Abstract
The temporal fascia is a double lamina sandwiching a thick fat layer above the zygomatic bony arch. To characterize each lamina, their developmental processes were examined in fetuses. We observed histological sections from 22 half-heads of 10 mid-term fetuses at 14–18 weeks (crown-rump length, 95–150 mm) and 12 near-term fetuses at 26–40 weeks (crown-rump length, 215–334 mm). The superficial lamina of the temporal fascia was not evident at mid-term. Instead, a loose subcutaneous tissue was attached to the thin, deep lamina of the temporal fascia covering the temporalis muscle. At near-term, the deep lamina became thick, while the superficial lamina appeared and exhibited several variations: i) a mono-layered thick membrane (5 specimens); ii) a multi-layered membranous structure (6) and; iii) a cluster of independent thick fasciae each of which were separated by fatty tissues (1). In the second and third patterns, fatty tissue between the two laminae was likely to contain longitudinal fibrous bands in parallel with the deep lamina. Varying proportions of the multi-layered superficial lamina were not attached to the zygomatic arch, but extended below the bony arch. Whether or not lobulation or septation of fatty tissues was evident was not dependent on age. The deep lamina seemed to develop from the temporalis muscle depending on the muscle contraction. In contrast, the superficial lamina developed from subcutaneous collagenous bundles continuous to the cheek. Therein, a difference in development was clearly seen between two categories of the fasciae.
The temporal fascia is a double-lamina structure sandwiching a fatty tissue layer above the zygomatic bony arch and below the superior temporal line of the parietal bone [1-3], i.e., the superficial and deep laminae of the temporal facia (SLTF, DLTF). Because of its specific structure, the DLTF is a convenient and useful tissue for application to plastic and reconstructive surgery [4, 5]. In a morphometrical and histological study, Morales-Avalos et al. [6] demonstrated the advantages of the DLTF for graft use in terms of its fiber arrangement. Surprisingly, in living humans, an excellent ultrasound technique is able to demonstrate details of the multilaminar STFL and its individual variation [7].
Stuzin et al. [8] simply termed the SLTF and DLTF the “superficial temporal fascia and deep temporal fascia”, respectively. Likewise, Watanabe et al. [9] considered the SLTF to be the upper extension of the superficial musculoaponeurotic system defined by Mitz and Peyronie [10]: this membranous structure divides the subcutaneous fatty tissue into superficial and deep layers. Their insight led us to hypothesize that the SLTF develops as a superficial fascia in the subcutaneous tissue, while the DLTF develops as a muscle-covering fascia from the temporalis muscle. As an analogy to the latter, Cho et al. [11] have demonstrated a process whereby the multi-laminated femoral fascia develops from the quadriceps femoris muscle. Consequently, the aim of the present study was to investigate any development and growth differences between the SLTF and the DLTF. As the concept “deep fascia” includes several types of fasciae with quite different origins and developmental processes (for details, see the Discussion), we use the term “muscle-covering fascia” in this study.
The study was performed in accordance with the provisions of the Declaration of Helsinki 1995 (as revised in 2013). We used histological sections from 10 mid-term and 12 near-term human fetuses.
Paraffin-embedded serial sections of 10 mid-term fetus heads (approximately 14–18 weeks of gestational age [GA]; crown-rump length [CRL], 95–150 mm) were part of the large collection kept at the Department of Anatomy and Embryology of Complutense University, Madrid, the embryos having been obtained as a result of miscarriages and ectopic pregnancies at the Department of Obstetrics Complutense University. No information about the genetic background of the embryos and/or abortion was available. The sectional plane was frontal at a right angle to the zygomatic arch and, for each midterm-fetus, more than 2,000 sections contained an almost whole head: i.e., a large volume from the orbital roof to the mandibular base (supero-inferior axis) and from the eyelids to the internal jugular vein (antero-posterior axis). Therefore, we had used these sections for multiple previous studies: for instance, a fetus used for the present Fig. 1 (95 mm CRL) had been shown in Fig. 4 in a study of the palate and incisive bone [12]. The sections were stained with hematoxylin and eosin (H&E) or azan. This study was approved by the Ethics Committees of Complutense University (B08/374) and Tokyo Dental College (No. 932–2).
