Abstract
Objective
To examine the efficacy of MSH6 and PMS2 immunohistochemistry (IHC) as a screening method for Lynch syndrome in endometrial cancer patients.
Methods
Through multidisciplinary discussions, an institutional MSH6 and PMS2 IHC-initiated cascade test (MSH6, PMS2 IHC→microsatellite instability [MSI] assay→germline mismatch repair [MMR] gene sequencing) was developed to screen for Lynch syndrome in endometrial cancer patients. Testing was performed on a consecutive cohort of 218 newly diagnosed endometrial cancer patients who underwent surgery at a tertiary hospital in the Republic of Korea between August 2018 and December 2020. The number of MMR deficiencies (MSH6 or PMS2 loss in IHC) and results of subsequent tests (MSI assay and germline MMR gene sequencing) were examined.
Results
MMR deficiency was detected in 52 of the 218 patients (24.0%). Among these 52 patients, 34 (65.0%) underwent MSI testing, of which 31 (91.0%) exhibited high MSI. Of the 31 patients with MSI-high status, 15 (48.0%) underwent germline MMR gene sequencing. Subsequently, Lynch syndrome was diagnosed in five patients (33.0%).
Lynch syndrome (LS) is a cancer-prone syndrome associated with a mutation in one of four mismatch repair (MMR) genes: MLH1, MSH2, MSH6, or PMS2 [1]. MMR deficiency (MMRd) results in microsatellite instability (MSI), hypermutated phenotypes, and an increased cancer risk [2]. Women with LS are at an increased risk of colorectal, endometrial, ovarian, renal, pelvic, and ureteral cancers [1] and most commonly develop colorectal and endometrial cancers (EC) [3,4].
EC is often the sentinel cancer in women with LS [5]. Therefore, the diagnosis of LS in EC patients could enable early cancer surveillance and provide significant survival benefits. The diagnosis of LS in EC patients is an essential step in clinical management, as it allows cascade testing to identify family members who may also have the disease [6]. Therefore, it is essential to screen EC patients for LS.
Various strategies have been suggested to identify individuals with LS. These typically include a combination of clinical/family history-based criteria, such as Amsterdam I/II criteria [7], Revised Bethesda guidelines [8], tumor tissue testing (immunohistochemistry [IHC], MSI), algorithm-based prediction models, or universal germline genetic testing. Each of these strategies has certain advantages and disadvantages. Screening using clinical/family history criteria or algorithm-based prediction models is inexpensive but strongly relies on the availability of accurate risk information [9]. Moreover, universal screening requires more resources than clinical or family history-based approaches. Nonetheless, universal screening offers additional clinical insights, including MSI status or specific protein expression absence, and enhances accessibility to germline genetic testing among individuals at risk [10,11].
The National Comprehensive Cancer Network 2018 guidelines recommend universal screening of endometrial tumors for loss of MMR protein expression [12] because aberrant MMR protein expression is a hallmark of LS. IHC screening is an excellent technique for determining LS risk by detecting the functional protein products of MLH1, MSH2, MSH6, and PMS2 in tumor tissues [13]. However, resource limitations have hindered the adoption of universal screening tests. For example, in Korea, universal screening using IHC for four MMR markers or MSI assays is not widely adopted because the health insurance policy does not cover this screening test.
Therefore, it is necessary to develop a feasible LS screening strategy for EC patients through a multidisciplinary discussion. This study examines the feasibility and performance of the developed LS screening strategy.
This study was approved by the Institutional Review Board (B-2104-681-103). Women with EC who underwent MSH6 and PMS2 IHC-initiated cascade testing for LS between August 2018 and December 2020 were included. Pathological diagnosis, treatment, and follow-up of all the included women were performed at an academic hospital in the Republic of Korea.
Data, including age, histological type, cancer staging, MMR IHC expression profiles, and subsequent tests (MSI assay and MMR gene sequencing), were retrieved from the medical records. Patients characteristics of those with LS were collected.
In August 2018, IHC-initiated cascade testing for MSH6 and PMS2 for LS screening in EC patients was conducted through multidisciplinary discussions with gynecologic oncologists, colorectal surgeons, pathologists, and laboratory medicine doctors (Fig. 1). Universal screening was adopted for its higher sensitivity [14,15], considering the challenges of acquiring detailed family histories. Accordingly, all women diagnosed with any type of EC underwent IHC testing for LS.
