Abstract
Cardiac organoids have emerged as invaluable tools for assessing the impact of diverse substances on heart function. This report introduces guidelines for general requirements for manufacturing cardiac organoids and conducting cardiac organoid-based assays, encompassing protocols, analytical methodologies, and ethical considerations. In the quest to employ recently developed three-dimensional cardiac organoid models as substitutes for animal testing, it becomes imperative to establish robust criteria for evaluating organoid quality and conducting toxicity assessments. This guideline addresses this need, catering to regulatory requirements, and describes common standards for organoid quality and toxicity assessment methodologies, commensurate with current technological capabilities. While acknowledging the dynamic nature of technological progress and the potential for future comparative studies, this guideline serves as a foundational framework. It offers a comprehensive approach to standardized cardiac organoid testing, ensuring scientific rigor, reproducibility, and ethical integrity in investigations of cardiotoxicity, particularly through the utilization of human pluripotent stem cell-derived cardiac organoids.
The purpose of the guidelines for the practical application of cardiac organoids is to provide standards for the quality of cardiac organoids and guidance on their utilization in toxicological assessments for non-clinical purposes. Cardiac toxicity in pharmaceutical development has become a serious issue, particularly with the reporting of numerous drugs inducing ventricular arrhythmias through QT prolongation, leading to the establishment of the International Council for Harmonisation of Technical Requirements for Pharmaceuticals for Human Use (ICH) S7B guideline on delayed ventricular repolarization in 2005, which has been utilized since then. However, these evaluation systems fail to comprehensively reflect the complex and intricate electrophysiological phenomena of the heart (1). In addition, in vivo animal experiments are continually challenged due to limitations in predicting human toxicity caused by inter-species differences and ethical issues associated with animal experimentation.
With the emergence of induced pluripotent stem cells (iPSCs) and the development of cardiomyocyte differentiation techniques, multinational regulatory agencies have cooperated to utilize cardiomyocytes differentiated from human stem cells for non-clinical testing. In the revised guidelines issued in August 2022, methods such as multi-electrode array (MEA) testing using human stem cell-derived differentiated myocardial cells are proposed for follow-up studies (2).
Furthermore, recent research efforts are underway to mimic organ development processes in vitro and create three-dimensional (3D) organoid (mini-organ) models that can better simulate the 3D in vivo environment compared to conventional two-dimensional (2D) cell models. These models are expected to improve upon the limitations of existing 2D-based in vitro testing methods. Ensuring the safety of not only pharmaceutical products but also industrial chemicals and cosmetic products that may be encountered in daily life is essential. Thus, the development of in vitro human mimetic cardiac models capable of effectively detecting their toxicity will play a crucial role in safely and efficiently introducing new products to the market.
The primary objective of this guideline is to establish minimum quality criteria for cardiac organoids, aiming to enhance the predictive accuracy of toxicity assessments based on cardiac organoid models. By minimizing evaluation uncertainties, this guideline seeks to improve the reliability and robustness of toxicity assessment methods. Various models of cardiac organoids are being developed using different production methods and utilization strategies, necessitating optimization of production methods and analytical techniques for functional analysis of each model. This guideline provides universally applicable minimum quality requirements for cardiac organoids and presents example protocols for their selective application in toxicity assessments. Test substances applicable to cardiac organoid-based toxicity assessments covered in this guideline may include pharmaceuticals, chemicals, and cosmetics. Equipment systems, reagents, and materials used in the production and analysis of organoids can be substituted with others demonstrating similar or equivalent performance. Continuous updates to this guideline are necessary to align with advancements in technology, societal consensus, market demands, and regulatory science trends, as well as considerations of feasibility and cost-effectiveness.
This standard guideline elaborates on the production of cardiac organoids for non-clinical testings, which cover aspects such as cell source, culture conditions, quality requirements and assessment, storage and preservation, utilization in testing methods, and ethical considerations. While various methods for cardiac organoid production have been reported, this guideline provides a relatively detailed protocol that can be universally applied. In the section on organoid utilization in testing methods, representative analysis systems for cardiac arrhythmia-related toxicity assessment, contractility-related toxicity assessment, and myocardial cell cytotoxicity assessment are presented, using organoids produced according to the production protocol outlined in this guideline as examples. These guidelines can be extended and applied to model cardiac diseases and utilize cardiac regenerative therapies through future updates based on scientific evidence-driven quality assessments.
The schematic of the overall process of manufacturing cardiac organoids using human pluripotent stem cells is shown in Fig. 1.
