm/s for baPWV.98 BBs also improved PWV and PP compared to placebo but were less potent than ACEIs and ARBs.99 Conversely, a meta-analysis of eight studies found no difference between ACEIs and atenolol in reducing PWV.100 Yen et al.101 demonstrated no significant difference in the reduction of arterial stiffness among ACEIs, ARBs and CCBs. Another study showed that the effect of ARB on the improvement of the PWV level is not superior to CCBs and BBs.102 According to meta-analysis including 28 included, no significant difference in PWV was observed among various antihypertensive medications.103 Although diuretics were most effective in preventing HF in patients with hypertension, the effect was also observed with RAAS blockers.104105 In summary, among hypertension drugs, RAAS blockers appear to be more effective in reducing arterial stiffness, while BBs seem to have relatively less efficacy in this regard. Nonetheless, it is worth noting that some studies indicate no significant difference between various antihypertensive drugs. Therefore, additional research will be necessary in the future to further explore this topic.
Statins improve arterial stiffness through their anti-inflammatory actions and enhancement of endothelial function.106 In a meta-analysis of 6 randomized controlled trials (RCTs), various statins including simvastatin, atorvastatin, rosuvastatin, lovastatin, and fluvastatin significantly reduced aortic PWV by 2.31 standardized mean difference compared to control or placebo groups.107 Another meta-analysis of 18 trials demonstrated a significant reduction in aortic AIx following statin therapy (weighted mean difference, −2.40%).108 Similarly, D’elia et al.109 conducted a meta-analysis of 11 studies, revealing a 6.8% reduction in PWV associated with statin therapy, independent of changes in BP and lipid profiles. Furthermore, a retrospective cohort study involving 5,105 Chinese adults found that statin users had significantly lower baseline baPWV and slower progression over 4.8 years compared to non-statin users.110 However, in contrast, a study analyzing 1,242 participants in the Multi-Ethnic Study of Atherosclerosis cohort reported no change in carotid artery stiffness, as measured by carotid ultrasound, after 9.4 years, regardless of therapy duration or statin intensity.111 Collectively, the evidence suggests that statin treatment can improve arterial stiffness, supported by multiple studies. Nevertheless, the limited sample size and a shortage of well-designed RCTs warrant the need for larger studies with robust designs to strengthen the existing evidence base.
In diabetes management, sodium-glucose cotransporter 2 inhibitors and glucagon-like peptide-1 receptor agonists not only facilitate glucose control but also exhibit potential in reducing arterial stiffness, which is attributed to weight loss and anti-inflammatory properties. An animal study demonstrated that treatment with dapagliflozin significantly lowered aortic PWV in diabetic mice.112 In human studies, a 2-day treatment with dapagliflozin in type 2 diabetic patients was shown to improve cfPWV as well as oxidative stress and endothelial function.113 Furthermore, a study involving 58 type 2 diabetic patients revealed that empagliflozin ameliorates arterial stiffness, as evidenced by reduced central SBP and PP, along with decreased forward and reflected wave amplitude. The analysis identified that, apart from age and sex, reductions in systolic 24-hour ambulatory BP and high-sensitivity CRP levels were significant determinants of the improvement, underscoring empagliflozin’s potential anti-inflammatory impact on vascular health.114 Additionally, Chilton et al.115 reported that a 12-week treatment with empagliflozin in type 2 diabetic patients significantly reduced the ambulatory arterial stiffness index and PP. In a RCT, liraglutide was shown to improve cfPWV more than metformin after 6 months of treatment in patients with type 2 diabetes.116 Combination therapy with empagliflozin and liraglutide demonstrated an even greater improvement in PWV in patients with type 2 diabetes.117118
Most studies that have reported results regarding drugs improving arterial stiffness are small-scale and have not been conducted on patients with HFpEF. Additional research is needed to determine the specific effects of these drugs on arterial stiffness and their effectiveness in HFpEF patients.
Increased arterial stiffness is closely associated with LV diastolic dysfunction, a precursor to HFpEF. Arterial stiffness is linked with cardiovascular risk factors prevalent in HFpEF, including hypertension, diabetes, and obesity. Patients with HFpEF exhibit greater arterial stiffness compared to those without HFpEF. Consequently, assessing arterial stiffness helps identify individuals at high risk for HFpEF, facilitating early intervention. Moreover, measuring arterial stiffness aids in the diagnosis and monitoring of HFpEF, with temporal changes indicating disease progression or response to treatment. Improving arterial stiffness through lifestyle changes and pharmacological treatments may enhance LV diastolic function. Parameters of arterial stiffness offer prognostic value in HFpEF, independently predicting adverse outcomes and mortality. Incorporating assessments of arterial stiffness into risk stratification could guide personalized management for HFpEF patients.
