Abstract
Background
Urinary tissue inhibitor of metalloproteinase-2 (TIMP-2) and insulin-like growth factor-binding protein 7 (IGFBP7) are G1 cell arrest biomarkers that have demonstrated accuracy and validity in predicting and diagnosing acute kidney injury (AKI). This study aimed to evaluate the validity of [TIMP-2]×[IGFBP7] in diagnosing acute allograft dysfunction and its utility in distinguishing acute rejection (AR) from nonrejection causes in kidney transplantation.
Methods
This study included 48 adult living donor kidney transplant recipients (KTRs; 18 with AR, 15 with nonrejection causes of AKI, and 15 with stable grafts). Urinary TIMP-2 and IGFBP7 were measured, and [TIMP-2]×[IGFBP7] was calculated in all subjects.
Results
IGFBP7, TIMP-2, and [TIMP-2]×[IGFBP7] were statistically significantly higher in KTRs with acute allograft dysfunction than in those with stable grafts. [TIMP-2]×[IGFBP7] was statistically significantly higher in KTRs with AR than in those with nonrejection AKI. [TIMP-2]×[IGFBP7] at a cutoff level of 0.278 (ng/mL)2/1,000 had an area under the curve (AUC) of 0.99 with a sensitivity of 100% and a specificity of 93.3% in diagnosing acute allograft dysfunction, while at a cutoff level of 0.803 (ng/mL)2/1,000 had an AUC of 0.939 with a sensitivity of 94.4% and a specificity of 83.3% in diagnosing AR.
Renal transplantation is the preferred treatment for patients with end-stage renal disease (ESRD). Acute rejection (AR) remains an important risk factor for renal allograft loss [1,2]. The diagnosis of AR still relies only on histopathological examinations; however, graft biopsy is an invasive procedure that may be associated with serious adverse events [3]. Serum creatinine (sCr) is a delayed and insensitive biomarker of changes in kidney function that does not indicate the severity of the injury until a steady state has been reached in an acute kidney injury (AKI). Moreover, its concentration does not differentiate between structural kidney damage and functional hemodynamic triggers, and it can be affected by many factors [4]. The Translational Research Investigating Biomarker Endpoints in AKI (TRIBE-AKI) study evaluated the performance of different urinary biomarkers in the diagnosis of AKI in the native kidney upon entry to the intensive care unit (ICU); no biomarker had an area under the curve (AUC) above 0.7 in the diagnosis or prediction of AKI [5]. Induction of cell-cycle arrest is not only associated with increased risk for AKI, but may also serve as a mechanistic link between AKI and CKD, since sustained cell-cycle arrest results in a senescent cell phenotype and leads to fibrosis [6]. Tissue inhibitor of metalloproteinase-2 (TIMP-2) has a molecular weight of approximately 24 kDa, and insulin-like growth factor-binding protein 7 (IGFBP7) has a molecular mass of 29 kDa [7]. Both of these proteins are expressed and secreted by renal tubular cells, and are involved in G1 cell cycle arrest during the early phases of cellular stress or injury caused by various insults (e.g., sepsis, ischemia, oxidative stress, and toxins) [8]. The urinary [TIMP-2]×[IGFBP7] biomarker was approved by the Food and Drug Administration (FDA) in 2014 and is commercially available in the United States (NephroCheck VR, Ortho Clinical Diagnostics). The accuracy and validity of [TIMP-2]×[IGFBP7] as a biomarker in predicting AKI have been proven in multiple studies with different populations and different clinical situations, such as patients with cardiac arrest, cardiac and major surgery, acute decompensated heart failure, and delayed graft dysfunction (DGF) following deceased-donor transplantation [9].
Some researchers have suggested that [TIMP-2]×[IGFBP7] for the prediction of AKI might be most applicable in patients at high risk of AKI as kidney transplant recipients (KTRs) and less accurate in those at lower risk [10]. Therefore, the identification and validation of diagnostic and prognostic biomarkers that are less invasive and more sensitive, and that could potentially replace graft biopsy in diagnosing AR, are of significant value. The role of these biomarkers in distinguishing the causes of acute allograft dysfunction, however, remains to be investigated. In this study, we examined the potential role of [TIMP-2]×[IGFBP7] in differentiating AR from other causes of acute allograft dysfunction in early KTRs.
The clinical study protocol was approved by the local Ethics Committee of the Faculty of Medicine, Cairo University (No. MS 17-5-17), in compliance with the Helsinki Declaration II, Finland. Written informed consent was obtained from all participants.
The current study is a single-center, observational cross-sectional study designed to evaluate the sensitivity and specificity of urinary biomarkers for cell cycle arrest, namely TIMP-2 and IGFBP7, in diagnosing acute allograft dysfunction and AR from other causes of AKI in KTRs. We recruited 48 adult living KTRs from the kidney transplantation (KT) outpatient clinic at the Cairo University Hospitals. All subjects underwent living donor KT, with a posttransplantation duration ranging from 1 to 6 months, and had a baseline sCr level of less than 1.5 mg/dL. KTRs with comorbidities that could potentially influence the results, such as diabetes mellitus, liver disease, heart failure, peripheral vascular disease, urinary tract infection, proteinuria, or any abnormal urine analysis findings like albumin and bilirubin, were excluded. Additionally, those with a recurrence of the original disease or who had received multiple transplants were also excluded.
