This article has been corrected. See "Corrigendum to “Clinical Features and Treatment of Pediatric Cerebral Cavernous Malformations” by Phi et al. (J Korean Neurosurg Soc 67 : 299-307, 2024)" in Volume 67 on page 486.
Abstract
Cerebral cavernous malformation (CCM) is a vascular anomaly commonly found in children and young adults. Common clinical presentations of pediatric patients with CCMs include headache, focal neurological deficits, and seizures. Approximately 40% of pediatric patients are asymptomatic. Understanding the natural history of CCM is crucial and hemorrhagic rates are higher in patients with an initial hemorrhagic presentation, whereas it is low in asymptomatic patients. There is a phenomenon known as temporal clustering in which a higher frequency of symptomatic hemorrhages occurs within a few years following the initial hemorrhagic event. Surgical resection remains the mainstay of treatment for pediatric CCMs. Excision of a hemosiderin-laden rim is controversial regarding its impact on epilepsy outcomes. Stereotactic radiosurgery is an alternative treatment, especially for deepseated CCMs, but its true efficacy needs to be verified in a clinical trial.
Cerebral cavernous malformation (CCM) is a vascular anomaly found in approximately 0.5% of population [2]. CCM is characterized by intermingled sinusoidal vessels without high-flow arteriovenous shunting. Typically, CCM lacks intervening brain parenchyma. In 2018, the International Society for the Study of Vascular Anomalies classified it as a form of venous malformations [13]. CCM differs from developmental venous anomaly (DVA), a common aberrant venous channel found in the deep subcortical area. However, the frequent co-occurrence of CCM and DVA supports the possibility of their shared origins [6]. Although other terms such as cavernous angioma or cavernoma have been used, “cavernous malformation” has become the standard, emphasizing its nature as a malformation rather than a neoplasm.
CCMs are typically diagnosed in young adults, with the peak age at diagnosis in the third and fourth decades of life [49]. However, around one third of patients with CCMs are diagnosed during pediatric ages [36], although solid statistics on this proportion are lacking due to biases in clinical studies. Previously, CCM was considered as a congenital lesion [1], with reports even suggesting in utero diagnosis [20]. However, CCM can develop postnatally, especially adjacent to preexisting DVA [11,14]. The discovery of causative genes for familial CCM and well-known association of radiation therapy and de novo genesis of CCMs also support the notion that CCM is a postnatal disease. Consistent with this, the prevalence of CCM increases with age, with rates of only 0.23% in infants, 0.56% in 6–12 years, and 0.88% in 13–18 years reported in one study [2].
Approximately 15–30% of patients have multiple CCMs [2,36]. In general, 80% of patients with CCMs have sporadic disease while the remaining 20% are considered familial disease [15]. However, more than half of patients with multiple CCMs have a family history, with reports indicating family history in up to 75% of these cases. The most frequent site of CCM occurrence is supratentorial, especially in subcortical areas. However, CCMs can develop in anywhere in the brain, including the basal ganglia, cerebellum, brainstem, and optic chiasm. A study on pediatric CCMs reported that supratentorial (lobar) locations constituted 78% of cases, followed by the brainstem (8%), thalamus and basal ganglia (7%), and cerebellum (6%) [19].
Intralesional bleeding is common in CCMs, and their volumes fluctuate over time, resulting in a slowly incremental slope. Occasionally, extralesional bleeding can occur, leading to acute expansion and mass effects on adjacent brain tissue [3]. Repeated hemorrhages and the absorption of blood products induce inflammation and leave a typical hemosiderin rim on magnetic resonance images (MRIs), along with tough gliotic layers surrounding the lesion. However, hemorrhage from CCMs is typically not life-threatening, unlike hemorrhages from arteriovenous malformations.
Common clinical presentations of pediatric patients with CCMs include headache, focal neurological deficits (FNDs), and seizures [49]. Headaches and FNDs are typically associated with acute or subacute bleeding, while seizures can result from both subacute bleeding and chronic inflammatory changes around the lesion. The occurrence of FNDs depends on the location of the CCM [2]. Lesions in the brainstem and deep cerebral regions such as the basal ganglia and thalamus frequently cause FNDs because minor hemorrhages and perilesional edema can affect critical nuclei or white matter tracts within these areas.
However, it’s important to note that 25–45% of pediatric patients with CCMs are asymptomatic, with the lesions being incidentally discovered [2,19,43]. The advancement of MRI technologies, especially the widespread use of susceptibility-weighted imaging (SWI) in routine brain imaging, has led to the increased detection of incidental CCMs in the pediatric population.
Understanding the natural history is crucial due to its benign nature and the fact that many affected individuals remain asymptomatic throughout their lives. The debate over hemorrhage rates in CCM has persisted, partly due to variations in definition of clinically meaningful hemorrhages. To enhance clarity, the term “symptomatic documented hemorrhage” is recommended, denoting acute or subacute symptoms (such as headache, FND, or seizures) along with radiologically, pathologically, or surgically confirmed intra- or extra-lesional hemorrhage [3]. Importantly, the definition does not require a simple increase in lesion size or the presence of a hemosiderin rim on imaging.