Pluripotent stem cells, such as embryonic stem cells (ESCs) and iPSCs, can be utilized for the production of cardiac organoids. If the intention is to directly obtain source cells from humans for organoid production, it must adhere to ethical considerations and receive institutional review board (IRB) approval.
Quality control recommendations: The quality of pluripotent stem cells used for organoid production significantly affects both the efficiency of organoid production and the quality of the produced organoids. Therefore, quality control (QC) of stem cells is crucial. It is necessary to periodically verify if the quality requirements of the cells (cell viability, purity, contamination prevention, genetic stability, self-renewal and differentiation capability, safety, identification, characterization, etc.) are met. It is recommended to establish protocols for cell quality management and maintain records for cell history management in each laboratory to systematically manage them.
A step-by-step guide outlining the sequential stages, from initial cell sourcing and culture initiation to the maturation and QC of cardiac organoids. This process encompasses key steps such as embryoid body (EB) formation, differentiation induction, and QC of cardiac organoids, aimed at generating functional and physiologically relevant cardiac organoids for non-clinical applications.
Cell sources, characteristics, and quality requirements:
a.Cell sources and characteristics
Pluripotent stem cells include ESCs and iPSCs. ESCs are cell lines obtained from the inner cell mass of blastocysts from early-stage embryos and have the ability to propagate as undifferentiated cells. iPSCs are generated by inducing pluripotency in somatic cells, such as skin or blood cells, through the introduction of specific reprogramming factors. Similar to ESCs, iPSCs are capable of differentiating into cells of almost all organs in the body. These pluripotent stem cells exhibit characteristics of self-renewal and pluripotency, enabling them to differentiate into various cell types representing the three germ layers: endoderm, mesoderm, and ectoderm.
b.Cell quality requirements
For cell quality requirements, stem cells should exhibit high viability, with over 90% viability necessary for maintaining natural differentiation or pluripotency. Additionally, purity is essential, with stem cells expressing specific surface markers like SSEA-4 and TRA-1-60, confirmed via flow cytometry analysis. To prevent contamination, stem cells must remain free from bacteria, fungi, viruses, and mycoplasma. Genetic stability is crucial, validated through chromosomal analysis and whole-genome sequencing to detect variations or abnormalities. Stem cells should possess self-renewal and differentiation capabilities, enabling long-term culture and tissue formation. Safety measures are paramount, aiming to minimize potential adverse effects. Finally, accurate identification and characterization of stem cells through marker analysis are essential for ensuring their quality and reliability (Table 1).
Essential components and reagents:
a.Media composition
To produce cardiac organoids from stem cells, various differentiation-inducing factors and maturation-promoting factors are added to the basal media for cardiac organoid culture. Different laboratories may utilize different media compositions based on their differentiation strategies and protocols. The basal media for cardiac organoids should necessarily include components essential for cell growth and maintenance. One representative example is the RPMI-1640 medium, which includes the essential components (Table 2).
b.Essential growth factors
Various growth factors are utilized to support the formation, growth, and function of cardiac organoids. The following growth factors can be used to efficiently induce differentiation of cardiac constituent cells:
∙Fibroblast growth factor: Plays a crucial role in the growth and differentiation of cardiac cells, particularly influencing the proliferation and formation of myocardial cells. Recommanded concentration range: 10∼100 ng/mL.
∙Epidermal growth factor: Regulates cell differentiation and proliferation, influencing the proliferation and survival of cardiac cells. Recommanded concentration range: 10∼100 ng/mL.
∙Vascular endothelial growth factor: Plays a significant role in vascular growth and the formation of the cardiac vascular network. Recommanded concentration range: 10∼100 ng/mL.
∙Insulin: Regulates cell energy metabolism and influences cell growth and differentiation. Recommanded concentration range: 1∼10 μg/mL.
∙Note: Optimizing the concentrations of these factors is indeed critical for the successful development and maturation of cardiac organoids. The optimal dosages may vary depending on factors such as the cell type, culture conditions, and the specific protocol being used.
c.Reagents
∙WNT signal regulators
-WNT activators (WNT agonists): Used to activate the WNT signaling pathway.
-WNT inhibitors (WNT antagonists): Used to inhibit the WNT signaling pathway and regulate cardiac organoid differentiation.
∙Extracellular matrix (ECM): Used for stem cell attachment culture and 3D organoid culture.
∙Rho-associated coiled-coil kinase (ROCK) inhibitor: Utilized to enhance stem cell survival and proliferation.