The decision to use IHC for MMR markers as the primary test, instead of the MSI assay, was based on both cost-effectiveness and comparable sensitivity [15,16]. Staining for MMR proteins was performed using primary monoclonal antibodies.
Antibodies against MSH6 (GRBP.P1/2. D4, 1:200; Serotec Inc., Raleigh, NC, USA) and PMS2 (A16-4, 1:200; BD PharMingen, Franklin Lakes, NJ, USA) were used according to the manufacturer’s instructions. Assessment of MMR protein expression involved nuclear staining of tumor cells and subsequent comparison with the nuclear staining of internal positive control cells, such as non-neoplastic epithelial cells, stromal cells, and lymphocytes. Expression was defined as abnormal if one or more of the MMR proteins were completely absent from all tumor cell nuclei [17] (Fig. 2). The selection of these markers was based on previously reported data indicating comparable sensitivity between IHC for MSH6 and PMS2 and the traditional four-marker tests (MLH1, MSH2, MSH6, and PMS2) [18]. These findings indicate that MSH6 and PMS2 can effectively serve as standalone markers for the detection of LS. Notably, our decision to screen for MSH6 and PMS2 was supported by a recent prospective study that investigated and compared LS screening methods with clinical diagnostic criteria, such as the Amsterdam II criteria and revised Bethesda guidelines. This study included the assessment of MSI and IHC of MMR proteins in tumor tissues [15], and the results of this study further validate the use of MSH6 and PMS2 as reliable markers for LS screening.
For patients showing MSH6 or PMS2 loss on IHC, a polymerase chain reaction (PCR)-based MSI assay was conducted to validate the IHC test results, considering the potential subjectivity of IHC. Further, macrodissection and DNA extraction (InstaGene Matrix; Bio-Rad, Hercules, CA, USA) were performed on tumor and non-neoplastic normal tissues, respectively. DNA samples were subjected to PCR using a National Cancer Institute (NCI) panel of five markers, including two mononucleotide markers (BAT-26 and BAT-25) and three dinucleotide markers (D5S346, D17S250, and D2S123). PCR products were analyzed using an automated sequencer (ABI 3731 Genetic Analyzer; Applied Biosystems, Foster City, CA, USA). Microsatellite status was assessed to determine changes in the allele profiles of tumor cells compared to those of the corresponding non-neoplastic normal tissues. MSI-high status was defined as two or more NCI markers with unstable peaks, while MSI-low/microsatellite stable status was defined as one or no NCI markers with unstable peaks [8].
Germline MMR gene sequencing was performed in patients with high MSI. Targeted panel sequencing of inherited cancer-related genes, including MLH1, MSH2, MSH6, and PMS2, was performed using an Ion S5 XL system (Thermo Fisher Scientific, Cambridge, MA, USA). A methylation test for MLH1 was not performed owing to the unavailability of an in-house test. IHC-initiated cascade testing for MSH6 and PMS2 was performed at our institute in August 2018.
The number of negative IHC results for MSH6 and PMS2 and subsequent tests was determined. The proportion of patients with MSI-high status among those with negative IHC expression of MSH6 and PMS2 was examined to evaluate the requirement for the MSI assay as a subsequent test. Additionally, the characteristics of the diagnosed LS cases were summarized.
In total, 218 women with EC (mean age, 55 years) underwent our screening protocol. Of these EC cases, 213 (88.0%) were classified as endometrioid and 12 (4.9%) as serous. Stage I and II EC were diagnosed in 196 women (81.0%), with histological grade 1–2 observed in 171 women (70.7%) (Table 1).
MSH6 and PMS2 were not expressed in 52 patients. Of these 52 women, 34 underwent MSI testing, resulting in 31 cases being identified as MSI-high. Of these 31 patients with MSI-high status, 15 underwent germline MMR gene sequencing, leading to the diagnosis of LS in five cases (Fig. 1).
Of the 34 women who underwent the MSI assay, 31 showed MSI-high status (91.2%), while three exhibited MSI-low and microsatellite-stable results.
The clinical, pathological, and genetic characteristics of LS patients are summarized in Table 2. Of the five patients diagnosed with LS, four were older than 50 years. All patients were histologically diagnosed with endometrioid cancer. The identified mutated gene was MLH1 in one patient, MSH2 in two patients, MSH6 in one patient, and PMS2 in one patient.
The appropriate methods and strategies for LS screening in patients with EC remain debatable [1]. However, recent reports and guidelines support universal molecular screening rather than clinical criteria [15,19,20] because approximately 70.0% of LS patients do not meet the Bethesda guidelines or Amsterdam II criteria [21].