Semiserial sections from 12 near-term fetus heads (GA approximately 26–40 weeks; CRL 215–334 mm; 0.1-mm interval) had been previously prepared by our group for studies of the palate and sphenoid [12-14]. The near-term fetuses were part of the collection kept at the Department of Anatomy, Akita University, Akita, Japan. They had been donated by their families to the Department in 1975–1985 and preserved in 10% w/w neutral formalin solution for more than 30 years. Data for these specimens included the date of donation and the number of gestational weeks, but did not include the name of the family, obstetrician or hospital, or the reason for abortion. The use of these specimens for research was approved by the Akita University Ethics Committee (No. 1428). Before routine procedures for paraffin embedding, the fetal limb specimens were decalcified by incubating them at room temperature in Plank–Rychlo solution (AlCl2/6H2O, 7.0 w/v%; HCl, 3.6; HCOOH, 4.6) for 3–7 days. The sectional plane was frontal at right angle to the zygomatic arch and, for each near-term fetus, more than 200 sections contained an almost whole temporal region of the left or right half of the head: i.e., a 30- or 40-mm-cubic tissue mass from the zygomatic arch, via the temporomandibular joint, to the inner ear vestibule (antero-posterior axis) and from the temporal muscle to the mandibular lingula (supero-inferior axis). Therefore, using the same series of near-term fetus sections, two studies are now on review (one about the fossa mandibularis and another of Meckel’s cartilage remnant). The sections were stained with H&E or Masson trichrome. All photographs for histology were taken with a Nikon Eclipse 80 (Nikon).
In 10 mid-term fetuses with a GA of 14–18 weeks, the SLTF was not evident (Fig. 1). Instead, a loose subcutaneous tissue was attached to a muscle-covering fascia, i.e., the DLTF. Above the zygomatic arch, the temporalis muscle fibers originated from the DLTF and inserted to a thick intramuscular tendon. The attachment of muscle fibers to the DLTF tended to become evident with increased age (Fig. 1B vs. Fig. 1C). When the DLTF-attaching muscle fibers became dense, the fascia appeared to become clear and thick. Conversely, the DLTF was rather unclear immediately above the zygomatic arch (Fig. 1E) because of the relatively low density of muscle fibers attached to the fascia. Temporalis muscle fibers from the temporal bone squamosa also inserted to the intramuscular tendon.
In 12 near-term specimens at a GA of 26–40 weeks, the temporalis muscle was 3–4 times as thick as that at mid-term at the level of the zygomatic arch, but the increase in thickness was less than 2–3-fold in the subcutaneous tissue. The DLTF was thick and provided muscle origins. In contrast, the SLTF exhibited several variations in lamination: i) a mono-layered membrane (7 specimens; Fig. 2); ii) a multi-layered membranous structure or a thick bundle of thin membranes (4 specimens; Fig. 3) and; iii) a cluster of independent thick fasciae each of which were separated by fatty tissue (1=the largest specimen; Fig. 4). Thus, the majority or the first pattern appeared to correspond to a classical double-layered morphology (see Introduction), but it was seen in both small and large fetuses (Fig. 2A vs. Fig. 2B, C). Along the superficial side of the STLF, clusters of thin fibers tended to be seen together (Figs. 2B, 3C) and appeared to be miniature versions of the independent thick fasciae seen in the largest specimens.
