Abstract
Serum creatinine and serum cystatin C are the most widely used renal biomarkers for calculating the estimated glomerular filtration rate (eGFR), which is used to estimate the severity of kidney damage. In this review, we present the basic characteristics of these biomarkers, their advantages and disadvantages, some basic history, and current laboratory measurement practices with state-of-the-art methodology. Their clinical utility is described in terms of normal reference intervals, graphically presented with age-dependent reference intervals, and their use in eGFR equations.
Renal biomarkers such as creatinine and cystatin C (cysC) are relatively small molecules found in the blood. Molecules <40 kDa are filtered from the blood by the kidney glomeruli, whereas albumin and other large molecules >40 kDa are not. Glomeruli are clusters of tiny blood vessels in the kidneys that allow water, dissolved substances, and wastes to pass through their walls while retaining blood cells and larger proteins. The filtrate fluid that passes through the glomerular walls is the so-called “primitive” urine. From this fluid, the kidneys reabsorb cysC, glucose, ions, and many other substances. The remaining fluid and wastes (including creatinine) are carried to the bladder and excreted as urine. The rate at which the blood is filtered is termed the glomerular filtration rate (GFR). A decline in kidney function leads to a decrease in the GFR and to increases in blood creatinine and cysC concentrations, which occur because the kidneys are no longer able to properly filter the blood at a normal rate, resulting in their accumulation in the blood.
Creatinine is a waste product without any known activity in the blood. It is the catabolite of creatine (and phosphocreatine), which originates from the muscles and dietary meat. Creatinine is a low-molecular-weight (113 Da) product of the non-enzymatic conversion of creatine to creatinine directly, or from phosphocreatine to phosphocreatinine followed by dephosphorylation to creatinine, and is generally produced at a fairly constant rate by the muscles. The kidney is the main elimination route; creatinine is largely freely filtered at the glomeruli and not reabsorbed, whereas a small proportion is secreted by the proximal tubules (depending on the GFR level) and a minimal part is excreted extra-renally. Under normal circumstances, the rates of creatinine production and excretion are fairly constant. Thus, healthy kidneys filter creatinine out of the blood via the urine. This is a dynamic process that leads to a dynamic equilibrium, with a constant amount of creatinine in the blood. As such, serum creatinine (SCr) measured by a blood test can inform the physician how well the kidneys are working. When the kidneys are not working well, the SCr concentration increases. SCr concentrations are measured in milligrams per deciliter (mg/dL) or, in the international standard unit, micromoles per L (µmol/L). To convert SCr in µmol/L to mg/dL, it is divided by 88.4.
Most routine SCr assays in current use have evolved from the chromogenic reaction first described by Jaffe in 1886 [1]. Folin was the first to quantify creatinine in biological fluids in 1905 [2]. Over the years, the Jaffe assay has been further developed. At present, SCr is measured mainly by two methods: the Jaffe and enzymatic methods, both of which are colorimetric. In the Jaffe reaction, creatinine forms a yellow-to-red-colored product upon addition of alkaline picrate. After color development, the color intensity is quantified by measuring the optical density. However, picrate can also react with other components known as pseudo-chromogens (Jaffe-like chromogens), such as acetoacetate, pyruvate, substances with a ketone group, proteins, glucose, and ascorbic acid [3]. Jaffe assays are also prone to other complex interactions with bilirubin or certain drugs. Many manufacturers have attempted to improve the performance characteristics of the Jaffe reaction by compensating for these interferences (e.g., rate blanking and subtraction of a fixed factor to compensate for nonspecific reactions) [4]. By subtracting the specific factor, the assay becomes more equivalent with the enzymatic method. However, a certain degree of imprecision remains, and compensation does not work in children and patients who have “non-standard” protein concentrations.
The principle of enzymatic assays was first described in the 1930s, but since 1970, enzymatic assays have been developed to improve creatinine specificity. Enzymatic assays are based on different successive enzymatic reactions. The enzymes used vary according to the manufacturer. The analytical specificity (fewer interferences) and sensitivity of enzymatic assays are better than those of Jaffe assays. The higher cost of the enzymatic SCr assay is the main reason why the Jaffe or compensated Jaffe assays are still used in practice.
For low SCr concentrations, which may occur in young children, the results of the compensated SCr Jaffe assay may turn negative due to overcompensation, but generally, Jaffe SCr is higher than enzymatic SCr. Therefore, enzymatic assays should be favored in pediatric patients as well as in specific situations where Jaffe assays are known to be prone to interference (e.g., bilirubin and ketoacidosis). Notably, enzymatic assays also are subject to interference from bilirubin, albeit to a lesser extent, and from certain drugs. The gain in precision (i.e., a smaller random error) with the enzymatic assays as compared to that of the Jaffe assays is an intrinsic characteristic of the assay and is independent of the standardization procedure, which improves bias (fixed and proportional) or systematic error (for both Jaffe and enzymatic assays). The random error (imprecision) is consistently lower for enzymatic assays (analytical imprecision [CVA] around 2%) than for Jaffe assays (CVA around 5.5%) [3, 4]. This better analytical performance of enzymatic assays will have consequences on the accuracy of GFR-estimating equations, especially in high GFR ranges.
The concept of standardization of creatinine measurements may look simple. The basic idea is that all laboratories calibrate their creatinine assays against a calibration material provided by manufacturers for which the creatinine concentration is traceable to international standards and has been determined with a higher-order method, namely, tandem mass spectrometry coupled with liquid or gas chromatography (LC- or GC-MS/MS, respectively). SCr measurement by MS is accurate and reproducible [3].
Before the introduction of Standard Reference Material (SRM) 967 in 2007 (a commutable international standard provided by the US National Institute of Standards and Technology [NIST]) [5] and the launch of the Creatinine Standardization Program (which obliged manufacturers to align with this standard), there was no standardization of SCr measurements [6]. Since then, most manufacturers have used the NIST standards to calibrate their SCr assays, and since 2017, one can assert that most creatinine assays are correctly calibrated against NIST Standard 967, except maybe some Jaffe assays. Such calibrated methods are generally termed isotope dilution (ID)MS-traceable methods. Since the Creatinine Standardization Program requested manufacturers to standardize their creatinine assays to the SRM, we can theoretically expect that a same sample will produce the same result in any laboratory in the world, regardless of the method (Jaffe or enzymatic) used and the manufacturer [4]. Enzymatic assays have also reached the goal of decreased inter-assay variability and thus have reduced systematic differences between assays [3, 7].
