Abstract
The cervical spine plays a critical role in supporting the skull, maintaining horizontal gaze, and facilitating walking. Its unique characteristics, including the widest range of motion among spinal segments, have led to extensive research on cervical sagittal alignment. Various parameters have been proposed to evaluate cervical alignment, with studies investigating their clinical significance, correlation with symptoms, and implications for surgical interventions. Recent findings suggest that cervical sagittal alignment not only impacts the cervical spine but also influences global spine-pelvic alignment through compensatory mechanisms. This comprehensive review examines classical and new parameters of cervical sagittal alignment and considers the dynamic and muscular factors associated with it.
The cervical spine plays a crucial role in supporting the axial load of the skull, enabling horizontal gaze, and facilitating ambulation. Compared to other spinal segments, the cervical spine exhibits the widest range of motion. Various parameters have been proposed to evaluate cervical sagittal alignment as an attempt to understand its unique characteristics. Extensive researches have been conducted to explore the clinical significance of these parameters, their association with pre- and postoperative symptoms, and their implications in surgical interventions [1,2,5-7,9,11,13,15,18-20,21,24,25,29-31,35,37,39]. Ames et al. [2] presented a classification system for cervical spine deformity through a modified Delphi approach, adopting widely accepted cervical alignment parameters as modifiers. Ames’ classification system is currently the widely accepted classification system for cervical spine deformity. Subsequent research has introduced new parameters. Protopsaltis et al. [29] introduced the concept of C2 slope (C2S) and Fujiyoshi et al. [6] introduced the concept of K-line. In this review article, the parameters selected as modifiers in the Ames’ classification are categorized as classical parameters, while the newly proposed parameters from subsequent research are categorized as new parameters. New studies are actively conducted to comprehensively understand cervical spine sagittal balance by measuring parameters not only from plain radiograph but also from dynamic radiograph, which includes extension and flexion views [13,21]. Additionally, there is ongoing research evaluating the characteristics of cervical muscles through magnetic resonance imaging to understand their impact on cervical spine sagittal balance after cervical spine surgery [17,27,28]. This review aims to consolidate the latest insights into cervical alignment by discussing classical and new parameters of cervical sagittal alignment, as well as the dynamic and muscle factors associated with it.
Cervical lordosis (CL) is measured using various methods (e.g., the modified Cobb method, Jackson 50 physiological stress lines, and Harrison’s posterior tangent method) (Fig. 1) [1]. Among these, the modified Cobb method is widely used due to its convenience and high reliability between measurements. Two lines are drawn along the inferior end plates of C2 and C7 to measure CL. Additional lines are then drawn perpendicular to these initial lines, and the angle formed by the perpendicular lines represents the value of CL. Although numerous studies have investigated CL, a definitive definition of physiological normal values has yet to be established [7,8,16]. This lack of consensus can be attributed to a high prevalence of kyphotic cervical alignment in asymptomatic individuals [16]. Consequently, researchers have emerged focusing on understanding CL as a compensatory mechanism to maintain horizontal gaze in an upright position [5,11,16,20]. In 2012, Lee et al. [20] were the first to report an association between CL and the thoracic inlet parameters. The thoracic inlet angle (TIA) is defined as the angle between a line perpendicular to the midpoint of the upper endplate of the T1 vertebra and a line connecting the end of the sternum and the midpoint of the upper endplate of the T1 vertebra. The T1 slope (T1S) is defined as the angle between the upper endplate of the T1 vertebral body and a horizontal line. Neck tilt is defined as the angle between a line connecting the end of the sternum and the midpoint of the upper endplate of the T1 vertebra and a vertical line. Anatomically, the cervical spine is located above the T1 vertebral body, fixed to the sternum and the first rib, with minimal range of motion. Greater TIS means inclined