For any of the patterns of the SLTF, fatty tissue between the SLTF and DLTF contained various amounts of fibers, which divided the fatty tissues into small lobules. Especially in the second and third patterns, the fatty tissue tended to contain longitudinal fibrous bands in parallel with the DLTF (Figs. 3B, 4C). Whether or not lobulation or septation of fatty tissues was evident in the SLTF and DLTF was not dependent on age (Fig. 3B vs. Fig. 3D). In some specimens (Figs. 3C, 4C), greater or lesser portions of the multi-layered STLF were not attached to the zygomatic arch but extended below the bony arch toward the cheek. The middle temporal artery took a wavy course in the fatty tissue (Fig. 3D).
The temporalis muscle fibers originated from the DLTF, but immediately above the zygomatic arch there was a narrow space between the muscle and the fascia. Thus, in contrast to mid-term specimens (Fig. 1B, E), the lowest part of the DLTF appeared to “detach” from the temporalis muscle (Figs. 2A, C, 3C). Moreover, in a single specimen (GA 40 weeks), fatty tissues separated the DLTF from the muscle to form a solitary muscle mass detached from the intramuscular tendon (Fig. 4D).
The present study seems to provide the first demonstration of the near-term morphologies of the temporal fascia, being characterized by variations in the structure of the SLTF. Development of the subcutaneous or superficial SLTF was considerably delayed relative to the muscle-covering DLTF. The SLTF appeared to change from a mono-layered membrane, via a multi-layered membranous structure, to a cluster of independent thick fasciae each of which were separated by fatty tissue, but apparently dependent on the thickness of the subcutaneous tissue. Notably, this step-by-step process did not clearly depend on fetal size or age, but maturation of the SLTF appeared to correlate with the thickness of the subcutaneous tissue. A multi-layered muscle-covering fascia develops from the muscle itself, depending on muscle contractions [11]. However, as seen in adults [9], the temporalis muscle was partly separated from the DLTF by fatty tissue immediately above the zygomatic arch. The changing topographical relationship between the muscle and bone might lead to detachment of muscle fibers from the DLTF.
Similarly to the DLTF, development of the SLTF might also be influenced by temporalis muscle activity. However, the SLTF was a sheet-like structure comprising thickened and/or bundled collagenous fibers in the subcutaneous tissue. The driving force for the thickening and bundling appeared to come from the expanding skin, including the galea aponeurotica, as well as the underlying muscles. In fact, greater or lesser parts of the multi-layered STLF did not attach to the zygomatic arch, but extended below the bony arch, being apparently continuous with the musculoaponeurotic system of the cheek [10].
Here, we avoided use of the term “deep fascia” and instead applied the term “muscle-covering fascia”. We were concerned that the former term was likely to give a misleading impression of 1) a fascia lining a deeply-located muscle or 2) a deeply-located fascia distant from skeletal muscles. A typical example of the former is the transversalis fascia covering the internal aspect of the rectus abdominis and the scalenus fascia covering the superior aspect of the scalenus anterior muscle. In contrast, the latter is known well to surgeons as Denonvillier’s fascia [15-17], the fascia of Treitz [18-20] or the renal fascia [21-23].
Taken together, the present findings have demonstrated that the temporal fascia is composed of 1) a later-developing superficial facia and 2) an early-developing deep fascia. The thicker subcutaneous tissue tends to contain well-differentiated superficial fasciae, while higher activity of the temporalis muscle appears to play a role in growth of the deep fascia. The temporal fascia seems to be the best sample to show a difference in development between two categories of the fasciae.
Notes
References
1. Schaeffer JP. Morris' human anatomy: a complete systematic treatise. 11th ed. Blakiston;1953. p. 426.
2. Williams PL. Gray's anatomy: the anatomical basis of medicine and surgery. 38th ed. Churchill Livingstone;1995. p. 800.
3. Standring S. Gray's anatomy: the anatomical basis of clinical practice. 39th ed. Churchill Livingstone;2005. p. 499.
4. Lam D, Carlson ER. 2014; The temporalis muscle flap and temporoparietal fascial flap. Oral Maxillofac Surg Clin North Am. 26:359–69. DOI: 10.1016/j.coms.2014.05.004. PMID: 25086696.


