Abstract
Background
This study aimed to retrospectively assess results of intracranial meningioma surgery with or without intraoperative neuromonitoring (IONM) in a single institution.
Methods
Two cohorts (a historical cohort and a monitoring cohort) were collected for the analysis. Before IONM was introduced, a total of 107 patients underwent intracranial meningioma operation without IONM from January 2000 to December 2008 by one neurosurgeon (historical cohort). After IONM was introduced, a total of 99 patients with intracranial meningioma were operated under IONM between November 2018 and February 2023 by two neurosurgeons (monitoring cohort). A retrospective comparison was made on the complications from meningioma surgery between the two groups.
Results
In the monitoring cohort, warning signals of motor evoked potential (MEPs) or somatosensory evoked potential (SSEPs) were alarmed in 10 patients. Two of these 10 patients aborted the operation and eight of these 10 patients with warning signals underwent tumor resection. Of these eight patients, five showed postoperative morbidity. Five of 89 patients without warning signals developed neurological deficits. In the historical cohort, 14 of 107 patients showed postoperative morbidity or mortality.
Meningiomas are among the most common intracranial tumors. They account for approximately 35.5% of all primary brain tumors in adults [1]. Surgical resection is the primary treatment modality for these tumors. Complete removal while preserving neurological function is the ultimate goal.
In recent years, there has been growing interest in the use of intraoperative neuromonitoring (IONM) during surgery of brain tumor to enhance patient safety and optimize surgical outcomes [2]. Previous reports have offered insights into the effectiveness of IONM in the resection of intra-axial tumors near eloquent areas of the central nervous system such as gliomas [345]. IONM has also shown considerable promise in various neurosurgical procedures [67].
The use of IONM for the resection of intracranial meningiomas has been reported. Policicchio et al. [8] have managed infiltrative lesions of the sulcus centralis not only by IONM, but also by 3D ultrasound to identify the tumor-tissue interface. Awake craniotomies for meningioma resection may maximize resection safety and result in earlier patient recovery with reduced length of hospital stay and decreased costs [9]. However, IONM is not considered a routine clinical practice for the removal of cranial extra-axial lesions, especially for supratentorial lesions not involving cranial nerves.
The objective of the present study was to retrospectively investigate results of intracranial meningioma surgery with or without IONM to assess the utility of its usage for meningioma surgery.
The database was searched retrospectively for patients who underwent resection of meningioma at our institution. The study was conducted in accordance with the Institutional Review Board of St. Vincent’s Hospital (VC17TESI0080). The requirement of prior patient consent was waived. Patients were divided into two cohorts: 1) those who underwent resection without IONM, and 2) those who underwent resection with IONM. Before IONM was introduced, a total of 107 patients with intracranial meningiomas underwent resection without IONM from January 2000 to December 2008 by one neurosurgeon (historical cohort). A total of 99 patients with intracranial meningioma underwent resection under IONM between November 2018 and February 2023 by two neurosurgeons (monitoring cohort). A retrospective comparison was made on the incidence of complications from meningioma surgery between the two groups.
IONM was performed with Xltek Protektor32 (Natus Medical Inc., Pleasanton, CA, USA) under total intravenous anesthesia (TIVA) by a trained electrophysiology team. Motor evoked potentials (MEPs) and somatosensory evoked potentials (SSEPs) were monitored for all patients. Brainstem auditory evoked potential, visual evoked potential, or facial nerve was monitored depending on the location of the tumor, such as skull base or posterior fossa meningiomas. For MEPs, a constant voltage stimulator was used for transcranial electrical stimulation performed through a pair of spiral scalp electrodes using 3–5 pulses of 200–400 V with an interstimulus interval of 1–3 ms. Each pulse was 50 µs in duration. Muscle responses were recorded by subdermal needle electrodes placed in or over the following muscle groups bilaterally: flexor carpi radialis and flexor carpi ulnaris (arm); thenar and hypothenar muscles (hand); tibialis anterior (leg); medial gastrocnemius (leg); and abductor hallucis and abductor digiti minimi (foot). Neurological status was assessed preoperatively, during the hospitalization, and 3 to 6 months postoperatively by neurosurgeons. A warning sign of MEP and SSEP was defined as a decrease in amplitude by 50% or a prolongation of latency by 10% or 2.5 µs. Neurological impairments including motor deficits, sensory disturbances like paresthesia, and cranial nerve dysfunction were documented and evaluated in conjunction with IONM data. Mortality (death) was also included. Those with subdural hemorrhage, abscess/empyema, or cerebrospinal fluid leakage were excluded.