thoracic inlet which requires greater CL to maintain horizontal gaze. Lee et al. [20] demonstrated a statistically significant increase in CL with increasing TIA and T1S values, suggesting compensatory changes in CL as a result. Pearson coefficient between TIA and CL was -0.488 and pearson coefficient between T1S and CL was -0.624. Through numerous studies on thoracolumbar spine alignment, the association between the shape of the pelvis, measured by pelvic incidence (PI), and lumbar lordosis (LL) has been established [3,4,22,32,36]. The mismatch between the pelvis and lumbar spine, measured by the PI-LL value, has been shown to be correlated with Health-Related Quality of Life (HRQL) and peri operative symptoms and clinical outcomes [3,22,32,36]. The relationship between T1S and CL is similar to relationship between PI and LL in thoracolumbar spinal deformity (Fig. 2). As a result, T1S-CL has been proposed as an analogue parameter to measure the mismatch between T1S and CL. In a study by Oe et al. [25], cervical sagittal alignment parameters and HRQL, measured by Euro QOL (EQ-5D), were reviewed in a cohort of 656 patients aged 50 years and above. The average T1S value was reported to be 33.2°±3.7° for males and 31.7°±8.2° for females, while the average T1S-CL value was 21.3°±9.4° for males and 17.9°±8.5° for females [33]. Statistically significant lower EQ-5D scores were observed in cases where T1S was ≥40° or T1S-CL was ≥20°. T1S-CL has been identified as a valuable predictor of postoperative complications and associated with poorer clinical outcomes and HRQL [1,2,5,9]. Hyun et al. [9] reported an association between T1S-CL and clinical outcomes in patients who had multilevel cervical fusion surgery and found that T1S-CL >22.2° was associated with Neck Disability Index (NDI) >25 and cervical malalignment.
As measuring global spinal sagittal alignment using the C2-S1 or C7-S1 sagittal vertical axis (SVA), C2-7 SVA, distance between C2 plumb line and posterior, superior endplate of C7 body, has been corelated to greater neck disability, decreased quality of life, myelopathy and increased risk of postoperative complications in patients undergoing cervical spine surgery (Fig. 3) [1,2,33,38]. Tang et al. [38] reported a correlation between C2-7 SVA and NDI and proposed a threshold of C2-7 SVA 40 mm using a regression model in the study of patients who had posterior cervical fusion surgery. Smith et al. [33] were the first to report on the correlation between C2-7 SVA and modified Japanese Orthopedic Association (mJOA) in a study involving 56 patients with cervical spondylotic myelopathy (CSM). Ames et al. [2] proposed a cervical deformity (CD) classification system and adopted C2-7 SVA and T1S-CL as modifiers. By incorporating expert opinions and literature review, they categorized C2-7 SVA into three groups with score 0, 1, 2 respectively : less than 4 cm, between 4 cm and 8 cm, and greater than 8 cm. Similarly, T1S-CL was categorized into three groups : less than 15° and between 15° and 20°, and greater than 20°.
The most important role of the cervical spine is to maintain horizontal gaze and ensure the necessary field of vision through the craniocervical range of motion. The chin-brow to vertical angle (CBVA) is a measurement tool used to assess horizontal gaze. It is defined as the angle between a line drawn from the patient’s chin to brow and a vertical line. Measuring the CBVA, the patient is positioned with extended hips and knees, and the neck is maintained in a neutral position. When the neck is flexed, the CBVA becomes positive. Suk et al. [37] studied 34 ankylosing spondylitis patients with cervical ankylosis who underwent pedicle subtraction extension osteotomy for kyphotic deformity correction. Seven patients with a CBVA less than -10° (overcorrection) exhibited significantly low scores on horizontal gaze, especially in walking downstairs [37]. Proper CBVA has been linked to improved horizontal gaze, ambulation, and activities of daily living including cooking and toileting where downward gaze is required [10,35,37]. Given the fundamental impact of horizontal gaze on human function and the influence of CBVA on spinal deformity correction, CBVA has been included as a modifier in the cervical spinal deformity classification.