5. Pitman MJ, Rubino SM, Cooper AL. 2014; Temporalis fascia transplant for vocal fold scar and sulcus vocalis. Laryngoscope. 124:1653–8. DOI: 10.1002/lary.24536. PMID: 24281875.


6. Morales-Avalos R, Soto-Domínguez A, García-Juárez J, Saucedo-Cardenas O, Bonilla-Galvan JR, Cardenas-Serna M, Guzmán-López S, Elizondo-Omaña RE. 2017; Characterization and morphological comparison of human dura mater, temporalis fascia, and pericranium for the correct selection of an autograft in duraplasty procedures. Surg Radiol Anat. 39:29–38. DOI: 10.1007/s00276-016-1692-z. PMID: 27177905.


7. Lee HJ, Kim HM, Ahn HS, Lee JH, Kim HJ. 2024; Novel clinical anatomical consideration of the superficial and deep layers of the deep temporal fascia. Plast Reconstr Surg. 153:591–9. DOI: 10.1097/PRS.0000000000010507. PMID: 37010473.


8. Stuzin JM, Baker TJ, Gordon HL. 1992; The relationship of the superficial and deep facial fascias: relevance to rhytidectomy and aging. Plast Reconstr Surg. 89:441–9. discussion 450–1. DOI: 10.1097/00006534-199203000-00008. PMID: 1741467.
9. Watanabe K, Han A, Inoue E, Iwanaga J, Tabira Y, Yamashita A, Kikuchi K, Haikata Y, Nooma K, Saga T. 2023; The key structure of the facial soft tissue: the superficial musculoaponeurotic system. Kurume Med J. 68:53–61. DOI: 10.2739/kurumemedj.MS682008. PMID: 37062726.


10. Mitz V, Peyronie M. 1976; The superficial musculo-aponeurotic system (SMAS) in the parotid and cheek area. Plast Reconstr Surg. 58:80–8. DOI: 10.1097/00006534-197607000-00013. PMID: 935283.


11. Cho KH, Jang HS, Abe H, Yamamoto M, Murakami G, Shibata S. 2018; Fetal development of fasciae around the arm and thigh muscles: a study using late stage fetuses. Anat Rec (Hoboken). 301:1235–43. DOI: 10.1002/ar.23804. PMID: 29575697.


12. Kim JH, Oka K, Jin ZW, Murakami G, Rodríguez-Vázquez JF, Ahn SW, Hwang HP. 2017; Fetal development of the incisive canal, especially of the delayed closure due to the nasopalatine duct: a study using serial sections of human fetuses. Anat Rec (Hoboken). 300:1093–103. DOI: 10.1002/ar.23521. PMID: 27860365.


13. Kim JH, Shibata S, Abe H, Murakami G, Rodríguez-Vázquez JF. 2019; Topographical variations of the incisive canal and nasopalatine duct in human fetuses. Anat Cell Biol. 52:426–35. DOI: 10.5115/acb.19.111. PMID: 31949982. PMCID: PMC6952684.


14. Yamamoto M, Jin ZW, Hayashi S, Rodríguez-Vázquez JF, Murakami G, Abe S. 2021; Association between the developing sphenoid and adult morphology: a study using sagittal sections of the skull base from human embryos and fetuses. J Anat. 239:1300–17. DOI: 10.1111/joa.13515. PMID: 34268732. PMCID: PMC8602018.


15. Kinugasa Y, Murakami G, Uchimoto K, Takenaka A, Yajima T, Sugihara K. 2006; Operating behind Denonvilliers' fascia for reliable preservation of urogenital autonomic nerves in total mesorectal excision: a histologic study using cadaveric specimens, including a surgical experiment using fresh cadaveric models. Dis Colon Rectum. 49:1024–32. DOI: 10.1007/s10350-006-0557-7. PMID: 16732487.


16. Kim JH, Kinugasa Y, Hwang SE, Murakami G, Rodríguez-Vázquez JF, Cho BH. 2015; Denonvilliers' fascia revisited. Surg Radiol Anat. 37:187–97. DOI: 10.1007/s00276-014-1336-0. PMID: 25008480.


17. Muraoka K, Hinata N, Morizane S, Honda M, Sejima T, Murakami G, Tewari AK, Takenaka A. 2015; Site-dependent and interindividual variations in Denonvilliers' fascia: a histological study using donated elderly male cadavers. BMC Urol. 15:42. DOI: 10.1186/s12894-015-0034-5. PMID: 25962380. PMCID: PMC4433060.


18. Androulakis J, Colborn GL, Skandalakis PN, Skandalakis LJ, Skandalakis JE. 2000; Embryologic and anatomic basis of duodenal surgery. Surg Clin North Am. 80:171–99. DOI: 10.1016/S0039-6109(05)70401-1. PMID: 10685148.


19. Rouvièr H, Delmas A. Anatomie humaine-descriptive, topographique et fonctionnelle. 2nd ed. Masson;1974. p. 450–68. DOI: 10.1016/s0039-6109(05)70401-1.
20. Cho BH, Kimura W, Song CH, Fujimiya M, Murakami G. 2009; An investigation of the embryologic development of the fascia used as the basis for pancreaticoduodenal mobilization. J Hepatobiliary Pancreat Surg. 16:824–31. DOI: 10.1007/s00534-009-0126-2. PMID: 19517056.


21. Hayes MA. 1950; Abdominopelvic fasciae. Am J Anat. 87:119–61. DOI: 10.1002/aja.1000870105. PMID: 14771009.


22. Matsubara A, Murakami G, Niikura H, Kinugasa Y, Fujimiya M, Usui T. 2009; Development of the human retroperitoneal fasciae. Cells Tissues Organs. 190:286–96. Erratum in: Cells Tissues Organs 2013;197:88. DOI: 10.1159/000209231. PMID: 19321993.