The subjects were categorized into three groups: group I consisted of 18 KTRs experiencing acute allograft dysfunction, with a graft biopsy indicating acute cellular rejection; group II comprised 15 KTRs with acute allograft dysfunction but without rejection-induced AKI; and group III included 15 KTRs with stable allograft functions, characterized by a stable sCr level ranging from 0.5 mg/dL to 1.5 mg/dL. The standard immunosuppression regimen included a calcineurin inhibitor (either cyclosporine or tacrolimus), mycophenolate mofetil, and glucocorticoids. Acute graft dysfunction was identified by a sudden increase in sCr of more than 25% from the baseline. AR was diagnosed and classified according to the Banff 2019 classification [11]. T cell-mediated rejection (TCMR) is an inflammatory response primarily affecting renal tubules and arterioles, mediated by T cell CD8+, and typically peaks around 3 months postoperation. Urine samples were collected from each study participant diagnosed with AKI prior to graft biopsy or the administration of antirejection treatment, and were then frozen at −80 °C for subsequent analysis.
Levels of TIMP-2 and IGFBP7 were assessed in urine samples, following the manufacturer's instructions for the enzyme-linked immunosorbent assay (ELISA). The specific kits used were the Human TIMP2 ELISA Kit (Abcam), and the Human IGFBP7 ELISA Kit (Biorbyt). The ELISA procedure was typically completed within an approximate timeframe of 4 hours. To account for variations in urine concentration, TIMP-2 levels in urine were expressed in ng/mg creatinine, while IGFBP7 levels were expressed in pg/mg creatinine. The results were then multiplied ([TIMP-2]×[IGFBP7]), yielding a value expressed in (ng/mL)2/1,000.
Statistical analysis was conducted using MedCalc (MedCalc Software). We used summary statistics, which included the mean±standard error. Categorical data were summarized using both absolute and relative frequencies. The chi-square test was employed to compare the categorical variables (frequencies) of various groups. To compare ordinal data from different groups, we used the independent-samples t-test. Univariate regression analysis was utilized to test the association of each marker as an independent variable against other variables. The correlation coefficient was used to define the strength and direction of the linear relationship between two variables. A P-value of <0.05 was deemed statistically significant, while a P-value of <0.001 was considered highly statistically significant. We used receiver operating curve (ROC) curve analysis to measure the sensitivity, specificity, optimum cutoff point (using the Youden index), and the AUC of candidate markers in diagnosing acute allograft dysfunction and AR. Predictive values were then calculated from the data obtained through the ROC analysis.
The demographic, clinical, and laboratory characteristics of patients and healthy controls are summarized in Table 1. The study included 48 adult living donor KTRs: 18 KTRs with AR (average, sCr 5.01±0.71 mg/dL), 15 KTRs with nonrejection AKI (average, sCr 2.71±0.38 mg/dL), and 15 KTRs with stable graft function (average, sCr 0.86±0.06 mg/dL). The cohort consisted of 12 females (five with AR, three with nonrejection AKI, and four with stable graft function) and 36 males (13 with AR, 12 with nonrejection AKI, and 11 with stable graft function). Hypertension was present in 41 of the total cohort (15 with AR, 13 with nonrejection AKI, and 13 with stable graft function). The 18 subjects with AR were categorized according to the Banff 2019 classification histological grades as follows: three were grade IA, two were grade IB, five were grade IIA, two were grade IIB, and six were grade III. The 15 subjects with nonrejection causes of acute allograft dysfunction had the following pathological diagnoses: eight had acute tubular necrosis, six had calcineurin-induced nephrotoxicity, and one had BK virus-induced pathological effects.
Urinary IGFBP7 and TIMP-2 levels, as well as [TIMP-2]×[IGFBP7] levels, were all significantly higher in KTRs with acute allograft dysfunction (n=33) than in KTRs with stable grafts (n=15) and significantly higher in the AR group than in the nonrejection AKI group (Fig. 1). A statistically significant positive correlation was also observed between each of the IGFBP7 and TIMP-2 markers and sCr (r=0.3055, P<0.001 and r=0.2999, P<0.001, respectively).
The diagnostic capabilities of both markers in identifying acute allograft dysfunction were evaluated using ROC curve analysis. The results showed that IGFBP7 could diagnose acute allograft dysfunction with a sensitivity of 97.0%, a specificity of 93.3%, and an AUC of 0.97 at a cutoff level of 0.65 pg/mg creatinine. TIMP-2 demonstrated a sensitivity of 69.7%, a specificity of 100%, and an AUC of 0.91 for diagnosing acute allograft dysfunction at a cutoff level of 519.3 ng/mg creatinine. [TIMP-2]×[IGFBP7], at a cutoff level of 0.278 (ng/mL)2/1,000 exhibited a sensitivity of 100%, a specificity of 93.3%, and an AUC of 0.99 for diagnosing acute allograft dysfunction (Fig. 2). The calculated predictive values for IGFBP7, TIMP-2, and [TIMP-2]×[IGFBP7] revealed that TIMP-2 had the highest positive predictive value, while [TIMP-2]×[IGFBP7] had the highest negative predictive value (Table 2).
Multiple regression analysis was done in the AR group to determine whether creatinine, the estimated glomerular filtration rate (eGFR), urea, and age could positively predict [TIMP-2]×[IGFBP7]. The results showed a nonsignificant effect (P=0.495, F=0.980, and R2=0.407) and in the nonrejection AKI group, [TIMP-2]×[IGFBP7] was not predicted by creatinine, eGFR, urea, or age (P=0.503, F=0.995, and R2=0.499). Furthermore, in the stable graft group, [TIMP-2]×[IGFBP7] was not predicted by creatinine, eGFR, urea, or age (P=0.985, F=0.167, and R2=0.143) (Table 3).
