Abstract
Objective
Methods
Results
Notes
AUTHOR CONTRIBUTIONS
Conceptualization: YZ, HA. Data curation: SHC. Formal analysis: SHC, Min-Soo K. Investigation: YZ, Min-Seok K. Min-Soo K. Methodology: YZ, HA, JHC. Project administration: JHC. Resources: HA, MHO. Supervision: JHC. Validation: Min-Seok K. Visualization: MHO. Writing–original draft: YZ. Writing–review & editing: HA, JHC.
REFERENCES



























Figure 1
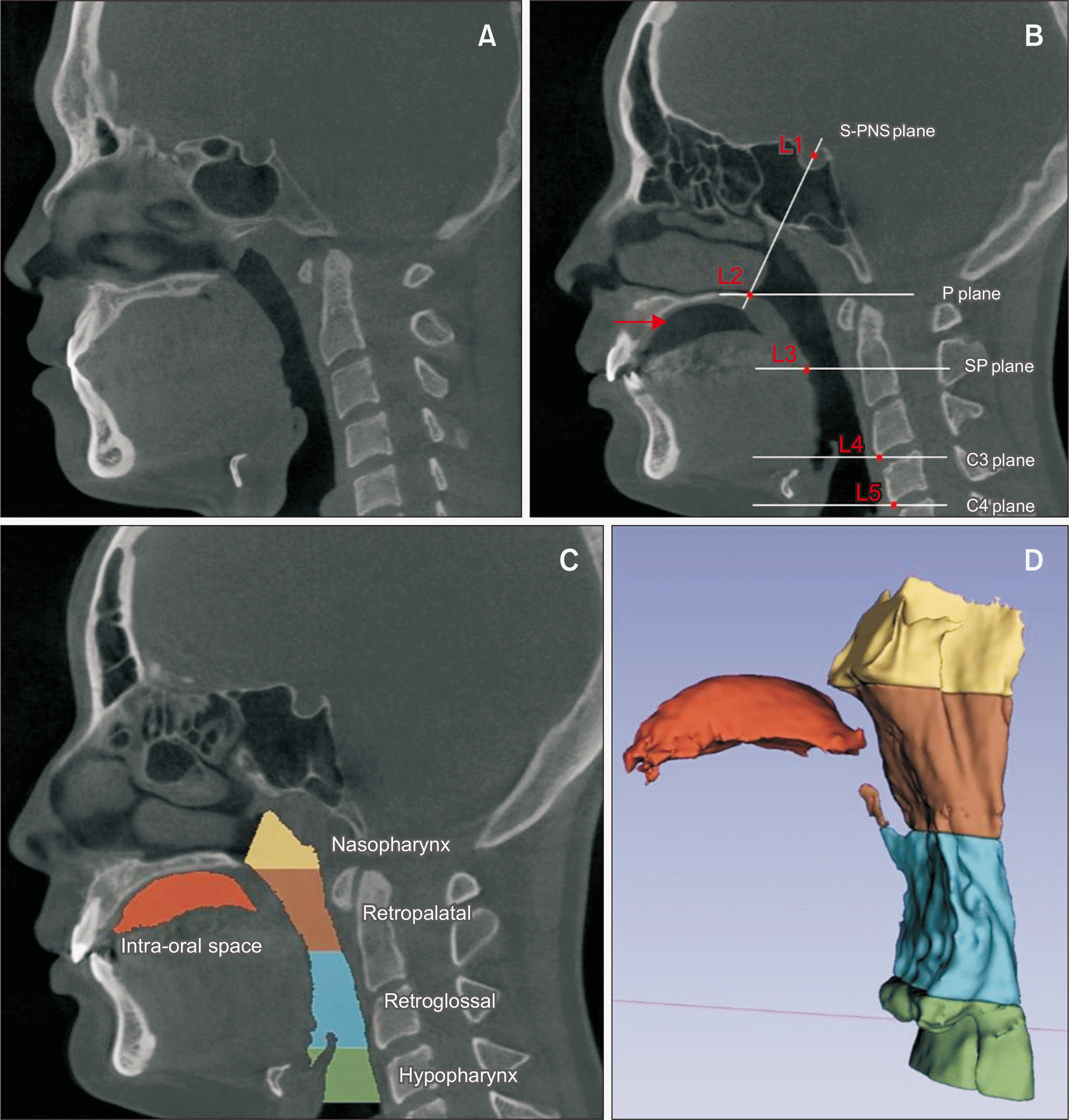
Figure 2
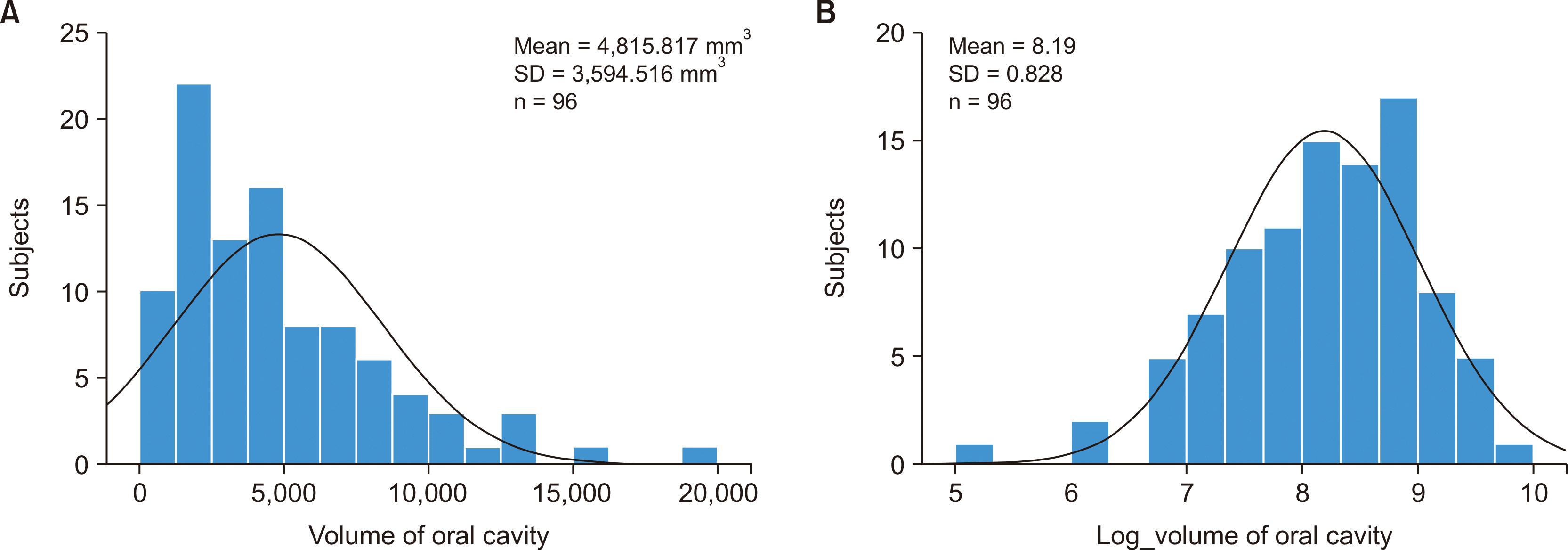
Figure 3
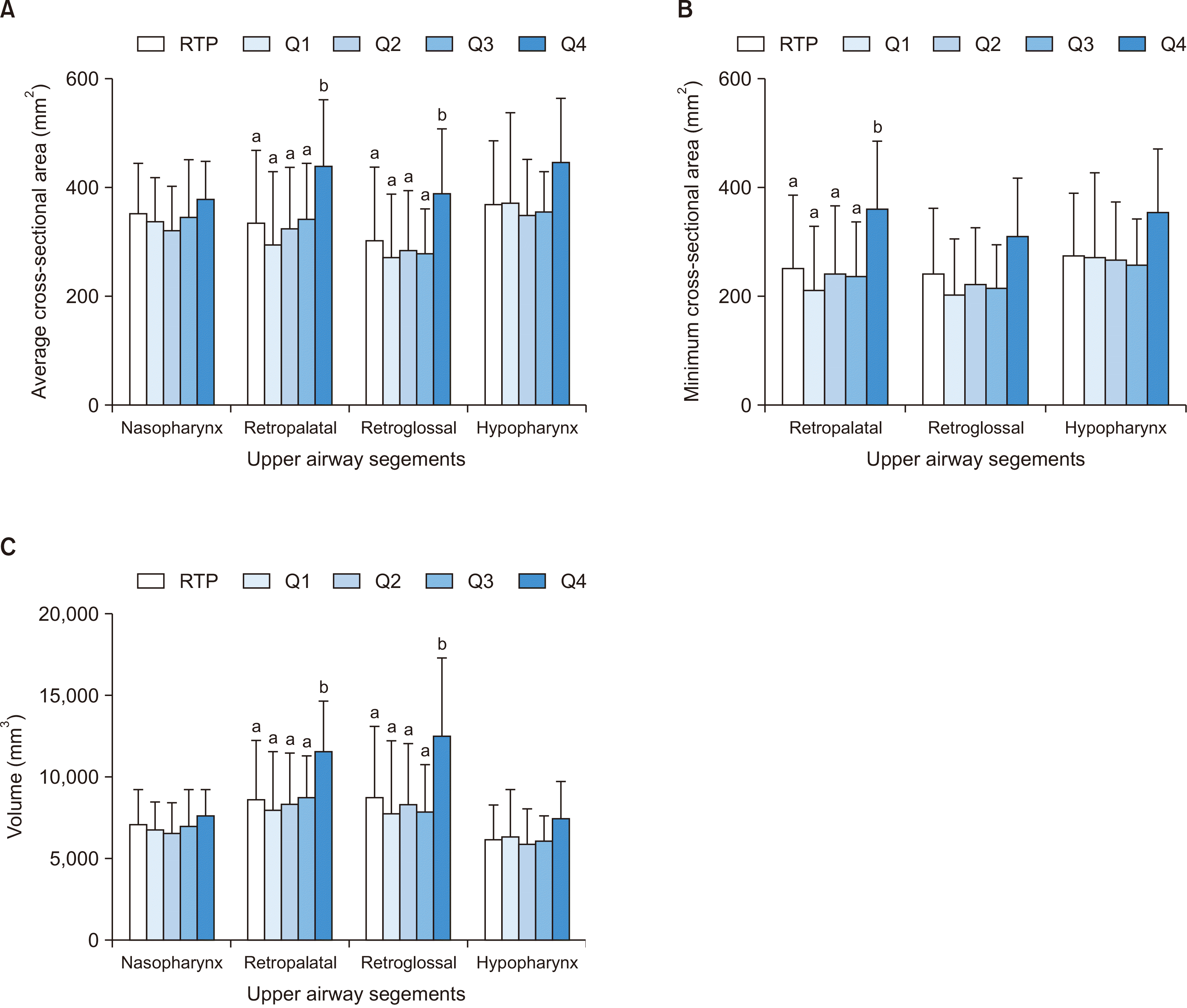
Table 1
Table 2
Overall | RTP group (n = 98) | LTP group (n = 96) | P value |