Hoste, et al. [4] demonstrated that the intra-run CV and bias from the target values were higher at low creatinine concentrations. Particularly, Jaffe and enzymatic dry chemistry methods showed higher errors. Depending on the equation used, calculated estimated GFR (eGFR) values corresponding with the reported creatinine concentration ranges resulted in a different GFR classification in 47% of cases [4]. The authors concluded that although most creatinine assays claim to be traceable to the gold standard (ID-GC/MS), large inter-assay differences exist. The inaccuracy in the lower concentration range is of particular concern and may lead to clinical misinterpretation when the creatinine-based eGFR of the patient is used for chronic kidney disease (CKD) staging. Boutten, et al. [8] found that, despite substantial improvements in traceability to the IDMS reference method and precision, compensated Jaffe creatinine assays, in contrast to enzymatic assays, do not achieve the desirable specifications of the National Kidney Disease Educational Program at normal creatinine concentrations. Piéroni, et al. [6] stated that the standardization of creatinine assays resulted in a clear improvement of eGFR in the general population, but alternative methods should be used when creatinine production or metabolism is impaired. Delanaye, et al. [3] questioned whether the added value of using enzymatic SCr assays and/or using combined biomarkers to estimate GFR at the individual level or in a population is sufficient to justify the higher cost of these methods compared to a basic Jaffe creatinine measurement. Further research is required to improve method harmonization.
Measuring SCr is cheap and is part of the routine biochemical blood tests that are commonly requested in daily clinical practice worldwide. However, the clinical interpretation of SCr is not straightforward because the SCr concentration changes with age, sex, muscle mass, nutritional habits, lifestyle, race, tubular secretion, protein intake, and other factors. Therefore, physicians prefer using eGFR, calculated using SCr-based eGFR equations. However, given the inverse relationship between SCr and GFR, variation in SCr will have an inverse impact on the precision of eGFR equations, particularly at low or normal-to-low creatinine concentrations (or in the high or normal-to-high GFR range). As a biomarker, SCr reflects both renal function and muscle mass; therefore, when renal function is normal, SCr-based eGFR correlates less with measured GFR than when renal function is abnormal [9]. Moreover, when GFR levels are very low (e.g., in patients on dialysis with GFR=0), creatinine concentrations only vary because of differences in muscle mass. It is recommended to report SCr with two decimals when expressed in mg/dL and with one decimal when expressed in µmol/L. eGFR results should be reported as whole numbers without decimals [10].
SCr concentrations vary with the amount of muscle mass and, consequently, with population characteristics (such as age, sex, race, nutritional habits, and lifestyle). At the population level, the evolution of SCr with age can be described as follows: SCr of the newborn is the same as that of the mother (~0.70 mg/dL) but rapidly declines to 0.20–0.25 mg/dL within 4–6 weeks thereafter. As the child grows, it gains muscle mass and SCr increases linearly to ~0.60 mg/dL around the age of 14 yrs, and the increase rate is the same in girls and boys. After puberty, the increase rate remains the same in girls but accelerates in boys (due to the extra gain in muscle mass compared to that in girls) and stabilizes at ~0.70 mg/dL in young women and 0.90 mg/dL in young men (18–25 yrs). So-called SCr growth curves have been established for healthy Europeans aged 2–40 yrs [9] but are very similar for Koreans, Chinese, and other ethnicities, although the curves may be (slightly) shifted to lower or higher values in these populations (Fig. 1, left panels). For example, in young healthy African American men, average or median SCr values of 1.03 mg/dL have been reported [11], whereas the average concentrations hardly differ between healthy European American women and African American women (0.70 mg/dL vs. 0.72 mg/dL). After the age of 50–60 yrs, the SCr concentration slightly increases, probably due to normal renal senescence.
SCr median growth curves define the so-called Q values, which can be used to rescale SCr (Fig. 1, right panels). The advantage of rescaling SCr to SCr/Q is that reference intervals for SCr/Q can generally be defined as [0.67; 1.33], independent of age, race, and sex. For example, for adult women, with Q=0.70 mg/dL, the normal values lie between 0.67×0.70=0.47 mg/dL and 1.33×0.70=0.93 mg/dL, and for adult men, with Q=0.90 mg/dL, the reference interval becomes [0.60–1.20 mg/dL]. For boys and girls of 14 yrs of age, the median SCr value (or Q-value) is 0.60 mg/dL, defining a reference interval of 0.60×0.67= 0.40 to 0.60×1.33=0.80. This has been confirmed by large reference interval studies [12, 13]. The advantage of the rescaled SCr/Q value is that the median value equals “1” independent of age, sex, and race. Q values can easily be obtained as the median SCr values from large local hospital databases [12]. Deviation from the median value of “1” is indicative of normal variation as long as SCr/Q remains between 0.67 and 1.33, whereas abnormally high values (SCr/Q>1.33) may be related to reduced kidney function and CKD and SCr/Q<0.67 may be caused by (severely) reduced muscle mass or hyperfiltration.
Although SCr/Q can be used to clinically interpret SCr, nephrologists prefer using eGFR obtained from eGFR equations and expressed in mL/min/1.73m2 because it better reflects the inverse association between GFR and creatinine. SCr-based eGFR equations have been used since the 1970s in pediatric (e.g., the bedside Schwartz equation [14, 15]) and adult populations (e.g., the Cockcroft–Gault equation [16]). SCr/Q can also be applied to eGFR equations, as has been done in the Full Age Spectrum (FAS) equation [17] and the more recent European Kidney Function Consortium (EKFC) equation [9]. The FAS and EKFC equations are designed such that a subject (aged 2–40 yrs) with a median healthy creatinine concentration (corresponding to SCr/Q=1) has median kidney function, corresponding to a GFR value of 107.3 mL/min/1.73m2. This value of 107.3 mL/min/1.73m2 had been established as the median measured GFR in apparently healthy kidney donors [18]. Moreover, the FAS and EKFC equations have the important advantage of covering the full age range, from 2 to 100 yrs, without any discontinuity at the transition between pediatric and adult nephrology care. This avoids implausible increases at the age of 18 yrs, which are frequently observed when changing from the pediatric Schwartz equation to the adult CKD Epidemiology Collaboration equation [19] and the current Kidney Disease: Improving Global Outcomes (KDIGO)-recommended equations [20]. Moreover, the FAS and EKFC equations are age-based, not height-based, equations (e.g., the bedside Schwartz equation), allowing the reporting of eGFR for all ages, along with SCr, by the clinical laboratory. Height is not generally provided by the clinician at the time of blood sampling, making it impossible for laboratories to systematically calculate the eGFR. Based on the relationship between eGFR-EKFC and SCr/Q, symmetrical reference limits based on SCr/Q=1.33 can be calculated as e.g., eGFR-EKFC median± (107.3–107.3/1.331.132)[×0.990(age–40)] and are shown in Fig. 2.
