Abstract
Background
The quick and easy nature of point-of-care (POC) testing devices allows regular monitoring of serum lipid levels to increase efficiency. The purpose of this study was to assess a POC lipid analyzer, Barozen Lipid Plus (MICO Biomed Co., Ltd.), which uses capillary blood to measure total cholesterol (TC), triglycerides (TGs), and high-density lipoprotein cholesterol (HDL-C).
Methods
Capillary and venous blood samples were collected from 110 participants at a single center in Korea between June 10 and June 26, 2021. TC, TG, and HDL-C measurements using Barozen Lipid Plus were compared with measurements using our reference device, the Roche-Hitachi Cobas 8000 c702 (Hitachi High-Technologies Corporation). This study followed the guidelines of the Clinical and Laboratory Standards Institute and the Clinical Laboratory Improvement Amendments. We surveyed participants regarding the convenience of the POC device using a questionnaire following the completion of blood collection.
Results
When compared to the reference equipment, the measurements obtained using Barozen Lipid Plus were more than 95% satisfactory within TC±10%, TG±25%, and HDL-C±30%. The coefficient of variation in the repeatability testing was within 5% for TC, 5% for TGs, and 7% for HDL-C. The survey results indicated high levels of satisfaction. No adverse events were reported.
Dyslipidemia is an imbalance of blood lipid levels characterized by elevated total cholesterol (TC), elevated triglycerides (TGs), elevated low-density lipoprotein cholesterol (LDL-C), and decreased high-density lipoprotein cholesterol (HDL-C). Hypercholesterolemia is a well-established causal factor of atherosclerotic cardiovascular diseases [1]. In 2008, the World Health Organization reported a global hypercholesterolemia prevalence of 39% [2]. Hypertriglyceridemia is observed in up to 35% of acute pancreatitis cases [3]. Furthermore, HDL-C levels are useful in predicting the risk of coronary heart disease [4,5]. In Korea, the prevalence of dyslipidemia increased from 8% in 2005 to 22.3% in 2019 [6].
It is widely advised that adults aged 20 years and older should be tested for traditional atherosclerotic cardiovascular disease (ASCVD) risk factors, including plasma lipid profiles, at least every 4 to 6 years [7]. In patients with elevated LDL-C levels, LDL-C is measured every 4 to 12 weeks until the target level is reached, repeating the test every 3 to 12 months thereafter to assess adherence [8]. Lipid levels are easily affected by lifestyle changes and drug adherence and therefore require frequent and regular monitoring. Point-of-care (POC) testing devices are widely used in primary care settings because of their convenience. POC testing reduces the discomfort associated with venous puncture, requires smaller volumes of blood, and produces results on the spot.
We conducted a study to test the validity of a new POC lipid analysis device named Barozen Lipid Plus (MICO Biomed Co., Ltd. Seongnam, Korea). In accordance with the guidelines of the Clinical and Laboratory Standards Institute (CLSI) and the Clinical Laboratory Improvement Amendments (CLIA), we evaluated the system accuracy, measurement repeatability, intermediate measurement precision, interference, and patient satisfaction level.
Ethical statements: This study was conducted in accordance with the Declaration of Helsinki. The study was approved by the Institutional Review Board (IRB) of Soonchunhyang University Seoul Hospital (IRB No: 2021-03-027). Ethical approval and written informed consent were obtained prior to enrollment.
This study was conducted at a single center in Korea from June 10 to June 26, 2021. Healthy individuals and patients with dyslipidemia aged >19 years were included in this study. Participants were excluded if they had incapacitating psychological conditions, had a change in medication within 1 month, or had participated in other clinical trials within 1 month. This study followed the CLSI guidelines and 100 participants were required [9]. We recruited 110 participants who met our inclusion criteria, accounting for 10% of possible dropouts during the trial.
Capillary and venous blood samples were collected on the same day from each subject. The fingertip skin was punctured using a lancet, and we obtained up to 300 μL of capillary blood from each participant using a 15-μL capillary tube for multiple testing. Barozen Lipid Plus MMD-1/MLS-2 was used to test the capillary blood. Three test-strip lots manufactured on different dates were used. A single venous blood sample of approximately 4 mL was obtained after capillary blood collection using the conventional method of venipuncture and then tested using the Roche-Hitachi Cobas 8000 c702 standard analyzer (Hitachi High-Technologies Corporation, Tokyo, Japan). In this study, the Roche-Hitachi Cobas 8000 c702 was used as the reference equipment to measure lipid levels twice, conforming to the ISO 17511:20200 metrological traceability requirements [10]. The Roche-Hitachi Cobas 8000 c702 is a widely used chemistry analyzer with proven reliability [11]. The TC, TG, and HDL-C levels were measured. The ambient temperature was maintained at 23°C±5°C throughout this clinical trial. The ambient relative humidity was maintained at <80% and the ambient light was maintained at <2,000 lux.