23. Kim JH, Hayashi S, Jin ZW, Murakami G, Rodríguez-Vázquez JF. 2020; Variations in laminar arrangements of the mesocolon and retropancreatic fascia: a histological study using human fetuses near term. Tokai J Exp Clin Med. 45:214–23. PMID: 33300593.
Fig. 1
The temporal fascia without its superficial lamina in three mid-term fetuses. Frontal sections. Azan staining. (A, B) A 95-mm crown-rump length (CRL) fetus (gestational age [GA] approximately 13 weeks); (C, D) (1.2 mm posterior to panel C) a 113-mm CRL fetus (GA approximately 15 weeks); (E) a 137-mm CRL fetus (GA approximately 16 weeks). (B) Shows a higher-magnification view of the square in (A). Arrowheads indicate the deep lamina of the temporal fascia providing origins for the temporalis muscle (TM) and ending at the zygomatic arch (ZA). The latter muscle fibers insert to an intramuscular tendon (triangles). Asterisks in (E) indicate subcutaneous bleeding during sampling of the specimen. (A, C–E) Were prepared at the same magnification (scale bars in panels A and B, 1 mm). CPM, coronoid process of the mandible.
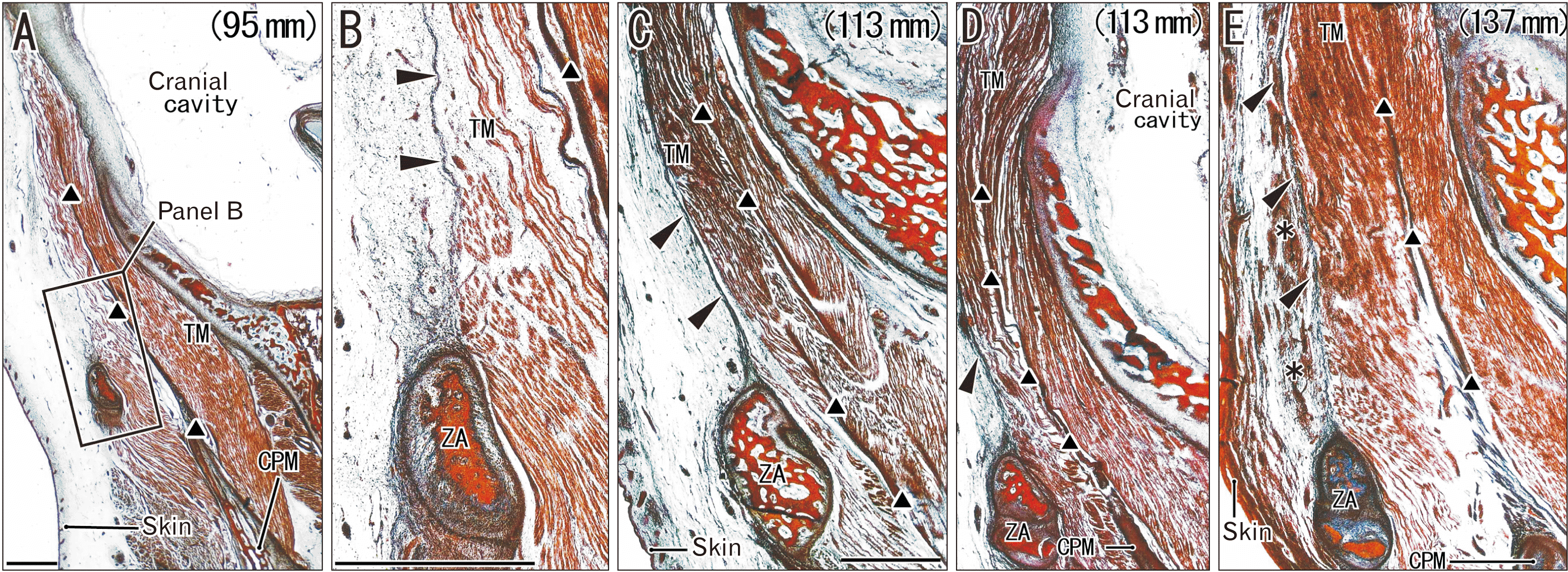
Fig. 2
The mono-layered superficial lamina of the temporal fascia in two near-term fetuses. Frontal sections. H&E staining. (A) A 215-mm crown-rump length (CRL) fetus (gestational age [GA] approximately 26 weeks); (B, C) (4.5 mm posterior to panel B) a 260-mm CRL fetus (GA approximately 31 weeks). Arrows indicate the superficial lamina of the temporal fascia underlying the subcutaneous tissue, while arrowheads indicate the deep lamina providing origins for the temporalis muscle (TM) and ending at the zygomatic arch (ZA). The latter muscle fibers insert to an intramuscular tendon (triangles). All panels were prepared at the same magnification (scale bar in panel A, 1 mm).
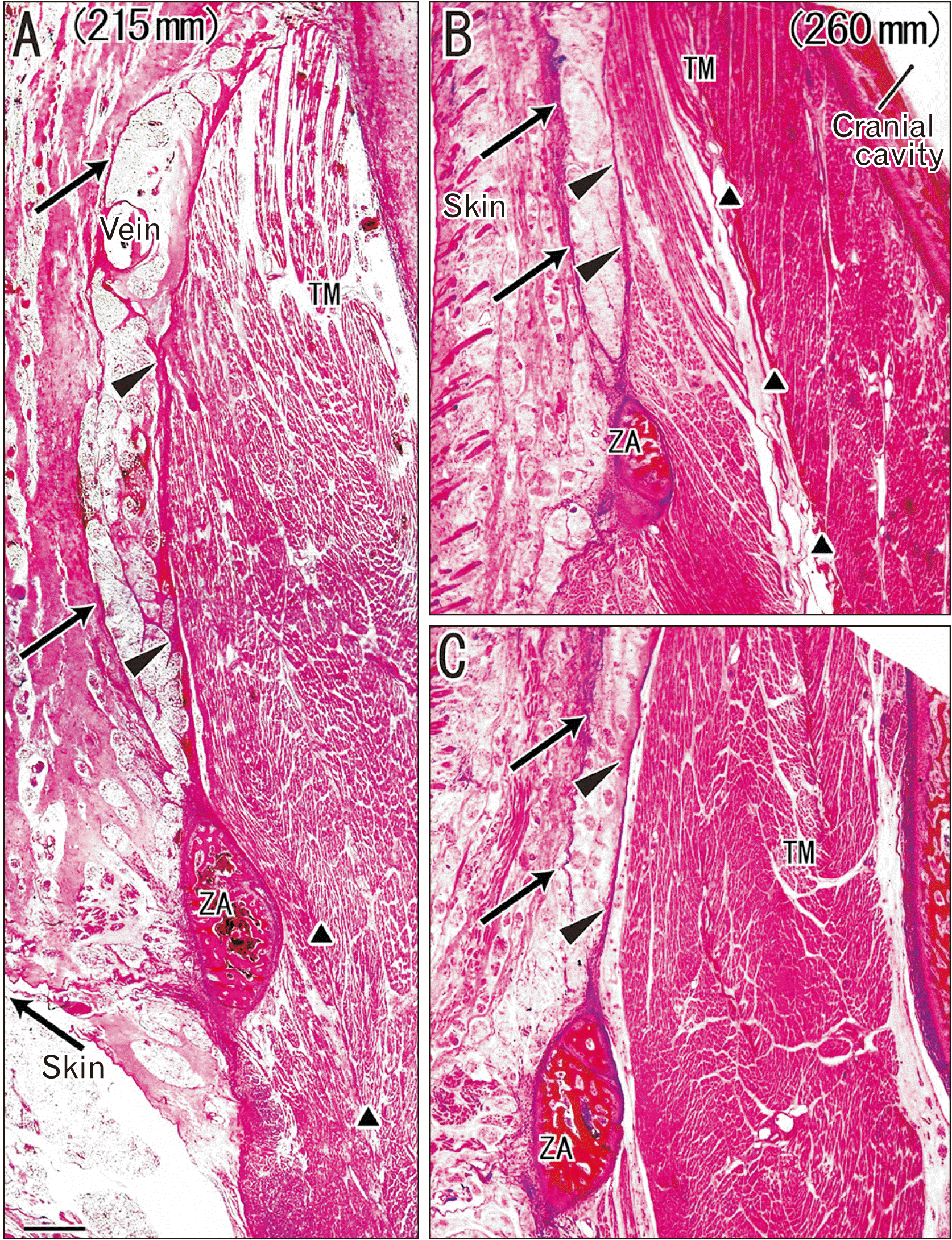
Fig. 3