Currently, various indicators of arterial stiffness are utilized. There is a need for the development of more sensitive and specific biomarkers or imaging modalities to accurately assess arterial stiffness and its impact on HFpEF. Additionally, further studies are required to elucidate the underlying mechanisms linking arterial stiffness to HFpEF. This involves researching the molecular and cellular pathways that contribute to both arterial stiffening and the pathogenesis of HFpEF, which could identify novel therapeutic targets. Conducting longitudinal studies is also necessary to better understand the temporal relationship between changes in arterial stiffness and the development or progression of HFpEF. This can assist in identifying critical periods where interventions could be most effective. Investigating and developing new pharmacological treatments that directly target the mechanisms of arterial stiffness and its effects on the heart is crucial. Further research into the role of lifestyle interventions, such as diet, exercise, and weight management, in reducing arterial stiffness and improving outcomes in HFpEF patients is also important. This includes exploring the potential for preventive strategies in at-risk populations. Developing and validating models that integrate measures of arterial stiffness with other clinical and biomarker data to improve risk stratification, prognostication, and management of HFpEF patients is essential. By focusing on these areas, future research and clinical efforts can significantly advance our understanding and management of the complex relationship between arterial stiffness and HFpEF, ultimately leading to better patient outcomes.
Current evidence suggests that arterial stiffness influences HFpEF development and that information on arterial stiffness could be used in the diagnosis, treatment monitoring, and risk stratification of HFpEF. Recognizing and addressing arterial stiffness in clinical practice can have important implications for risk assessment, treatment selection, and patient outcomes in HFpEF. However, further studies are required to substantiate the value of interventions targeting arterial stiffness in HFpEF.
References
1. McDonagh TA, Metra M, Adamo M, Gardner RS, Baumbach A, Böhm M, et al. 2021 ESC guidelines for the diagnosis and treatment of acute and chronic heart failure. Eur Heart J. 2021; 42(36):3599–3726. PMID: 34447992.
2. Tsao CW, Aday AW, Almarzooq ZI, Anderson CAM, Arora P, Avery CL, et al. Heart disease and stroke statistics-2023 update: a report from the American Heart Association. Circulation. 2023; 147(8):e93–e621. PMID: 36695182.
3. Krumholz HM, Chen YT, Wang Y, Vaccarino V, Radford MJ, Horwitz RI. Predictors of readmission among elderly survivors of admission with heart failure. Am Heart J. 2000; 139(1 Pt 1):72–77. PMID: 10618565.
4. Hu SS, Kong LZ, Gao RL, Zhu ML, Wang W, Wang YJ, et al. Outline of the report on cardiovascular disease in China, 2010. Biomed Environ Sci. 2012; 25(3):251–256. PMID: 22840574.
5. Okura Y, Ramadan MM, Ohno Y, Mitsuma W, Tanaka K, Ito M, et al. Impending epidemic: future projection of heart failure in Japan to the year 2055. Circ J. 2008; 72(3):489–491. PMID: 18296852.
6. Lee JH, Lim NK, Cho MC, Park HY. Epidemiology of heart failure in Korea: present and future. Korean Circ J. 2016; 46(5):658–664. PMID: 27721857.
7. Park JJ, Choi DJ. Current status of heart failure: global and Korea. Korean J Intern Med. 2020; 35(3):487–497. PMID: 32392657.
8. Owan TE, Hodge DO, Herges RM, Jacobsen SJ, Roger VL, Redfield MM. Trends in prevalence and outcome of heart failure with preserved ejection fraction. N Engl J Med. 2006; 355(3):251–259. PMID: 16855265.
9. Bhatia RS, Tu JV, Lee DS, Austin PC, Fang J, Haouzi A, et al. Outcome of heart failure with preserved ejection fraction in a population-based study. N Engl J Med. 2006; 355(3):260–269. PMID: 16855266.
10. Meta-analysis Global Group in Chronic Heart Failure (MAGGIC). The survival of patients with heart failure with preserved or reduced left ventricular ejection fraction: an individual patient data meta-analysis. Eur Heart J. 2012; 33(14):1750–1757. PMID: 21821849.
11. McMurray JJ. Clinical practice. Systolic heart failure. N Engl J Med. 2010; 362(3):228–238. PMID: 20089973.
12. Sutton MG, Sharpe N. Left ventricular remodeling after myocardial infarction: pathophysiology and therapy. Circulation. 2000; 101(25):2981–2988. PMID: 10869273.