The monitoring cohort included 27 males and 72 females (M:F ratio=1:2.7). Their mean age was 60.4 years (range, 25 to 85 years). Locations of meningiomas included cerebral convexity (28%), parasagittal (24%), cerebellopontine angle (8%), falx (8%), and tuberculum sellae (7%). The maximal diameter of the tumor had a mean value of 36.9 mm (range, 12 to 80 mm). Simpson grades I, II, III, and IV resections were achieved in 13%, 65%, 4%, and 18%, respectively. Histological diagnosis was WHO grade I in 85%, WHO grade 2 in 13%, and WHO grade 3 in 2% (Table 1).
Baseline MEPs and SSEPs were not elicited in three patients. One patient woke up during TIVA. Therefore, MEPs and SSEPs monitoring were successfully recorded in 99 patients. Warning signal of MEPs or SSEPs was alarmed in 10 patients. Two of these 10 patients aborted the operation. Of these two patients, one died due to postoperative edema and one developed cranial nerve palsy. Eight of the 10 patients with a warning sign underwent tumor resection. Of them, five had postoperative morbidity, including four patients who had hemiparesis and one patient who had paresthesia.
Eighty-nine patients completed tumor resection without any warning signals. However, hemiparesis, cranial nerve palsy, and paresthesia developed in 1, 1, and 3 patients, respectively (Fig. 1). Table 2 provides a detailed summary of patients who experienced postoperative deficits in the monitoring cohort.
Meningiomas located in the perirolandic cortex showed a high likelihood of postoperative complications even in the IONM group.
A total of 107 patients with meningioma underwent resection without IONM. Their characteristics are summarized in Table 3. Common locations of meningioma were cerebral convexity (27%), parasagittal (23%), and sphenoid wing (16%). Common pathology of meningiomas were meningothelial (44%), fibrous (20%), and transitional (19%). Postoperatively, 5, 5, 2, and 2 patients had hemiparesis, cranial nerve palsy, paresthesia, and death, respectively.
A 66-year-old woman presented with several episodes of transient weakness on the left hand. Preoperative MR images showed an extra-axial mass with peritumoral edema adjacent to the right frontal motor cortex (Fig. 2A). Craniotomy and total resection (Simpson grade II) were performed. MEPs change did not occur during the process. However, a warning sign of SSEPs was identified (Fig. 2B). Grade 3 motor power of the left lower limb was found postoperatively. The proprioception of the left foot was impaired. Postoperative diffusion MR image showed restriction around the resection cavity (Fig. 2C). The weakness was fully recovered 5 months postoperatively, although impaired proprioception remained.
A 66-year-old woman presented with facial hypoesthesia. Preoperative MR images showed a well enhancing extra-axial mass lesion in the right prepontine cistern (Fig. 3A and B). A lateral suboccipital craniotomy was done for the resection. The right abducens nerve was sacrificed during tumor removal. Warning signs of both MEPs and SSEPs were elicited. Vital signs, status of anesthesia, laboratory findings, and surgical factors were evaluated. Although we temporarily halted the procedure and waited for the recovery of IONM, both MEPs and SSEPs showed no change (Fig. 3C and D). We decided to abort the procedure. The patient developed stuporous mentality and recovered gradually to alertness at 4 months after the surgery. Sequalae of the left hemiparesis and lower cranial nerve palsy were identified.
In 99 cases of meningioma surgery using IONM, warning signs in MEP or SSEP occurred in 10 patients. Of those, eight proceeded with surgery. Of them, five showed neurological deficits. Even in 89 patients without warning signs, neurological deficits occurred in five patients postoperatively. Thus, out of 99 patients, 10 developed neurological deficits that differed from IONM’s predictions. The sensitivity and specificity of transcranial MEP monitoring for brain tumor operations have been reported to be approximately 75% and 77.8%, respectively [10]. They are relatively low compared to those of IONM for spinal operations and aneurysmal operations. Sensitivity for aneurysm surgery is higher than that for brain tumor surgery because vascular compromise directly affects the corticospinal tract [11]. Deep white matter stimulation by transcranial stimulation seems to be one of the reasons why false negatives have happened more in patients undergoing brain than in spinal surgeries [12]. In our series, postoperative neurological deficit was caused by two reasons: vascular injury and direct parenchymal injury. Vascular injury is more concerning than direct injury for lesions that are extrinsic to the brain.
An insult to the venous system during meningioma surgery adjacent to the central sulcus is highly associated with neurological deficit [131415]. One patient with a warning signal of SSEP developed paresthesia postoperatively. Three patients without any warning signals of SSEP or MEP unfortunately developed paresthesia. Postoperative diffusion MRI showed a high signal adjacent to the primary sensory cortex, which was thought to be a possible cause of postoperative neurological deficit [16]. During surgical procedures, the emergence of ischemic insults can prompt alterations in IONM parameters. These changes can serve as critical indicators for neurosurgeons who can then take immediate corrective actions such as mitigating brain retraction, optimizing hemodynamic parameters by elevating blood pressure, or halting tumor dissection to prevent further neural compromise.