However, universal screening requires additional resources and is not cost-effective in some populations, limiting its implementation. For example, the cost of IHC for MMR proteins, MSI testing, and germline sequencing has been reported to be approximately 143, 214, and 500 USD per patient, respectively, in China [15]. In our institute, the approximate cost for IHC testing of MMR proteins (two markers), MSI test, and germline sequencing is 70, 180, and 700 USD, respectively. These costs may be even higher in other countries.
This study investigated the performance of a new and cheaper universal screening test for LS, which involves testing for only two MMR proteins (MSH6 and PMS2) in a group of women with EC. These two proteins were chosen based on previously reported data showing comparable sensitivity between IHC for these MMR markers (MSH6 and PMS2) and the four-marker tests (MLH1, MSH2, MSH6, and PMS2) [18].
This decision to screen for MSH6 and PMS2 was supported by a recent prospective study that explored LS screening methods and compared the clinical diagnostic criteria (Amsterdam II criteria and the revised Bethesda guidelines), MSI tests, and IHC for MMR proteins in tumor tissues [15].
IHC was performed on 102 patients in the above study, which revealed that 28 patients (27.5%) were MMR protein-deficient, including 14 with both MLH1 and PMS2 protein deletion, five with both MSH2 and MSH6 deletion, three with MSH6 deletion, three with PMS2 deletions, and one each with MLH1, MSH2, and MLH1 and MSH6 deletions [15]; 26 of the 28 patients (92.8%) had at least one MSH6 or PMS2 deletion.
Previous studies have shown that testing for the loss of MMR function via IHC or MSI is a cost-effective screening test with high sensitivity and specificity [19,20]. Additionally, the concordance between the four- and two-MMR protein (MSH6 and PMS2) tests was high, along with the same sensitivity and specificity for LS screening [18], all of which support our theory and screening protocol (i.e., testing only for PMS2 and MSH6).
In our cohort, MMR gene sequencing confirmed LS in five women (2.0%) with EC after screening. The prevalence of LS in EC patients is consistent with the reported range of 2.1–3.9% [19,20]. However, other studies have reported a higher prevalence of 5.9–6.6% [15,22]; the different screening protocols and study populations most likely drive these variations.
Our findings suggest a high concordance between high MSI and two protein MMRd, in which 31 of 34 patients (91.0%) with two protein MMRd exhibited MSI-high status, which is consistent with the results of another prospective study (94.8%) [15]. These findings support the accuracy of the screening protocol.
MMRd was observed in 52 women in our cohort; ultimately, five (9.6%) were diagnosed with LS. In another study [15] of 28 cases of MMRd, six women (21.4%) were diagnosed with LS; however, in that study [15], the rate of LS was higher than that in our cohort (5.4% vs. 2.0%), which can explain this difference.
Our model excludes the family history-based screening step from the screening strategy because of its low accuracy and changes in the current social environment. Small family size and incomplete knowledge of family cancer history can limit screening accuracy and lead to incorrect clinical decisions [23]. These data indirectly support the sensitivity, specificity, cost-effectiveness, and availability of the screening model.
These results highlight several key findings. Implementation of a cascade testing strategy based on MSH6 and PMS2 IHC for LS screening in EC patients revealed a subset of cases with loss of MSH6 or PMS2 expression. A subsequent MSI assay confirmed a high MSI status in most of these cases, validating the utility of MSI testing as a follow-up step. Furthermore, germline MMR gene sequencing in MSI-high patients identified a subset of LS patients, demonstrating the clinical relevance of this approach.
However, the study also observed discordance between MMR IHC and gene sequencing in some cases. Despite showing MSH6 and PMS2 loss in IHC testing, not all cases exhibited mutations in these genes upon sequencing. This discordance could arise from various factors such as technical limitations of the methods, somatic mutations not detected by sequencing, or the involvement of other MMR genes.
The strength of this study is that the screening strategy was devised through multidisciplinary discussions with experts. This screening test was performed in our institute over the last 2 years, and the LS diagnosis rate was compatible with other research results.
Notably, to ensure the validity and reliability of the experimental results, controls are vital in IHC studies as they serve as reference points for comparing the experimental samples, thus allowing the assessment of staining specificity and accuracy. We used non-neoplastic normal tissues expressing MMR proteins as the internal controls.