13. Berk BC, Fujiwara K, Lehoux S. ECM remodeling in hypertensive heart disease. J Clin Invest. 2007; 117(3):568–575. PMID: 17332884.
14. Hartupee J, Mann DL. Neurohormonal activation in heart failure with reduced ejection fraction. Nat Rev Cardiol. 2017; 14(1):30–38. PMID: 27708278.
15. Youn JC, Ahn Y, Jung HO. Pathophysiology of heart failure with preserved ejection fraction. Heart Fail Clin. 2021; 17(3):327–335. PMID: 34051965.
16. Shim CY. Heart failure with preserved ejection fraction: the major unmet need in cardiology. Korean Circ J. 2020; 50(12):1051–1061. PMID: 33150751.
17. Tucker WD, Arora Y, Mahajan K. Anatomy, blood vessels. StatPearls. Treasure Island, FL, USA: StatPearls Publishing;2024.
18. Cavalcante JL, Lima JA, Redheuil A, Al-Mallah MH. Aortic stiffness: current understanding and future directions. J Am Coll Cardiol. 2011; 57(14):1511–1522. PMID: 21453829.
19. Chirinos JA, Segers P, Hughes T, Townsend R. Large-artery stiffness in health and disease: JACC state-of-the-art review. J Am Coll Cardiol. 2019; 74(9):1237–1263. PMID: 31466622.
20. Lee HY, Oh BH. Aging and arterial stiffness. Circ J. 2010; 74(11):2257–2262. PMID: 20962429.
21. Lyle AN, Raaz U. Killing me unsoftly: causes and mechanisms of arterial stiffness. Arterioscler Thromb Vasc Biol. 2017; 37(2):e1–e11. PMID: 28122777.
22. Kim HL, Kim SH. Pulse wave velocity in atherosclerosis. Front Cardiovasc Med. 2019; 6:41. PMID: 31024934.
23. Tomiyama H, Yamashina A. Non-invasive vascular function tests: their pathophysiological background and clinical application. Circ J. 2010; 74(1):24–33. PMID: 19920359.
24. Kim HL. Arterial stiffness and hypertension. Clin Hypertens. 2023; 29(1):31. PMID: 38037153.
25. Ohkuma T, Ninomiya T, Tomiyama H, Kario K, Hoshide S, Kita Y, et al. Brachial-ankle pulse wave velocity and the risk prediction of cardiovascular disease: an individual participant data meta-analysis. Hypertension. 2017; 69(6):1045–1052. PMID: 28438905.
26. Vlachopoulos C, Aznaouridis K, Stefanadis C. Prediction of cardiovascular events and all-cause mortality with arterial stiffness: a systematic review and meta-analysis. J Am Coll Cardiol. 2010; 55(13):1318–1327. PMID: 20338492.
27. Park JB, Sharman JE, Li Y, Munakata M, Shirai K, Chen CH, et al. Expert consensus on the clinical use of pulse wave velocity in Asia. Pulse (Basel). 2022; 10(1-4):1–18. PMID: 36660436.
28. Laurent S, Cockcroft J, Van Bortel L, Boutouyrie P, Giannattasio C, Hayoz D, et al. Expert consensus document on arterial stiffness: methodological issues and clinical applications. Eur Heart J. 2006; 27(21):2588–2605. PMID: 17000623.
29. Kollias A, Kyriakoulis KG, Gravvani A, Anagnostopoulos I, Stergiou GS. Automated pulse wave velocity assessment using a professional oscillometric office blood pressure monitor. J Clin Hypertens (Greenwich). 2020; 22(10):1817–1823. PMID: 32762109.
30. Kang J, Kim HL, Lim WH, Seo JB, Zo JH, Kim MA, et al. Relationship between brachial-ankle pulse wave velocity and invasively measured aortic pulse pressure. J Clin Hypertens (Greenwich). 2018; 20(3):462–468. PMID: 29370481.
31. Dart AM, Kingwell BA. Pulse pressure--a review of mechanisms and clinical relevance. J Am Coll Cardiol. 2001; 37(4):975–984. PMID: 11263624.
32. Kim HL, Koo BK, Joo SK, Kim W. Association of arterial stiffness with the histological severity of nonalcoholic fatty liver disease. Hepatol Int. 2020; 14(6):1048–1056. PMID: 33269420.
33. Weber T. The role of arterial stiffness and central hemodynamics in heart failure. Int J Heart Fail. 2020; 2(4):209–230. PMID: 36262174.
34. Domanski M, Norman J, Wolz M, Mitchell G, Pfeffer M. Cardiovascular risk assessment using pulse pressure in the first national health and nutrition examination survey (NHANES I). Hypertension. 2001; 38(4):793–797. PMID: 11641288.