Vascular insufficiency and venous infarction often develop postoperatively and give rise to neurological deterioration 1 or 2 days later [17]. Loss of proprioception and paresthesia make patients hard to walk. Although the motor function is intact on examination, patients feel very uneasy when walking outdoors and experience awkwardness when ascending or descending stairs. Therefore, it is imperative for neurosurgeons to react promptly to alterations observed in SSEPs. Perilesional edema and bleeding can be causes of false negative, which is clinically more important [12]. In gliomas, peri- or postoperative ischemic lesions play crucial roles in the development of surgery-related motor deficits [18]. Umemura et al. [19] have suggested that stimulation intensity of monitoring should be minimized to accurately reflect the point of operation, improve sensitivity, and reduce the risk of postoperative paralysis.
Next, we examined results of meningioma surgeries performed during the period without IONM from January 2000 to December 2008. The average age of the historical cohort was 53.8 years, younger than that of the monitoring cohort. The location of the tumor and pathological classification were similar between the two cohorts. It was difficult to compare the size and extent of tumor resection because past imaging data were unavailable. It was noted that 12 (11%) of 107 patients developed neurological morbidity or mortality in the historical cohort compared with 12 (12%) of 99 patients in the monitoring cohort. Such similarity suggests that the complexity of meningioma surgery itself rather than the decreased utility of IONM is the main challenge [20]. Differences in the experience of the surgeons may be relevant. Successful meningioma removal can be achieved by experienced surgeons even without the help of IONM. In the monitoring cohort, two operations with IONM warning were aborted during surgery. One patient was described in case 2 and another one died because of severe brain edema postoperatively. Cessation of operation is a rare occasion for any reason. It was noteworthy that other factors associated with the loss of IONM were considered before making the intraoperative decision to abort the operation. Ostrý et al. [21] have evaluated IONM during resection of Rolandic area meningiomas, describing its utility as a decision-supporting tool to continue or stop the resection when the cleavage plane is lost. Eichberg et al. [22] have made similar use of IONM for the resection of parasagittal and falx meningiomas. However, they experienced patients who developed postoperative foot drop with preserved MEPs. A patient awoke postoperatively neurologically intact, but developed a venous infarct with hemorrhagic conversion with resulting hemiplegia. Paldor et al. [23] have investigated IONM’s impact on surgical workflow in supratentorial meningiomas. Their rates of postoperative deficit were as follows: an overall new deficit rate of 12.5% and a fixed deficit rate of 2.5%. In one-third of cases, IONM enabled resection of the part of the tumor overlying the motor area after maximal debulking. Therefore, a safer resection with less manipulation of eloquent areas can be performed. The rate of temporary deficit was more than halved when IONM-induced workflow was undertaken. They suggested a novel classification system of interventions or modifications due to IONM during the operation. We agree with them that IONM-guided decision is useful with an impact during meningioma surgery in eloquent areas. There is a need to develop a protocol that neurosurgeons can agree upon through more research to respond appropriately to constantly changing IONM data.
Although IONM is increasingly employed to enhance neurosurgical procedures, our analysis indicates that determining its superiority in terms of safety for meningioma resections is challenging. Although data suggest its potential benefits, the complexity of these surgeries and the variability of tumor presentations necessitate more extensive research to solidify these findings.
In conclusion, IONM helps neurosurgeons determine the surgical technique and approach for resecting an intracranial meningioma. However, further research is necessary to develop a protocol guiding how to respond to changing IONM data.
Notes
Author Contributions:
Conceptualization: Seung Ho Yang.
Data curation: Na Il Shin, Hye Jin Hong.
Formal analysis: Young Il Kim.
Funding acquisition: Seung Ho Yang.
Investigation: Il Sup Kim.
Methodology: Jae Hoon Sung.
Project administration: Seung Ho Yang.
Resources: Sang Won Lee.
Software: Na Il Shin.
Supervision: Sang Won Lee.
Validation: Young Il Kim.
Visualization: Na Il Shin.
Writing—original draft: Na Il Shin.
Writing—review & editing: Seung Ho Yang.
Availability of Data and Material
The data generated during and/or analyzed during the study are available from the corresponding authors on reasonable request.
References
1. Jung KW, Ha J, Lee SH, Won YJ, Yoo H. An updated nationwide epidemiology of primary brain tumors in Republic of Korea. Brain Tumor Res Treat. 2013; 1:16–23. PMID: 24904884.