Regarding the diagnostic ability of both markers in diagnosing AR, the ROC curve analysis revealed that IGFBP7 was able to diagnose AR with a sensitivity of 77.8%, a specificity of 86.7%, and an AUC of 0.881 at a cutoff level of 1.3 pg/mg creatinine. TIMP-2 was able to diagnose AR with a sensitivity of 94.4 %, a specificity of 56.7%, and an AUC of 0.800 at a cutoff level of 439.1 ng/mg creatinine. [TIMP-2]×[IGFBP7], at a cutoff level of 0.803 (ng/mL)2/1,000, had a sensitivity of 94.4 %, a specificity of 83.3 %, and an AUC of 0.939 for diagnosing AR (Fig. 2). The calculated respective predictive values for IGFBP7, TIMP-2, and [TIMP-2]×[IGFBP7] showed the highest positive predictive value for IGFBP7, and the highest negative predictive value for the [TIMP-2]×[IGFBP7] (Table 2).
No significant relationship was found between the examined markers and the histological grade of AR or outcome after acute allograft dysfunction of any etiology. Out of 18 KTRs with AR, 10 (55.6%) recovered, 7 (38.9%) were maintained on regular dialysis, and 1 (5.5%) died. Meanwhile, out of the 15 KTRs with nonrejection AKI, 11 (73%) recovered and 4 (27%) were maintained on regular dialysis.
AR is defined as an increase in sCr levels after ruling out other causes of graft dysfunction. It is also marked by a rapid decrease in glomerular filtration rate and renal function, with distinct diagnostic features observable on kidney allograft biopsy. AR, which may be either antibody-mediated or T cell-mediated, can occur at any time posttransplant [12]. Therefore, early detection of AR is crucial for managing the graft and enhancing long-term graft survival. Kidney allograft biopsy, along with the Banff histopathologic criteria, remains the gold standard for diagnosing AR in KTRs. However, this procedure is invasive, costly, and carries a risk of serious complications. Additionally, histologic assessments often suffer from significant interobserver variability in interpretation, which can impact the timeliness of diagnosis [13].
In recent decades, various studies have called into question the reliability of different urinary biomarkers, either individually or in combination, for the early diagnosis and prediction of AR and for distinguishing it from other causes of acute allograft dysfunction. In cases of AKI, renal cell cycle arrest typically occurs 1–2 days before a significant decrease in glomerular filtration rate is observed. Therefore, TIMP-2 and IGFBP7 are considered to be earlier biomarkers for AKI than sCr. TIMP2 and IGFBP7, which are G1 cell cycle arrest (indicative of cellular stress) biomarkers, are released by tubular cells during the initial stages of renal tubular injury [14]. The urinary [TIMP-2]×[IGFBP7] NephroCheck test, which has been FDA-approved as an AKI biomarker, is commercially available and can be completed in only 20 minutes. The test provides a numeric result (AKI risk), making it rapid and straightforward for clinical use, particularly in KTRs, who are considered a high-risk group. A rapid decrease in the AKI risk score has been associated with the recovery of kidney function, while a persistently high AKI risk score has been linked to the development of ESRD [15].
During early cellular stress, there is upregulation of p53 and p21 expression. These proteins inhibit the activity of cyclin-dependent kinase complexes (CyclD-CDK4 and CyclE-CDK2), which are essential for cell cycle progression, leading to a transient G1 cell cycle arrest. This pause allows cells the chance to repair DNA damage and restore functionality [16].
Urinary [TIMP-2]×[IGFBP7] has been examined in the Sapphire, Opal, and Topaz studies for its ability to detect and validate predictions and diagnoses of AKI in native kidneys. It has been shown to provide early detection and risk stratification for imminent AKI in over 1,800 heterogeneous critically ill patients at risk of developing moderate to severe AKI. Multiple studies have been conducted to confirm their validity, with many reporting positive results and good performance in predicting AKI, as seen in Di Leo et al. [17]. However, another study by Bell et al. [18] reported a negative result, indicating that it did not predict AKI in ICU patients. El Minshawy et al. [19] found that urinary [TIMP-2]×[IGFBP7] served as a sensitive and specific biomarker for predicting pregnancy-related AKI at a cutoff value of 0.33 (ng/mL)2/1,000. The estimated sensitivity and specificity were 100% with both negative and positive predictive values of 100% [19].
In KT, Pianta et al. [20] evaluated the utility of [TIMP-2]× [IGFBP7] along with five inflammatory markers for predicting DGF in 56 recipients of deceased-donor kidneys. They found that only TIMP-2 and vascular endothelial growth factor-A, not [TIMP-2]×[IGFBP7], significantly improved the prediction of DGF at 4 and 12 hours [20]. In contrast, Yang et al. [21] reported that the [TIMP-2]×[IGFBP7] test, when administered immediately after transplantation, could serve as an early predictive biomarker of DGF in KT, with an AUC of 0.867 (sensitivity, 86%; specificity, 71%) at a cutoff value of 1.39. Urinary [TIMP-2]×[IGFBP7] was also investigated by Lameire et al. [22], who compared its levels among KTRs, healthy subjects, and adults with AKI. They concluded that the diagnostic performance of [TIMP-2]×[IGFBP7] was effective in assessing the risk for AKI, predicting the need for renal replacement therapy, and forecasting AKI-related complications, as well as short- and long-term prognoses [22]. In our study, we aimed to compare urinary levels of IGFBP7 and TIMP-2 separately, as well as their product [TIMP-2]×[IGFBP7], in cases of acute allograft dysfunction due to either AR or nonrejection causes, as well as in stable grafts. Our results indicated that levels were significantly higher in AR than in nonrejection causes of AKI. This could be speculated to be due to the role of cytotoxic T lymphocytes in apoptosis, leading to the overexpression of p53 and explaining the severity of renal dysfunction and the marked decline in the eGFR in the AR group. The tumor suppressor protein p53 is known for its role in cell cycle arrest, and the expression of p53 in tubular nuclei during acute renal allograft rejection suggests the presence of damaged DNA. This finding may shed light on some of the mechanisms that govern apoptotic tubular cell death during acute renal allograft rejection [23].