Demographic and clinical data were collected by interviewing participants prior to blood collection. The height, weight, and blood pressure of the participants were measured before sampling, along with a general physical examination of all systems. The participants were instructed to report any abnormal reactions during the investigation. After completing the measurement procedures, the participants were asked to complete a feedback survey.
Precision was evaluated according to CLSI EP05-A3 [12]. Capillary samples from six concentration ranges were used for repeatability testing of TC, TGs, and HDL-C (Supplementary Table 1). Each sample was allocated to three test-strip lots, and each lot was tested using 10 different Barozen Lipid Plus devices.
TC, TG, and HDL-C levels were individually divided into three concentration ranges. Capillary samples representing each concentration range were tested with three test-strip lots with 10 different Barozen Lipid Plus devices twice per day (Supplementary Table 2). This measurement was repeated for 10 consecutive days, with each sample resulting in 600 measurements.
The measurement procedure and bias estimation were compared between instruments according to CLSI EP09-A3 [13]. Each participant’s capillary blood sample was tested on three test strips from three different lots using two different Barozen Lipid Plus meters, resulting in six measurements per participant. These values were compared with those obtained from the participant’s venous blood tested on the Roche-Hitachi Cobas 8000 c702. TC, TG, and HDL-C concentrations in blood samples were distributed among four, five, and four groups, respectively, according to clinical relevance (Supplementary Table 3).
Based on CLSI EP07, two samples of TC, TGs, and HDL-C were acquired separately and mixed with 11 potentially interfering substances [14]. The 66 samples were tested with three test-strip lots using two Barozen Lipid Plus devices, and each measurement was repeated five times. The same samples were tested without the interfering substances, also using three test-strip lots and two devices and repeating each measurement five times. These values were compared with the measurements obtained using the reference equipment.
Barozen Lipid Plus is a compact POC device that measures blood TC, TG, and HDL-C levels. The measurement ranges for TC, TGs, and HDL-C were 50 to 450 mg/dL, 30 to 650 mg/dL, and 10 to 100 mg/dL, respectively. The results are reported in the following order: TC, TGs, HDL-C, LDL-C, TC/HDL-C, and LDL-C/HDL-C, first in mg/dL and then in mmol/L. Similar to portable glucometers, this device uses capillary blood injected into a dedicated test strip. The measurement takes up to 3 minutes. The device is powered by three AAA batteries and weighs up to 91 g.
Data analysis was performed according to the requirements of CLIA. Passing-Bablok regression analysis was performed to examine accuracy. Other data were evaluated using the mean, standard deviation, and coefficient of variation (CV). All statistical analyses were performed using Microsoft Excel 2016 (Microsoft Corporation, Redmond, WA, USA) and SAS version 9.4 (SAS Institute Inc., Seattle, WA, USA) at a significance level of 0.05.
The age and sex distributions of the participants included in this study are presented in Table 1. This study included 110 participants, 37.3% of whom were in their twenties. Fifty-three participants (48.2%) were male.
For all six concentration levels of TC, TGs, and HDL-C, the CV was within 5%, 5%, and 7%, respectively (Table 2).
For all three concentration levels of TC, TGs, and HDL-C, the CV was within 5%, 5%, and 7%, respectively (Table 3).
Passing-Bablok regression analysis revealed an excellent correlation for the values obtained between the Barozen Lipid Plus and Roche-Hitachi Cobas 8000 c702 systems. The correlation coefficients for TC, TG, and HDL-C levels were 0.977, 0.993, and 0.984, respectively. The regression coefficients for TC, TG, and HDL-C levels were 0.958 (95% confidence interval [CI], 0.942–0.974), 0.980 (95% CI, 0.971–0.989), and 0.936 (95% CI, 0.923–0.949), respectively. In accordance with the CLIA guidelines, we confirmed that the tolerance was greater than 95% for TC±10%, TG±25%, and HDL-C±30% (Table 4, Fig. 1).
Eleven potential interfering substances (acetaminophen, ascorbic acid, citric acid, ibuprofen, urea, unconjugated bilirubin, uric acid, heparin [Li], heparin [Na], K2-ethylenediaminetetraacetic acid, and caffeine) were evaluated in accordance with the CLSI and CLIA guidelines. No substance produced a measured value of more than ±10% of the control sample value without the substance, thus meeting the acceptance criteria (Table 5).
The questionnaire analysis to evaluate the convenience of the POC cholesterol measuring device showed relatively high satisfaction. A total of 81.8% of the participants found the measuring system easy to use by themselves (Supplementary Table 4). No adverse reactions were observed or reported during this clinical trial (Supplementary Table 5).