By including non-neoplastic normal tissues, we established a reliable baseline for MMR protein expression analysis. These tissues inherently express MMR proteins and show consistent staining patterns. Comparison of the staining intensity and pattern in neoplastic tissues with these internal controls enabled us to accurately determine the presence or absence of MMR proteins. The use of non-neoplastic normal tissues as internal controls eliminates the need for additional external controls. This streamlined approach enhanced the internal validity of our results, allowing us to confidently assess the complete loss of MMR proteins in neoplastic tissues, without introducing potential confounding factors associated with external controls.
Overall, the inclusion of non-neoplastic normal tissues expressing MMR proteins as an internal control strengthened the reliability and accuracy of our IHC analysis. These internal controls provided a reliable reference for MMR protein expression, simplifying the experimental design without compromising the integrity of our findings.
Currently, there are no common national screening strategies for LS in South Korea. Other researchers have launched testing strategies for LS; however, these strategies are not necessarily suitable for different populations, as they do not have the same healthcare system, insurance coverage, and genetic features. Therefore, it is necessary to develop a straightforward and cost-effective strategy for LS screening. The LS screening algorithm used in this study may be valuable for this purpose. Further prospective studies are required to confirm these hypotheses.
The current study had some limitations. The investigation into the potential link between EC risk and family history, while accounting for the proband’s MMR status and excluding probands with pathogenic MMR mutations, indicated that MMR genes may not adequately explain the familial EC risk [24]. In addition, polygenic interactions have been proposed as the most likely cause of cancer in LS patients [25]. Therefore, additional studies on familial EC are required [26].
Moreover, the limited generalizability of our findings is attributed to the retrospective design and small sample size of the current study, as well as the limited number of women whose MMR genes were evaluated. To confirm the sensitivity and specificity of this screening test, it would be ideal to include a control group of patients who underwent MMR protein IHC testing along with MSI and gene sequence testing for LS. Furthermore, evaluating the MMR genes in women with negative IHC results would provide valuable insights. Instead, we used data from other studies to compare and confirm the effectiveness of the screening tests.
Moreover, the high numbers of missing values in the screening tests is a limitation of our study; as mentioned, this test was not covered by insurance, and some patients refused to complete the screening tests (MSI and gene sequence test). Finally, this study presents a comprehensive cascade testing strategy for LS screening in EC patients based on MSH6 and PMS2 IHC. While this approach offers simplification and efficiency, the observed discordance between MMR IHC and gene sequencing in some cases highlights the need for continued research and refinement of strategies to enhance the accuracy and clinical utility of LS screening in EC.
Based on these results, we updated the LS screening guidelines (Fig. 3). As mentioned, a family history-based screening strategy is inefficient. Hence, we initiated IHC testing for two minor markers, MSH6 and PMS2. MSI testing was not included in the updated version owing to the high accuracy achieved through MSI testing using MMR protein IHC. MMR gene sequencing was chosen as the second test for the final diagnosis, considering the patient’s economic status. According to the Korean National Healthcare Insurance, a patient with a suspected hereditary disease can be compensated for 50.0% of the cost of a genetic panel test.
Along with the cost issue, among the five patients with LS, three showed discordance between MMR IHC and next-generation sequencing. Therefore, the LS gene panel test appeared to be more viable than individual genetic tests. The outcomes of this retrospective investigation can serve as a foundation for future prospective studies that incorporate follow-up assessments.
To identify LS in women presenting with EC, IHC screening for two MMR proteins was proposed. According to our results, LS gene sequencing is a sensitive and cost-effective screening test. The MSI and IHC results for the two MMR proteins were highly concordant in the EC screening.
References
1. Lynch HT, Snyder CL, Shaw TG, Heinen CD, Hitchins MP. Milestones of Lynch syndrome: 1895–2015. Nat Rev Cancer. 2015; 15:181–94.


