Abstract
Objective
To evaluate the effects of an electric toothbrush with vibrational frequencies of 125 Hz and 150 Hz on the orthodontic tooth movement (OTM) rate and the production of prostaglandin E2 (PGE2).
Methods
Out of thirty patients (aged 18–25 years; 16 females and 14 males), ten patients each formed Group A and B, who used electric toothbrushes with 125 Hz and 150 Hz vibrations, respectively. The remaining ten patients (Group C) served as the control group and did not use electric toothbrushes. The rate of OTM and levels of PGE2 using microcapillary pipettes were calculated before the start of retraction (T0), on the 30th day (T1), on the 60th day (T2), and on the 90th day (T3) from the start of retraction in all the groups.
Results
There was a statistically significant difference in the mean OTM values and PGE2 levels in all three groups at different time intervals, with the maximum difference seen in Group B compared to Group A and least in Group C at T1, T2 and T3.
Conclusions
The rate of OTM and levels of PGE2 were highest in patients who used an electric toothbrush with 150 Hz mechanical vibration compared to those who used an electric toothbrush with 125 Hz mechanical vibration and least in patients who did not use an electric toothbrush. Mechanical vibration led to an increase in the PGE2 levels and accelerated the OTM.
Accelerating tooth movement can shorten the duration of orthodontic therapy. The mechanical stimulation brought on by orthodontic appliances results in bone remodeling and periodontal tissue adaptability. Changes are made to the hemotactic situation before the tooth movement eventually speeds up.1 Physical trauma (piezopuncture, electric currents, resonant vibration, alveolar corticotomy, lasers, etc.) and pharmacological administration have attempted to accelerate the tooth movement (corticosteroids, vitamin D3, and prostaglandins).2 Every previously employed method had drawbacks such as localized discomfort, decalcification, root resorption, and other medication side effects.3 A surgically noninvasive procedure to hasten tooth movement is required due to the negative effects of all previously tried treatments. Increased orthodontic tooth movement (OTM) rate has been seen in mechanical vibration. There have not been many studies on mechanical vibration speeding up tooth movement in animals.4 Without harming the periodontal tissues, mechanical vibrations of low magnitude and higher frequency have been shown to accelerate OTM.5
Earlier means of assessing accelerated tooth movements were measurements made on either physical models made before and after tooth movements or directly intraorally. The disadvantage of these methods was the failure to subtract the components of anchor loss through the mesial movement of posteriors. Hence a more accurate method will be to assess the biomarkers like prostaglandin E2 (PGE2) to measure accelerated tooth movements.
Periodontal cells produce biologically active substances when an orthodontic force is applied, which causes the connective tissues to remodel, osteoclasts to develop, and as a result causes tooth movement.6 Orthodontic tooth movement triggers an inflammatory response that results in the release of several cytokines, including osteoprotegerin, matrix metalloproteinases, tumor necrosis factor, lactate dehydrogenase, etc.7 A proinflammatory mediator known as PGE2 is produced by the cells of periodontal ligaments. This biochemical mediator stimulates osteoclastic activity, which occurs during OTM.
This biomarker is identified as a stimulator of the resorption of bone. In vitro studies and studies on animal models have been used to assess the role of PGE2 on OTM during orthodontic treatment.8
The molecules involved in the bone modeling and remodeling process can be detected in gingival crevicular fluid (GCF).9,10 GCF is the exudate reflecting the biological events in the periodontal tissues and helps in detecting levels of certain biomarkers.11
Though substantial literature has been published regarding the relation of vibration to accelerated tooth movement, limited information is available on the low magnitude and higher frequency mechanical vibratory effects on the OTM and PGE2 production rate. Hence the present study was undertaken to evaluate the low magnitude and higher frequency mechanical vibratory effects on the rate of OTM and production of PGE2 in GCF.