It should be noted that standardization of SCr is essential for accurate use of unbiased eGFR equations when these equations were developed using standardized SCr, as has recently been demonstrated [21]. Recently, it has been shown that the EKFC equation performs very well in populations other than White Europeans, notably in Black populations of Europe, Brazil, and Africa [22] and in the Korean and Chinese populations [23-25].
Alternatively, the clearance of endogenous creatinine can be used as an estimate of GFR, although it overestimates the result (by ~25%) because of proximal renal tubular secretion of creatinine [26]. Creatinine clearance (CrCl) can be calculated as follows: CrCl=(Ucr×V)/Pcr, where Ucr is the urinary creatinine concentration, V is the urinary volume rate in mL/min, and Pcr is the plasma SCr concentration. Creatinine clearance requires 24-hr urine collection, which is difficult to obtain in clinical practice. Therefore, creatinine clearance has a very high imprecision and is currently not recommended to assess GFR. The Cockcroft–Gault equation was developed to predict creatinine clearance (in mL/min) instead of GFR.
CysC, a low-molecular-weight protein (13 kDa) freely filtered by the kidneys, is an established alternative to creatinine for GFR estimation [27]. Unlike creatinine, cysC is fully reabsorbed and catabolized in the proximal renal tubules, with no tubular secretion, in healthy subjects. Under normal conditions, cysC is not found at detectable concentrations in the urine. Serum cysC is relatively constant between 2 and 50 yrs of age but increases more rapidly than SCr thereafter [28]. Increased cysC (beyond age-specific normal levels) is indicative of an underlying kidney problem. Serum cysC is expressed in mg/L with two decimals.
In healthy conditions, cysC is constantly produced by all nucleated cells. However, certain conditions affect the production of cysC. Very large doses of glucocorticoids have been described to increase cysC production, whereas low and medium doses have no effect [29]. Thyroid dysfunction, even when mild, also has a major impact on cysC concentrations [30]. Inflammation has been suggested to affect cysC concentrations, but this is still debated.
As with creatinine, cysC results may vary according to the assay used. The development of automated, rapid, and precise particle-enhanced immunoturbidimetric and immunonephelometric methods has allowed large-scale use of serum cysC as a clinically useful GFR marker. To standardize testing, an international certified reference material, ERM-DA471/IFCC, was developed by The International Federation of Clinical Chemistry and Laboratory Medicine [31]. However, this standard is intended for use in the calibration of immunoassay-based in-vitro diagnostic devices. The certified cysC mass concentration in ERM-DA471/IFCC, if reconstituted according to the specified procedure, is 5.48 mg/L, with the expanded uncertainty being 0.15 mg/L. This concentration is far above the median healthy concentration (~0.83 mg/L) and may therefore not have good accuracy in the normal range. An LC-MS method has been developed but has not been presented as a reference method thus far and is not widely available. The dependence of cysC results upon the analytical method has been a major source of controversy. Before recommending a more widespread use of serum cysC concentrations, it is important to ensure that cysC measurements in clinical laboratories are accurate and that different measurement procedures agree with each other. Disagreement would lead to variation in eGFR values reported by different laboratories, which would be misinterpreted as actual GFR changes.
In 2014, an assessment of laboratories’ performance in the College of American Pathologists Cystatin C Proficiency Testing Program revealed a concerning lack of measurement procedure agreement and substantial bias for some measurement procedures [32]. While a certified reference material for cysC (ERM-DA471/IFCC) is available, there are currently no certified reference measurement procedures for cysC to definitively establish target values for the cysC reference material [33]. However, studies have shown that manufacturers have markedly improved the accuracy and between-method agreement of cysC measurement procedures since 2014, which allows for greater confidence in eGFR relying on cysC [34, 35]. For example, standardization of the Roche Gen2 assay improved the accuracy of cysC measurement compared to that of the Siemens assay [34]. The researchers found negligible method bias and equal performance of both assays in estimating GFR, indicating that successful calibration has led to major progress in cysC analysis. However, despite the improved performance of cysC measurement procedures, the accuracy and between-method agreement of cysC fall well short of those of creatinine, which still is the most widely accepted filtration marker for GFR estimation. Moreover, while at present, all cysC assay kits are traceable to the ERM-DA471/IFCC reference material, ERM-DA471/IFCC-non-traceable kits were still distributed to users of older Siemens platforms, such as the ADVIA Centaur and Siemens Dimension Vista, until the end of 2020 [35]. The old Siemens Cystatin C assay for the ADVIA Chemistry and Atellica CH platforms was taken from the market in 2020 and replaced with Cystatin C_2, which is traceable to ERM-DA471 (Siemens private communication). Harmonization and accuracy of cysC measurement procedures are important to ensure accurate estimates of GFR when using standard equations across different reagent and/or instrument platforms.
With the recent improvements in the accuracy and precision of cysC assays, it may be concluded that cysC is ready for clinical use [36]. KDIGO guidelines recommend the use of eGFR based on cysC in situations where eGFR based on creatinine may be less accurate. Moreover, to reduce over-diagnosis of CKD resulting from inaccurate creatinine-based GFR estimates, UK and international guidelines recommend that cysC-based GFR estimates be used to confirm or exclude the diagnosis in individuals with GFR 45–59 mL/min/1.73m2 and no albuminuria (CKD G3aA1). The 2019 KDIGO Controversies Conference on CKD Screening expert panel concluded that both SCr and cysC are required for initial CKD diagnosis and staging. In line herewith, at least a one-time assessment of cysC-based eGFR may be recommended for patients at high risk of CKD [37]. However, there is still doubt that this additional testing is worth the additional cost [38]. The 10 times higher cost of cysC testing compared to that of SCr testing remains a major disadvantage. However, the concomitant use of SCr and cysC measurements for GFR estimation has been shown to provide greater accuracy than reliance on a single biomarker [28, 39]. In the past year, with the spotlight on issues related to racial injustice in the US, heightened scrutiny of race-based medicine practices has led to criticism of SCr-based eGFR because of the need to specify whether the patient is Black or non-Black for eGFR computation. This has led to calls to discontinue race-based reporting of SCr-based eGFR and a vigorous debate on how to best achieve this goal [20, 40]. One of many options under discussion is the use of the cysC-based eGFR equation, which does not include a race parameter because, in contrast to SCr, cysC is totally independent of race [28, 41-43]. This has important implications for avoiding race-based medicine when estimating GFR, as creatinine-based eGFR has been facing increased criticism for its inclusion of a race parameter. However, certified reference measurement procedures for cysC have to be established to approach the accuracy and between-method agreement achieved for standardized creatinine measurement procedures [35].