35. Moyá-Amengual A, Ruiz-García A, Pallarés-Carratalá V, Serrano-Cumplido A, Prieto-Díaz MÁ, Segura-Fragoso A, et al. Elevated pulse pressure and cardiovascular risk associated in Spanish population attended in primary care: IBERICAN study. Front Cardiovasc Med. 2023; 10:1090458. PMID: 37229234.
36. Nürnberger J, Keflioglu-Scheiber A, Opazo Saez AM, Wenzel RR, Philipp T, Schäfers RF. Augmentation index is associated with cardiovascular risk. J Hypertens. 2002; 20(12):2407–2414. PMID: 12473865.
37. Betge S, Kretzschmar D, Figulla HR, Lichtenauer M, Jung C. Predictive value of the augmentation index derived vascular age in patients with newly diagnosed atherosclerosis. Heart Vessels. 2017; 32(3):252–259. PMID: 27401737.
38. Morioka T, Mori K, Emoto M. Is stiffness parameter β useful for the evaluation of atherosclerosis?~ its clinical implications, limitations, and future perspectives ~. J Atheroscler Thromb. 2021; 28(5):435–453. PMID: 33583910.
39. Hayashi K, Handa H, Nagasawa S, Okumura A, Moritake K. Stiffness and elastic behavior of human intracranial and extracranial arteries. J Biomech. 1980; 13(2):175–184. PMID: 7364778.
40. Shirai K, Hiruta N, Song M, Kurosu T, Suzuki J, Tomaru T, et al. Cardio-ankle vascular index (CAVI) as a novel indicator of arterial stiffness: theory, evidence and perspectives. J Atheroscler Thromb. 2011; 18(11):924–938. PMID: 21628839.
41. Shirai K, Utino J, Otsuka K, Takata M. A novel blood pressure-independent arterial wall stiffness parameter; cardio-ankle vascular index (CAVI). J Atheroscler Thromb. 2006; 13(2):101–107. PMID: 16733298.
42. Sun CK. Cardio-ankle vascular index (CAVI) as an indicator of arterial stiffness. Integr Blood Press Control. 2013; 6:27–38. PMID: 23667317.
43. Hametner B, Wassertheurer S, Mayer CC, Danninger K, Binder RK, Weber T. Aortic pulse wave velocity predicts cardiovascular events and mortality in patients undergoing coronary angiography: a comparison of invasive measurements and noninvasive estimates. Hypertension. 2021; 77(2):571–581. PMID: 33390046.
44. Obokata M, Reddy YNV, Borlaug BA. Diastolic dysfunction and heart failure with preserved ejection fraction: understanding mechanisms by using noninvasive methods. JACC Cardiovasc Imaging. 2020; 13(1 Pt 2):245–257. PMID: 31202759.
45. Borlaug BA. The pathophysiology of heart failure with preserved ejection fraction. Nat Rev Cardiol. 2014; 11(9):507–515. PMID: 24958077.
46. Bianco CM, Farjo PD, Ghaffar YA, Sengupta PP. Myocardial mechanics in patients with normal LVEF and diastolic dysfunction. JACC Cardiovasc Imaging. 2020; 13(1 Pt 2):258–271. PMID: 31202770.
47. Tan W, Li X, Zheng S, Li X, Zhang X, Pyle WG, et al. A porcine model of heart failure with preserved ejection fraction induced by chronic pressure overload characterized by cardiac fibrosis and remodeling. Front Cardiovasc Med. 2021; 8:677727. PMID: 34150870.
48. Messerli FH, Rimoldi SF, Bangalore S. The transition from hypertension to heart failure: contemporary update. JACC Heart Fail. 2017; 5(8):543–551. PMID: 28711447.
49. Paulus WJ, Tschöpe C. A novel paradigm for heart failure with preserved ejection fraction: comorbidities drive myocardial dysfunction and remodeling through coronary microvascular endothelial inflammation. J Am Coll Cardiol. 2013; 62(4):263–271. PMID: 23684677.
50. Franssen C, Chen S, Unger A, Korkmaz HI, De Keulenaer GW, Tschöpe C, et al. Myocardial microvascular inflammatory endothelial activation in heart failure with preserved ejection fraction. JACC Heart Fail. 2016; 4(4):312–324. PMID: 26682792.
51. van Heerebeek L, Paulus WJ. Understanding heart failure with preserved ejection fraction: where are we today? Neth Heart J. 2016; 24(4):227–236. PMID: 26909795.