C2S is an angle between the C2 lower endplate and the horizontal line. Protopsaltis et al. [29] proposed C2S as a novel cervical sagittal alignment parameter that is a mathematical approximation of T1S-CL. In this study, the C2S was found to have correlations with T1S-CL and C2-7 SVA. And CD patients showed correlation between C2S and one-year postoperative outcomes; the group with cervical region deformity apex showed correlation with EQ-5D, the cervicothoracic region group showed correlations with NDI, mJOA, neck pain, and EQ-5D. Passfall et al. [26] investigated the correlation between C2S and HRQL metrics and the development of postoperative radiographic complication, distal junctional failure, in CD patients who underwent cervical fusion surgery. The results showed significant associations between 3-month C2S and HRQL metrics, the development of distal junctional failure. Post fusion 3-month C2S <10° associated with a higher chance of achieving optimal outcomes by logistic regression analysis. C2S involves the measurement of a single angle, resulting in less interobserver error compared to the measurement of three angular slopes in parameters like T1S-CL. And the endplate of the C2 vertebra is typically more visible on plain radiographs compared to the endplates of C7 or T1. These advantages make the C2 slope a valuable parameter for assessing CD and cervico-thoracic junctional harmony.
The K-line tilt is a radiographic parameter used to assess the sagittal alignment of the cervical spine in patients with ossification of the posterior longitudinal ligament (OPLL). It is measured as the angle between the K-line, which connects the midpoints of the spinal canal at C2 and C7, and a vertical line. The concept of the K-line was first introduced by Fujiyoshi et al. [6]. Patients are divided into two groups based on the K-line : the K-line (+) group, where OPLL does not exceed the K-line, and the K-line (-) group, where OPLL exceeds the K-line (Fig. 4). The patients in the K-line (-) group did not experience satisfactory posterior shift of the spinal cord or neurological improvement after posterior decompression surgery [6]. Kim et al. [14] evaluated the relationship between the K-line tilt and other cervical sagittal alignment parameters. In that study, K-line tilt was statistically correlated with C2-7 SVA and T1S-CL. Sakai et al. [31] investigated the impact of K-line tilt on surgical outcomes after cervical laminoplasty in a study involving 62 patients with K-line(+) cervical OPLL. Preoperative and postoperative radiographic measurements and clinical outcomes assessed by Japanese Orthopedic Association scoring system for cervical myelopathy (C-JOA score) were evaluated. In multivariate analysis, K-line tilt was identified as a preoperative risk factor, with a cutoff K-line tilt of 20° to predict postoperative kyphotic deformity and poor C-JOA score recovery.
Cervical sagittal alignment has been shown to interact with thoracolumbar and pelvic alignment as part of the global spine alignment. Ames et al. [1] reported in a study of 55 asymptomatic individuals that the pelvic, lumbar, thoracic, and cervical spinal regions are not independent but exhibit a reciprocal chain reaction. According to the research, as the PI increased, LL also increased (Pearson coefficient, 0.52). As LL increased, thoracic kyphosis (TK) also increased (Pearson coefficient, -0.34), and as TK increased, CL increased (Pearson coefficient, -0.51). Based on these understandings, studies on reciprocal changes in sagittal alignment after spinal segment surgery have emerged [12,23,34]. Smith et al. [34] investigated postoperative reciprocal changes in the cervical spine after lumbar pedicle subtraction osteotomy (PSO) for LL correction in 77 patients exhibiting sagittal spinopelvic malalignment. After lumbar PSO, reciprocal changes were observed in CL (from 30.8° to 21.6°, p<0.001), C2-7SVA (from 27.0 mm to 22.9 mm), and T1S (from -38.9° to -30.4°, p<0.001). Furthermore, Mizutani et al. [23] classified cervical kyphosis into head balanced and trunk balanced types based on the position of the C7 plumb line and reported its association with thoracolumbar alignment changes after cervical reconstruction surgery. Spinal segments maintain global spine alignment through a reciprocal compensation mechanism to achieve horizontal gaze and upright gait. Therefore, in understanding CD, we must always consider the reciprocal mechanism of global spine alignment to comprehend the underlying causes.