Lipid profiles have been widely evaluated for disease prevention and management. LDL-C, the most abundant apolipoprotein B-100 (ApoB)-containing lipoprotein, is the established cause of ASCVD, and efforts to lower LDL-C levels are being made to reduce the risk of cardiovascular events [15]. TGs, which are found in ApoB-containing lipoproteins, also increase the risk of ASCVD [16]. With its inverse relationship with ASCVD, HDL-C aids in the prediction of cardiovascular events; however, its preventive role has not yet been proven [17,18]. The LDL-C target goal is tailored to the level of cardiovascular risk determined by age, sex, race, region, TC level, HDL-C level, blood pressure, smoking status, and comorbidities: <116 mg/dL, <100 mg/dL, <70 mg/dL, and <55 mg/dL for low-, moderate-, high-, and very high-risk groups, respectively [19-21]. The treatment goal for lowering TGs has not yet been established, although TG levels of >150 mg/dL are known to increase cardiovascular risk [22]. Therefore, based on the 2019 European Society of Cardiology/European Atherosclerosis Society guidelines, drugs that lower TG levels can be considered for high-risk patients when their TG level is >200 mg/dL [19]. TG-lowering drugs are also used in hypertriglyceridemia-induced acute pancreatitis, as TG levels of >500 mg/dL are associated with an increased risk [23].
Current medical evidence does not indicate exactly which test should be performed at which intervals [24]. The expert consensus is to test the patient’s lipids twice at an interval of 1 to 12 weeks before beginning treatment and 4 to 12 weeks after starting lipid-lowering treatment or adjusting the treatment dose [8,19]. Dietary habits, body weight, physical activity, alcohol consumption, and smoking significantly affect lipid profiles. These lifestyle factors can change daily, consequently causing fluctuations in lipid measurements [25]. For this reason, regular and frequent lipid monitoring has been shown to enhance treatment adherence and improve disease management [26-28]. General practitioners and patients were also satisfied with POC cholesterol testing in terms of convenience, efficiency, and cost [29]. POC devices are minimally invasive, enable rapid diagnoses, and are available at clinical management sites for efficient patient care. They also decrease examination costs and diminish the space requirements for large equipment and storage, thus allowing use in smaller primary care settings. Barozen Lipid Plus integrates all the aforementioned advantages of POC testing for screening and monitoring plasma lipids. Full lipid profiles of TC, LDL-C, HDL-C, and TGs are recommended for lipid measurements, all of which are provided by Barozen Lipid Plus. Based on our study results, the measurements using the POC device were reliable, although the CVs for HDL-C were higher than those for TC and LDL-C. This may be due to the smaller interval ranges defined in the Supplementary Tables. The ratio of TC to HDL-C is also reported by this device, which, in an analysis of data from the Framingham study, showed an association with coronary heart disease, whereas LDL-C did not [4]. Therefore, our findings suggest that the POC lipid analyzer is an easy and effective way to test lipids that is especially well-suited for primary care settings. Further meta-analyses on the reliability of different POC lipid analyzers will be helpful for broader implementation in clinical settings.
This study had some limitations. First, the fasting duration was not specified. Although studies have shown that fasting is not routinely required, many clinicians still ask their patients to fast for 8 to 12 hours in accordance with the National Cholesterol Education Program guidelines [30,31]. This study did not require the participants to fast; this inconsistency may have affected the test results. Second, this device uses the Friedewald formula, LDL-C=TC−HDL-C−(TG/5), which limits its use in monitoring patients with TG levels of >400 mg/dL. This formula is known to become less accurate as TG levels increase, especially when LDL-C is <70 mg/dL [32,33]. Nonetheless, it is the most widely used formula to determine LDL-C levels, and most treatment guidelines for dyslipidemia have been developed based on studies performed using calculated LDL-C levels. In the primary care setting, the use of calculated LDL-C is assumed to be sufficiently accurate [34]. Third, the measurement of non-HDL-C and ApoB is recommended in patients with diabetes or hypertriglyceridemia and concomitantly low LDL-C levels, which the Barozen Lipid Plus does not measure [35-37].
In conclusion, when compared with the Roche/Hitachi Cobas 8000 c720 reference device, the performance of the Barozen Lipid Plus was acceptable in terms of system accuracy, precision, and potential substance interference according to the CLSI and CLIA guidelines.
Supplementary materials
Supplementary Tables 1 to 5 can be found via https://doi.org/10.12701/jyms.2023.00528.
Supplementary Table 1.
Intervals for measurement repeatability evaluation
Supplementary Table 2.
Intervals for intermediate measurement precision evaluation
Supplementary Table 3.
Concentration distribution of total cholesterol, triglyceride, and HDL cholesterol in samples for system accuracy evaluation
Supplementary Table 4.
Questionnaire following lipid analysis with Barozen Lipid PLus
References
2. World Health Organization (WHO). The global health observatory: raised cholesterol [Internet]. Geneva: WHO;2008. [cited 2023 May 24]. https://www.who.int/data/gho/indicator-metadata-registry/imr-details/3236.
3. Olesen SS, Harakow A, Krogh K, Drewes AM, Handberg A, Christensen PA. Hypertriglyceridemia is often under recognized as an aetiologic risk factor for acute pancreatitis: a population-based cohort study. Pancreatology. 2021; 21:334–41.