∙Stem cell and cardiac organoid dissociation reagents
∙Maturation inducers
Cultural process and requirements:
a.Culture protocols
The culture protocol for cardiac organoids can include various media compositions and culture conditions based on the differentiation strategies adopted by each laboratory. It can be broadly divided into four stages as shown in Table 3.
Example of a cardiac organoid culture protocol:
1)Rinse the human iPSCs being maintained in a CO2 incubator with DPBS twice and treated with cell dissociation reagent at 1 mL/well for a 6-well plate and incubate for 4 minutes in the incubator. After removing the cell dissociation reagent, use a pipette to detach the cells from the plate bottom and transfer them to a 15 mL tube.
2)Take 10 μL of the obtained cells and dilute with 0.4% trypan blue solution at a 1:1 ratio. Place 10 μL of the diluted cell solution onto a cell counting slide and measure the number of viable cells using a cell counting machine.
3)Prepare 0.5∼2.4×106 cells and evenly distribute them onto wells of a pre-coated 3D cell culture device. Use a pipette to evenly distribute the cell suspension across all wells.
4)Place the plate in a CO2 incubator at 37°C for 15 minutes to ensure proper cell settling. Shake the plate every 2∼3 minutes to ensure uniform cell settling.
5)Check under a microscope to confirm cells are settled at the bottom of the well and remove excess cell suspension from outside the well. Carefully add plating media to the dish periphery. Incubate the dish in a CO2 incubator at 37℃.
6)Replace with fresh stem cell culture media the next day after cell plating.
7)Begin differentiation when the cell aggregates reach approximately 300 μm in diameter, typically 2 days after cell plating. Until differentiation, change the media daily with fresh stem cell culture media.
8)On the day of differentiation, transfer the cell aggregates from the 3D cell culture device to a 6-well clear bottom ultra-low attachment microplate using a 1 mL pipette and medium. Depending on the number of 3D cell aggregates, divide them into 2∼3 wells per 6-well plate.
9)Remove the existing stem cell culture media and replace it with differentiation induction media containing WNT activators. Use 2 mL per well for a 6-well plate.
10)After 48 hours, switch to differentiation induction media containing WNT inhibitors.
11)Replace the media with fresh differentiation induction media every 2 days.
12)On the 8th day of differentiation, switch to maintenance culture media containing insulin and change the media every 2∼3 days to maintain the cardiac organoids.
b.Culture environment conditions
∙Cell incubator: Culturing is typically performed in a cell culture incubator set at 37℃ with 5% CO2. The relative humidity within the incubator should be maintained at 95% or higher to prevent cell dehydration, which can affect proliferation and survival.
∙Cell culture methods: Culturing methods can be categorized into adherent culture or suspension culture, each requiring suitable culture vessels and media. Stem cell maintenance culture typically utilizes adherent culture vessels coated with stem cell culture-grade ECM, while processes post-EB formation may involve the use of ultra-low attachment culture vessels. Bioreactors can be advantageous for suspension culture, facilitating mass production and expansion.
∙Sterile manipulation: Stem cell culture procedures should be conducted using aseptic techniques within a microbiological safety cabinet, and researchers should wear appropriate protective clothing.
Size and morphology: Organoids developed by different laboratories may exhibit varied sizes, ranging from hundreds of micrometers to several millimeters, depending on the differentiation protocols.
In non-chambered organoids, exceeding a certain size can lead to central cell death due to oxygen and nutrient deprivation, emphasizing the need to establish size criteria to ensure organoid viability. Thus, it is crucial to establish size criteria that prevent central cell death (necrotic core) resulting from oxygen and nutrient deprivation.
While size and morphology are essential, functional criteria such as toxicological assessments are often prioritized for evaluating organoid quality.
Healthy organoids exhibit rhythmic beating, which should be monitored during differentiation, culture, and testing phases.
Cell composition and organ-specific gene expression: Organoids typically consist of cardiomyocytes and non-myocytes, with cardiomyocytes predominating. Cardiomyocytes can be further categorized into nodal, atrial, and ventricular types. Non-myocytes include fibroblasts and vascular cells, which comprise endothelial cells, pericytes, and smooth muscle cells.
Cell composition analysis is typically conducted through cellular type-specific markers using Fluorescence activated cell sorter (FACS) analysis.
Quantitative reverse transcription polymerase chain reaction (qRT-PCR) and similar techniques for analyzing cardiac-specific gene expression may exhibit high variability between experimental batches and lack established quantitative criteria for correlation with cardiac function. Hence, they are more suitable as qualitative confirmation assays for cardiac differentiation rather than establishing quantitative quality evaluation criteria.
Examples of cellular type- and cardiac-specific genes are shown in Table 4.