52. Lim GB. Neurohormonal activation in HFpEF. Nat Rev Cardiol. 2019; 16(12):700.
53. Weber T, Chirinos JA. Pulsatile arterial haemodynamics in heart failure. Eur Heart J. 2018; 39(43):3847–3854. PMID: 29947746.
54. Mottram PM, Haluska BA, Leano R, Carlier S, Case C, Marwick TH. Relation of arterial stiffness to diastolic dysfunction in hypertensive heart disease. Heart. 2005; 91(12):1551–1556. PMID: 16287739.
55. Lam CS, Donal E, Kraigher-Krainer E, Vasan RS. Epidemiology and clinical course of heart failure with preserved ejection fraction. Eur J Heart Fail. 2011; 13(1):18–28. PMID: 20685685.
56. O’Rourke MF, Hashimoto J. Mechanical factors in arterial aging: a clinical perspective. J Am Coll Cardiol. 2007; 50(1):1–13. PMID: 17601538.
57. Borlaug BA, Melenovsky V, Redfield MM, Kessler K, Chang HJ, Abraham TP, et al. Impact of arterial load and loading sequence on left ventricular tissue velocities in humans. J Am Coll Cardiol. 2007; 50(16):1570–1577. PMID: 17936156.
58. Kang S, Fan HM, Li J, Fan LY, Miao AY, Bao Y, et al. Relationship of arterial stiffness and early mild diastolic heart failure in general middle and aged population. Eur Heart J. 2010; 31(22):2799–2807. PMID: 20797980.
59. Cauwenberghs N, Knez J, D’hooge J, Thijs L, Yang WY, Wei FF, et al. Longitudinal changes in LV structure and diastolic function in relation to arterial properties in general population. JACC Cardiovasc Imaging. 2017; 10(11):1307–1316. PMID: 28330663.
60. Kim HL, Im MS, Seo JB, Chung WY, Kim SH, Kim MA, et al. The association between arterial stiffness and left ventricular filling pressure in an apparently healthy Korean population. Cardiovasc Ultrasound. 2013; 11(1):2. PMID: 23302225.
61. Kim HL, Seo JB, Chung WY, Kim SH, Kim MA, Zo JH. Independent association between brachial-ankle pulse wave velocity and global longitudinal strain of left ventricle. Int J Cardiovasc Imaging. 2015; 31(8):1563–1570. PMID: 26298315.
62. Park KT, Kim HL, Oh S, Lim WH, Seo JB, Chung WY, et al. Association between reduced arterial stiffness and preserved diastolic function of the left ventricle in middle-aged and elderly patients. J Clin Hypertens (Greenwich). 2017; 19(6):620–626. PMID: 28194861.
63. Namba T, Masaki N, Matsuo Y, Sato A, Kimura T, Horii S, et al. Arterial stiffness is significantly associated with left ventricular diastolic dysfunction in patients with cardiovascular disease. Int Heart J. 2016; 57(6):729–735. PMID: 27829641.
64. Einarsen E, Gerdts E, Waje-Andreassen U, Naess H, Fromm A, Saeed S. Association of increased arterial stiffness with diastolic dysfunction in ischemic stroke patients: the Norwegian Stroke in the Young Study. J Hypertens. 2020; 38(3):467–473. PMID: 31725075.
65. Milewska A, Krauze T, Piskorski J, Minczykowski A, Wykrętowicz A, Guzik P. Association between high arterial stiffness and left ventricular filling pressures in patients with acute myocardial infarction. Pol Arch Med Wewn. 2015; 125(11):814–822. PMID: 26400572.
66. Kong MG, Kim HL, Kim MA, Kim M, Park SM, Yoon HJ, et al. Relationships between blood pressure measurements and target organ damage: data from the Korea women’s chest pain registry. J Clin Hypertens (Greenwich). 2018; 20(12):1724–1730. PMID: 30362256.
67. Kim HL, Seo JB, Chung WY, Kim SH, Kim MA, Zo JH. Association between invasively measured central aortic pressure and left ventricular diastolic function in patients undergoing coronary angiography. Am J Hypertens. 2015; 28(3):393–400. PMID: 25125636.
68. Kim KJ, Kim HL, Kang DY, Park SH, Lim WH, Seo JB, et al. Correlations between invasively measured aortic pressures and left ventricular end-diastolic pressure in patients undergoing coronary angiography. Blood Press Monit. 2019; 24(5):241–247. PMID: 31490246.
69. Aizawa Y, Okumura Y, Saito Y, Ikeya Y, Nakai T, Arima K. Association of renal resistance index and arterial stiffness on clinical outcomes in patients with mild-to-moderate renal dysfunction and presence or absence of heart failure with preserved ejection fraction. Heart Vessels. 2020; 35(12):1699–1708. PMID: 32591893.