4. Ingelsson E, Schaefer EJ, Contois JH, McNamara JR, Sullivan L, Keyes MJ, et al. Clinical utility of different lipid measures for prediction of coronary heart disease in men and women. JAMA. 2007; 298:776–85.


5. Emerging Risk Factors Collaboration, Di Angelantonio E, Sarwar N, Perry P, Kaptoge S, Ray KK, et al. Major lipids, apolipoproteins, and risk of vascular disease. JAMA. 2009; 302:1993–2000.


6. Ministry of Health and Welfare, Korea Centers for Disease Control and Prevention. Korea Health Statistics 2019: Korea National Health and Nutrition Examination Survey (KNHANES VIII-1) [Internet]. Cheongju: Korea Centers for Disease Control and Prevention; 2020 [cited 2023 May 24]. https://knhanes.kdca.go.kr/knhanes/sub04/sub04_04_01.do.
7. Arnett DK, Blumenthal RS, Albert MA, Buroker AB, Goldberger ZD, Hahn EJ, et al. 2019 ACC/AHA guideline on the primary prevention of cardiovascular disease: a report of the American College of Cardiology/American Heart Association Task Force on clinical practice guidelines. Circulation. 2019; 140:e596–646.
8. Grundy SM, Stone NJ, Bailey AL, Beam C, Birtcher KK, Blumenthal RS, et al. 2018 AHA/ACC/AACVPR/AAPA/ABC/ACPM/ADA/AGS/APhA/ASPC/NLA/PCNA guideline on the management of blood cholesterol: a report of the American College of Cardiology/American Heart Association Task Force on clinical practice guidelines. J Am Coll Cardiol. 2019; 73:e285–350.
9. Clinical and Laboratory Standards Institute (CLSI). EP09: Measurement procedure comparison and bias estimation using patient samples. 3rd ed. Wayne, PA: CLSI;2018.
10. International Organization for Standardization (ISO). ISO 17511:2020 In vitro diagnostic medical devices: requirements for establishing metrological traceability of values assigned to calibrators, trueness control materials and human samples. 2nd ed. Geneva: ISO;2020.
11. Kim SY, Jeong TD, Lee W, Chun S, Min WK. Performance evaluation of the Roche-Hitachi Cobas 8000 c702 chemistry autoanalyzer. Lab Med Online. 2014; 4:132–9.