---|---|---|---|
Age | 0.197* | ||
Mean ± SD | 25.6 ± 9.1 | 24.1 ± 6.9 | |
Min–Max | 18–58 | 18–48 | |
Median | 38 | 33 | |
95% CI | 23.8–27.5 | 22.7–25.5 | |
Sex (%) | 0.253† | ||
Male | 42 (42.9) | 49 (51.0) | |
Female | 56 (57.1) | 47 (49.0) | |
ANB (°) | 0.138* | ||
Mean ± SD | 2.0 ± 3.7 | 1.2 ± 3.5 | |
Min–Max | −6 to 10 | −6.5 to 8 | |
95% CI | 1.3–2.7 | 0.5–1.9 | |
SN-MP (°) | 0.975* | ||
Mean ± SD | 35.7 ± 5.7 | 35.7 ± 6.7 | |
Min–Max | 38.5–50 | 21–51 | |
95% CI | 34.4–36.8 | 34.3–37.0 | |
ODI | 0.168* | ||
Mean ± SD | 70.3 ± 9.8 | 68.3 ± 10.1 | |
Min–Max | 43–92 | 29–98 | |
95% CI | 68.3–72.3 | 66.3–70.3 | |
PP-MP (°) | 0.905* | ||
Mean ± SD | 25.1 ± 5.6 | 25.2 ± 6.0 | |
Min–Max | 6–38 | 7–38 | |
95% CI | 24.0–26.4 | 24.0–26.5 | |
FMA (°) | 0.741* | ||
Mean ± SD | 26.8 ± 5.1 | 27.0 ± 5.9 | |
Min–Max | 10–40 | 12–39.5 | |
95% CI | 25.7–27.8 | 25.8–28.2 |
RTP, resting tongue position; LTP, low tongue position; SD, standard deviation; Min, minimum; Max, maximum; CI, confidence intervals; ANB, A point-nasion-B point angle; SN-MP, sella-nasion plane to mandibular plane angle; ODI, overbite depth indicator; PP-MP, palatal plane to mandibular plane angle; FMA, Frankfort-mandibular plane angle.