The reported hemorrhage rate of CCM in children and young adults was 1.6% per patient-year and 0.9% per lesionyear [2]. Intriguingly, the same study reported a much higher hemorrhage rate (8.0%) in symptomatic patients compared to incidental cases (0.2%). In adult studies, the 5-year cumulative rates of symptomatic hemorrhage are approximately 20% [4,22]. A comprehensive meta-analysis identified initial symptomatic presentation with bleeding and a brainstem location as significant risk factors of symptomatic hemorrhage during follow-up [22]. Particularly noteworthy is the high hemorrhage risk of patients with brainstem CCM presenting with intracerebral hemorrhage (ICH) or FND, estimated at 30.8% [22]. However, age, sex, and multiplicity of CCM were not identified as risk factor in the meta-analysis. Similar findings were observed in a cohort study of untreated pediatric CCM patients [43]. The 5-year cumulative risk of symptomatic hemorrhage was 15.9% in 129 patients aged ≤18 years. Higher cumulative risks were observed in patients with initial presentation of ICH (30.2%), those with a brainstem location (29.5%), and individuals with family history of CCM (17.2%). It remains uncertain whether brainstem CCM really bleeds more frequently or if symptomatic hemorrhages are over-represented in this location due to the brainstem’s vulnerability. Overall, one third of pediatric patients with brainstem CCM experience an aggressive clinical course characterized with recurrent hemorrhage and neurological decline [52].
Numerous studies on CCM have documented temporal clustering of hemorrhages, with a higher frequency of symptomatic hemorrhages occurring within 2 or 3 years following the initial hemorrhagic event [2,8,49]. Gross et al. [19], found that annual hemorrhage rate (AHR) for pediatric CCMs with a hemorrhagic presentation was highest at 18.2% in the first 3 years, decreasing to 4.8% after 3 years and further to 3.3% after 5 years. The temporal pattern is important for patient counseling and assessment of treatment efficacy [19].
Seizures are the most common clinical symptom of CCM [47]. Moreover, CCM represents a significant structural etiology of chronic drug-resistant epilepsy. Data from the European Epilepsy Brain Bank (EEBB) which collects surgical specimens from epilepsy surgeries, revealed that vascular malformations constituted 6.1% of all specimens, ranking next only to hippocampal sclerosis, tumors, and cortical malformations [10]. Among vascular malformations, CCM was the most prevalent entity. Interestingly, in the EEBB data, the proportion of vascular malformations was 3.1% in children but rose to 7.2% in adults, reflecting an increasing incidence of CCM (and arteriovenous malformations) with ages.
CCMs lack functioning neural tissue within them, and are not inherently epileptogenic; however, they can trigger epileptic seizures by affecting adjacent brain tissue [7]. Extravasation of blood product and the resulting formation of a hemosiderin rim may contribute to epileptogesis [24,53]. Histopathological studies of perilesional tissues in CCM have shown a higher frequency of gliosis and reactive astrocytes in CCMs associated with epilepsy. Notably, siderotic vessels were found exclusively in perilesional tissues of CCMs associated with epilepsy [40].
The location of CCM is strongly correlated with development of epilepsy. One study demonstrated that 87% of the patients with temporal lobe CCM had epilepsy, with 50% of them experiencing drug-resistant epilepsy, whereas only 15% of those with non-temporal CCM had drug-resistant epilepsy [47]. The presence of a hemosiderin rim on imaging was identified as an independent risk factor, supporting its role in the epileptogenesis [55].
A small proportion of CCM develop during infancy (Fig. 1). In their review of data from 36 infantile patients with CCMs before the age of 2, Goyal et al. [18] found that nearly all but one patient presented with symptoms, predominantly seizures or FNDs. Notably, the proportion of CCMs located in the brainstem was higher (30%) compared to usual proportion of less than 10% in all-age cohorts. While thirteen patients were initially managed conservatively, no symptomatic hemorrhage was observed during 26 total person-years of observation. However, it is important to note that nearly two-thirds of patients in the literature review received surgery shortly after initial presentation, so the observed low hemorrhage rate during follow-up cannot be generalized. Some CCMs are detected prenatally through ultrasonography [7]. These congenital CCMs may lead to bleeding and symptoms in utero, potentially causing significant brain damage and fetal problems [20,21,28].
Treatment of CCM is typically recommended when symptoms related to CCM develop and persist. Hemorrhage from a CCM can result in acute or subacute symptoms, such as headaches, although these symptoms often gradually subside in many patients. However, recurrent hemorrhages can lead to fluctuating symptoms or a stepwise decline in neurological functions due to incomplete recovery from FNDs, thereby justifying the need for definitive treatment. Seizures refractory to medical treatment is another major indication of treatment [36].
Surgical removal of CCM is the mainstay of treatments. Cortical and subcortical CCMs can be approached directly. Typically, surgery is performed through a small cortical incision, and sometime through a deep corridor to access the lesion. Removing liquefied portions of CCM containing subacute blood is advisable to reduce the brain pressure and create a working space for dissection. Many CCMs are surrounded by a gliotic layer, which serves as a good dissection plane during surgery. Complete removal of a CCM is crucial. A study revealed that out of 126 patients, 24 had a remnant CCM after surgery, and seven of them experienced at least one recurrent hemorrhage [16]. It is important to identify any DVA associated with CCM preoperatively and preserve it during surgery, as DVAs drain normal brain tissues nearby [39].
For patients with CCM-related epilepsy, the seizure-free rate after surgery is 75–85% [31,42]. One study on children presenting with CCM-related seizures revealed that the probability of anti-seizure medication discontinuation at 1 year is significantly higher in patients undergoing surgery (88%) compared to those under observation (53%) [48]. Several studies have reported that excision of the hemosiderin-stained rim along with CCM itself leads to better seizure outcome than removal of only the CCM, but these studies are small-sized and retrospective [9,23,50]. Moreover, some studies found no difference in outcome [12,54]. More data are needed to resolve this issue. A meta-analysis indicated that extended lesionectomy, involving cortical resection beyond the boundary of CCMs either with or without electrocorticography, does not contributed to better seizure control than lesionectomy alone [44]. Extended lesionectomy is reserved for specialized cases in which concomitant focal cortical dysplasia is suspected [30].