Periodic confirmation of the presence of myocardial and non-myocardial cells within cardiac organoids, in accordance with the specified ratios and ranges determined by the organoid production protocol, is necessary for reliably evaluating the effects of test substance.
Organ-specific functionality:
∙Electrophysiological function: Myocardial cells within the cardiac organoid exhibit action potentials generated by inward ion channel currents (Na+, Ca2+, etc.) involved in depolarization and outward ion channel currents (such as hERG, IKs) associated with repolarization. These action potentials can be recorded as field potentials outside the cell membrane. Patch-clamp assays allow for the analysis of ion channel currents and action potentials, while MEA analysis enables field potential measurements.
∙Contractile function: Cardiac organoids undergo spontaneous contraction and relaxation, driven by intracellular calcium oscillations. Imaging techniques can be employed to measure changes in organoid length or quantify contractile force using calcium imaging.
Others:
∙Cellular metabolic analysis: The metabolic analysis of cardiac organoids represents a critical avenue for understanding cellular function. Central to this examination is the assessment of oxygen consumption rate (OCR), a key metric that identifies the metabolic activity within these structures. By closely monitoring OCR, researchers obtain information about the efficiency of oxidative phosphorylation and broader cellular respiration processes, which can also be utilized in drug testing with cardiac organoids. Complementing this assessment is the pivotal role of adenosine triphosphate (ATP) analysis, which delves into the intricacies of cellular energy dynamics. ATP, serving as the fundamental energy currency of cells, offers a glimpse into the metabolic state and functional resilience of cardiac organoids.
∙Chromosomal karyotype analysis: Performing karyotype analysis on cardiac organoids before and after differentiation ensures genetic stability, enhancing confidence in the reliability of organoid-based assays for evaluating the effects of test substances.
∙Cell identity analysis: The short tandem repeat (STR) analysis or similar methods can be utilized to periodically assess the identity of cardiac organoids and the pluripotent stem cells from which they originate, serving as a quality assurance (QA) measure.
∙Sterile manipulation: Stem cell culture procedures should be conducted using aseptic techniques within a microbiological safety cabinet, and researchers should wear appropriate protective clothing.
Practical troubleshooting methods: Despite employing a well-established protocol demonstrating consistent efficiency in cardiac organoid differentiation, instances of failure in this process necessitate the researcher to reassess stem cell quality, evaluate potential compromises in the activity of differentiation materials, and verify the proper execution of the stock formulation.
After cardiac organoid production, assess the differentiation efficiency of cardiac organoids batch-wise (confirm spontaneous beating) and periodically (e.g., weekly) monitor the beats per minute (BPM) under the microscope to ensure it remains between 30∼90 bpm (0.5∼1.5 Hz).
When using the MEA system for measuring cardiac organoid function, utilize a detection threshold of 300 μV for field potential amplitude (FPA) and utilize BPM between 30∼90 bpm for test substance assessment. Only treat with test substances when BPM, FPA, and field potential duration corrected by Fridericia’s formula (FPDcF) are stable during the pre-treatment stabilization period.
When conducting contractility assessment tests such as imaging-based cardiac organoid contractility analysis or calcium imaging analysis, evaluate the impact of test substances using indicators such as signal magnitude (amplitude, peak-to-peak), velocity (dV/dt, time-to-peak) obtained from images of the entire 3D organoid using calcium-sensitive dye.
For cardiac cell toxicity testing using the 3D cell viability assay, confirm a survival rate of over 90% in the untreated group or vehicle-treated group before conducting the assay.
If freezing preservation of the produced cardiac organoids is feasible, it is desirable for QA purposes to store samples that have been frozen for at least once a year. These stored samples should be thawed and compared for purity, functionality, etc., both before and after freezing or immediately after freezing to ensure no change in quality.
Storage protocol:
a.Freezing process
1)Before freezing, confirm the quality of cardiac organoids by PCR using culture media for the absence of fungi and microbes and select only cardiac organoids maintaining a beating rate of 0.5∼1.5 Hz.
2)Transfer the cardiac organoids intended for freezing to a 15-mL conical tube.
3)Centrifuge at 150 ×g for 1 minute to sediment the organoids and remove the supernatant.
4)Resuspend the cardiac organoids in freezing preservation solution and transfer them to cryotubes.
5)Place cryotubes in a cryotank and store in a deep freezer for 24 hours or freeze using a controlled-rate freezer before transferring to an LN2 tank for long-term storage.
b.Thawing process
1)Retrieve the frozen organoid stock from the LN2 tank and rapidly thaw to approximately 50% in a 37℃ water bath.