70. Takagi K, Ishihara S, Kenji N, Iha H, Kobayashi N, Ito Y, et al. Clinical significance of arterial stiffness as a factor for hospitalization of heart failure with preserved left ventricular ejection fraction: a retrospective matched case-control study. J Cardiol. 2020; 76(2):171–176. PMID: 32268988.
71. Kim HL, Chung J, Han S, Joh HS, Lim WH, Seo JB, et al. Arterial stiffness and its associations with left ventricular diastolic function according to heart failure types. Clin Hypertens. 2023; 29(1):8. PMID: 36918917.
72. Reddy YNV, Andersen MJ, Obokata M, Koepp KE, Kane GC, Melenovsky V, et al. Arterial stiffening with exercise in patients with heart failure and preserved ejection fraction. J Am Coll Cardiol. 2017; 70(2):136–148. PMID: 28683960.
73. Tartière-Kesri L, Tartière JM, Logeart D, Beauvais F, Cohen Solal A. Increased proximal arterial stiffness and cardiac response with moderate exercise in patients with heart failure and preserved ejection fraction. J Am Coll Cardiol. 2012; 59(5):455–461. PMID: 22281248.
74. Kitzman DW, Herrington DM, Brubaker PH, Moore JB, Eggebeen J, Haykowsky MJ. Carotid arterial stiffness and its relationship to exercise intolerance in older patients with heart failure and preserved ejection fraction. Hypertension. 2013; 61(1):112–119. PMID: 23150511.
75. Anastasio F, Testa M, Ferreri C, Rossi A, Ruocco G, Feola M. The analysis of arterial stiffness in heart failure patients: the prognostic role of pulse wave velocity, augmentation index and stiffness index. J Clin Med. 2022; 11(12):3507. PMID: 35743576.
76. Tokitsu T, Yamamoto E, Oike F, Hirata Y, Tsujita K, Yamamuro M, et al. Clinical significance of brachial-ankle pulse-wave velocity in patients with heart failure with preserved left ventricular ejection fraction. J Hypertens. 2018; 36(3):560–568. PMID: 29084082.
77. Beale AL, Meyer P, Marwick TH, Lam CSP, Kaye DM. Sex differences in cardiovascular pathophysiology: why women are overrepresented in heart failure with preserved ejection fraction. Circulation. 2018; 138(2):198–205. PMID: 29986961.
78. Shim CY, Park S, Choi D, Yang WI, Cho IJ, Choi EY, et al. Sex differences in central hemodynamics and their relationship to left ventricular diastolic function. J Am Coll Cardiol. 2011; 57(10):1226–1233. PMID: 21371640.
79. Kim KJ, Kim HL, Kim MJ, Kim CH, Lim WH, Seo JB, et al. Gender difference in the association between aortic pulse pressure and left ventricular filling pressure in the elderly: an invasive hemodynamic study. J Card Fail. 2017; 23(3):224–230. PMID: 28087427.
80. Kim HL, Lim WH, Seo JB, Chung WY, Kim SH, Kim MA, et al. Association between arterial stiffness and left ventricular diastolic function in relation to gender and age. Medicine (Baltimore). 2017; 96(1):e5783. PMID: 28072727.
81. Kim HL, Weber T. Pulsatile hemodynamics and coronary artery disease. Korean Circ J. 2021; 51(11):881–898. PMID: 34595882.
82. Tritakis V, Tzortzis S, Ikonomidis I, Dima K, Pavlidis G, Trivilou P, et al. Association of arterial stiffness with coronary flow reserve in revascularized coronary artery disease patients. World J Cardiol. 2016; 8(2):231–239. PMID: 26981218.
83. Wilkinson IB, Franklin SS, Cockcroft JR. Nitric oxide and the regulation of large artery stiffness: from physiology to pharmacology. Hypertension. 2004; 44(2):112–116. PMID: 15262901.
84. Jain S, Khera R, Corrales-Medina VF, Townsend RR, Chirinos JA. “Inflammation and arterial stiffness in humans”. Atherosclerosis. 2014; 237(2):381–390. PMID: 25463062.
85. Massaro M, Scoditti E, Carluccio MA, De Caterina R. Oxidative stress and vascular stiffness in hypertension: a renewed interest for antioxidant therapies? Vascul Pharmacol. 2019; 116:45–50. PMID: 30946986.
86. Neves MF, Cunha AR, Cunha MR, Gismondi RA, Oigman W. The role of renin-angiotensin-aldosterone system and its new components in arterial stiffness and vascular aging. High Blood Press Cardiovasc Prev. 2018; 25(2):137–145. PMID: 29476451.