All participants were chosen from the outpatient Department of Orthodontics and Dentofacial Orthopedics at our institution after receiving written informed consent from each participant and distributing a patient information sheet outlining the study’s objectives. Ethical committee approval was received from the Ethics Committee of Sri Rajiv Gandhi College of Dental Sciences and Hospital Bangalore (approval no: SRGCDS/2019/635).
The overall sample size was calculated using the G*Power analysis program to be 30, taking into account the effect size to be tested at 0.25 and the power of the study at 80% (version 3.0.1, Henrich-Heine-Universitat, Dusseldorf, Germany). A total of 30 patients formed the sample through simple randomization, with ages ranging from 18 to 25 years (16 females and 14 males). Our study comprised healthy patients who needed orthodontic treatment and required permanent first maxillary premolar extractions as part of orthodontic treatment. Patients with healthy periodontal conditions, gingival index (GI) scores of 1 or less, no pockets, generalized depths of probing greater than 3 mm, and no radiographic evidence of bone loss were included in our study. They also had not used any anti-inflammatory drugs in the previous month. Patients who smoked or had any systemic condition were not allowed to participate in the trial.
Thirty patients in total were enrolled in the trial. Two subgroups made up the experimental group. Ten patients made up Group A, who were instructed to use an electric toothbrush with a 125 Hz vibration, and ten patients in Group B, who were instructed to use an electric toothbrush with a 150 Hz vibration. The ten patients that were left comprised Group C, which served as the control group and did not use an electric toothbrush.
The patient was informed of the experimental process, and their consent was acquired. GI measurements were taken at each visit, and individuals with a GI of 1 were deemed to have satisfactory oral health.
Each patient was bonded after extracting the permanent first maxillary premolars; the patient received a pre-adjusted edgewise appliance with a 0.022 × 0.025-inch slot MBT prescription (3M Gemini metal brackets; 3M Unitek Corp., Monrovia, CA, USA). The first maxillary permanent molars received molar bands. To level and align the maxillary arch initially, a 0.016 inch NiTi archwire was inserted. En masse retraction of the anterior consolidated section, consisting of six anterior teeth, was carried out utilizing friction mechanics and an elastomeric chain after reaching 19 × 25 SS wire (Alastik; 3M Unitek Corp.). The elastomeric chain pulled the anterior segment back with an average force of 200 g.6
One day after the start of retraction, patients in the experimental and control groups were recommended to use the electric toothbrush we developed with vibration frequencies of 125 Hz and 150 Hz (Figure 1). Following the usual orthodontic cleaning of all the teeth where the patients were instructed to hold the electric toothbrush at an angle of 45° to the gum line and rotate it in a circular motion with very gentle pressure, the patients were then instructed to use a specially designed electric toothbrush on the labial and palatal surfaces of all six anterior teeth for six minutes once a day. They used the electric toothbrush for 1 minute for each tooth. Patients were instructed to stop using it and report right away if they experienced any discomfort.
A palatal plug was prepared on the maxillary cast with the help of acrylic.12 This plug was placed on the medial area of the rugae with 0.7 mm SS wire embedded in it. The wire was passed to the distal surfaces of both the maxillary canines, as shown in Figure 2. The first three palatal rugae were marked over the acrylic plug on the initial model as a reference landmark. The plug was then transferred to the progress model, and movement of the teeth was measured using a digital caliper from the free end of the stainless steel wires to the mesial surface of the maxillary first molar in both experimental and control groups.13,14 The tooth movement was calculated by measuring the extraction space using a vernier caliper before the commencement of en masse retraction (T0), on the 30th day (T1), on the 60th day (T2), and on the 90th day (T3) from the start of retraction in groups A, B, and C on both the right and left sides in all the three groups.