According to our results, urinary [TIMP-2]×[IGFBP7] had the best performance for diagnosing acute graft dysfunction, as compared to each marker on its own (AUC, 0.99). The cutoff value identified (0.278 [ng/mL]2/1,000) was comparable to the current FDA-approved value (0.3 [ng/mL]2/1,000), which was derived from the Opal study [24]. Furthermore, we found that the diagnostic performance of [TIMP-2]×[IGFBP7] may be superior in detecting acute allograft dysfunction in KTRs, with an AUC of 0.99, 100% sensitivity, and 93.3% specificity, compared to its performance in AR, where it showed an AUC of 0.93, 94.4% sensitivity, and 83.3% specificity. The Opal study reported a sensitivity of only 89% and a specificity of 50% for [TIMP-2]×[IGFBP7] in the detection of AKI in critically ill patients, none of whom were KTRs [24]. This might suggest that [TIMP-2]×[IGFBP7] has a superior diagnostic value for acute allograft dysfunction in KTRs compared to its value in critically ill patients. However, these results should be interpreted with caution due to the small sample size of our study.
While IGFBP7 demonstrated higher sensitivity and lower specificity than TIMP-2 in detecting acute allograft dysfunction, TIMP-2 exhibited higher sensitivity but lower specificity than IGFBP7 in the detection of AR. [TIMP-2]×[IGFBP7] provided exhibited high diagnostic performance for AR with a cutoff value different from that used for acute allograft dysfunction. At a cutoff level of 0.803 (ng/mL)2/1,000 (AUC, 0.939), [TIMP-2]×[IGFBP7] achieved a sensitivity of 94.4% and a specificity of 83.3% for diagnosing AR. This suggests that at this cutoff point, prompt antirejection management should be considered while awaiting the results of a graft biopsy.
Compared to other urinary biomarkers evaluated for diagnosing AR, Tinel et al. [25] demonstrated that combined urinary CXCL9 and CXCL10 could achieve high accuracy in AR diagnosis (AUC, 0.85; 95% confidence interval [CI], 0.80–0.89). However, levels may also be elevated due to urinary tract infections and BK virus reactivation [25]. Muthukumar et al. [26] found that urinary FOXP3 mRNA levels were higher in patients with biopsy-proven AR grafts than in those with other causes of graft dysfunction and in those with stable allograft function and normal allograft biopsies. Additionally, there was a significant inverse relationship between FOXP3 mRNA levels and sCr measured during an episode of AR, as well as between urinary FOXP3 levels and the time from KT to the development of AR [26]. Li et al. [27] reported that urinary cell levels of perforin and granzyme B mRNA were highly accurate in predicting AR (perforin mRNA sensitivity 83%, specificity 83%; granzyme B mRNA sensitivity 79%, specificity 77%) compared to stable controls. However, their gene expression also appeared to be upregulated in clinical settings other than AR, including urinary tract infection, cytomegalovirus infection, chronic allograft nephropathy, and delayed graft function. Urinary neutrophil gelatinase-associated lipocalin measurement, at least individually, cannot help distinguish the etiology of acute graft dysfunction [28]. The availability of a rapid test like NephroCheck, which measures both urinary IGFBP7 and TIMP-2, can assist in the early diagnosis and management of AR until graft biopsy results are available. Combining urinary biomarkers with clinical characteristics enables us to achieve the highest clinical relevance and provide targeted therapy for our patients at the appropriate time.
To the best of our knowledge, no prior studies have attempted to use urinary [TIMP-2]×[IGFBP7] in KTRs to assess AR and to differentiate between AR and nonrejection causes of acute graft dysfunction. [TIMP-2]×[IGFBP7] levels were found to be significantly higher in KTRs with acute graft dysfunction compared to those with stable graft function. Furthermore, levels were significantly higher in KTRs with AR than in those with nonrejection causes of acute allograft dysfunction. A significant positive correlation was observed between the values of IGFBP7 and TIMP-2 markers and sCr, but not with the histological grade of AR in renal biopsy or with the outcome. Whether the elevated levels of [TIMP-2]×[IGFBP7], increased mean sCr, and severe decline in the eGFR in the AR group are due to cytotoxic T lymphocyte-induced apoptosis and overexpression of the tumor suppressor protein p53, or to the immunological processes associated with rejection, remains to be investigated.
Some limitations of this study include the small sample size. Furthermore, evaluating baseline levels of [TIMP-2]×[IGFBP7] prior to any form of renal injury could enhance the value and sensitivity when serially comparing its postinjury levels across the various groups. Another limitation is that all patients with AR had only TCMR, as they all underwent living donor KT with a duration ranging between 1 and 6 months. It is also important to note that the biomarkers under investigation were studied in patients with AR who had a mild decline in renal function, as well as in patients with subclinical AR who maintained normal sCr levels. This was done to assess the potential of these biomarkers for clinical use in KT.
However, further data collection is recommended before implementing these biomarkers into clinical practice. While these new biomarkers hold significant therapeutic potential and could theoretically enhance both short- and long-term graft outcomes by early identification of allograft dysfunction, particularly AR, thus enabling tailored therapy, their precise role and clinical impact remain speculative. Therefore, they should be interpreted with caution and only in conjunction with clinical findings, while also considering the cost and availability of the tests. Urinary [TIMP-2]×[IGFBP7] may be useful in distinguishing AR from other causes of AKI in KTRs.