12. Clinical and Laboratory Standards Institute (CLSI). EP05-A3: Evaluation of precision of quantitative measurement procedures. 3rd ed. Wayne, PA: CLSI;2014.
13. Clinical and Laboratory Standards Institute (CLSI). EP09-A3: Measurement procedure comparison and bias estimation using patient samples; approved guideline. Wayne, PA: CLSI;2013.
14. Clinical and Laboratory Standards Institute (CLSI). EP07: Interference testing in clinical chemistry. Wayne, PA: CLSI;2018.
15. Ference BA, Ginsberg HN, Graham I, Ray KK, Packard CJ, Bruckert E, et al. Low-density lipoproteins cause atherosclerotic cardiovascular disease. 1. Evidence from genetic, epidemiologic, and clinical studies. A consensus statement from the European Atherosclerosis Society Consensus Panel. Eur Heart J. 2017; 38:2459–72.


16. Ference BA, Kastelein JJP, Ray KK, Ginsberg HN, Chapman MJ, Packard CJ, et al. Association of triglyceride-lowering LPL variants and LDL-C-lowering LDLR variants with risk of coronary heart disease. JAMA. 2019; 321:364–73.


17. Emerging Risk Factors Collaboration, Di Angelantonio E, Gao P, Pennells L, Kaptoge S, Caslake M, et al. Lipid-related markers and cardiovascular disease prediction. JAMA. 2012; 307:2499–506.


18. Andrews J, Janssan A, Nguyen T, Pisaniello AD, Scherer DJ, Kastelein JJ, et al. Effect of serial infusions of reconstituted high-density lipoprotein (CER-001) on coronary atherosclerosis: rationale and design of the CARAT study. Cardiovasc Diagn Ther. 2017; 7:45–51.


19. Mach F, Baigent C, Catapano AL, Koskinas KC, Casula M, Badimon L, et al. 2019 ESC/EAS Guidelines for the management of dyslipidaemias: lipid modification to reduce cardiovascular risk. Eur Heart J. 2020; 41:111–88.
20. Grundy SM, Stone NJ, Bailey AL, Beam C, Birtcher KK, Blumenthal RS, et al. 2018 AHA/ACC/AACVPR/AAPA/ABC/ACPM/ADA/AGS/APhA/ASPC/NLA/PCNA guideline on the management of blood cholesterol: a report of the American College of Cardiology/American Heart Association Task Force on clinical practice guidelines. Circulation. 2019; 139:e1082–143.
21. JBS3 Board. Joint British Societies’ consensus recommendations for the prevention of cardiovascular disease (JBS3). Heart. 2014; 100(Suppl 2):ii1–67.
22. Triglyceride Coronary Disease Genetics Consortium and Emerging Risk Factors Collaboration, Sarwar N, Sandhu MS, Ricketts SL, Butterworth AS, Di Angelantonio E, et al. Triglyceride-mediated pathways and coronary disease: collaborative analysis of 101 studies. Lancet. 2010; 375:1634–9.