Serum cysC has been well established as an early and accurate biomarker of CKD that is particularly helpful in patients for whom creatinine is an inadequate marker or GFR measurement is impractical. Current research questions no longer focus on if but on when and how often cysC should be used in the evaluation of CKD patients. Recent studies are investigating how to manage discrepancies between SCr- and cysC-based eGFR results (see below). However, transitioning all reagents and eGFR equations to the newly established International Standard is critical for developing generalizable data [44]. CysC measurement may be particularly useful in those cases where creatinine measurement is not appropriate, e.g., patients who have liver cirrhosis, are very obese, are malnourished, or have a reduced muscle mass. In healthy children, cysC concentrations may vary according to age (rapid decline immediately after birth to stable values) and slightly differ between boys and girls, and this variability is more pronounced during infancy and puberty. Around the age of 18 yrs, cysC values stabilize at around 0.79 mg/L for women and 0.86 mg/L for men, until the age of 50 yrs. After the age of 50 yrs, cysC concentrations increase more rapidly than creatinine concentrations. Median cysC values in healthy subjects seem independent of muscle mass and much less dependent on population-specific characteristics. Even the difference between men and women is small, which allows GFR estimation from cysC to be independent of race and sex [28, 42, 45].
A Danish study established reference intervals for plasma cysC in women (0.58–1.00 mg/L) and men (0.62–1.04 mg/L) and revealed no sex-related differences; therefore, a common reference interval of 0.61–1.01 mg/L was suggested [46]. In a sub-Saharan population, reference intervals for cysC were 0.57–1.03 mg/L for women and 0.70–1.10 mg/L for men [47]. The Canadian Laboratory Initiative on Pediatric Reference Intervals study established a reference interval of 0.62–1.11 mg/L for 2–19-yr-olds [48]. Given that a median value of 0.83 mg/L was proposed as the race- and sex-independent median cysC-value (Q-value) in the cysC-based EKFC-eGFR equation [28] and by applying the same “rule” as used for SCr to find lower and upper reference limits for cysC, the lower limit was determined to be 0.67×0.83=0.56 mg/L and the upper limit to be 1.33×0.83= 1.10 mg/L. In other words, the reference interval for cysC is independent of age, race, and sex. However, this reference interval is valid only for adolescents and adults aged 18–50 yrs, as beyond the age of 50 yrs, cysC values increase rapidly (probably linked to the normal renal senescence). On the other side of the age spectrum, notably, in children, Ziegelasch, et al. [49] found that cysC concentrations depend on age, sex, and height, particularly during infancy and puberty. They recommended the use of age- and sex-specific reference values for serum cysC concentrations for estimating kidney function in clinical practice. Data from Uppsala, Sweden (unpublished) can only confirm that cysC values increase during puberty in both girls and boys. In addition, it should be noted that small differences can still be found depending on the assay used to determine cysC, which may affect the reference intervals. Overall, the reference interval for cysC is not as age/sex/race-dependent as those for SCr, making clinical interpretation easier.
Because of the relative independency of cysC on age, sex, and race, cysC-based eGFR equations are substantially easier to develop and are consequently also relatively independent of sex and race. This is demonstrated by the recently developed sex- and race-independent EKFC equation [28]. This cysC-based equation has the same mathematical form as the SCr-based EKFC equation, but the rescaled biomarker SCr/Q is replaced by the rescaled biomarker cysC/Q’. The basic idea that SCr/Q=1 corresponds to GFR=107.3 still applies, and consequently, cysC/Q’=1 corresponds to GFR=107.3 mL/min/1.73m2. This also implies that Fig. 2 applies to the cysC-based EKFC equation. Composite equations incorporating both SCr and cysC have been proposed, but the mean of combined single-biomarker equations yields similar results in terms of accuracy and precision. In general, composite SCr/cysC-based eGFR equations and combined single-biomarker eGFR equations yield improved P30 values (+5–10%) (P30 is the percentage of patients with eGFR within 30% of measured GFR) and are less biased than the single-biomarker equations [28, 41, 50].
A possible advantage of cysC is that being a large molecule, its filtration may be restricted, and cysC blood concentrations may rise sooner (i.e., in case of minor glomerular damage) than those of creatinine, as observed in the elderly. CysC is better than creatinine in predicting the risk of cardiovascular disease and mortality [51]. Recently, substantial attention has been paid to discrepant SCr- and cysC-based eGFR results. In some studies, more than one third of patients had discrepant results of more than 15 mL/min/1.73m2 [37, 43, 52]. Cases that highlight such clinical scenarios have been presented by Chen, et al. [37]. Others have suggested that patients with a cysC-based eGFR <60% of the SCr-based eGFR may suffer from “shrunken pore syndrome” [43, 52].
Finally, cysC is a biomarker that can serve as an adjunct or alternative to SCr to evaluate kidney function for drug dosing. The 2012 KDIGO CKD guidelines suggest cysC testing when precision is required for dosing of medications with narrow therapeutic windows. However, many drug dosing guidelines still rely on CrCl thresholds based on the Cockcroft–Gault equation for dosing. However, a systematic review of 28 studies (around 3,500 patients), which evaluated the use of cysC in predicting drug clearance of 16 different medications, including antibiotics and anticoagulants, showed that cysC-based eGFR predicted observed drug clearance and blood concentrations as well as or better than SCr in nearly all studies [53]. Further studies on this important topic are required.
eGFR from SCr, cysC, or both may be unbiased, but precision remains low. However, it is by far the cheapest and fastest method to roughly estimate kidney function. In specific conditions, eGFR from both biomarkers may largely differ, or when a precise GFR measurement is required (e.g., for kidney donation), eGFR from both biomarkers may not even be sufficiently precise. In such cases, GFR measured based on iohexol plasma clearance is the best clinical practice [54].
We can conclude that current eGFR equations based on SCr and/or cysC are at their limits of accuracy and precision and only give rough estimates of the true GFR. Improvements can be made in the calibration of cysC and the harmonization of methods for measuring GFR. The EKFC-eGFR equations have the advantage of covering the full age range, being applicable to both children and adults and avoiding implausible jumps at the transition between pediatric and adult nephrology care. An online EKFC-eGFR calculation tool can be found here (see also the Supplemental Data and Supplemental Data Fig. S1, with an example of the input and output of this calculation tool): https://ekfccalculator.pages.dev.
Supplementary materials can be found via https://doi.org/10.3343/alm.2023.0237
Notes
REFERENCES
1. Jaffe M. 1886; Über den Niederschlag, welchen Pikrinsäure in normalen harn erzeugt und über eine neue Reaction des Kreatinins. Z Physiol Chem. 10:391–400. DOI: 10.1515/bchm1.1886.10.5.391.