87. Tanaka H, Safar ME. Influence of lifestyle modification on arterial stiffness and wave reflections. Am J Hypertens. 2005; 18(1):137–144. PMID: 15691628.
88. Mahmud A, Feely J. Divergent effect of acute and chronic alcohol on arterial stiffness. Am J Hypertens. 2002; 15(3):240–243. PMID: 11939614.
89. Vlachopoulos C, Hirata K, Stefanadis C, Toutouzas P, O’Rourke MF. Caffeine increases aortic stiffness in hypertensive patients. Am J Hypertens. 2003; 16(1):63–66. PMID: 12517685.
90. Salvi P, Giannattasio C, Parati G. High sodium intake and arterial stiffness. J Hypertens. 2018; 36(4):754–758. PMID: 29489612.
91. D’Elia L, Galletti F, La Fata E, Sabino P, Strazzullo P. Effect of dietary sodium restriction on arterial stiffness: systematic review and meta-analysis of the randomized controlled trials. J Hypertens. 2018; 36(4):734–743. PMID: 29084085.
92. Hummel SL, Seymour EM, Brook RD, Kolias TJ, Sheth SS, Rosenblum HR, et al. Low-sodium dietary approaches to stop hypertension diet reduces blood pressure, arterial stiffness, and oxidative stress in hypertensive heart failure with preserved ejection fraction. Hypertension. 2012; 60(5):1200–1206. PMID: 23033371.
93. Hummel SL, Seymour EM, Brook RD, Sheth SS, Ghosh E, Zhu S, et al. Low-sodium DASH diet improves diastolic function and ventricular-arterial coupling in hypertensive heart failure with preserved ejection fraction. Circ Heart Fail. 2013; 6(6):1165–1171. PMID: 23985432.
94. Tanaka H. Antiaging effects of aerobic exercise on systemic arteries. Hypertension. 2019; 74(2):237–243. PMID: 31256721.
95. Crisci G, De Luca M, D’Assante R, Ranieri B, D’Agostino A, Valente V, et al. Effects of exercise on heart failure with preserved ejection fraction: an updated review of literature. J Cardiovasc Dev Dis. 2022; 9(8):241. PMID: 36005405.
96. Janić M, Lunder M, Sabovič M. Arterial stiffness and cardiovascular therapy. BioMed Res Int. 2014; 2014:621437. PMID: 25170513.
97. Shahin Y, Khan JA, Chetter I. Angiotensin converting enzyme inhibitors effect on arterial stiffness and wave reflections: a meta-analysis and meta-regression of randomised controlled trials. Atherosclerosis. 2012; 221(1):18–33. PMID: 22209214.
98. Peng F, Pan H, Wang B, Lin J, Niu W. The impact of angiotensin receptor blockers on arterial stiffness: a meta-analysis. Hypertens Res. 2015; 38(9):613–620. PMID: 25854987.
99. Niu W, Qi Y. A meta-analysis of randomized controlled trials assessing the impact of beta-blockers on arterial stiffness, peripheral blood pressure and heart rate. Int J Cardiol. 2016; 218:109–117. PMID: 27232921.
100. Xie H, Luo G, Zheng Y, Peng F, Xie L. A meta-analytical comparison of atenolol with angiotensin-converting enzyme inhibitors on arterial stiffness, peripheral blood pressure and heart rate in hypertensive patients. Clin Exp Hypertens. 2017; 39(5):421–426. PMID: 28534649.
101. Yen CH, Lai YH, Hung CL, Lee PY, Kuo JY, Yeh HI, et al. Angiotensin receptor blockades effect on peripheral muscular and central aortic arterial stiffness: a meta-analysis of randomized controlled trials and systematic review. Zhonghua Minguo Xinzangxue Hui Zazhi. 2014; 30(2):98–107.
102. Chen X, Huang B, Liu M, Li X. Effects of different types of antihypertensive agents on arterial stiffness: a systematic review and meta-analysis of randomized controlled trials. J Thorac Dis. 2015; 7(12):2339–2347. PMID: 26793356.
103. Ye L, Yang X, Hu J, Chen Q, Wang J, Li X. Impact of antihypertensive agents on arterial stiffness in hypertensive patients. Int J Cardiol. 2018; 273:207–212. PMID: 29960763.
104. Sciarretta S, Palano F, Tocci G, Baldini R, Volpe M. Antihypertensive treatment and development of heart failure in hypertension: a Bayesian network meta-analysis of studies in patients with hypertension and high cardiovascular risk. Arch Intern Med. 2011; 171(5):384–394. PMID: 21059964.