2)Add 0.5 mL of thawing medium (organoid maintenance culture medium+10 μM ROCK inhibitor) and pipette to completely dissolve the freezing preservation solution.
3)Transfer the organoids and dilution medium to a 15-mL conical tube and add thawing medium to a total volume of 10 mL.
4)Centrifuge at 150 ×g for 1 minute to sediment the organoids and add 1 mL of thawing medium for washing 1∼2 times.
5)Transfer the washed organoids to a low attachment plate and culture in thawing medium.
6)Replace with organoid maintenance culture medium without ROCK inhibitor the next day and change the medium every 2∼3 days thereafter.
Quality checkpoints:
∙Post-thaw beating: Analyze the recovery of beating function during a stabilization period of 1 week after thawing the cardiac organoids using a platform that allows analysis under a microscope or of cardiac beating characteristics. Consider test substance impact assessment only for cardiac organoids maintaining a beating rate of 0.5∼1.5 Hz, similar to before freezing. Subsequently, perform tests applying the QC criteria required for each toxicity evaluation method.
∙Microorganism testing: Mycoplasma testing after thawing is performed by PCR on the organoid culture medium cultured for 48 hours. Compare with a positive control group and confirm the negativity of the PCR product. Subsequently, perform mycoplasma testing when deemed necessary during organoid maintenance culture or periodically (e.g., every 1∼2 weeks).
The objective of assays utilizing cardiac organoids is to perform non-clinical testings related to cardiac arrhythmia induction, contractility impact, and cardiomyocyte toxicity. These tests utilize indices related to cardiac organoid function such as electrophysiological analysis, contractility-related indicators, and survival-related indicators.
Note: This guideline primarily covers non-clinical in vitro/ex vivo cardiac toxicity evaluations related to arrhythmia, contractility, and cardiac cells’ cytotoxicity. Updates may be possible in the future as research results on chronic toxicity and cardiac remodeling accumulate, allowing for validation and standardization of these assays.
Essential equipments:
-MEA Analysis Equipment
-MEA Analysis Software
-Clean Bench
-CO2 incubator
-MEA plates
-Pipette & tips
∙MEA plate preparation: Dispense 4 μL of fibronectin (final conc. 50 μg/mL, diluted in DPBS [Mg+/Ca2+]) onto the central electrodes of each well of the MEA plate. Ensure that the fibronectin does not dry out by adding sterile 3rd distilled water to the reservoirs at the edges of the plate. Incubate the fibronectin-coated plate in a cell culture incubator for 30 minutes.
∙Sample plating: Remove the fibronectin-coated MEA plate from the incubator and remove any excess fibronectin. Immediately place the cardiac organoids onto the fibronectin-coated regions. Minimize the surrounding culture media to reduce organoid movement. Return the MEA plate with the organoids to the cell culture incubator for another 30 minutes. Then, add 0.4 mL of maintenance culture media to each well.
∙Sample maintenance: Every 2∼3 days, remove 0.2 mL of the existing culture media from each well and add 0.2 mL of fresh maintenance culture media. At least 4 hours before recording field potentials, replace all the remaining culture media in each well with 0.4 mL of fresh media.
∙Field potential recording: Turn on the equipment and set the temperature to 37℃ for preheating. Attach the MEA plate to the MEA apparatus and inject CO2 while recording for at least 20 minutes to confirm stabilization. After stabilization, record control values for 20 minutes before treating with the test substance. Use only wells where the FPA is above the detection threshold of 300 μV and the BPM are between 30 and 90 for the test substance evaluation.
∙Test substance preparation: Dissolve the test substance in a suitable solvent such as water or dimethyl sulfoxide (DMSO) to prepare a primary stock solution. Dilute the primary stock solution in solvent or maintenance culture media to prepare secondary stock solutions, ensuring that each test concentration achieves a 1000X dilution.
∙Test substance testing: Set the program to record data at 1-minute intervals over 5 minutes and evaluate the test substance response in at least three wells per substance, considering well-to-well variation. To add the substance sequentially from low to high concentrations, use 1/10 volume of fresh media (40 μL for a 48-well plate) in the same well to maintain temperature at 37℃. Sequentially add the substance every 20 minutes from low to high concentrations to achieve the final test concentrations.
∙Vehicle control testing: Record the vehicle control group by sequentially treating each test substance with the same concentration of solvent used for the test substance.