2. Wiedemayer H, Fauser B, Sandalcioglu IE, Schäfer H, Stolke D. The impact of neurophysiological intraoperative monitoring on surgical decisions: a critical analysis of 423 cases. J Neurosurg. 2002; 96:255–262. PMID: 11838799.


3. Bello L, Comi A, Riva M, Pessina F, Alfiero T, Raneri F, et al. Glioma surgery: tailoring intraoperative neurophysiological strategies to clinical conditions enhances resection, extends indications, and keeps patient functional integrity. Neuro Oncol. 2014; 16(suppl 3):iii47.


4. Nossek E, Korn A, Shahar T, Kanner AA, Yaffe H, Marcovici D, et al. Intraoperative mapping and monitoring of the corticospinal tracts with neurophysiological assessment and 3-dimensional ultrasonography-based navigation: clinical article. J Neurosurg. 2011; 114:738–746. PMID: 20799862.


5. You H, Qiao H. Intraoperative neuromonitoring during resection of gliomas involving eloquent areas. Front Neurol. 2021; 12:658680. PMID: 34248818.


6. Kothbauer K, Deletis V, Epstein FJ. Intraoperative spinal cord monitoring for intramedullary surgery: an essential adjunct. Pediatr Neurosurg. 1997; 26:247–254. PMID: 9440494.
7. Lall RR, Lall RR, Hauptman JS, Munoz C, Cybulski GR, Koski T, et al. Intraoperative neurophysiological monitoring in spine surgery: indications, efficacy, and role of the preoperative checklist. Neurosurg Focus. 2012; 33:E10.


8. Policicchio D, Ticca S, Dipellegrini G, Doda A, Muggianu G, Boccaletti R. Multimodal surgical management of cerebral lesions in motor-eloquent areas combining intraoperative 3D ultrasound with neurophysiological mapping. J Neurol Surg A Cent Eur Neurosurg. 2021; 82:344–356. PMID: 33352612.
9. Bakhshi SK, Jawed N, Shafiq F, Enam SA. Awake craniotomy for resection of intracranial meningioma: first case series from a low- and middle-income country. Cureus. 2021; 13:e18716. PMID: 34790471.
10. Tanaka S, Tashiro T, Gomi A, Takanashi J, Ujiie H. Sensitivity and specificity in transcranial motor-evoked potential monitoring during neurosurgical operations. Surg Neurol Int. 2011; 2:111. PMID: 21886884.


11. Kang D, Yao P, Wu Z, Yu L. Ischemia changes and tolerance ratio of evoked potential monitoring in intracranial aneurysm surgery. Clin Neurol Neurosurg. 2013; 115:552–556. PMID: 22795547.


12. Lee JJ, Kim YI, Hong JT, Sung JH, Lee SW, Yang SH. Intraoperative monitoring of motor-evoked potentials for supratentorial tumor surgery. J Korean Neurosurg Soc. 2014; 56:98–102. PMID: 25328645.


13. Cai Q, Wang S, Zheng M, Wang X, Liu R, Liu L, et al. Risk factors influencing cerebral venous infarction after meningioma resection. BMC Neurol. 2022; 22:259. PMID: 35831795.