REFERENCES
1. Meier-Kriesche HU, Ojo AO, Hanson JA, Cibrik DM, Punch JD, Leichtman AB, et al. 2000; Increased impact of acute rejection on chronic allograft failure in recent era. Transplantation. 70:1098–100. DOI: 10.1097/00007890-200010150-00018. PMID: 11045649.
2. Opelz G. the Collaborative Transplant Study. 1997; Critical evaluation of the association of acute with chronic graft rejection in kidney and heart transplant recipients. Transplant Proc. 29:73–6. DOI: 10.1016/S0041-1345(96)00013-9. PMID: 9123162.
3. Tapia-Canelas C, Zometa R, López-Oliva MO, Jiménez C, Rivas B, Escuin F, et al. 2014; Complications associated with renal graft biopsy in transplant patients. Nefrologia. 34:115–9.
4. Oh DJ. 2020; A long journey for acute kidney injury biomarkers. Ren Fail. 42:154–65. DOI: 10.1080/0886022X.2020.1721300. PMID: 32050834. PMCID: PMC7034110.
5. Endre ZH, Pickering JW, Walker RJ, Devarajan P, Edelstein CL, Bonventre JV, et al. 2011; Improved performance of urinary biomarkers of acute kidney injury in the critically ill by stratification for injury duration and baseline renal function. Kidney Int. 79:1119–30. DOI: 10.1038/ki.2010.555. PMID: 21307838. PMCID: PMC3884688.
6. Yang L, Besschetnova TY, Brooks CR, Shah JV, Bonventre JV. 2010; Epithelial cell cycle arrest in G2/M mediates kidney fibrosis after injury. Nat Med. 16:535–43. DOI: 10.1038/nm.2144. PMID: 20436483. PMCID: PMC3928013.
7. Emlet DR, Pastor-Soler N, Marciszyn A, Wen X, Gomez H, Humphries WH 4th, et al. 2017; Insulin-like growth factor binding protein 7 and tissue inhibitor of metalloproteinases-2: differential expression and secretion in human kidney tubule cells. Am J Physiol Renal Physiol. 312:F284–96. DOI: 10.1152/ajprenal.00271.2016. PMID: 28003188. PMCID: PMC5336590.
8. Ortega LM, Heung M. 2018; The use of cell cycle arrest biomarkers in the early detection of acute kidney injury. Is this the new renal troponin? Nefrologia (Engl Ed). 38:361–7. DOI: 10.1016/j.nefro.2017.11.013. PMID: 29627229.
9. Fan W, Ankawi G, Zhang J, Digvijay K, Giavarina D, Yin Y, et al. 2019; Current understanding and future directions in the application of TIMP-2 and IGFBP7 in AKI clinical practice. Clin Chem Lab Med. 57:567–76. DOI: 10.1515/cclm-2018-0776. PMID: 30179848.
10. Edwards JK. 2015; How precise is NephroCheck®? Nat Rev Nephrol. 11:127. DOI: 10.1038/nrneph.2015.7. PMID: 25643663.
11. Loupy A, Haas M, Roufosse C, Naesens M, Adam B, Afrouzian M, et al. 2020; The Banff 2019 Kidney Meeting Report (I): updates on and clarification of criteria for T cell- and antibody-mediated rejection. Am J Transplant. 20:2318–31. DOI: 10.1111/ajt.15898. PMID: 32463180. PMCID: PMC7496245.
12. Kidney Disease: Improving Global Outcomes (KDIGO) Transplant Work Group. 2009; KDIGO clinical practice guideline for the care of kidney transplant recipients. Am J Transplant. 9 Suppl 3:S1–155. DOI: 10.1111/j.1600-6143.2009.02834.x. PMID: 19845597.
13. Glassock RJ. 2015; Con: kidney biopsy: an irreplaceable tool for patient management in nephrology. Nephrol Dial Transplant. 30:528–31. DOI: 10.1093/ndt/gfv044. PMID: 25801636.
14. Price PM, Safirstein RL, Megyesi J. 2009; The cell cycle and acute kidney injury. Kidney Int. 76:604–13. DOI: 10.1038/ki.2009.224. PMID: 19536080. PMCID: PMC2782725.
15. Pajenda S, Ilhan-Mutlu A, Preusser M, Roka S, Druml W, Wagner L. 2015; NephroCheck data compared to serum creatinine in various clinical settings. BMC Nephrol. 16:206. DOI: 10.1186/s12882-015-0203-5. PMID: 26651477. PMCID: PMC4674950.
16. Wang W, Saad A, Herrmann SM, Eirin Massat A, McKusick MA, Misra S, et al. 2016; Changes in inflammatory biomarkers after renal revascularization in atherosclerotic renal artery stenosis. Nephrol Dial Transplant. 31:1437–43. DOI: 10.1093/ndt/gfv448. PMID: 26908767. PMCID: PMC5009289.
17. Di Leo L, Nalesso F, Garzotto F, Xie Y, Yang B, Virzì GM, et al. 2018; Predicting acute kidney injury in intensive care unit patients: the role of tissue inhibitor of metalloproteinases-2 and insulin-like growth factor-binding protein-7 biomarkers. Blood Purif. 45:270–7. DOI: 10.1159/000485591. PMID: 29478052.
18. Bell M, Larsson A, Venge P, Bellomo R, Mårtensson J. 2015; Assessment of cell-cycle arrest biomarkers to predict early and delayed acute kidney injury. Dis Markers. 2015:158658. DOI: 10.1155/2015/158658. PMID: 25866432. PMCID: PMC4381987.