GCF samples were collected at T0, T1, T2 and T3 in groups A, B, and C. Mesial or distal surfaces of either of the maxillary canines were considered the test site.15,16 Selection of the sample site was done based on convenience and continued till the completion of the study.17 The test area was isolated using sterile gauze to prevent saliva contamination. A microcapillary pipette was used for the collection of GCF (Figure 3). At the entrance of the gingival sulcus on either of the proximal regions of the maxillary canines, the pipette was placed touching the gingival margin gently without stimulating it (extracrevicular approach).15,16 Using the calibration on the volumetric microcapillary pipette, a standardized volume of 1 µL was collected. Every sample collection was given a 10-minute window. From our investigation, we omitted the microcapillary pipettes that were contaminated with blood or saliva. The recovered GCF was immediately placed into Eppendorf tubes (0.5 mL), centrifuged again for ten minutes at 3,000 rpm, and then kept at –80°C until the time of the enzyme linked immunosorbent test (ELISA).17
The GCF samples were defrosted. The ELISA tests were conducted using a commercial test system from Raybiotech, Inc. (Norcross, GA, USA). A total of 120 samples were obtained by taking 40 samples from each of the patients in groups A, B, and C.
The data was obtained and sent for statistical analysis.
Tables 1 and 2 show statistically significant differences in the rate of OTM in all three groups at three different time intervals, with the maximum difference seen in Group B compared to Group A and the control group on both the right and left sides (Figures 4 and 5). Group B showed a significantly higher rate of OTM (1.463 ± 0.115 mm), while the control showed the least (0.644 ± 0.155 mm) on the right side at the end of the first month. The tooth movement on the right side was highest at the end of the first month in Group B, which gradually decreased negligibly from 1.117 ± 0.153 mm to 1.119 ± 0.221 mm at the end of the second and third-month respectively.
The tooth movement on the left side was highest in Group B (1.424 ± 0.220 mm) at the end of the first month to 1.311 ± 0.166 mm and 1.106 ± 0.318 mm at the end of the second and third month respectively.
Table 3 and Figure 6 show a comparison of the mean PGE2 levels (in pg/mL) between 3 groups at different time intervals using the Kruskal Wallis test followed by Dunn’s post hoc test. There was a statistically significant difference in all three groups at T1, T2, and T3, with the mean value of PGE2 seen more increased in Group B at T1, T2, and T3, followed by Group A and least in Group C.
Table 4 and Figure 7 show the comparison of the mean PGE2 levels (in pg/mL) between different time intervals in each group using Friedman’s test. There was a statistically significant difference at all the time intervals in all three groups, with a maximum increase in the value of PGE2 level at T1 followed by T2 and then T3 in all three groups compared to T0, which showed the least values.
One of the most important factors satisfying the orthodontic patient is the duration of the treatment. The fixed orthodontic treatment usually lasts longer, such as for two years; this can lead to harmful effects on the surrounding tissues. Due to this, many investigators tried finding better systems that could help reduce the time of orthodontic treatment could minimize the deleterious effects that were caused.18,19 There are many disadvantages of prolonged orthodontic treatment, especially in adults, such as resorption of the roots, pain, etc. The advent of investigating options that can reduce OTM has been a recent interest. The alveolar bone undergoing remodeling, inflammation, and the reaction of bone cells that have been caused due to the forces applied during orthodontic treatment causes the rapid movement of the tooth.18,20,21
Various techniques for accelerating the movement of the tooth during orthodontic treatment, such as electric current, magnets, laser beam, Wilckodontics, corticision, pulsed electromagnetic fields, and peizopuncture had their disadvantages.18-21 So we decided on using our customized electric toothbrush providing mechanical vibration of 125 Hz and 150 Hz each and assessed the level of PGE2 on their usage. The two intensities considered to be incorporated into the electric toothbrushes were based on a finite element study conducted in 2021.20
To visualize the definite effect of vibrational frequencies on the teeth and surrounding tissues, it was necessary to use the electric toothbrush for a longer duration. According to previous reports, the efficacy of all brushes (electric and manual) was attained after 30 seconds of brushing per quadrant or two minutes for the entire mouth. This was consistent with the findings of a more recent systematic review, which also demonstrated enhanced toothbrush efficacy after two minutes of brushing.21 While another study showed that better plaque management was correlated with a longer period of brushing (at least 2 minutes). Therefore, our study advised patients to brush their teeth for one minute per tooth.22
The periodontium and alveolar bone change as a result of the forces used to shift teeth during orthodontic treatment. Even when a smaller orthodontic force is used for a longer period, the periodontium becomes inflamed, leading to the resorption of the alveolar bone and, ultimately, tooth displacement.23 During this method of alveolar bone resorption, inflammatory mediators such as prostaglandins and cytokines are released.