Brainstem CCM3 pose a particular challenge for neurosurgeons. They have a higher hemorrhage rate and are more likely to cause symptoms (Fig. 2). Stepwise deterioration can be observed in patients who experience repeated hemorrhages from a brainstem CCM. Brainstem CCMs can be approached when the lesion is exposed or come very close to the surface of the brainstem [38]. A two-point method was proposed that an ideal surgical approach should follow a line connecting the center of a CCM and the closest surface point [38]. Intraoperative monitoring is helpful in identifying safe entry zones, as the anatomy of the brainstem may be distorted if there is a large underlying mass [17]. Mapping of the fourth ventricle floor is valuable for identifying critical landmarks such as midline sulcus, facial colliculus, and hypoglossal and vagal trigones [34]. The hemorsiderin-stained gliotic rim should be preserved for brainstem CCMs, and caution should be exercised to preserve any associated DVA [17].
For patient with a deep-seated CCM, such as those in the brainstem, surgical risks and complication rates are high. In such cases, SRS, particularly gamma knife radiosurgery (GKRS), can be considered as an alternative option for preventing subsequent hemorrhages and neurological decline [26]. In a study involving adult patients, the AHR of CCMs dropped from 15.3% before treatment to 2.6% during the first 2 years from GKRS [25]. This reduction in AHR was observed across all locations, including the cerebrum and brainstem. The initial experience of GKRS for CCMs resulted in relatively high rate of complications (approximately 20%), but radiation-related complication have decreased with modifications in radiation dose and techniques [32]. Recent studies reported temporary complication rates around 15% and permanent deficits in 4–5% of patients [25]. A multi-center study on GKRS in pediatric patients demonstrated that AHR decreased from 7.2% before treatment to 3.1% post-treatment, with 14.5% developed adverse radiation effects; 55% of the patients had no or minimal symptoms [33].
Criticism persists regarding the gradual decrement of AHR observed in these SRS studies, as it may merely reflect the natural history of CCMs, considering the temporal clustering of hemorrhagic events [2]. CCMs are intrinsically dynamic lesions, with some growing and others shrinking over time. Hemorrhagic rates can vary greatly between individuals and over time in the same patient. Without a randomized clinical trial, controversy regarding the efficacy of SRS would continue [51].
Identifying medications to enhance clinical outcome ranks among the top 10 research priorities for CCMs [5]. Medical treatment aims to prevent the de novo formation of CCMs, prevent CCM hemorrhage, and reduce the size of existing CCMs. However, no drug has been conclusively proven to achieve these goals in CCM treatment. Nonetheless, emerging evidence from epidemiological, preclinical, and clinical studies suggests that repurposing existing drugs or exploring novel target agents could benefit patients.
In a population-based study involving adult patients with CCM, the use of beta-blockers was associated with a reduced risk of new hemorrhage or persistent/progressive FND [56]. Conversely, female hormone therapy or oral contraceptive use was linked with a higher risk of CCM hemorrhages [57]. Preclinical studies have demonstrated that propranolol suppresses CCM formation in a zebrafish model with CCM2 gene disruption [29], and in a murine model with CCM3 gene-inducible knockout [35]. Recently published results from a randomized controlled phase 2 trial assessing the efficacy of propranolol for treating familial CCMs indicated a signal of efficacy, as the rate of new symptomatic hemorrhage or FNDs was lower in propranolol plus standard care group compared to the standard care alone (1.7 cases/100 person-years vs. 3.4 cases/100 person-years) [27].
The discovery of familial CCM-related genes, including CCM1, CCM2, and CCM3, has opened the door to the possibility of molecular target drugs for CCMs. The common biochemical derangement associated with the loss of CCM1, CCM2, and CCM3 is the activation of RhoA and increased Rho-associated coiled coil-forming kinase (ROCK) activity [41]. Fasudil, a specific ROCK inhibitor, has demonstrated the ability to suppress CCM development and prolong survival in a murine CCM model [46]. Statins, particularly atorvastatin, have shown efficacy in reducing lesion burden and hemorrhage in a murine CCM model by inhibiting ROCK activity [45]. Currently, a human randomized placebo-controlled trial is underway to evaluate the clinical impact of atorvastatin on CCMs [37].
CCM represents the most common cerebral vascular malformation requiring treatment in children and adolescents. However, the clinical courses of affected patients exhibit significant variability based on factors such as the patient’s age, lesion location, multiplicity, and family history. Additionally, the high and increasing proportion of asymptomatic patients further complicates the optimal management of CCM. Surgery remains the primary treatment modality for CCM. SRS presents an alternative option, particularly for deep-seated lesions that are not readily accessible; however, the efficacy of SRS requires further validation. Recent advancements in medical therapies offer promising avenues for non-invasive treatment and even prevention of CCMs in genetically predisposed children.
Notes
Conflicts of interest
Seung-Ki Kim has been editorial board of JKNS since November 2014. He was not involved in the review process of this review article. No potential conflict of interest relevant to this article was reported.
ACKNOWLEDGMENTS
This study was supported by a grant from SNUH Kun-hee Lee Child Cancer & Rare Disease Project, Republic of Korea (No. 22A-003-0100; to Phi JH).
References
1. Abla AA, Lekovic GP, Garrett M, Wilson DA, Nakaji P, Bristol R, et al. Cavernous malformations of the brainstem presenting in childhood: surgical experience in 40 patients. Neurosurgery. 67:1589–1589. discussion 1598-1599. 2010.