19. El Minshawy O, Khedr MH, Youssuf AM, Abo Elela M, Kamel FM, Keryakos HK. 2021; Value of the cell cycle arrest biomarkers in the diagnosis of pregnancy-related acute kidney injury. Biosci Rep. 41:BSR20200962. DOI: 10.1042/BSR20200962. PMID: 33295613. PMCID: PMC7786331.
20. Pianta TJ, Peake PW, Pickering JW, Kelleher M, Buckley NA, Endre ZH. 2015; Evaluation of biomarkers of cell cycle arrest and inflammation in prediction of dialysis or recovery after kidney transplantation. Transpl Int. 28:1392–404. DOI: 10.1111/tri.12636. PMID: 26174580.
21. Yang J, Lim SY, Kim MG, Jung CW, Cho WY, Jo SK. 2017; Urinary tissue inhibitor of metalloproteinase and insulin-like growth factor-7 as early biomarkers of delayed graft function after kidney transplantation. Transplant Proc. 49:2050–4. DOI: 10.1016/j.transproceed.2017.09.023. PMID: 29149959.
22. Lameire N, Vanmassenhove J, Van Biesen W, Vanholder R. 2016; The cell cycle biomarkers: promising research, but do not oversell them. Clin Kidney J. 9:353–8. DOI: 10.1093/ckj/sfw033. PMID: 27274818. PMCID: PMC4886923.
23. Wever PC, Aten J, Rentenaar RJ, Hack CE, Koopman G, Weening JJ, et al. 1998; Apoptotic tubular cell death during acute renal allograft rejection. Clin Nephrol. 49:28–34.
24. Hoste EA, McCullough PA, Kashani K, Chawla LS, Joannidis M, Shaw AD, et al. 2014; Derivation and validation of cutoffs for clinical use of cell cycle arrest biomarkers. Nephrol Dial Transplant. 29:2054–61. DOI: 10.1093/ndt/gfu292. PMID: 25237065. PMCID: PMC4209880.
25. Tinel C, Devresse A, Vermorel A, Sauvaget V, Marx D, Avettand-Fenoel V, et al. 2020; Development and validation of an optimized integrative model using urinary chemokines for noninvasive diagnosis of acute allograft rejection. Am J Transplant. 20:3462–76. DOI: 10.1111/ajt.15959. PMID: 32342614.
26. Muthukumar T, Dadhania D, Ding R, Snopkowski C, Naqvi R, Lee JB, et al. 2005; Messenger RNA for FOXP3 in the urine of renal-allograft recipients. N Engl J Med. 353:2342–51. DOI: 10.1056/NEJMoa051907. PMID: 16319383.
27. Li B, Hartono C, Ding R, Sharma VK, Ramaswamy R, Qian B, et al. 2001; Noninvasive diagnosis of renal-allograft rejection by measurement of messenger RNA for perforin and granzyme B in urine. N Engl J Med. 344:947–54. DOI: 10.1056/NEJM200103293441301. PMID: 11274620.
28. Ramirez-Sandoval JC, Herrington W, Morales-Buenrostro LE. 2015; Neutrophil gelatinase-associated lipocalin in kidney transplantation: a review. Transplant Rev (Orlando). 29:139–44. DOI: 10.1016/j.trre.2015.04.004. PMID: 26071983.
Fig. 1
Urinary insulin-like growth factor-binding protein 7 (IGFBP7) and tissue inhibitor of metalloproteinase-2 (TIMP-2) in acute graft dysfunction and acute graft rejection in kidney transplant recipients (KTRs). (A) Urinary IGFBP7 level was higher in KTRs with acute allograft dysfunction than those with stable grafts, with mean±standard error (SE) 1.65±0.16 pg/mg creatinine, and 0.44±0.05 pg/mg creatinine, respectively (P<0.001). (B) Urinary TIMP-2 level was higher in KTRs with acute allograft dysfunction than those with stable grafts, with mean±SE 681.60±32.18 ng/mg creatinine, and 407.65±20.61 ng/mg creatinine, respectively (P<0.001). (C) [TIMP-2]×[IGFBP7] was higher in KTRs with acute allograft dysfunction than those with stable grafts, with mean±SE 1.08±0.10 (ng/mL)2/1,000, and 0.18±0.03 (ng/mL)2/1,000, respectively (P<0.001). (D) Urinary IGFBP7 level was significantly higher in KTRs with acute rejection (AR) than those with nonrejection acute kidney injury (AKI), with mean±SE 2.06±0.23 pg/mg creatinine, and 1.16±0.12 pg/mg creatinine, respectively (P<0.001). (E) Urinary TIMP-2 level was higher in KTRs with AR than those with nonrejection AKI, with mean±SE 722.33±39.53 ng/mg creatinine, and 632.81±51.18 ng/mg creatinine, respectively (P=0.169). (F) [TIMP-2]×[IGFBP7] was significantly higher in KTRs with AR than those with nonrejection AKI, with mean±SE 1.38±0.14 (ng/mL)2/1,000, and 0.70±0.07 (ng/mL)2/1,000, respectively (P<0.001).