14. Ottenhausen M, Rumalla K, Younus I, Minkowitz S, Tsiouris AJ, Schwartz TH. Predictors of postoperative motor function in rolandic meningiomas. J Neurosurg. 2018; 130:1283–1288. PMID: 29799346.


15. Strand PS, Sagberg LM, Gulati S, Solheim O. Brain infarction following meningioma surgery-incidence, risk factors, and impact on function, seizure risk, and patient-reported quality of life. Neurosurg Rev. 2022; 45:3237–3244. PMID: 35902426.


16. Magill ST, Nguyen MP, Aghi MK, Theodosopoulos PV, Villanueva-Meyer JE, McDermott MW. Postoperative diffusion-weighted imaging and neurological outcome after convexity meningioma resection. J Neurosurg. 2021; 135:1008–1015. PMID: 33513570.


17. Nakase H, Shin Y, Nakagawa I, Kimura R, Sakaki T. Clinical features of postoperative cerebral venous infarction. Acta Neurochir (Wien). 2005; 147:621–626. PMID: 15770350.


18. Dützmann S, Geßler F, Bink A, Quick J, Franz K, Seifert V, et al. Risk of ischemia in glioma surgery: comparison of first and repeat procedures. J Neurooncol. 2012; 107:599–607. PMID: 22249690.


19. Umemura T, Nishizawa S, Nakano Y, Saito T, Kitagawa T, Miyaoka R, et al. Intraoperative monitoring of motor-evoked potential for parenchymal brain tumor removal: an analysis of false-negative cases. J Clin Neurosci. 2018; 57:105–110. PMID: 30145081.


20. Cabraja M, Stockhammer F, Mularski S, Suess O, Kombos T, Vajkoczy P. Neurophysiological intraoperative monitoring in neurosurgery: aid or handicap? An international survey. Neurosurg Focus. 2009; 27:E2.
21. Ostrý S, Netuka D, Beneš V. Rolandic area meningioma resection controlled and guided by intraoperative cortical mapping. Acta Neurochir (Wien). 2012; 154:843–853. PMID: 22426820.


22. Eichberg DG, Casabella AM, Menaker SA, Shah AH, Komotar RJ. Parasagittal and parafalcine meningiomas: integral strategy for optimizing safety and retrospective review of a single surgeon series. Br J Neurosurg. 2020; 34:559–564. PMID: 31284785.


23. Paldor I, Doron O, Peso D, Jubran M, Sviri GE. Intraoperative neuromonitoring during resection of cranial meningiomas and its effect on the surgical workflow. Neurosurg Rev. 2022; 45:1481–1490. PMID: 34632555.


Fig. 1
Summary of intraoperative neuroneuromonitoring changes and postoperative morbidities. MEP, motor evoked potential; SSEP, somatosensory evoked potential.
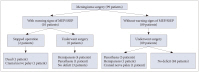
Fig. 2
A case with warning sings of somatosensory evoked potentials. A: Enhanced T1-weighted axial image showing a 23×30-mm-sized extra-axial mass adjacent to the right central sulcus. B: Intraoperative somatosensory evoked potentials were alarmed during the operation. C: Postoperative diffusion MR image showing restriction around the resection cavity.
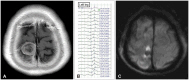
Fig. 3
A case of warning signs of motor evoked potentials and somatosensory evoked potentials. A and B: Enhanced T1-weighted and T2-weighted axial image showing 32×38-mm-sized and well enhancing extra-axial mass in the right prepontine cistern. This lesion abuts posterior aspect of clivus and compresses the pons. Diffuse dilatation of the ventricular system is noted. C and D: Intraoperative motor evoked potentials and somatosensory evoked potentials were alarmed but disappeared during operation. Decreased amplitudes are highlighted in red square with arrows.
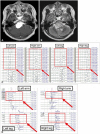
Table 1
Characteristics of monitoring cohort
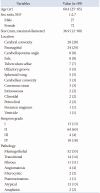
Table 2
Summary of patients with postoperative deficits
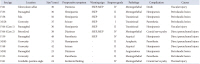
Table 3
Characteristics of historical cohort
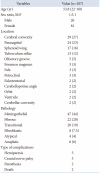