2. Ahadova A, Gallon R, Gebert J, Ballhausen A, Endris V, Kirchner M, et al. Three molecular pathways model colorectal carcinogenesis in Lynch syndrome. Int J Cancer. 2018; 143:139–50.
3. Barrow E, Robinson L, Alduaij W, Shenton A, Clancy T, Lalloo F, et al. Cumulative lifetime incidence of extracolonic cancers in Lynch syndrome: a report of 121 families with proven mutations. Clin Genet. 2009; 75:141–9.


4. Møller P, Seppälä T, Bernstein I, Holinski-Feder E, Sala P, Evans DG, et al. Cancer incidence and survival in Lynch syndrome patients receiving colonoscopic and gynaecological surveillance: first report from the prospective Lynch syndrome database. Gut. 2017; 66:464–72.


5. Lu KH, Dinh M, Kohlmann W, Watson P, Green J, Syngal S, et al. Gynecologic cancer as a “sentinel cancer” for women with hereditary nonpolyposis colorectal cancer syndrome. Obstet Gynecol. 2005; 105:569–74.


6. Hampel H. Genetic counseling and cascade genetic testing in Lynch syndrome. Fam Cancer. 2016; 15:423–7.


7. Vasen HF, Watson P, Mecklin JP, Lynch HT. New clinical criteria for hereditary nonpolyposis colorectal cancer (HNPCC, Lynch syndrome) proposed by the International Collaborative group on HNPCC. Gastroenterology. Gastroenterology. 1999; 116:1453–6.


8. Umar A, Boland CR, Terdiman JP, Syngal S, de la Chapelle A, Rüschoff J, et al. Revised Bethesda guidelines for hereditary nonpolyposis colorectal cancer (Lynch syndrome) and microsatellite instability. J Natl Cancer Inst. 2004; 96:261–8.
9. Kalloger SE, Allo G, Mulligan AM, Pollett A, Aronson M, Gallinger S, et al. Use of mismatch repair immunohistochemistry and microsatellite instability testing: Exploring Canadian practices. Am J Surg Pathol. 2012; 36:560–9.
10. Hampel H, Frankel WL, Martin E, Arnold M, Khanduja K, Kuebler P, et al. Screening for the Lynch syndrome (hereditary nonpolyposis colorectal cancer). N Engl J Med. 2005; 352:1851–60.


11. Cohen SA. Current Lynch syndrome tumor screening practices: a survey of genetic counselors. J Genet Couns. 2014; 23:38–47.


12. Koh WJ, Abu-Rustum NR, Bean S, Bradley K, Campos SM, Cho KR, et al. Uterine neoplasms, version 1.2018, NCCN clinical practice guidelines in oncology. J Natl Compr Canc Netw. 2018; 16:170–99.


13. Martin SA, Lord CJ, Ashworth A. Therapeutic targeting of the DNA mismatch repair pathway. Clin Cancer Res. 2010; 16:5107–13.


14. Adar T, Rodgers LH, Shannon KM, Yoshida M, Ma T, Mattia A, et al. Universal screening of both endometrial and colon cancers increases the detection of Lynch syndrome. Cancer. 2018; 124:3145–53.


15. Chao X, Li L, Wu M, Ma S, Tan X, Zhong S, et al. Comparison of screening strategies for Lynch syndrome in patients with newly diagnosed endometrial cancer: a prospective cohort study in China. Cancer Commun (Lond). 2019; 39:42.