Data analysis and interpretation: Data analysis involves using the average values of the last 1 minute after stabilization before treatment with the test substance and the average values of the last 1 minute recorded over 20 minutes after treatment with the test substance. BPM, FPA, and FPD values per well are used to calculate BPM and FPDcF. BPM is calculated as 60 divided by the beat period, FPA represents the size when the field potential is maximally depolarized, and FPD represents the duration until repolarization after depolarization. FPDcF is calculated as FPD divided by the square root of the beat period.
The relative percent value for each concentration of the test substance treatment is compared to the control value (100%) to present relative values. As analysis indicators for cardiac toxicity, phenomena such as quiescence of spontaneous beating, flatten phenomenon where T wave disappears, and various types of cardiac arrhythmias are separately indicated if present.
Essential equipments:
-Imaging Analysis Equipment
-Microscope
-Osmometer
-pH meter
-Pipette & tips
∙Preparation of contractility measurement solution: Prepare a 10X contractility measurement solution using 3rd distilled water (NaCl 1,414 mM, KCl 40 mM, NaH2PO4 3.3 mM, HEPES 100 mM, MgCl2 10 mM). Adjust the pH of the solution to 7.5∼7.6 using 3M NaOH solution. After preparation, filter the solution using a 0.02 μm bottle top filter and store it refrigerated. On the day of measurement, dilute the 10X contractility measurement solution to 1X using 3rd distilled water. Add 5.5 mM Glucose, 1.8 mM CaCl2, and 14.5 mM Mannitol, adjusting the pH to 7.3∼7.5 with 3M NaOH. Verify the osmolarity using an osmometer to ensure it falls within the range of 290∼320 mOsm.
∙Contractility measurement: Allow the contractility measurement solution to flow through the recording chamber at approximately 37℃ and a flow rate of 1∼2 mL/min for at least 10 minutes. Place the cardiac organoid to be analyzed in the recording chamber to stabilize it, ensuring it remains stationary. Adjust the microscope to locate and focus on the area of the organoid where contractility will be measured. Record the reaction under untreated conditions for at least 5 minutes using a solution without the test substance or with the same amount of vehicle used to prepare the test substance solution. Treat the organoids with the substance of interest for at least 3 minutes at each concentration, starting from the lowest concentration to the highest, to confirm consistent responses to the test substance. Record contractility-related indicators under each concentration condition sequentially.
Data analysis and interpretation: The average values of contractility-related indicators over 30 seconds at the last point of each concentration are used for assessing the impact of the test substance. Peak height represents the height from baseline to peak, departure velocity indicates the maximum contraction velocity reached in the departure phases, and return velocity represents the maximum relaxation velocity reached in the returning phases. Time to peak 50% denotes the time taken to reach 50% of maximum contraction, and time to baseline 50% indicates the time taken to reach 50% of maximum relaxation.
It is important to confirm that the contractility analysis indicators are stably recorded in the control before treatment with the test substance and to check the minimum signal size to avoid bias due to background noise. Statistical analysis is performed to assess the significance of changes in contractility indicators after treatment with the test substance compared to the vehicle control or the solution without the test substance.
Essential equipments:
-Clean bench
-CO2 incubator
-Centrifuge
-Cell counter
-Luminometer
-Pipette & tips
∙Sample plating: Place one cardiac organoid per well in a 96-well plate and add 50 μL of maintenance culture medium to ensure uniformity across all wells.
∙Substance treatment: Treat the cardiac organoids with the test substance in at least three wells, considering well-to-well variation. Dissolve the test substance in a solvent such as water or DMSO to prepare a stock solution. Dilute the stock solution in a maintenance culture medium to achieve a final concentration that is 2 times the target concentration. For negative control experiments, treat the wells containing organoids with the same concentration of solvent used for preparing the test substance solution. For positive control experiments, treat the wells containing organoids with 0.2% TX-100. To remove background noise, add only culture medium to wells without organoids.
∙Cell viability measurement: Allow the 3D cell viability assay reagent and the plate to equilibrate at room temperature for at least 30 minutes. Add 100 μL of 3D cell viability assay solution to each well and mix the solution thoroughly. After a 30-minute incubation at room temperature, measure luminescence using a microplate reader.
Data analysis and interpretation: The measured values from microplate readers are exported and saved as an Excel file, with background values subtracted to minimize interference from the medium. The positive control group is set as 100% cell toxicity, and the relative luminescence rate of the test substance-treated group compared to the positive control group is presented as a percentage. The cell toxicity of the negative control group is verified to be less than 10%.
The determination of toxicity using these indicators and setting cutoffs for judgment may be further developed and validated through subsequent testing methods development/validation using positive and negative control groups.