2. Al-Holou WN, O’Lynnger TM, Pandey AS, Gemmete JJ, Thompson BG, Muraszko KM, et al. Natural history and imaging prevalence of cavernous malformations in children and young adults. J Neurosurg Pediatr. 9:198–205. 2012.


3. Al-Shahi Salman R, Berg MJ, Morrison L, Awad IA; Angioma Alliance Scientific Advisory Board. Hemorrhage from cavernous malformations of the brain: definition and reporting standards. Angioma Alliance Scientific Advisory Board. Stroke. 39:3222–3230. 2008.


4. Al-Shahi Salman R, Hall JM, Horne MA, Moultrie F, Josephson CB, Bhattacharya JJ, et al. Untreated clinical course of cerebral cavernous malformations: a prospective, population-based cohort study. Lancet Neurol. 11:217–224. 2012.


5. Al-Shahi Salman R, Kitchen N, Thomson J, Ganesan V, Mallucci C, Radatz M, et al. Top ten research priorities for brain and spine cavernous malformations. Lancet Neurol. 15:354–355. 2016.


6. Aoki R, Srivatanakul K. Developmental venous anomaly: benign or not benign. Neurol Med Chir (Tokyo). 56:534–543. 2016.


8. Barker FG 2nd, Amin-Hanjani S, Butler WE, Lyons S, Ojemann RG, Chapman PH, et al. Temporal clustering of hemorrhages from untreated cavernous malformations of the central nervous system. Neurosurgery. 49:15–24. discussion 24-25. 2001.


9. Baumann CR, Schuknecht B, Lo Russo G, Cossu M, Citterio A, Andermann F, et al. Seizure outcome after resection of cavernous malformations is better when surrounding hemosiderin-stained brain also is removed. Epilepsia. 47:563–566. 2006.


10. Blumcke I, Spreafico R, Haaker G, Coras R, Kobow K, Bien CG, et al. Histopathological findings in brain tissue obtained during epilepsy surgery. N Engl J Med. 377:1648–1656. 2017.


11. Campeau NG, Lane JI. De novo development of a lesion with the appearance of a cavernous malformation adjacent to an existing developmental venous anomaly. AJNR Am J Neuroradiol. 26:156–159. 2005.
12. Casazza M, Broggi G, Franzini A, Avanzini G, Spreafico R, Bracchi M, et al. Supratentorial cavernous angiomas and epileptic seizures: preoperative course and postoperative outcome. Neurosurgery. 39:26–32. discussion 32-34. 1996.


13. Das A, Goyal A, Sangwan A, Bhalla AS, Kumar A, Kandasamy D, et al. Vascular anomalies: nomenclature, classification, and imaging algorithms. Acta Radiol. 64:837–849. 2023.


14. Detwiler PW, Porter RW, Zabramski JM, Spetzler RF. De novo formation of a central nervous system cavernous malformation: implications for predicting risk of hemorrhage. Case report and review of the literature. J Neurosurg. 87:629–632. 1997.


15. Flemming KD, Lanzino G. Cerebral cavernous malformation: what a practicing clinician should know. Mayo Clin Proc. 95:2005–2020. 2020.