2. Folin O. 1905; Approximately complete analyses of thirty "normal" urines. Am J Physiol. 13:45–65. DOI: 10.1152/ajplegacy.1905.13.1.45.


3. Delanaye P, Cavalier E, Pottel H. Serum creatinine: not so simple! Nephron. 2017; 136:302–8. DOI: 10.1159/000469669. PMID: 28441651.


4. Hoste L, Deiteren K, Pottel H, Callewaert N, Martens F. 2015; Routine serum creatinine measurements: how well do we perform? BMC Nephrol. 16:21. DOI: 10.1186/s12882-015-0012-x. PMID: 25803560. PMCID: PMC4358903.


5. Dodder NG, Tai SSC, Sniegoski LT, Zhang NF, Welch MJ. 2007; Certification of creatinine in a human serum reference material by GC-MS and LC-MS. Clin Chem. 53:1694–9. DOI: 10.1373/clinchem.2007.090027. PMID: 17660272.


6. Piéroni L, Bargnoux AS, Cristol JP, Cavalier E, Delanaye P. 2017; Did creatinine standardization give benefits to the evaluation of glomerular filtration rate? EJIFCC. 28:251–7.
7. Piéroni L, Delanaye P, Boutten A, Bargnoux AS, Rozet E, Delatour V, et al. 2011; A multicentric evaluation of IDMS-traceable creatinine enzymatic assays. Clin Chim Acta. 412:2070–5. DOI: 10.1016/j.cca.2011.07.012. PMID: 21803031.


8. Boutten A, Bargnoux AS, Carlier MC, Delanaye P, Rozet E, Delatour V, et al. 2013; Enzymatic but not compensated Jaffe methods reach the desirable specifications of NKDEP at normal levels of creatinine. Results of the French multicentric evaluation. Clin Chim Acta. 419:132–5. DOI: 10.1016/j.cca.2013.01.021. PMID: 23415696.


9. Pottel H, Björk J, Courbebaisse M, Couzi L, Ebert N, Eriksen BO, et al. 2021; Development and validation of a modified full age spectrum creatinine-based equation to estimate glomerular filtration rate: a cross-sectional analysis of pooled data. Ann Intern Med. 174:183–91. DOI: 10.7326/M20-4366. PMID: 33166224.


10. Miller WG, Kaufman HW, Levey AS, Straseski JA, Wilhelms KW, Yu HE, et al. 2022; National Kidney Foundation Laboratory Engagement Working Group recommendations for implementing the CKD-EPI 2021 race-free equations for estimated glomerular filtration rate: practical guidance for clinical laboratories. Clin Chem. 68:511–20. DOI: 10.1093/clinchem/hvab278. PMID: 34918062.


11. Delanaye P, Pottel H, Glassock RJ. 2022; Americentrism in estimation of glomerular filtration rate equations. Kidney Int. 101:856–8. DOI: 10.1016/j.kint.2022.02.022. PMID: 35283173.


12. Pottel H, Vrydags N, Mahieu B, Vandewynckele E, Croes K, Martens F. 2008; Establishing age/sex related serum creatinine reference intervals from hospital laboratory data based on different statistical methods. Clin Chim Acta. 396:49–55. DOI: 10.1016/j.cca.2008.06.017. PMID: 18621041.