16. Stelloo E, Jansen AML, Osse EM, Nout RA, Creutzberg CL, Ruano D, et al. Practical guidance for mismatch repair-deficiency testing in endometrial cancer. Ann Oncol. 2017; 28:96–102.


17. Kim G, Lee SK, Suh DH, Kim K, No JH, Kim YB, et al. Clinical evaluation of a droplet digital PCR assay for detecting POLE mutations and molecular classification of endometrial cancer. J Gynecol Oncol. 2022; 33:e15.
18. Yuan L, Chi Y, Chen W, Chen X, Wei P, Sheng W, et al. Immunohistochemistry and microsatellite instability analysis in molecular subtyping of colorectal carcinoma based on mismatch repair competency. Int J Clin Exp Med. 2015; 8:20988–1000.
19. Goodfellow PJ, Billingsley CC, Lankes HA, Ali S, Cohn DE, Broaddus RJ, et al. Combined microsatellite instability, MLH1 methylation analysis, and immunohistochemistry for Lynch syndrome screening in endometrial cancers from GOG210: an NRG Oncology and Gynecologic Oncology group study. J Clin Oncol. 2015; 33:4301–8.
20. Goverde A, Spaander MC, van Doorn HC, Dubbink HJ, van den Ouweland AM, Tops CM, et al. Cost-effectiveness of routine screening for Lynch syndrome in endometrial cancer patients up to 70years of age. Gynecol Oncol. 2016; 143:453–9.
21. Cohen SA, Leininger A. The genetic basis of Lynch syndrome and its implications for clinical practice and risk management. Appl Clin Genet. 2014; 7:147–58.


22. Mills AM, Liou S, Ford JM, Berek JS, Pai RK, Longacre TA. Lynch syndrome screening should be considered for all patients with newly diagnosed endometrial cancer. Am J Surg Pathol. 2014; 38:1501–9.


23. Tranø G, Sjursen W, Wasmuth HH, Hofsli E, Vatten LJ. Performance of clinical guidelines compared with molecular tumour screening methods in identifying possible Lynch syndrome among colorectal cancer patients: a Norwegian population-based study. Br J Cancer. 2010; 102:482–8.


24. Johnatty SE, Tan YY, Buchanan DD, Bowman M, Walters RJ, Obermair A, et al. Family history of cancer predicts endometrial cancer risk independently of Lynch syndrome: implications for genetic counselling. Gynecol Oncol. 2017; 147:381–7.