16. Fontanella MM, Agosti E, Zanin L, di Bergamo LT, Doglietto F. Cerebral cavernous malformation remnants after surgery: a single-center series with long-term bleeding risk analysis. Neurosurg Rev. 44:2639–2645. 2021.


17. Giliberto G, Lanzino DJ, Diehn FE, Factor D, Flemming KD, Lanzino G. Brainstem cavernous malformations: anatomical, clinical, and surgical considerations. Neurosurg Focus. 29:E9. 2010.


18. Goyal A, Fernandes-Torres J, Flemming KD, Williams LN, Daniels DJ. Clinical presentation, natural history, and outcomes for infantile intracranial cavernous malformations: case series and systematic review of the literature. Childs Nerv Syst. 39:1545–1554. 2023.


19. Gross BA, Du R, Orbach DB, Scott RM, Smith ER. The natural history of cerebral cavernous malformations in children. J Neurosurg Pediatr. 17:123–128. 2016.


20. Hayashi S, Kondoh T, Morishita A, Sasayama T, Sanabria EA, Kohmura E. Congenital cavernous angioma exhibits a progressive decrease in size after birth. Childs Nerv Syst. 20:199–203. 2004.


21. Henrich W, Stupin JH, Bühling KJ, Bührer C, Bassir C, Dudenhausen JW. Prenatal sonographic findings of thalamic cavernous angioma. Ultrasound Obstet Gynecol. 19:518–522. 2002.


22. Horne MA, Flemming KD, Su IC, Stapf C, Jeon JP, Li D, et al. Clinical course of untreated cerebral cavernous malformations: a meta-analysis of individual patient data. Lancet Neurol. 15:166–173. 2016.


23. Jin Y, Zhao C, Zhang S, Zhang X, Qiu Y, Jiang J. Seizure outcome after surgical resection of supratentorial cavernous malformations plus hemosiderin rim in patients with short duration of epilepsy. Clin Neurol Neurosurg. 119:59–63. 2014.


24. Josephson CB, Rosenow F, Al-Shahi Salman R. Intracranial vascular malformations and epilepsy. Semin Neurol. 35:223–234. 2015.


25. Karaaslan B, Gülsuna B, Erol G, Dağli Ö, Emmez H, Kurt G, et al. Stereotactic radiosurgery for cerebral cavernous malformation: comparison of hemorrhage rates before and after stereotactic radiosurgery. J Neurosurg. 136:655–661. 2021.


26. Kondziolka D, Lunsford LD, Flickinger JC, Kestle JR. Reduction of hemorrhage risk after stereotactic radiosurgery for cavernous malformations. J Neurosurg. 83:825–831. 1995.


27. Lanfranconi S, Scola E, Meessen JMTA, Pallini R, Bertani GA, Al-Shahi Salman R, et al. Safety and efficacy of propranolol for treatment of familial cerebral cavernous malformations (Treat_CCM): a randomised, open-label, blinded-endpoint, phase 2 pilot trial. Lancet Neurol. 22:35–44. 2023.


28. Lerner A, Gilboa Y, Gerad L, Malinger G, Kidron D, Achiron R. Sonographic detection of fetal cerebellar cavernous hemangioma with inutero hemorrhage leading to cerebellar hemihypoplasia. Ultrasound Obstet Gynecol. 28:968–971. 2006.


29. Li W, Shenkar R, Detter MR, Moore T, Benavides C, Lightle R, et al. Propranolol inhibits cavernous vascular malformations by β1 adrenergic receptor antagonism in animal models. J Clin Invest. 131:e154909. 2021.


30. Lin F, Gao Z, Li C, Wang D, He Q, Kang D, et al. Intra-operative electrocorticography results and postoperative pathological findings are associated with epileptic outcomes in patients with cerebral cavernous malformations presenting with epilepsy. Epilepsy Res. 174:106672. 2021.


31. Lin Q, Yang PF, Jia YZ, Pei JS, Xiao H, Zhang TT, et al. Surgical treatment and long-term outcome of cerebral cavernous malformationsrelated epilepsy in pediatric patients. Neuropediatrics. 49:173–179. 2018.


32. Lunsford LD, Khan AA, Niranjan A, Kano H, Flickinger JC, Kondziolka D. Stereotactic radiosurgery for symptomatic solitary cerebral cavernous malformations considered high risk for resection. J Neurosurg. 113:23–29. 2010.


33. Mantziaris G, Dumot C, Pikis S, Peker S, Samanci Y, Ardor GD, et al. Pediatric cerebral cavernous malformations and stereotactic radiosurgery: an analysis of 50 cases from a multicentric study. J Neurosurg Pediatr. 4:315–322. 2024.


34. Morota N, Deletis V. Brainstem surgery: functional surgical anatomy with the use of an advanced modern intraoperative neurophysiological procedure. Adv Tech Stand Neurosurg. 48:21–55. 2023.


35. Oldenburg J, Malinverno M, Globisch MA, Maderna C, Corada M, Orsenigo F, et al. Propranolol reduces the development of lesions and rescues barrier function in cerebral cavernous malformations: a preclinical study. Stroke. 52:1418–1427. 2021.