The determination of toxicity using these indicators and setting cutoffs for judgment may be further developed and validated through subsequent testing methods development/validation using positive and negative control groups.
The cardiac organoids outlined in this standard guideline include human ESCs or iPSCs, including differentiated pluripotent stem cells. Therefore, obtaining samples and their utilization should be conducted under IRB approval, with the informed consent of sample providers regarding their participation in research, sample collection, organoid research, and utilization, following the entire content of organoid research and application.
Notes
Authors’ Contribution
Conceptualization: SJA, HAL. Data curation: HAL, DHW, DSL, JO, CYK. Formal analysis: HAL, DHW, DSL, JO, CYK. Funding acquisition: HAL, SJA. Investigation: HAL, DHW, DSL, JO, CYK, ONB. Methodology: HAL, DHW, DSL, JO, CYK, ONB. Project administration: HAL, SJA. Resources: HAL, DHW, DSL, JO. Supervision: HAL, SJA. Validation: CYK, ONB. Writing – original draft: HAL, DHW, DSL, JO, CYK, ONB. Writing – review and editing: HAL, SJA.
References
1. ICH. 2005. Nov. ICH Topic S 7 B The Nonclinical Evaluation of the Potential for Delayed Ventricular Repolarization (QT Interval Prolongation) by Human Pharmaceuticals [Internet]. EMEA;London: Available from: https://www.ema.europa.eu/en/documents/scientific-guideline/ich-s-7-b-nonclinical-evaluation-potential-delayed-ventricular-repolarization-qt-interval-prolongation-human-pharmaceuticals-step-5_en.pdf. cited 2024 Apr 3.
2. ICH. 2022. Mar. 24. ICH Guideline E14/S7B: Clinical and Nonclinical Evaluation of QT/QTc Interval Prolongation and Proarrhythmic Potential - Questions and Answers [Internet]. International Council for Harmonisation;Geneva: Available from: https://www.ema.europa.eu/en/documents/scientific-guideline/ich-guideline-e14s7b-clinical-and-nonclinical-evaluation-qtqtc-interval-prolongation-and-proarrhythmic-potential-questions-and-answers-step-5_en.pdf. cited 2024 Apr 3.
3. Blinova K, Dang Q, Millard D, et al. 2018; International multisite study of human-induced pluripotent stem cell-derived cardiomyocytes for drug proarrhythmic potential assessment. Cell Rep. 24:3582–3592. DOI: 10.1016/j.celrep.2018.08.079. PMID: 30257217. PMCID: PMC6226030.


4. Zwartsen A, de Korte T, Nacken P, de Lange DW, Westerink RHS, Hondebrink L. 2019; Cardiotoxicity screening of illicit drugs and new psychoactive substances (NPS) in human iPSC-derived cardiomyocytes using microelectrode array (MEA) recordings. J Mol Cell Cardiol. 136:102–112. DOI: 10.1016/j.yjmcc.2019.09.007. PMID: 31526813.


5. Lee SJ, Kim HA, Kim SJ, Lee HA. 2021; Improving generation of cardiac organoids from human pluripotent stem cells using the aurora kinase inhibitor ZM447439. Biomedicines. 9:1952. DOI: 10.3390/biomedicines9121952. PMID: 34944767. PMCID: PMC8698385.


6. Trembinski DJ, Bink DI, Theodorou K, et al. 2020; Aging-regulated anti-apoptotic long non-coding RNA Sarrah augments recovery from acute myocardial infarction. Nat Commun. 11:2039. DOI: 10.1038/s41467-020-15995-2. PMID: 32341350. PMCID: PMC7184724.


7. Kim MS, Fleres B, Lovett J, et al. 2020; Contractility of induced pluripotent stem cell-cardiomyocytes with an MYH6 head domain variant associated with hypoplastic left heart syndrome. Front Cell Dev Biol. 8:440. DOI: 10.3389/fcell.2020.00440. PMID: 32656206. PMCID: PMC7324479.
8. Pointon A, Harmer AR, Dale IL, et al. 2015; Assessment of cardiomyocyte contraction in human-induced pluripotent stem cell-derived cardiomyocytes. Toxicol Sci. 144:227–237. DOI: 10.1093/toxsci/kfu312. PMID: 25538221.


9. Ergir E, Oliver-De La Cruz J, Fernandes S, et al. 2022; Generation and maturation of human iPSC-derived 3D organotypic cardiac microtissues in long-term culture. Sci Rep. 12:17409. DOI: 10.1038/s41598-022-22225-w. PMID: 36257968. PMCID: PMC9579206.