13. Ceriotti F, Boyd JC, Klein G, Henny J, Queraltó J, Kairisto V, et al. 2008; Reference intervals for serum creatinine concentrations: assessment of available data for global application. Clin Chem. 54:559–66. DOI: 10.1373/clinchem.2007.099648. PMID: 18202155.


14. Schwartz GJ, Haycock GB, Edelmann CM, Spitzer A. 1976; A simple estimate of glomerular filtration rate in children derived from body length and plasma creatinine. Pediatrics. 58:259–63. DOI: 10.1542/peds.58.2.259. PMID: 951142.


15. Schwartz GJ, Muñoz A, Schneider MF, Mak RH, Kaskel F, Warady BA, et al. 2009; New equations to estimate GFR in children with CKD. J Am Soc Nephrol. 20:629–37. DOI: 10.1681/ASN.2008030287. PMID: 19158356. PMCID: PMC2653687.


16. Cockcroft DW, Gault MH. 1976; Prediction of creatinine clearance from serum creatinine. Nephron. 16:31–41. DOI: 10.1159/000180580. PMID: 1244564.


17. Pottel H, Hoste L, Dubourg L, Ebert N, Schaeffner E, Eriksen BO, et al. 2016; An estimated glomerular filtration rate equation for the full age spectrum. Nephrol Dial Transplant. 31:798–806. DOI: 10.1093/ndt/gfv454. PMID: 26932693. PMCID: PMC4848755.


18. Pottel H, Hoste L, Yayo E, Delanaye P. 2017; Glomerular filtration rate in healthy living potential kidney donors: a meta-analysis supporting the construction of the full age spectrum equation. Nephron. 135:105–19. DOI: 10.1159/000450893. PMID: 27764827.


19. Pottel H, Björk J, Bökenkamp A, Berg U, Åsling-Monemi K, Selistre L, et al. 2019; Estimating glomerular filtration rate at the transition from pediatric to adult care. Kidney Int. 95:1234–43. DOI: 10.1016/j.kint.2018.12.020. PMID: 30922665.


20. Inker LA, Eneanya ND, Coresh J, Tighiouart H, Wang D, Sang Y, et al. 2021; New creatinine- and cystatin C-based equations to estimate GFR without race. N Engl J Med. 385:1737–49. DOI: 10.1056/NEJMoa2102953. PMID: 34554658. PMCID: PMC8822996.


21. Pottel H, Cavalier E, Björk J, Nyman U, Grubb A, Ebert N, et al. 2022; Standardization of serum creatinine is essential for accurate use of unbiased estimated GFR equations: evidence from three cohorts matched on renal function. Clin Kidney J. 15:2258–65. DOI: 10.1093/ckj/sfac182. PMID: 36381377. PMCID: PMC9664577.


22. Delanaye P, Vidal-Petiot E, Björk J, Ebert N, Eriksen BO, Dubourg L, et al. 2023; Performance of creatinine-based equations to estimate glomerular filtration rate in White and Black populations in Europe, Brazil and Africa. Nephrol Dial Transplant. 38:106–18. DOI: 10.1093/ndt/gfac241. PMID: 36002032.


23. Kim H, Hur M, Lee S, Lee GH, Moon HW, Yun YM. 2022; European Kidney Function Consortium Equation vs. Chronic Kidney Disease Epidemiology Collaboration (CKD-EPI) refit equations for estimating glomerular filtration rate: comparison with CKD-EPI equations in the Korean population. J Clin Med. 11:4323. DOI: 10.3390/jcm11154323. PMID: 35893414. PMCID: PMC9331398.


24. Jeong TD, Hong J, Lee W, Chun S, Min WK. 2023; Accuracy of the new creatinine-based equations for estimating glomerular filtration rate in Koreans. Ann Lab Med. 43:244–52. DOI: 10.3343/alm.2023.43.3.244. PMID: 36544336. PMCID: PMC9791020.


25. Zhao L, Li HL, Liu HJ, Ma J, Liu W, Huang JM, et al. 2023; Validation of the EKFC equation for glomerular filtration rate estimation and comparison with the Asian-modified CKD-EPI equation in Chinese chronic kidney disease patients in an external study. Ren Fail. 45:2150217. DOI: 10.1080/0886022X.2022.2150217. PMID: 36632770. PMCID: PMC9848359.


26. Soveri I, Berg UB, Björk J, Elinder CG, Grubb A, Mejare I, et al. 2014; Measuring GFR: a systematic review. Am J Kidney Dis. 64:411–24. DOI: 10.1053/j.ajkd.2014.04.010. PMID: 24840668.


27. Grubb A, Simonsen O, Sturfelt G, Truedsson L, Thysell H. 1985; Serum concentration of cystatin C, factor D and beta 2-microglobulin as a measure of glomerular filtration rate. Acta Med Scand. 218:499–503. DOI: 10.1111/j.0954-6820.1985.tb08880.x. PMID: 3911736.


28. Pottel H, Björk J, Rule AD, Ebert N, Eriksen BO, Dubourg L, et al. 2023; Cystatin C-based equation to estimate GFR without the inclusion of race and sex. N Engl J Med. 388:333–43. DOI: 10.1056/NEJMoa2203769. PMID: 36720134.


29. Risch L, Huber AR. 2002; Glucocorticoids and increased serum cystatin C concentrations. Clin Chim Acta. 320:133–4. DOI: 10.1016/S0009-8981(02)00044-X. PMID: 11983211.


30. Filler G, Bökenkamp A, Hofmann W, Le Bricon T, Martínez-Brú C, Grubb A. 2005; Cystatin C as a marker of GFR-history, indications, and future research. Clin Biochem. 38:1–8. DOI: 10.1016/j.clinbiochem.2004.09.025. PMID: 15607309.


31. Grubb A, Blirup-Jensen S, Lindström V, Schmidt C, Althaus H, Zegers I, et al. 2010; First certified reference material for cystatin C in human serum ERM-DA471/IFCC. Clin Chem Lab Med. 48:1619–21. DOI: 10.1515/CCLM.2010.318. PMID: 21034257.