23. Lindkvist B, Appelros S, Regnér S, Manjer J. A prospective cohort study on risk of acute pancreatitis related to serum triglycerides, cholesterol and fasting glucose. Pancreatology. 2012; 12:317–24.


24. Glasziou PP, Irwig L, Kirby AC, Tonkin AM, Simes RJ. Which lipid measurement should we monitor? An analysis of the LIPID study. BMJ Open. 2014; 4:e003512.


25. Bolodeoku J. Self-monitoring blood cholesterol (SMBC) testing: within-person day to day variation in a healthy volunteer. Med Res Archives. 2017; 5(9).
26. Price CP. Point of care testing. BMJ. 2001; 322:1285–8.
28. Gialamas A, Yelland LN, Ryan P, Willson K, Laurence CO, Bubner TK, et al. Does point-of-care testing lead to the same or better adherence to medication? A randomised controlled trial: the PoCT in General Practice Trial. Med J Aust. 2009; 191:487–91.


29. Cohen J, Piterman L, McCall LM, Segal L. Near-patient testing for serum cholesterol: attitudes of general practitioners and patients, appropriateness, and costs. Med J Aust. 1998; 168:605–9.


30. Nordestgaard BG, Langsted A, Mora S, Kolovou G, Baum H, Bruckert E, et al. Fasting is not routinely required for determination of a lipid profile: clinical and laboratory implications including flagging at desirable concentration cut-points-a joint consensus statement from the European Atherosclerosis Society and European Federation of Clinical Chemistry and Laboratory Medicine. Eur Heart J. 2016; 37:1944–58.


31. National Cholesterol Education Program (NCEP) Expert Panel on Detection, Evaluation, and Treatment of High Blood Cholesterol in Adults (Adult Treatment Panel III). Third Report of the National Cholesterol Education Program (NCEP) Expert Panel on Detection, Evaluation, and Treatment of High Blood Cholesterol in Adults (Adult Treatment Panel III) final report. Circulation. 2002; 106:3143–421.
32. Martin SS, Blaha MJ, Elshazly MB, Brinton EA, Toth PP, McEvoy JW, et al. Friedewald-estimated versus directly measured low-density lipoprotein cholesterol and treatment implications. J Am Coll Cardiol. 2013; 62:732–9.


33. Jialal I, Inn M, Siegel D, Devaraj S. Underestimation of low density lipoprotein-cholesterol with the friedewald equation versus a direct homogenous low density lipoprotein-cholesterol assay. Lab Med. 2017; 48:220–4.


34. Mora S, Rifai N, Buring JE, Ridker PM. Comparison of LDL cholesterol concentrations by Friedewald calculation and direct measurement in relation to cardiovascular events in 27,331 women. Clin Chem. 2009; 55:888–94.
35. Sniderman AD, Williams K, Contois JH, Monroe HM, McQueen MJ, de Graaf J, et al. A meta-analysis of low-density lipoprotein cholesterol, non-high-density lipoprotein cholesterol, and apolipoprotein B as markers of cardiovascular risk. Circ Cardiovasc Qual Outcomes. 2011; 4:337–45.