105. Fretheim A, Odgaard-Jensen J, Brørs O, Madsen S, Njølstad I, Norheim OF, et al. Comparative effectiveness of antihypertensive medication for primary prevention of cardiovascular disease: systematic review and multiple treatments meta-analysis. BMC Med. 2012; 10(1):33. PMID: 22480336.
106. Alidadi M, Montecucco F, Jamialahmadi T, Al-Rasadi K, Johnston TP, Sahebkar A. Beneficial effect of statin therapy on arterial stiffness. BioMed Res Int. 2021; 2021:5548310. PMID: 33860033.
107. Upala S, Wirunsawanya K, Jaruvongvanich V, Sanguankeo A. Effects of statin therapy on arterial stiffness: a systematic review and meta-analysis of randomized controlled trial. Int J Cardiol. 2017; 227:338–341. PMID: 27839806.
108. Sahebkar A, Pećin I, Tedeschi-Reiner E, Derosa G, Maffioli P, Reiner Ž. Effects of statin therapy on augmentation index as a measure of arterial stiffness: a systematic review and meta-analysis. Int J Cardiol. 2016; 212:160–168. PMID: 27038725.
109. D’elia L, La Fata E, Iannuzzi A, Rubba PO. Effect of statin therapy on pulse wave velocity: a meta-analysis of randomized controlled trials. Clin Exp Hypertens. 2018; 40(7):601–608. PMID: 29420075.
110. Zhou YF, Wang Y, Wang G, Zhou Z, Chen S, Geng T, et al. Association between statin use and progression of arterial stiffness among adults with high atherosclerotic risk. JAMA Netw Open. 2022; 5(6):e2218323. PMID: 35713899.
111. Nechyporenko A, Tedla YG, Korcarz C, Tattersall MC, Greenland P, Gepner AD. Association of statin therapy with progression of carotid arterial stiffness: the Multi-Ethnic Study of Atherosclerosis (MESA). Hypertens Res. 2023; 46(3):679–687. PMID: 36434289.
112. Lee DM, Battson ML, Jarrell DK, Hou S, Ecton KE, Weir TL, et al. SGLT2 inhibition via dapagliflozin improves generalized vascular dysfunction and alters the gut microbiota in type 2 diabetic mice. Cardiovasc Diabetol. 2018; 17(1):62. PMID: 29703207.
113. Solini A, Giannini L, Seghieri M, Vitolo E, Taddei S, Ghiadoni L, et al. Dapagliflozin acutely improves endothelial dysfunction, reduces aortic stiffness and renal resistive index in type 2 diabetic patients: a pilot study. Cardiovasc Diabetol. 2017; 16(1):138. PMID: 29061124.
114. Bosch A, Ott C, Jung S, Striepe K, Karg MV, Kannenkeril D, et al. How does empagliflozin improve arterial stiffness in patients with type 2 diabetes mellitus? Sub analysis of a clinical trial. Cardiovasc Diabetol. 2019; 18(1):44. PMID: 30922297.
115. Chilton R, Tikkanen I, Cannon CP, Crowe S, Woerle HJ, Broedl UC, et al. Effects of empagliflozin on blood pressure and markers of arterial stiffness and vascular resistance in patients with type 2 diabetes. Diabetes Obes Metab. 2015; 17(12):1180–1193. PMID: 26343814.
116. Lambadiari V, Pavlidis G, Kousathana F, Varoudi M, Vlastos D, Maratou E, et al. Effects of 6-month treatment with the glucagon like peptide-1 analogue liraglutide on arterial stiffness, left ventricular myocardial deformation and oxidative stress in subjects with newly diagnosed type 2 diabetes. Cardiovasc Diabetol. 2018; 17(1):8. PMID: 29310645.
117. Ikonomidis I, Pavlidis G, Thymis J, Birba D, Kalogeris A, Kousathana F, et al. Effects of glucagon-like peptide-1 receptor agonists, sodium-glucose cotransporter-2 inhibitors, and their combination on endothelial glycocalyx, arterial function, and myocardial work index in patients with type 2 diabetes mellitus after 12-month treatment. J Am Heart Assoc. 2020; 9(9):e015716. PMID: 32326806.
118. Bechlioulis A, Markozannes G, Chionidi I, Liberopoulos E, Naka KK, Ntzani EE, et al. The effect of SGLT2 inhibitors, GLP1 agonists, and their sequential combination on cardiometabolic parameters: a randomized, prospective, intervention study. J Diabetes Complications. 2023; 37(4):108436. PMID: 36842186.