Recently, there have been attempts in various studies to classify and understand CD more comprehensively by incorporating dynamic radiographs, which include extension and flexion view [13,21]. Kim et al. [13] conducted a two-step cluster analysis to understand the nature of severe CD and revealed distinct clinical subgroups of CD based on dynamic and static radiograph. In group 1, flat-neck deformity patients had a significant cervical mismatch (TS-CL) despite some compensatory ability. Group 2, focal deformity patients were characterized by focal kyphosis between two adjacent vertebrae without a large regional cervical kyphosis. However, global alignment of the cervical spine in these patients was not compromised due to significant compensation in T1S (<22°). Group 3 consisted of patients with cervicothoracic deformity, exhibiting a very large T1S and compensatory hyperlordosis of the cervical spine, resulting in a cervical mismatch. And the specific subgroups of CD patients identified in the study required different surgical interventions for optimal outcomes. Lee et al. [21] analyzed extension function, reservoir for maintaining CL, and loss of CL in 50 patients who underwent open door laminoplasty. Extension function was defined as extension CL minus neutral CL. The researchers concluded that extension function could predict significant kyphotic change after laminoplasty better than previously known risk factors, with a cutoff value of 14.8°. Comprehensive evaluation of a patient’s ability to compensate for their deformity through flexion and extension radiographs is crucial for preoperative planning.
The importance of evaluating cervical muscle characteristics in CD patients is also considered to optimize surgical outcomes. Muscle characteristics, fatty infiltration or sarcopenia were found to be associated with sagittal alignment, functional outcomes, and postoperative results [17,27,28]. Passias et al. [27,28] quantitatively described the cervical extensor musculature in CD patients and determined the associations between posterior musculature atrophy (fatty infiltration) and progressive sagittal deformity. Fatty infiltration was measured as a ratio of functional muscle (fat-free muscle) cross-sectional area over total muscle cross sectional area. Fatty infiltration, measured as a ratio of functional muscle (fat-free muscle) cross-sectional area over total muscle cross-sectional area, indicates increased infiltration as the ratio value decreases. In that study, increased fatty infiltration was associated with mal-aligned C2-7 SVA and impaired gait and baseline fatty infiltration ratio was the strongest predictor of 1year postoperative C2-7 SVA. Koshimizu et al. [17] focused on the impact of sarcopenia (loss of skeletal muscle mass and strength associated with aging) on sagittal alignment after cervical laminoplasty in CD patients. Sarcopenia is assessed by appendicular skeletal muscle index. The results showed that patients diagnosed with sarcopenia had greater C2-7 SVA at preoperative, postoperative, and 1-year follow-up assessments. Sarcopenia group patients also had lower SF-36 Health Survey Physical Function and JOA scores.
In this review article, various parameters related to cervical sagittal balance were summarized, along with dynamic and muscle factors. While classic parameters have provided fundamental concepts and approaches to understanding cervical sagittal balance, they have limitations as they only measure cervical parameters using simple plain radiographs. Subsequent studies have begun to explore the understanding of cervical sagittal balance in dynamic views. Advanced imaging techniques have been employed to consider factors beyond bony structures that influence cervical alignment. A comprehensive evaluation of cervical sagittal alignment should encompass both classical and novel parameters, dynamic influences, and muscular characteristics. Comprehending these parameters can optimize surgical interventions and enhance postoperative outcomes for individuals with cervical spine deformities. Nevertheless, further research is warranted to fully grasp the clinical significance of these parameters and their implications in cervical spine surgery. Ultimately, personalized surgical planning approaches could be investigated to enhance outcomes based on the individual cervical alignment parameters of each patient.
Notes
References
1. Ames CP, Blondel B, Scheer JK, Schwab FJ, Le Huec JC, Massicotte EM, et al. Cervical radiographical alignment: comprehensive assessment techniques and potential importance in cervical myelopathy. Spine (Phila Pa 1976). 38(22 Suppl 1):S149–S160. 2013.
2. Ames CP, Smith JS, Eastlack R, Blaskiewicz DJ, Shaffrey CI, Schwab F, et al. Reliability assessment of a novel cervical spine deformity classification system. J Neurosurg Spine. 23:673–683. 2015.