36. Paddock M, Lanham S, Gill K, Sinha S, Connolly DJA. Pediatric cerebral cavernous malformations. Pediatr Neurol. 116:74–83. 2021.


37. Polster SP, Stadnik A, Akers AL, Cao Y, Christoforidis GA, Fam MD, et al. Atorvastatin treatment of cavernous angiomas with symptomatic hemorrhage exploratory proof of concept (AT CASH EPOC) trial. Neurosurgery. 85:843–853. 2019.


38. Porter RW, Detwiler PW, Spetzler RF. Surgical technique for resection of cavernous malformations of the brain stem. Oper Tech Neurosurg. 3:124–130. 2000.


39. Porter RW, Detwiler PW, Spetzler RF, Lawton MT, Baskin JJ, Derksen PT, et al. Cavernous malformations of the brainstem: experience with 100 patients. J Neurosurg. 90:50–58. 1999.


40. Rajeswarie RT, Aravinda HR, Arivazhagan A, Bevinahalli NN, Rao MB, Mahadevan A. Evaluating the role of perilesional tissue in pathobiology of epileptogenesis of vascular malformations of the central nervous system. J Epilepsy Res. 12:53–61. 2022.


41. Richardson BT, Dibble CF, Borikova AL, Johnson GL. Cerebral cavernous malformation is a vascular disease associated with activated RhoA signaling. Biol Chem. 394:35–42. 2013.


42. Rosenow F, Alonso-Vanegas MA, Baumgartner C, Blümcke I, Carreño M, Gizewski ER, et al. Cavernoma-related epilepsy: review and recommendations for management--report of the Surgical Task Force of the ILAE Commission on Therapeutic Strategies. Epilepsia. 54:2025–2035. 2013.


43. Santos AN, Rauschenbach L, Saban D, Chen B, Herten A, Dinger TF, et al. Natural course of cerebral cavernous malformations in children: a five-year follow-up study. Stroke. 53:817–824. 2022.


44. Shang-Guan HC, Wu ZY, Yao PS, Chen GR, Zheng SF, Kang DZ. Is extended lesionectomy needed for patients with cerebral cavernous malformations presenting with epilepsy? A meta-analysis. World Neurosurg. 120:e984–e990. 2018.
45. Shenkar R, Peiper A, Pardo H, Moore T, Lightle R, Girard R, et al. Rho kinase inhibition blunts lesion development and hemorrhage in murine models of aggressive Pdcd10/Ccm3 disease. Stroke. 50:738–744. 2019.
46. Shenkar R, Shi C, Austin C, Moore T, Lightle R, Cao Y, et al. RhoA kinase inhibition with fasudil versus simvastatin in murine models of cerebral cavernous malformations. Stroke. 48:187–194. 2017.
47. Shih YC, Chou CC, Peng SJ, Yu HY, Hsu SPC, Lin CF, et al. Clinical characteristics and long-term outcome of cerebral cavernous malformationsrelated epilepsy. Epilepsia. 63:2056–2067. 2022.
48. Shim Y, Phi JH, Wang KC, Cho BK, Lee JY, Koh EJ, et al. Clinical outcomes of pediatric cerebral cavernous malformation: an analysis of 124 consecutive cases. J Neurosurg Pediatr. 30:474–483. 2022.
50. Stavrou I, Baumgartner C, Frischer JM, Trattnig S, Knosp E. Long-term seizure control after resection of supratentorial cavernomas: a retrospective single-center study in 53 patients. Neurosurgery. 63:888–896. discussion 897. 2008.
51. Steiner L, Karlsson B, Yen CP, Torner JC, Lindquist C, Schlesinger D. Radiosurgery in cavernous malformations: anatomy of a controversy. J Neurosurg. 113:16–21. discussion 21-22. 2010.
52. Velz J, Özkaratufan S, Krayenbühl N, Beccaria K, Akeret K, Attieh C, et al. Pediatric brainstem cavernous malformations: 2-center experience in 40 children. J Neurosurg Pediatr. 29:612–623. 2022.


53. Willmore LJ, Sypert GW, Munson JB. Recurrent seizures induced by cortical iron injection: a model of posttraumatic epilepsy. Ann Neurol. 4:329–336. 1978.


54. Zevgaridis D, van Velthoven V, Ebeling U, Reulen HJ. Seizure control following surgery in supratentorial cavernous malformations: a retrospective study in 77 patients. Acta Neurochir (Wien). 138:672–677. 1996.


55. Zhang P, Zhang H, Shi C, Zhou J, Dong J, Liang M, et al. Clinical characteristics and risk factors of cerebral cavernous malformation-related epilepsy. Epilepsy Behav. 139:109064. 2023.


56. Zuurbier SM, Hickman CR, Rinkel LA, Berg R, Sure U, Al-Shahi Salman R, et al. Association between beta-blocker or statin drug use and the risk of hemorrhage from cerebral cavernous malformations. Stroke. 53:2521–2527. 2022.