32. Eckfeldt JH, Karger AB, Miller WG, Rynders GP, Inker LA. 2015; Performance in measurement of serum cystatin C by laboratories participating in the College of American Pathologists 2014 CYS Survey. Arch Pathol Lab Med. 139:888–93. DOI: 10.5858/arpa.2014-0427-CP. PMID: 25884370.


33. Bargnoux AS, Piéroni L, Cristol JP, Kuster N, Delanaye P, Carlier MC, et al. 2017; Multicenter evaluation of cystatin C measurement after assay standardization. Clin Chem. 63:833–41. DOI: 10.1373/clinchem.2016.264325. PMID: 28188233.


34. Ebert N, Delanaye P, Shlipak M, Jakob O, Martus P, Bartel J, et al. 2016; Cystatin C standardization decreases assay variation and improves assessment of glomerular filtration rate. Clin Chim Acta. 456:115–21. DOI: 10.1016/j.cca.2016.03.002. PMID: 26947968.


35. Karger AB, Long T, Inker LA, Eckfeldt JH. College of American Pathologists Accuracy Based Committee and Chemistry Resource Committee. 2022; Improved performance in measurement of serum cystatin C by laboratories participating in the College of American Pathologists 2019 CYS survey. Arch Pathol Lab Med. 146:1218–23. DOI: 10.5858/arpa.2021-0306-CP. PMID: 35192685.


36. Ebert N, Shlipak MG. 2020; Cystatin C is ready for clinical use. Curr Opin Nephrol Hypertens. 29:591–8. DOI: 10.1097/MNH.0000000000000638. PMID: 32868529.


37. Chen DC, Potok OA, Rifkin D, Estrella MM. 2022; Advantages, limitations, and clinical considerations in using cystatin C to estimate GFR. Kidney360. 3:1807–14. DOI: 10.34067/KID.0003202022. PMID: 36514729. PMCID: PMC9717651.


38. Shardlow A, McIntyre NJ, Fraser SDS, Roderick P, Raftery J, Fluck RJ, et al. 2017; The clinical utility and cost impact of cystatin C measurement in the diagnosis and management of chronic kidney disease: a primary care cohort study. PLoS Med. 14:e1002400. DOI: 10.1371/journal.pmed.1002400. PMID: 29016597. PMCID: PMC5634538.


39. Pottel H, Delanaye P, Schaeffner E, Dubourg L, Eriksen BO, Melsom T, et al. 2017; Estimating glomerular filtration rate for the full age spectrum from serum creatinine and cystatin C. Nephrol Dial Transplant. 32:497–507. DOI: 10.1093/ndt/gfw425. PMID: 28089986. PMCID: PMC5837496.


40. Delgado C, Baweja M, Burrows NR, Crews DC, Eneanya ND, Gadegbeku CA, et al. 2021; Reassessing the inclusion of race in diagnosing kidney diseases: an interim report from the NKF-ASN task force. Am J Kidney Dis. 78:103–15. DOI: 10.1053/j.ajkd.2021.03.008. PMID: 33845065. PMCID: PMC8238889.


41. Inker LA, Schmid CH, Tighiouart H, Eckfeldt JH, Feldman HI, Greene T, et al. 2012; Estimating glomerular filtration rate from serum creatinine and cystatin C. N Engl J Med. 367:20–9. DOI: 10.1056/NEJMoa1114248. PMID: 22762315. PMCID: PMC4398023.


42. Ottosson Frost C, Gille-Johnson P, Blomstrand E, St-Aubin V, Leion F, Grubb A. 2022; Cystatin C-based equations for estimating glomerular filtration rate do not require race or sex coefficients. Scand J Clin Lab Invest. 82:162–6. DOI: 10.1080/00365513.2022.2031279. PMID: 35107398.


43. Malmgren L, Öberg C, den Bakker E, Leion F, Siódmiak J, Åkesson A, et al. 2023; The complexity of kidney disease and diagnosing it - cystatin C, selective glomerular hypofiltration syndromes and proteome regulation. J Intern Med. 293:293–308. DOI: 10.1111/joim.13589. PMID: 36385445. PMCID: PMC10107454.


44. Benoit SW, Ciccia EA, Devarajan P. 2020; Cystatin C as a biomarker of chronic kidney disease: latest developments. Expert Rev Mol Diagn. 20:1019–26. DOI: 10.1080/14737159.2020.1768849. PMID: 32450046. PMCID: PMC7657956.


45. Grubb A, Horio M, Hansson LO, Björk J, Nyman U, Flodin M, et al. 2014; Generation of a new cystatin C-based estimating equation for glomerular filtration rate by use of 7 assays standardized to the international calibrator. Clin Chem. 60:974–86. DOI: 10.1373/clinchem.2013.220707. PMID: 24829272.


46. Erlandsen EJ, Randers E. 2018; Reference intervals for plasma cystatin C and plasma creatinine in adults using methods traceable to international calibrators and reference methods. J Clin Lab Anal. 32:e22433. DOI: 10.1002/jcla.22433. PMID: 29573343. PMCID: PMC6817201.


47. Edinga-Melenge BE, Yakam AT, Nansseu JR, Bilong C, Belinga S, Minkala E, et al. 2019; Reference intervals for serum cystatin C and serum creatinine in an adult sub-Saharan African population. BMC Clin Pathol. 19:4. DOI: 10.1186/s12907-019-0086-7. PMID: 30923459. PMCID: PMC6423796.


48. Adeli K, Higgins V, Trajcevski K, White-Al Habeeb N. 2017; The Canadian laboratory initiative on pediatric reference intervals: a CALIPER white paper. Crit Rev Clin Lab Sci. 54:358–413. DOI: 10.1080/10408363.2017.1379945. PMID: 29017389.


49. Ziegelasch N, Vogel M, Müller E, Tremel N, Jurkutat A, Löffler M, et al. 2019; Cystatin C serum levels in healthy children are related to age, gender, and pubertal stage. Pediatr Nephrol. 34:449–57. DOI: 10.1007/s00467-018-4087-z. PMID: 30460495. PMCID: PMC6349798.