3. Ames CP, Smith JS, Scheer JK, Bess S, Bederman SS, Deviren V, et al. Impact of spinopelvic alignment on decision making in deformity surgery in adults: a review. J Neurosurg Spine. 16:547–564. 2012.


4. Buckland AJ, Vira S, Oren JH, Lafage R, Harris BY, Spiegel MA, et al. When is compensation for lumbar spinal stenosis a clinical sagittal plane deformity? Spine J. 16:971–981. 2016.


5. Chen J, Wang J, Wei X, Guan H, Wang B, Xu H, et al. The importance of preoperative t1 slope for determining proper postoperative c2-7 cobb’s angle in patients undergoing cervical reconstruction. J Orthop Surg Res. 15:507. 2020.


6. Fujiyoshi T, Yamazaki M, Kawabe J, Endo T, Furuya T, Koda M, et al. A new concept for making decisions regarding the surgical approach for cervical ossification of the posterior longitudinal ligament: the K-line. Spine (Phila Pa 1976). 33:E990–E993. 2008.
7. Hardacker JW, Shuford RF, Capicotto PN, Pryor PW. Radiographic standing cervical segmental alignment in adult volunteers without neck symptoms. Spine (Phila Pa 1976). 22:1472–1480. discussion 1480. 1997.


8. Hey HWD, Teo AQA, Tan KA, Ng LWN, Lau LL, Liu KG, et al. How the spine differs in standing and in sitting-important considerations for correction of spinal deformity. Spine J. 17:799–806. 2017.


9. Hyun SJ, Kim KJ, Jahng TA, Kim HJ. Clinical impact of t1 slope minus cervical lordosis after multilevel posterior cervical fusion surgery: a minimum 2-year follow up data. Spine (Phila Pa 1976). 42:1859–1864. 2017.


10. Iyer S, Lenke LG, Nemani VM, Fu M, Shifflett GD, Albert TJ, et al. Variations in occipitocervical and cervicothoracic alignment parameters based on age: a prospective study of asymptomatic volunteers using full-body radiographs. Spine (Phila Pa 1976). 41:1837–1844. 2016.


11. Jun HS, Kim JH, Ahn JH, Chang IB, Song JH, Kim TH, et al. T1 slope and degenerative cervical spondylolisthesis. Spine (Phila Pa 1976). 40:E220–E226. 2015.


12. Kim CW, Hyun SJ, Kim KJ. Systematic review of reciprocal changes after spinal reconstruction surgery : do not miss the forest for the trees. J Korean Neurosurg Soc. 64:843–852. 2021.


13. Kim HJ, Virk S, Elysee J, Passias P, Ames C, Shaffrey CI, et al. The morphology of cervical deformities: a two-step cluster analysis to identify cervical deformity patterns. J Neurosurg Spine. 32:353–359. 2019.


14. Kim HS, Kim TH, Park MS, Kim SW, Chang HG, Kim JH, et al. K-line tilt as a novel radiographic parameter in cervical sagittal alignment. Eur Spine J. 27:2023–2028. 2018.


15. Kim N, Suk KS, Kwon JW, Seo J, Ju H, Lee BH, et al. Clinical significance of the c2 slope after multilevel cervical spine fusion. J Neurosurg Spine. 38:24–30. 2022.


16. Kim SW, Kim TH, Bok DH, Jang C, Yang MH, Lee S, et al. Analysis of cervical spine alignment in currently asymptomatic individuals: prevalence of kyphotic posture and its relationship with other spinopelvic parameters. Spine J. 18:797–810. 2018.


17. Koshimizu H, Sakai Y, Harada A, Ito S, Ito K, Hida T. The impact of sarcopenia on cervical spine sagittal alignment after cervical laminoplasty. Clin Spine Surg. 31:E342–E346. 2018.


18. Lan Z, Wu Z, Xu W, Huang Y. Analysis of a radiographic parameter Kline tilt following adjacent two-level anterior cervical discectomy and fusion: a retrospective study. J Orthop Surg Res. 15:131. 2020.