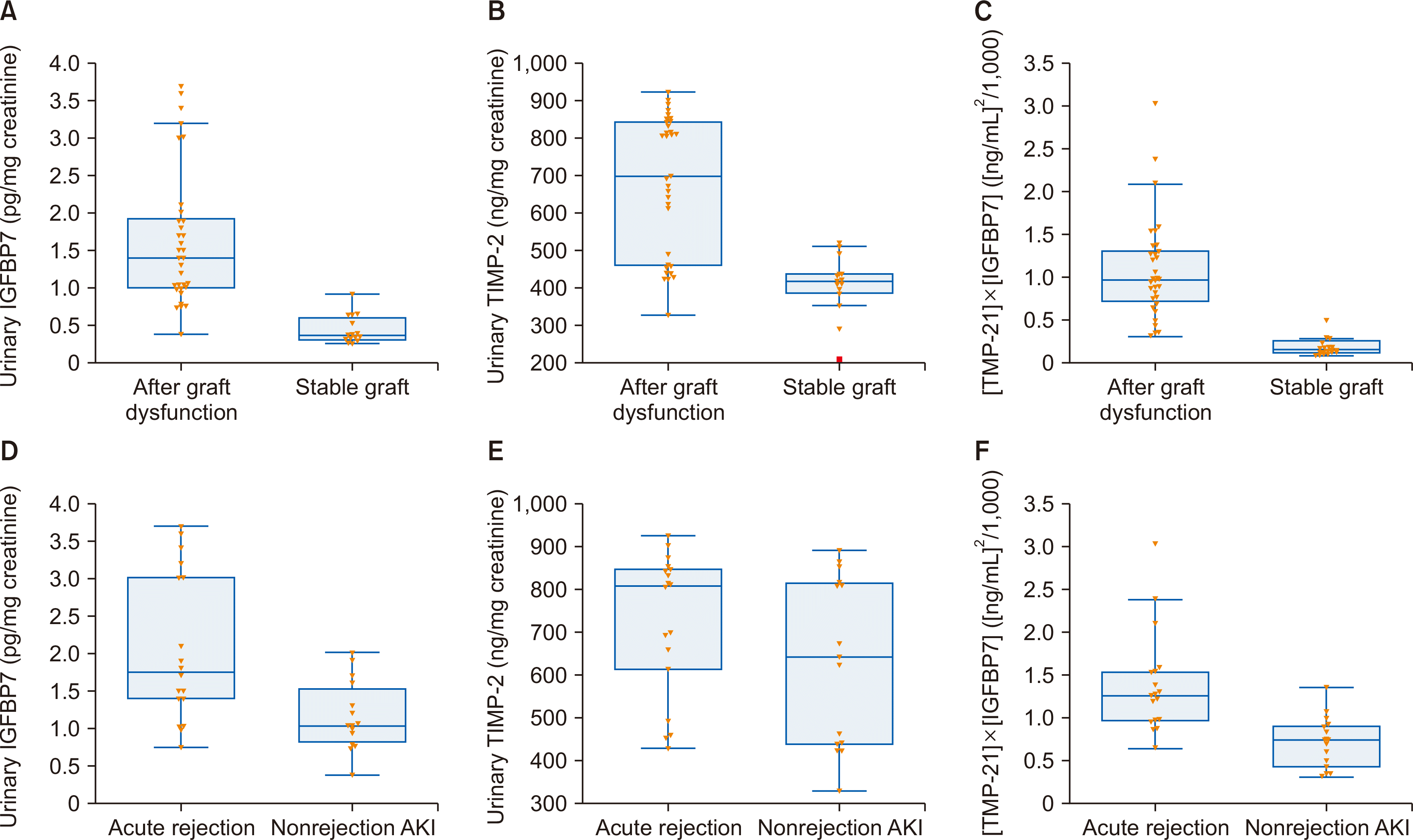
Fig. 2
Receiver operating characteristic (ROC) curve analysis of insulin-like growth factor-binding protein 7 (IGFBP7), tissue inhibitor of metalloprotease-2 (TIMP-2), and [TIMP-2]×[IGFBP7] as markers of acute graft dysfunction. (A) IGFBP7 was able to diagnose acute allograft dysfunction with a sensitivity of 97.0%, specificity of 93.3%, and area under the curve (AUC) of 0.97 at a cutoff level of 0.65 pg/mg creatinine. (B) TIMP-2 was able to diagnose acute allograft dysfunction with a sensitivity of 69.7%, specificity of 100%, and AUC 0.91 at cutoff level 519.3 ng/mg creatinine. (C) The [TIMP-2]×[IGFBP7] at its cutoff level 0.278 (ng/mL)2/1,000 had a sensitivity of 100%, specificity of 93.3%, and AUC 0.99 in diagnosing acute graft dysfunction. (D) ROC curves analysis of IGFBP7, TIMP-2, and the [TIMP-2]×[IGFBP7] as markers of acute rejection. IGFBP7 was able to diagnose acute rejection with a sensitivity of 77.8%, specificity of 86.7%, and AUC 0.881 at a cutoff level of 1.3 pg/mg creatinine. (E) TIMP-2 was able to diagnose acute rejection with a sensitivity of 94.4%, specificity of 56.7%, and AUC 0.800 at a cutoff level of 439.1 ng/mg creatinine. (F) The [TIMP-2]×[IGFBP7] at its cutoff level 0.803 (ng/mL)2/1,000 had a sensitivity of 94.4%, specificity of 83.3%, and AUC 0.939 in diagnosing acute rejection. Red stars correspond to optimum criterion cut-off points.