Fig. 1.
Passing-Bablock regression analysis of the correlation between Barozen Lipid Plus (MICO Biomed Co., Ltd. Seongnam, Korea) and Roche-Hitachi Cobas 8000 c702 (Hitachi High-Technologies Corporation, Tokyo, Japan) for (A) total cholesterol (TC), (B) triglycerides, and (C) high-density lipoprotein (HDL) cholesterol.
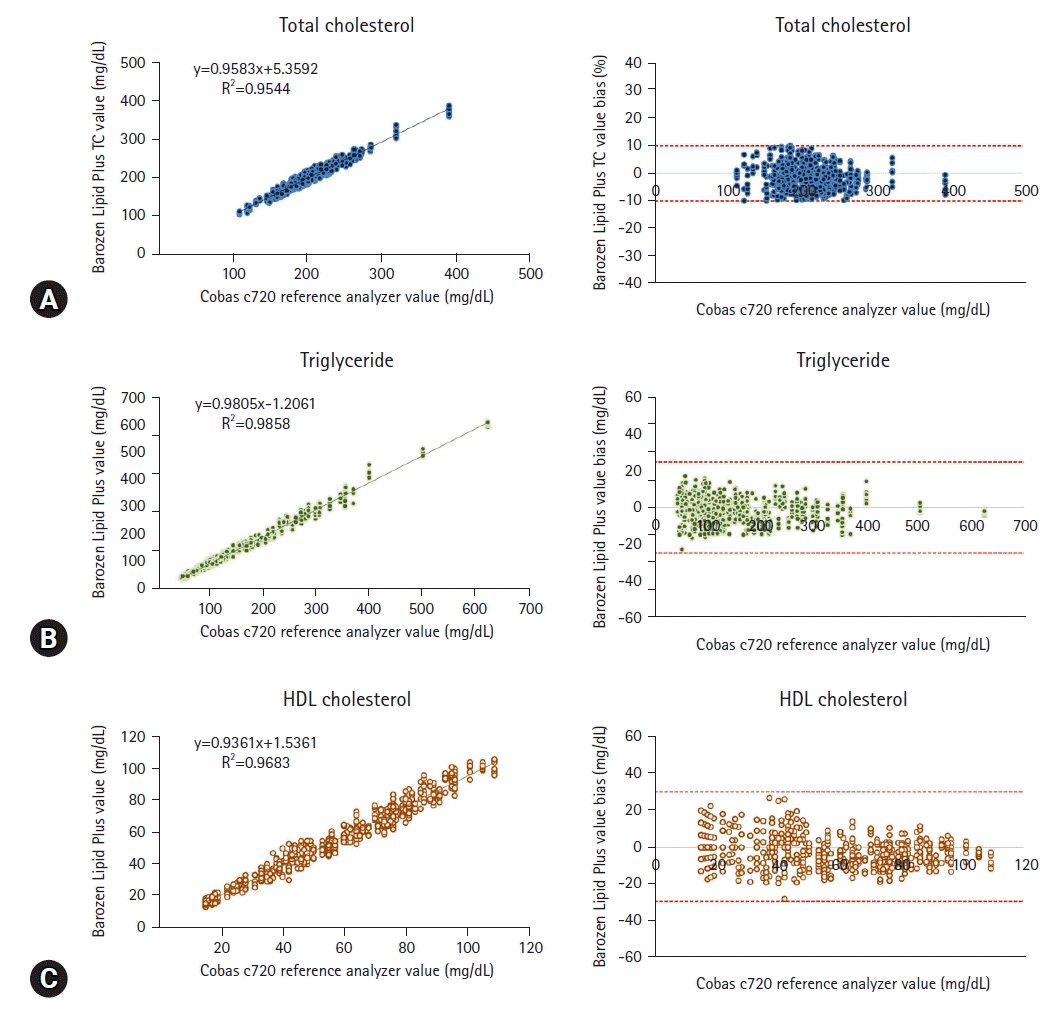
Table 1.
Demographic information of participants
Variable | Total |
---|---|
No. of patients | 110 |
Age (yr) | |
20s | 41 (37.3) |
30s | 27 (24.5) |
40s | 23 (20.9) |
50s | 12 (10.9) |
≥60s | 7 (6.4) |
Male sex | 53 (48.2) |
Table 2.
Measurement repeatability for total cholesterol, triglycerides, and HDL cholesterol obtained by the Barozen Lipid Plus
Table 3.
Measurement precision for total cholesterol, triglycerides, and HDL cholesterol obtained by the Barozen Lipid Plus
Table 4.
Measurement accuracy of the Barozen Lipid Plus compared to that of the Roche-Hitachi Cobas 8000 c702
Table 5.
Impact of potential interfering substances using the guidelines of the Clinical and Laboratory Standards Institute and Clinical Laboratory Improvement Amendments