19. Lee JK, Hyun SJ, Yang SH, Kim KJ. Clinical impact and correlations of odontoid parameters following multilevel posterior cervical fusion surgery. Neurospine. 19:912–920. 2022.


20. Lee SH, Kim KT, Seo EM, Suk KS, Kwack YH, Son ES. The influence of thoracic inlet alignment on the craniocervical sagittal balance in asymptomatic adults. J Spinal Disord Tech. 25:E41–E47. 2012.


21. Lee SH, Son DW, Lee JS, Sung SK, Lee SW, Song GS. Does extension dysfunction affect postoperative loss of cervical lordosis in patients who undergo laminoplasty? Spine (Phila Pa 1976). 44:E456–E464. 2019.


22. Legaye J, Duval-Beaupère G, Hecquet J, Marty C. Pelvic incidence: a fundamental pelvic parameter for three-dimensional regulation of spinal sagittal curves. Eur Spine J. 7:99–103. 1998.


23. Mizutani J, Strom R, Abumi K, Endo K, Ishii K, Yagi M, et al. How cervical reconstruction surgery affects global spinal alignment. Neurosurgery. 84:898–907. 2019.


24. Nemani VM, Louie PK, Drolet CE, Rhee JM. Defining cervical sagittal plane deformity - when are sagittal realignment procedures necessary in patients presenting primarily with radiculopathy or myelopathy? Neurospine. 19:876–882. 2022.


25. Oe S, Togawa D, Nakai K, Yamada T, Arima H, Banno T, et al. The influence of age and sex on cervical spinal alignment among volunteers aged over 50. Spine (Phila Pa 1976). 40:1487–1494. 2015.


26. Passfall L, Williamson TK, Krol O, Lebovic J, Imbo B, Joujon-Roche R, et al. Do the newly proposed realignment targets for c2 and t1 slope bridge the gap between radiographic and clinical success in corrective surgery for adult cervical deformity? J Neurosurg Spine. 37:368–375. 2022.


27. Passias PG, Segreto FA, Bortz CA, Horn SR, Frangella NJ, Diebo BG, et al. Fatty infiltration of cervical spine extensor musculature: is there a relationship with cervical sagittal balance? Clin Spine Surg. 31:428–434. 2018.
28. Passias PG, Segreto FA, Horn SR, Lafage V, Lafage R, Smith JS, et al. Fatty infiltration of the cervical extensor musculature, cervical sagittal balance, and clinical outcomes: an analysis of operative adult cervical deformity patients. J Clin Neurosci. 72:134–141. 2020.


29. Protopsaltis TS, Ramchandran S, Tishelman JC, Smith JS, Neuman BJ, Mundis GM Jr, et al. The importance of C2 slope, a singular marker of cervical deformity, correlates with patient-reported outcomes. Spine (Phila Pa 1976). 45:184–192. 2020.


30. Rao H, Chen Y, Xu W, Zhou Z. Clinical effects of preoperative k-line tilt on patient outcomes after laminoplasty for cervical ossification of the posterior longitudinal ligament. World Neurosurg. 150:e639–e644. 2021.


31. Sakai K, Yoshii T, Arai Y, Hirai T, Torigoe I, Inose H, et al. K-line tilt is a predictor of postoperative kyphotic deformity after laminoplasty for cervical myelopathy caused by ossification of the posterior longitudinal ligament. Global Spine J. 13:1005–1010. 2023.


32. Schwab FJ, Blondel B, Bess S, Hostin R, Shaffrey CI, Smith JS, et al. Radiographical spinopelvic parameters and disability in the setting of adult spinal deformity: a prospective multicenter analysis. Spine (Phila Pa 1976). 38:E803–E812. 2013.
33. Smith JS, Lafage V, Ryan DJ, Shaffrey CI, Schwab FJ, Patel AA, et al. Association of myelopathy scores with cervical sagittal balance and normalized spinal cord volume: analysis of 56 preoperative cases from the aospine north america myelopathy study. Spine (Phila Pa 1976). 38(22 Suppl 1):S161–S170. 2013.
34. Smith JS, Shaffrey CI, Lafage V, Blondel B, Schwab F, Hostin R, et al. Spontaneous improvement of cervical alignment after correction of global sagittal balance following pedicle subtraction osteotomy. J Neurosurg Spine. 17:300–307. 2012.