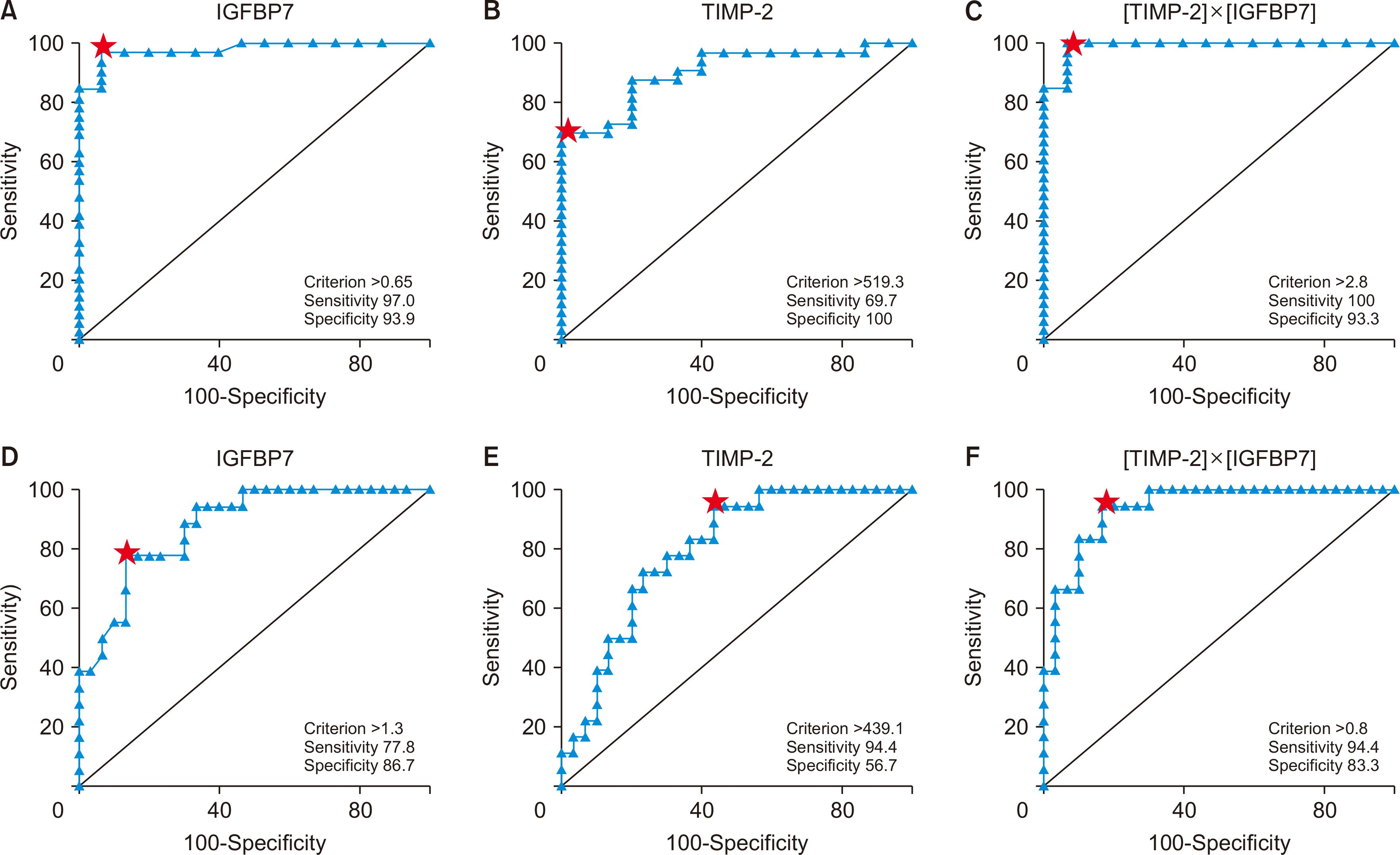
Table 1
Descriptive statistics of three groups of renal allograft recipients
Table 2
Predictive values of the examined markers for the diagnosis of acute graft dysfunction and acute rejection
Table 3
Results of multiple regression analyses
Model | Unstandardized coefficients | Standardized coefficients | t-value | Significance | 95% CI for B | |||
---|---|---|---|---|---|---|---|---|
|
|
|
||||||
B | Standard error | Beta | Lower bound | Upper bound | ||||
Coefficients (group with AR)a) | ||||||||
(Constant) | 0.561 | 1.598 | - | 0.351 | 0.733 | −3.000 | 4.123 | |
Creatinine | −0.182 | 0.128 | −0.932 | −1.420 | 0.186 | −0.468 | 0.104 | |
eGFR | 0.019 | 0.032 | 0.361 | 0.594 | 0.566 | −0.053 | 0.091 | |
Urea | 0.017 | 0.007 | 1.700 | 2.271 | 0.056 | 0.002 | 0.035 | |
Age | −0.041 | 0.026 | −0.575 | −1.582 | 0.145 | −0.098 | 0.017 | |
Coefficients (group with nonrejection AKI)a) | ||||||||
(Constant) | 1.252 | 1.191 | - | 1.052 | 0.328 | −1.564 | 4.069 | |
Creatinine | −0.132 | 0.129 | −0.652 | −1.019 | 0.342 | −0.437 | 0.174 | |
eGFR | −0.008 | 0.009 | −0.558 | −0.887 | 0.404 | −0.030 | 0.013 | |
Urea | 0.003 | 0.003 | 0.579 | 0.945 | 0.376 | −0.005 | 0.011 | |
Age | 0.006 | 0.010 | 0.211 | 0.575 | 0.584 | −0.017 | 0.028 | |
Coefficients (group with stable grafts)a) | ||||||||
(Constant) | −0.359 | 1.255 | - | −0.286 | 0.783 | −3.327 | 2.609 | |
Creatinine | −0.002 | 0.399 | −0.005 | −0.005 | 0.996 | −0.947 | 0.943 | |
eGFR | 0.001 | 0.004 | 0.251 | 0.255 | 0.806 | −0.009 | 0.011 | |
Urea | 0.001 | 0.004 | 0.147 | 0.343 | 0.742 | −0.008 | 0.010 | |
Age | 0.002 | 0.021 | 0.035 | 0.082 | 0.937 | −0.047 | 0.051 |