Table 3
Table 4
Measurements |
RTP group (n = 98) |
LTP group (n = 96) | Mean (95% CI) | P value | |||
---|---|---|---|---|---|---|---|
Q1 (n = 15) | Q2 (n = 27) | Q3 (n = 41) | Q4 (n = 13) | ||||
ACA (mm2) | |||||||
Nasopharynx | 350.9 ± 92.2 | 337.4 ± 81.2 | 323.1 ± 78.8 | 346.2 ± 102.7 | 378.4 ± 69.3 | 346.8 (334.0–359.7) | 0.440 |
Retropalatal | 334.3 ± 133.1a | 297.1 ± 131.7a | 325.9 ± 111.9a | 342.0 ± 102.4a | 438.6 ± 123.3b | 338.9 (321.1–356.7) | 0.034* |
Retroglossal | 302.1 ± 135.1a | 273.1 ± 114.6a | 284.4 ± 110.6a | 278.1 ± 82.0a | 389.1 ± 118.6b | 298.2 (281.0–315.4) | 0.048* |
Hypopharynx | 369.4 ± 116.1 | 371.1 ± 165.4 | 348.9 ± 102.8 | 355.5 ± 73.5 | 445.9 ± 118.4 | 368.9 (352.9–384.8) | 0.110 |
MCA (mm2) | |||||||
Retropalatal | 254.2 ± 133.4a | 212.8 ± 116.8a | 244.6 ± 122.8a | 240.2 ± 97.9a | 363.1 ± 122.3b | 254.0 (236.2–271.9) | 0.016* |
Retroglossal | 243.1 ± 120.4 | 205.9 ± 101.0 | 224.9 ± 102.6 | 216.6 ± 79.1 | 312.1 ± 106.8 | 236.7 (221.2–252.2) | 0.051 |
Hypopharynx | 276.3 ± 114.2 | 274.4 ± 153.8 | 269.0 ± 105.1 | 259.7 ± 84.5 | 356.8 ± 116.8 | 277.0 (261.1–292.9) | 0.101 |
Volume (mm3) | |||||||
Nasopharynx | 7,097.9 ± 2,173.0 | 6,787.9 ± 1,704.1 | 6,622.9 ± 1,826.4 | 7,001.5 ± 2,270.0 | 7,716.5 ± 1,526.1 | 7,028.9 (6,735.0–7,322.8) | 0.600 |
Retropalatal | 8,607.4 ± 3,640.5a | 8,017.7 ± 3,554.3a | 8,388.2 ± 3,082.8a | 8,799.2 ± 2,536.8a | 11,563.9 ± 3,098.6b | 8,769.9 (8,292.0–9,247.9) | 0.035* |
Retroglossal | 8,777.7 ± 4,342.8a | 7,815.2 ± 4,420.3a | 8,350.0 ± 3,704.2a | 7,880.0 ± 2,915.8a | 12,585.0 ± 4,736.2b | 8,709.2 (8,122.9–9,295.5) | 0.006** |
Hypopharynx | 6,203.6 ± 2,120.7 | 6,402.9 ± 2,828.1 | 5,895.1 ± 2,199.0 | 6,117.7 ± 1,547.1 | 7,522.0 ± 2,228.4 | 6,246.3 (5,948.2–6,544.3) | 0.214 |
Table 5
P value | R2 | |
---|---|---|
ACA (mm2) | ||
Nasopharynx | 0.133 | 0.129 |
Retropalatal | 0.004 | 0.220 |
Retroglossal | < 0.001 | 0.379 |
Hypopharynx | 0.001 | 0.260 |
MCA (mm2) | ||
Retropalatal | < 0.001 | 0.303 |
Retroglossal | < 0.001 | 0.400 |
Hypopharynx | < 0.001 | 0.307 |
Volume (mm3) | ||
Nasopharynx | 0.737 | 0.056 |
Retropalatal | 0.004 | 0.224 |
Retroglossal | < 0.001 | 0.375 |
Hypopharynx | < 0.001 | 0.399 |
ACA, average cross-sectional area; MCA, minimum cross-sectional area; R2, coefficient of determination.
See Table 2 for definitions of measurement.