Fig. 1
Lynch syndrome screening guideline for endometrial cancer patients. IHC, immunohistochemistry; MSI, microsatellite instability; MSS, microsatellite stable; MSI-L, microsatellite instability-low; MSI-H, microsatellite instability-high; MMR, mismatc repair gene.
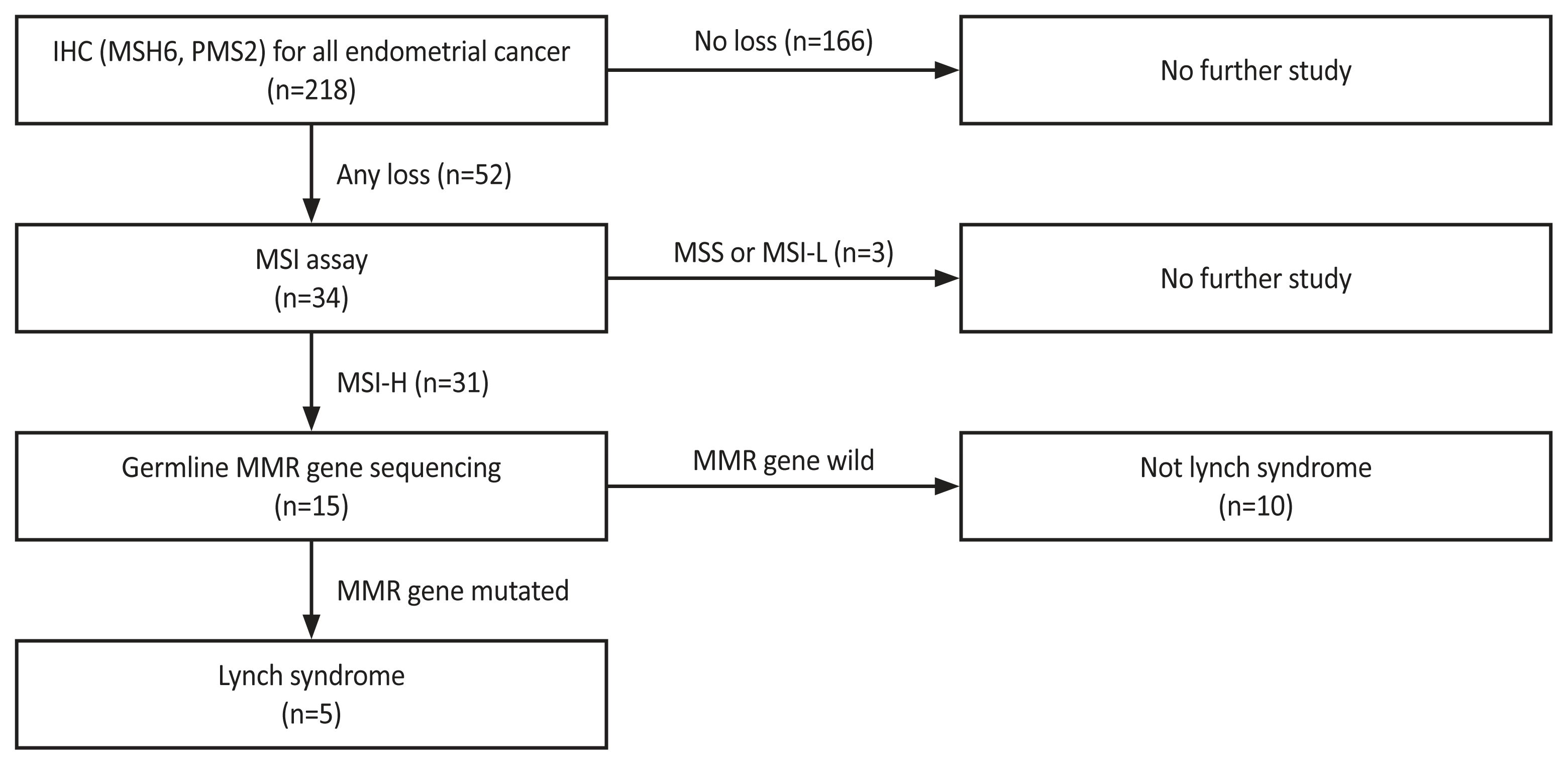
Fig. 2
Representative immunohistochemical images of MSH6 and PMS2 protein expression. (A) Intact MSH6 expression. Complete loss of MSH6 (B) and PMS2 (C) expression (×100 magnification).