57. Zuurbier SM, Santos AN, Flemming KD, Schmidt B, Jabbarli R, Lanzino G, et al. Female hormone therapy and risk of intracranial hemorrhage from cerebral cavernous malformations: a multicenter observational cohort study. Neurology. 100:e1673–e1679. 2023.
Fig. 1.
A 6-month-old infant presented with a recent onset of poor head control, which began 2 weeks prior to evaluation. At the time of presentation, her developmental milestones were within the expected range. No focal neurologic deficits were noted apart from the poor head control. MRI revealed a huge multicystic mass with a hemosiderin rim involving the left thalamus and midbrain (A and B). Mild ventriculomegaly was also observed. Additionally, T2-weighted images displayed small, hypointense lesions in the white matter of the right frontal and parietal lobes, and left occipital lobe, indicative of multiple CCMs (arrows) (C). Surgical intervention was performed via an interhemispheric transcallosal approach, resulting in the complete resection of the left thalamic CCM (D). Subsequently, the patient developed hydrocephalus necessitating ventriculoperitoneal shunt placement. Three months postoperatively, a follow-up MRI revealed a large cystic mass located at the right foramen of Monro and thalamus (arrow) (E). Furthermore, an increase in the size of preexisting CCMs in the right frontal and parietal lobes (arrows), and new lesion development in the right occipital horn were observed (F). The patient underwent multiple surgical procedures for growing hemorrhagic lesions. Genetic analysis identified an inactivating germline mutation of the CCM3 gene in both the patient and her father. MRI : magnetic resonance image, CCM : cerebral cavernous malformation.