The multi-layered superficial lamina of the temporal fascia in two near-term fetuses. Frontal sections. H&E staining (A, B) and Masson trichrome staining (C, D). (A, B) A 225-mm crown-rump length (CRL) fetus (gestational age [GA] approximately 28 weeks); (C, D) a 228-mm CRL fetus (GA approximately 28 weeks). (B, D) are higher-magnification views of the squares in (A, C), respectively. Arrowheads indicate the deep lamina of the temporal fascia covering the temporalis muscle (TM) and attached to the zygomatic arch (ZA). The superficial lamina of the temporal fascia appears to be multi-layered. Between the superficial and deep laminae, there are irregularly arrayed fiber bundles (stars and arrows in panel B), fatty tissue lobules and the middle temporal artery (D). Asterisks in (B) indicate an artifactual space. (A, B) (or B, D) Were prepared at the same magnification (scale bar in panels A and B, 1 mm).
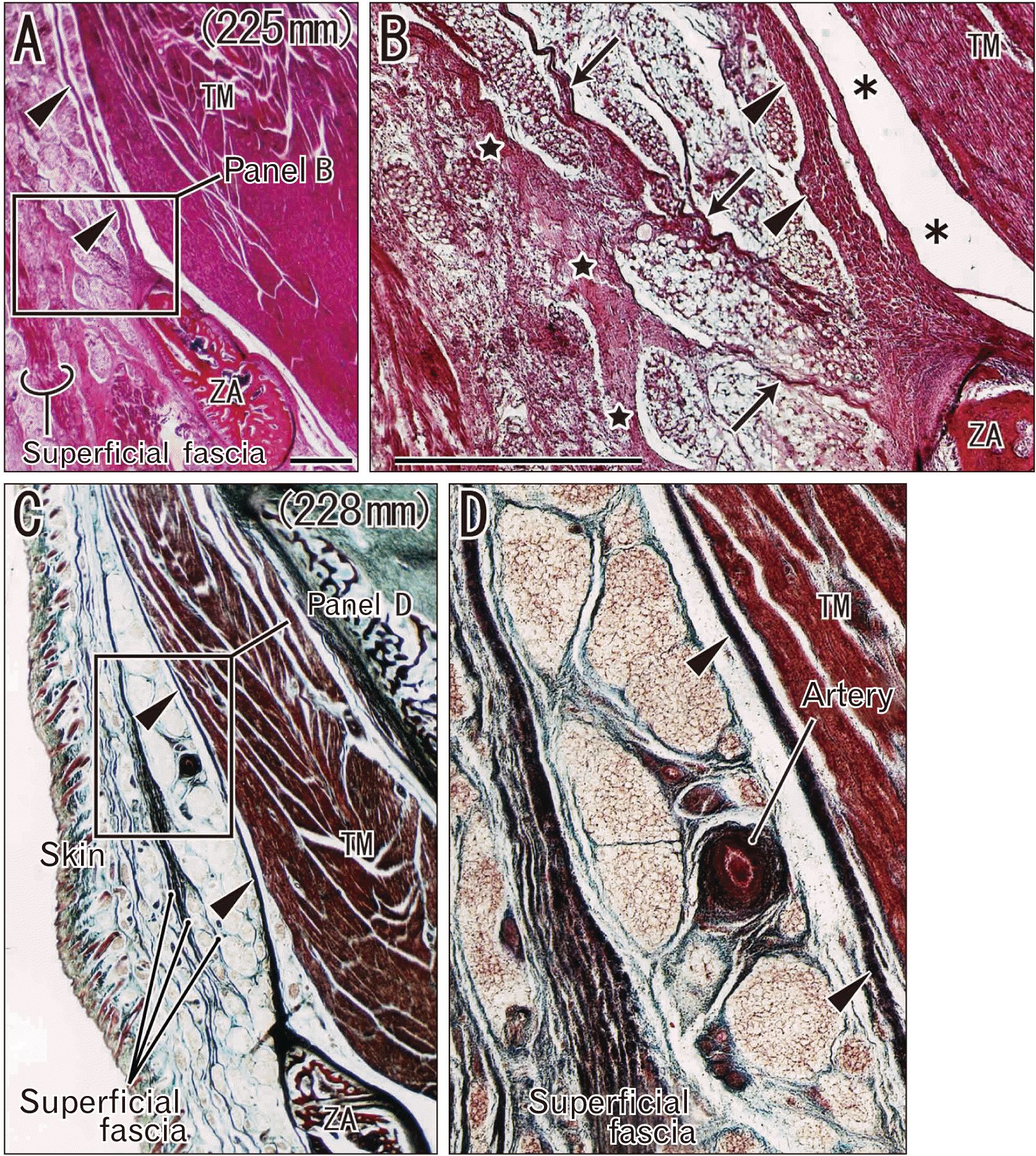
Fig. 4