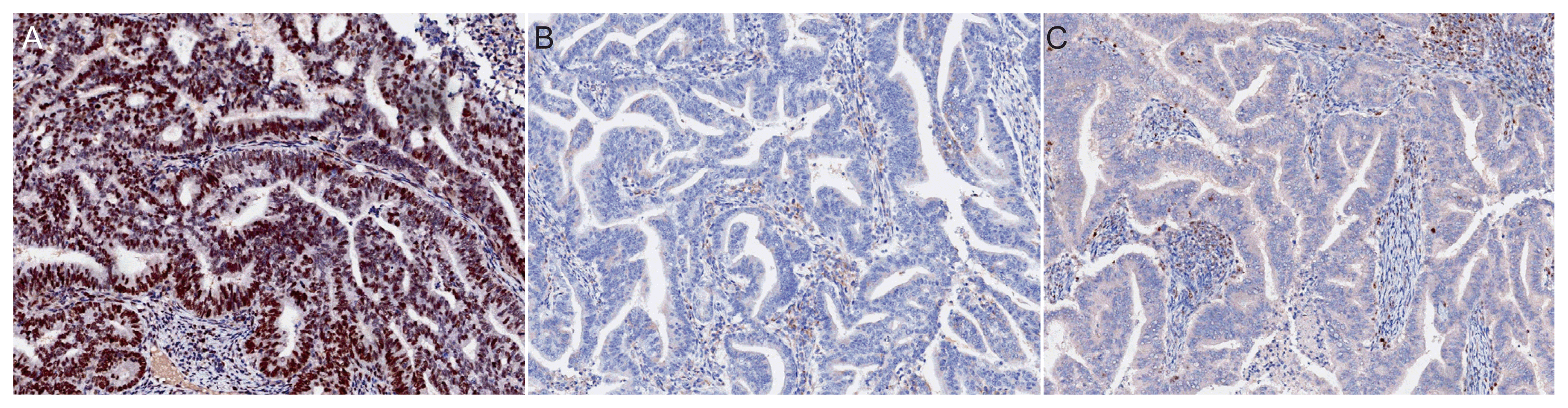
Fig. 3
Updated guideline for Lynch syndrome screening. IHC, immunohistochemistry; MMR, mismatch repair gene.
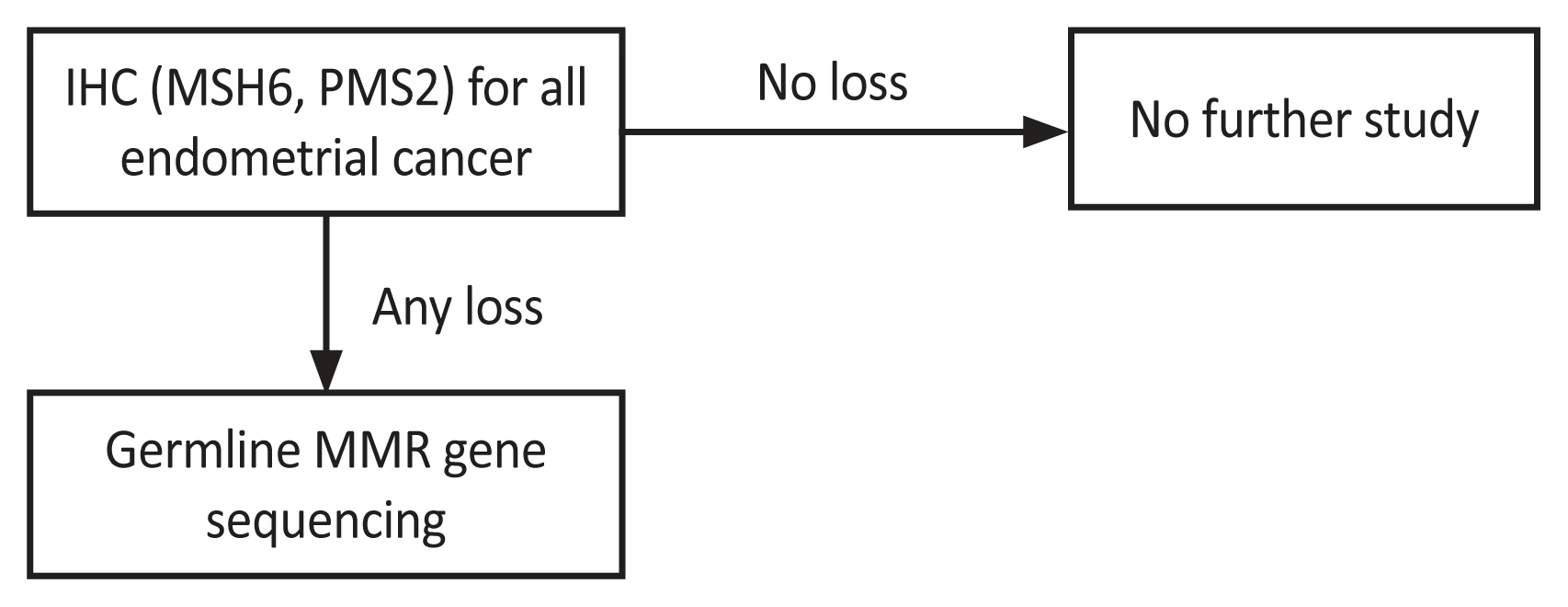
Table 1
Baseline data and pathological characteristics of women with endometrial cancer who underwent our screening protocol
Table 2
Characteristics of women diagnosed with Lynch syndrome