50. Grubb A, Nyman U, Björk J. 2012; Improved estimation of glomerular filtration rate (GFR) by comparison of eGFRcystatin C and eGFRcreatinine. Scand J Clin Lab Invest. 72:73–7. DOI: 10.3109/00365513.2011.634023. PMID: 22121923. PMCID: PMC3279136.
51. Shlipak MG, Matsushita K, Ärnlöv J, Inker LA, Katz R, Polkinghorne KR, et al. 2013; Cystatin C versus creatinine in determining risk based on kidney function. N Engl J Med. 369:932–43. DOI: 10.1056/NEJMoa1214234. PMID: 24004120. PMCID: PMC3993094.


52. Grubb A, Lindström V, Jonsson M, Bäck SE, Åhlund T, Rippe B, et al. Reduction in glomerular pore size is not restricted to pregnant women. Evidence for a new syndrome: "shrunken pore syndrome.". Scand J Clin Lab Invest. 2015; 75:333–40. DOI: 10.3109/00365513.2015.1025427. PMID: 25919022. PMCID: PMC4487590.


53. Teaford HR, Barreto JN, Vollmer KJ, Rule AD, Barreto EF. 2020; Cystatin C: a primer for pharmacists. Pharmacy (Basel). 8:35. DOI: 10.3390/pharmacy8010035. PMID: 32182861. PMCID: PMC7151673.


54. Agarwal R, Delanaye P. 2019; Glomerular filtration rate: when to measure and in which patients? Nephrol Dial Transplant. 34:2001–7. DOI: 10.1093/ndt/gfy363. PMID: 30520986.


Fig. 1
Median SCr versus age (black curves) and reference limits (red curves) in healthy women and men [12] (left panels) converted to rescaled SCr/Q (right panels). The dots are the actual data from Pottel, et al. [12]. The median SCr concentration in the left panels corresponds to SCr/Q=1 in the right panels. The lower and upper limits in the left panels correspond to SCr/Q=0.67 and SCr/Q=1.33, respectively, in the right panels.
Abbreviations: SCr, serum creatinine; SCr/Q, serum creatinine-to-Q value ratio.
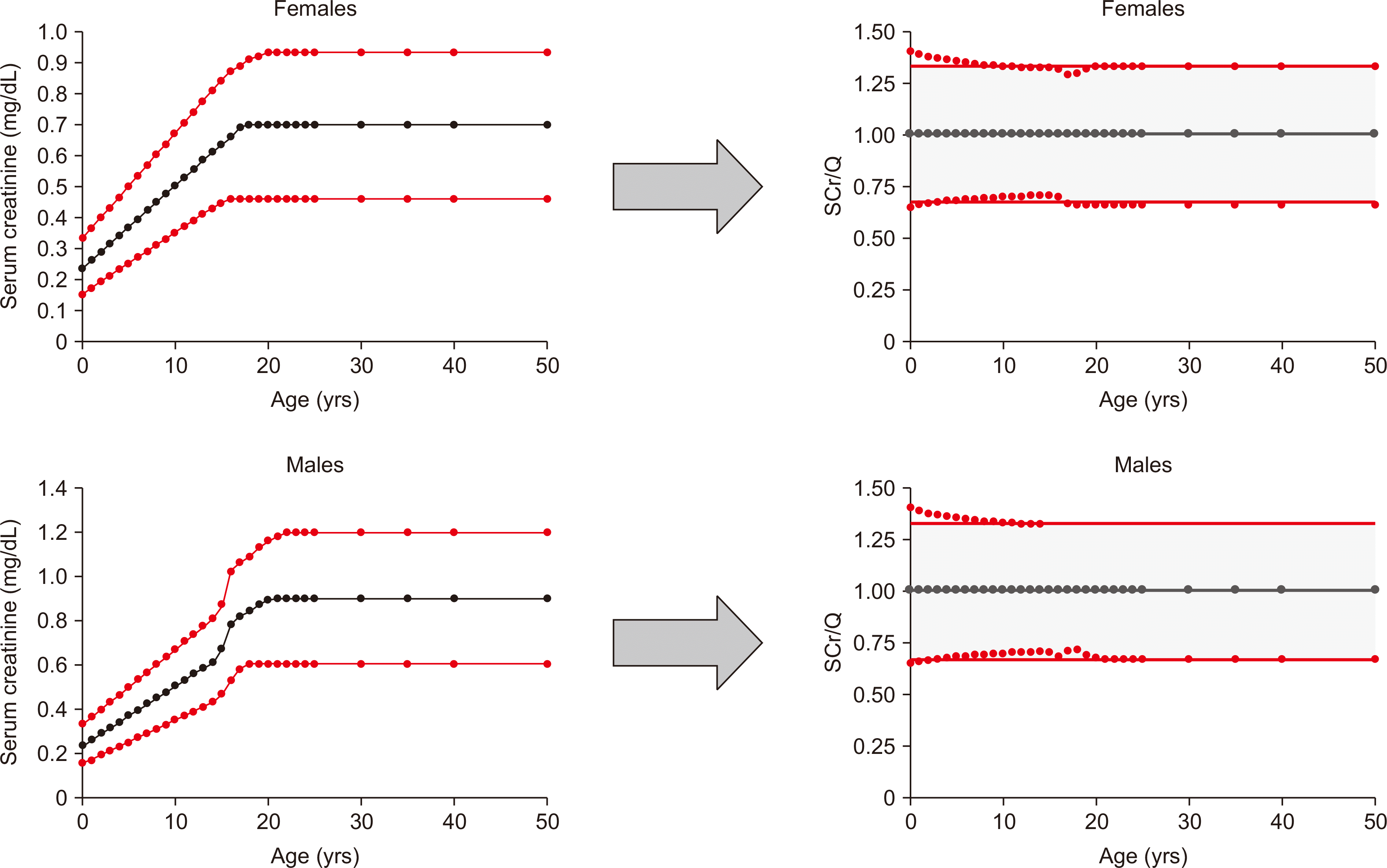