The superficial lamina of the temporal fascia comprising a cluster of multiple independent fasciae. Frontal sections. H&E staining. A 334-mm crown-rump length (CRL) fetus (gestational age [GA] approximately 40 weeks). (A) Shows a plane 14 mm anterior to (C). (B, D) are higher-magnification views of the squares in (A, C), respectively. During the histological procedure, the temporalis muscle (TM) was torn (asterisks) along almost all of the intramuscular tendon (triangles). Arrowheads indicate the deep lamina of the temporal fascia covering the muscle and attached to the zygomatic arch (ZA), but fatty tissues (stars) separate the muscle from the fascia. A muscle fragment is contained within the fatty tissue and appears to be detached from the intramuscular tendon (D). The superficial lamina is composed of a cluster of multiple independent fasciae each of which are separated by fatty tissue (B, D). (A, B) (or B, D) Were prepared at the same magnification (scale bar in panels A and B, 1 mm).
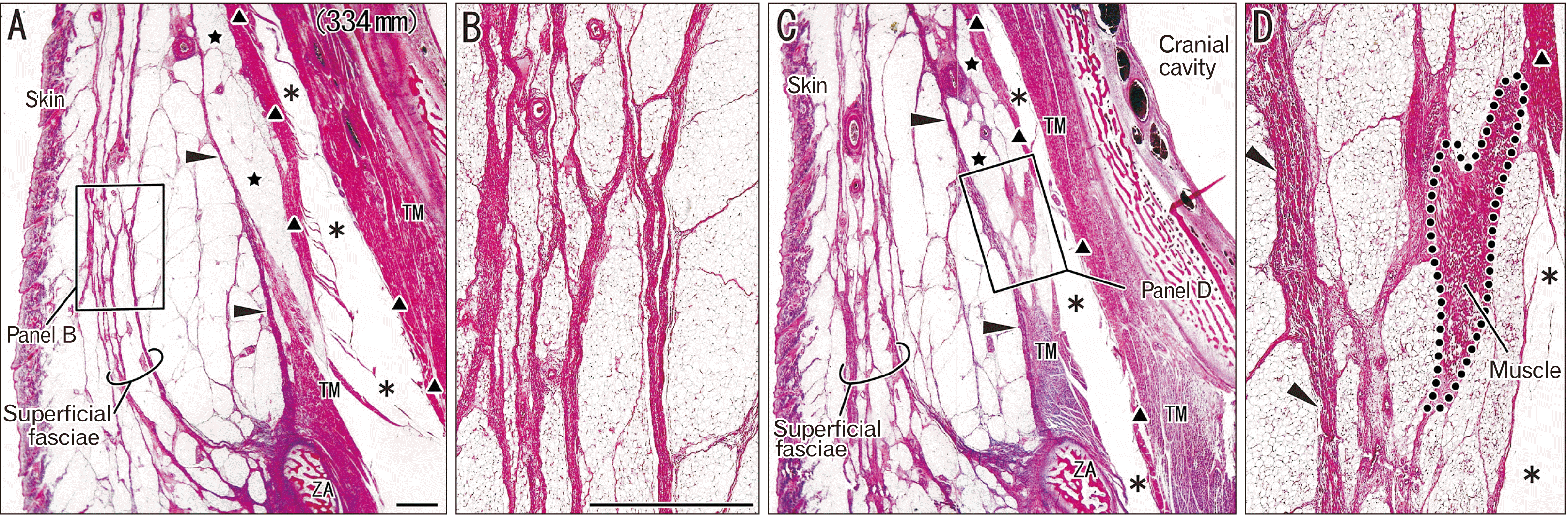