35. Song K, Su X, Zhang Y, Liu C, Tang X, Zhang G, et al. Optimal chin-brow vertical angle for sagittal visual fields in ankylosing spondylitis kyphosis. Eur Spine J. 25:2596–2604. 2016.


36. Sparrey CJ, Bailey JF, Safaee M, Clark AJ, Lafage V, Schwab F, et al. Etiology of lumbar lordosis and its pathophysiology: a review of the evolution of lumbar lordosis, and the mechanics and biology of lumbar degeneration. Neurosurg Focus. 36:E1. 2014.


37. Suk KS, Kim KT, Lee SH, Kim JM. Significance of chin-brow vertical angle in correction of kyphotic deformity of ankylosing spondylitis patients. Spine (Phila Pa 1976). 28:2001–2005. 2003.


Fig. 1.
Cervical lordosis measurement methods. A : Modified Cobb method. The angle between lines drawn perpendicular to inferior endplates of C2 and C7. B : Jackson physiological stress lines. The angle between lines parallel to the posterior surfaces of the C2 and C7 vertebral bodies. C : Harrison's posterior tangent method, the sum of the angles for the overall cervical curvature is measured by lines parallel to the posterior surfaces of all cervical vertebral bodies from C2 to C7.
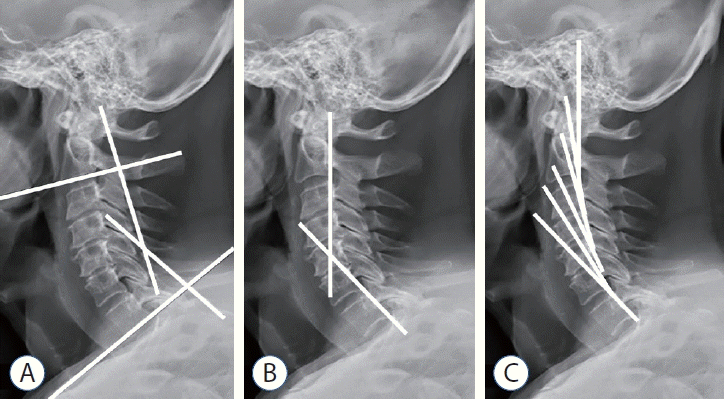
Fig. 2.
Comparison between TIA and PI. Greater TIA or T1S requires more CL, as greater PI requires more lumbar lordosis. TIA : thoracic inlet angle, NT : neck tilt, T1S : T1 slope, PI : pelvic incidence, PT : pelvic tilt, SS : sacral slope.
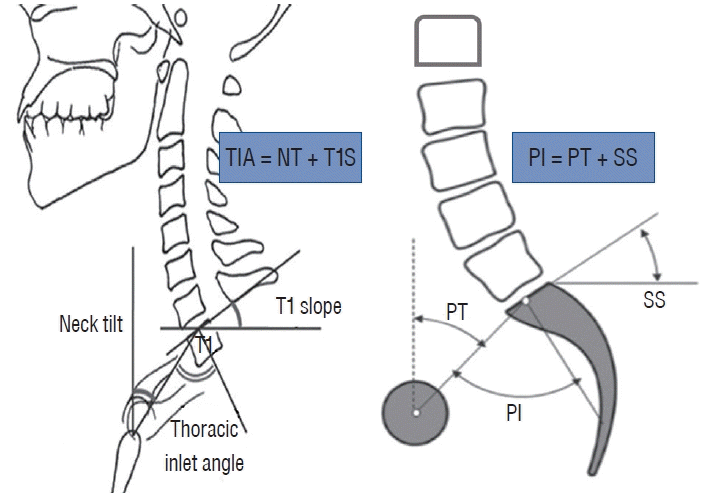