10. Wang H, Sheehan RP, Palmer AC, et al. 2019; Adaptation of human iPSC-derived cardiomyocytes to tyrosine kinase inhibitors reduces acute cardiotoxicity via metabolic reprogramming. Cell Syst. 8:412–426.e7. DOI: 10.1016/j.cels.2019.03.009. PMID: 31078528. PMCID: PMC6657491.


11. Wu X, Chen Y, Luz A, Hu G, Tokar EJ. 2022; Cardiac development in the presence of cadmium: an in vitro study using human embryonic stem cells and cardiac organoids. Environ Health Perspect. 130:117002. DOI: 10.1289/EHP11208. PMID: 36321828. PMCID: PMC9628677.


Fig. 1
Comprehensive process for cardiac organoid manufacturing. EB: embryoid body, ESCs: embryonic stem cells, iPSCs: induced pluripotent stem cells, QC: quality control, ECM: extracellular matrix.
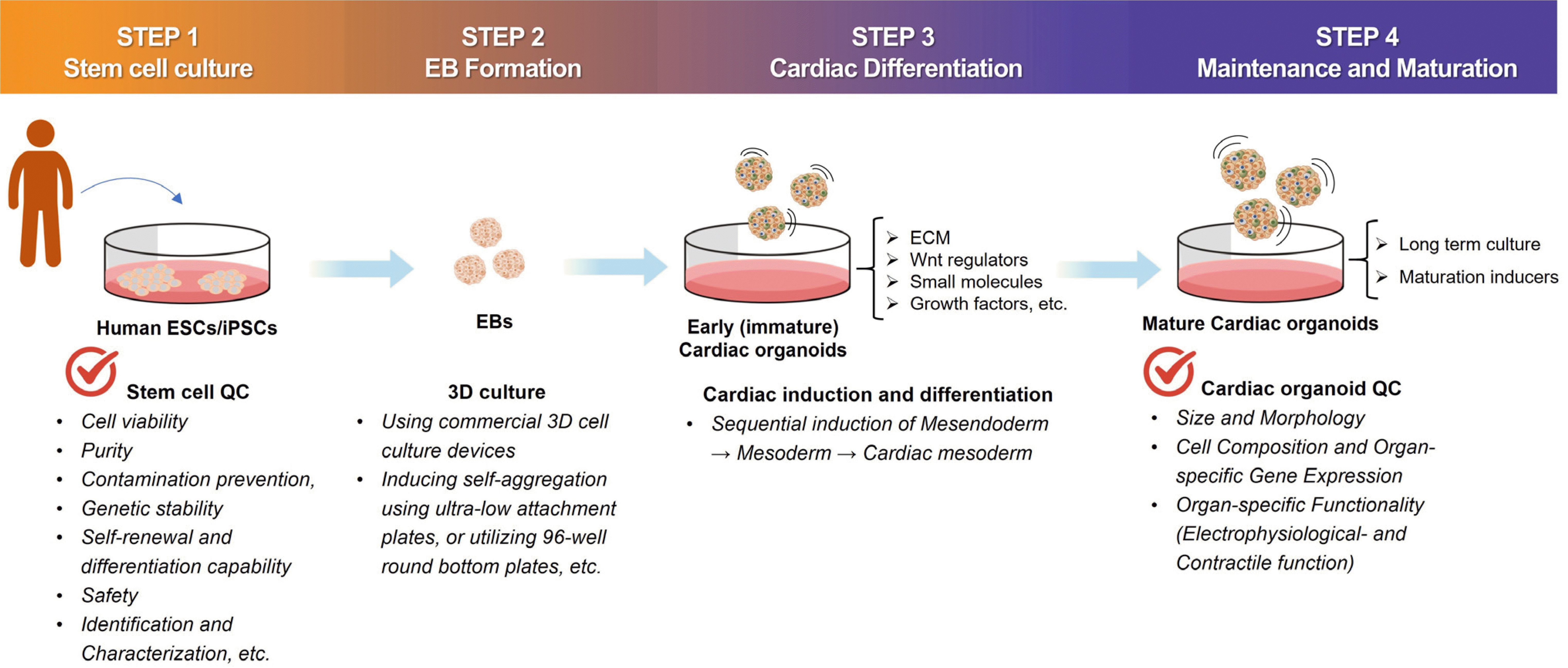
Table 1
Quality requirements for stem cells in cardiac organoid research
Table 2
Main ingredients of basal media in cardiac organoid cultures
Table 3
Key stages in development of cardiac organoids
Table 4
Specific markers for cardiac organoid characterization