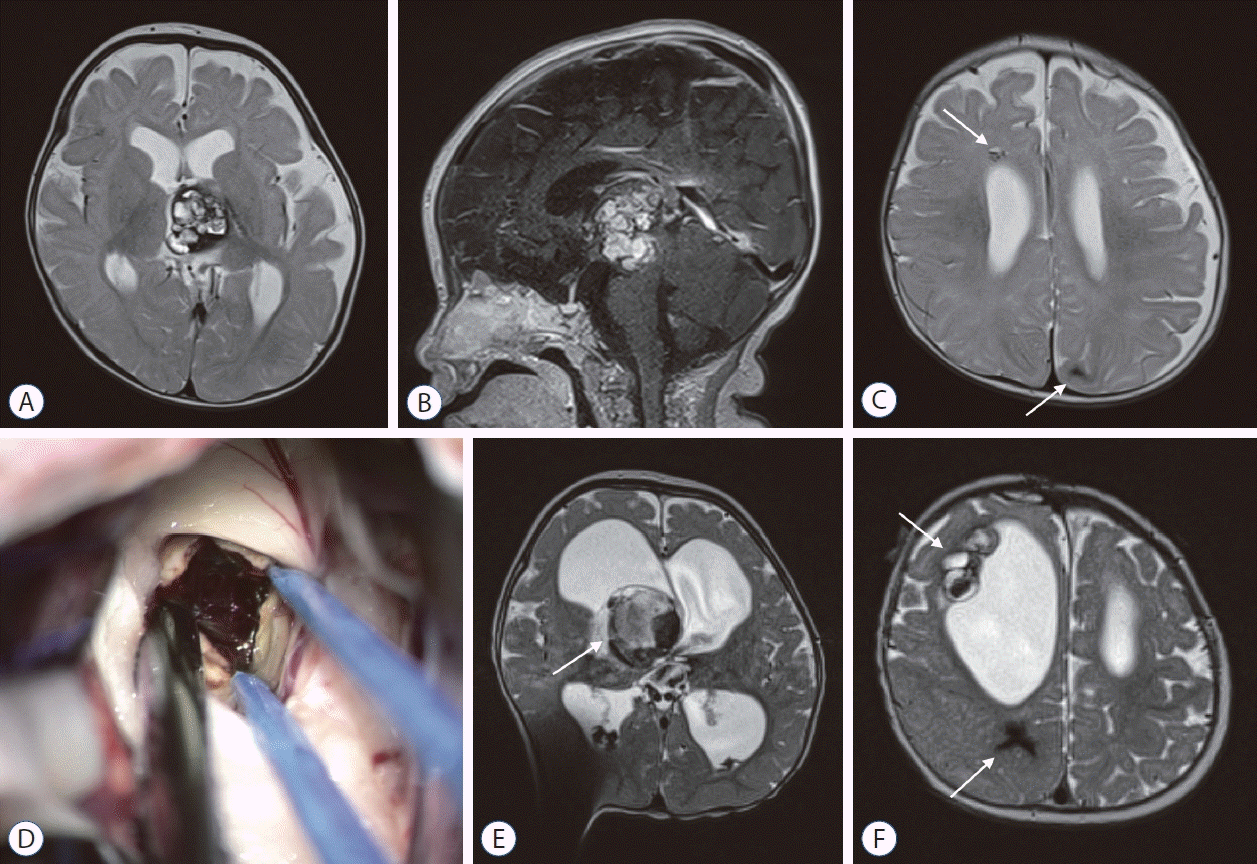
Fig. 2.
A 13-month-old female presented with acute onset of left 6th nerve palsy. Initial CT scan revealed acute round-shaped bleeding in the left pontine tegmentum (A). Conservative management led to mild perilesional edema observed in an MRI taken 1 week later (B). However, at the 10th day post-initial event, the patient developed right hemiparesis, with MRI showing increased hemorrhage size and development of a hemosiderin rim around the core (C and D). Surgical removal of the pontine cavernous malformation was indicated. Under intraoperative monitoring, a suboccipital telovelar approach was performed to dispose of the fourth ventricle floor, with the cavernous malformation exposed in the upper pons (E). The fourth ventricle floor was severely distorted by the underlying mass and midline sulcus could not be identified. Floor mapping identified critical landmarks, and the exposure hole of cavernous malformation was located 4 mm above the left facial colliculus. After removal of subacute hematoma, the mass was completely removed in pieces via gentle dissection from the cavity wall (F and G). The patient experienced gradual recovery of hemiparesis and 6th nerve palsy during follow-up. CT : computed tomography, MRI : magnetic resonance image.
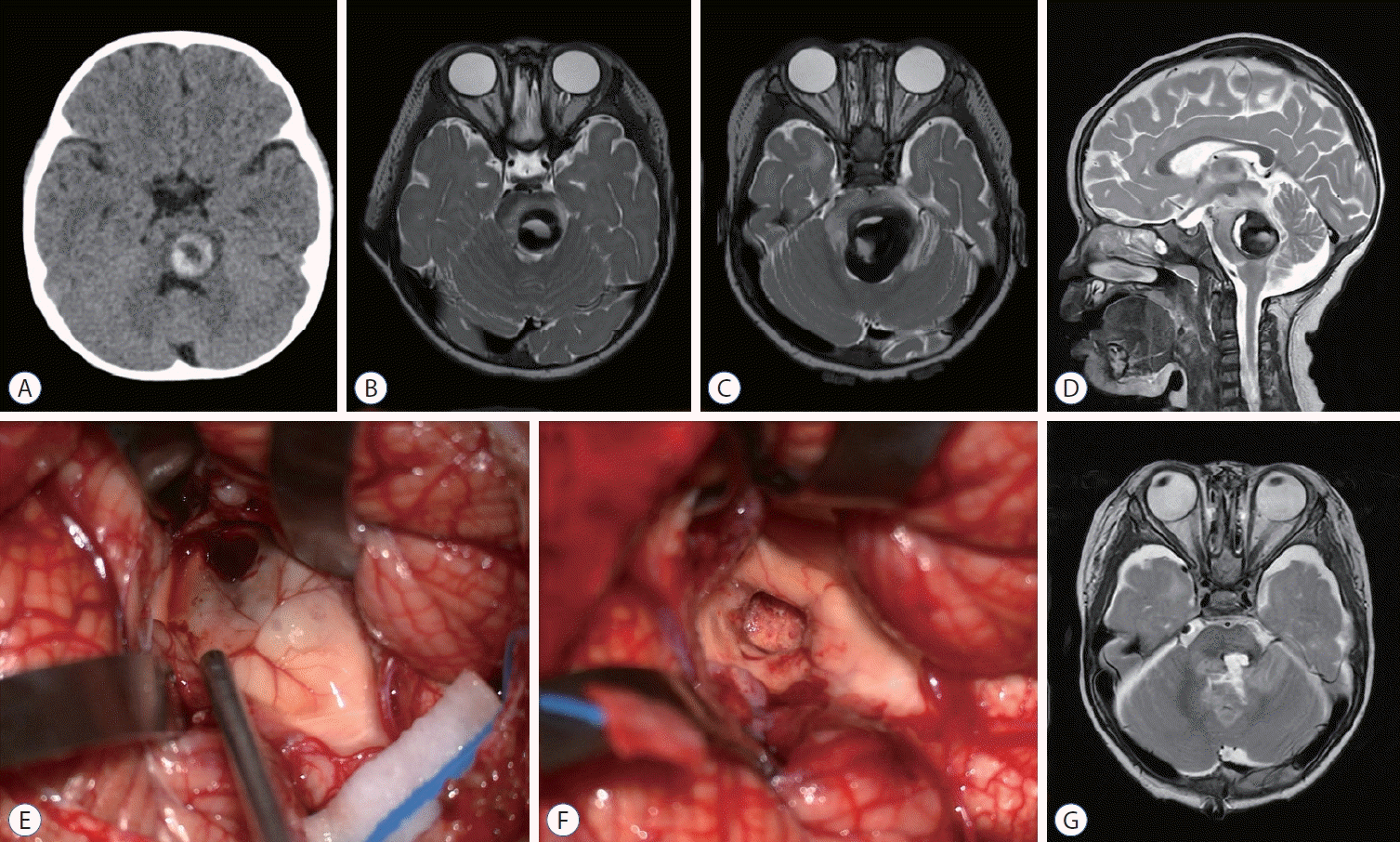