Bowman24 reported a 30% statistically significant increase in the rate of OTM, where 3 mm/month and 2.1 mm/month movement of the tooth were seen in the maxilla and mandible, respectively. This was after the introduction of Acceledent in 2009, where this appliance, when used for 20 minutes/day, was believed to stimulate the differentiation of cells and its maturation, leading to faster bone remodeling and movement of teeth.
In vitro studies have shown the vibratory forces, which are intermittent induced expressions of receptor activator of nuclear factor kappa-B ligand (RANKL) in human periodontal ligament stem cells (PDLSCs). Various studies showed that the short duration, high-frequency resonance vibration, and orthodontic forces accelerate the rate of OTM.25,26 The vibratory device accelerated the rate of OTM by 2–3 mm/month by enhancing periodontal and alveolar modelling.27
A study done by Alikhani et al.28 where the maxillae of mice were exposed to 0.3 g acceleration at various frequencies such as static, 30 Hz, 60 Hz, 100 Hz, and 200 Hz showed a significant increase in the rate of alveolar bone formation and mineral density of bone as different frequencies.
PGE2 has long been known to be a powerful bone resorption activator. Prostaglandins, along with thromboxanes and leukotrienes, are the hormones that are the chemical messengers belonging to the family of eicosanoids.23,29
Prostaglandins, a key chemical messenger for bone resorption produced by the activated cells, were shown to increase bone resorption through cyclic adenosine monophosphate (cAMP) by Raisz et al.30 and Dowsett et al.31 According to a study by Leiker et al.,32 exogenous doses of PGE2 given to rats over an extended period improved tooth mobility.
Yamasaki et al.33 were among the first researchers in orthodontics to look into the involvement of prostaglandins in bone resorption brought on by OTM. In a related investigation on cats, Davidovitch et al.34 discovered elevated levels of PGE2 in the alveolar bone following the application of orthodontic force. Following the application of orthodontic forces, PGE2 triggers the synthesis of mRNA and RANKL, which aids in the start of osteoclastic activity, resulting in bone resorption and migration of the tooth from the ayma.35
The results of our study showed that there was an increase in the level of PGE2 with the increase in the frequency of mechanical vibration, which was per the study done by Benjakul et al.36 This study suggested that both vibration and compressive stress are likely to induce RANKL expression in PDL cells via the cyclooxygenase (COX/PGE2) signalling pathway. The study was on the effects of low magnitude high frequency (LMHF) mechanical vibrations, which showed that the applied vibrations in the increasing frequency on the periodontal tissue cells caused an increase in RANKL expression in the PDL cells and increased in the release of PGE2.37 RANKL and PGE2 are known to stimulate osteoclastic bone resorption; thus it was shown that the mechanical vibrations applied on the teeth led to the promotion of bone resorption.38
PGE2 is a mediator of inflammation that reacts to many stimuli. Interleukin (IL)-1 levels and tooth movement rates were raised by vibration in orthodontic patients, according to Fukushima et al.39 An inflammatory cytokine called IL-1 has the ability to increase RANKL expression and osteoclastic activity. The expression of RANKL in response to vibration in PDL cells, either vibration alone or combined with compressive stress, depends on the cyclooxygenase pathway. Vibration may directly increase the production of inflammatory cytokines such as PGE2, IL-6 and IL-8.40
Previous studies have shown that the compressive forces increased the level of PGE2 and RANKL in the periodontal tissue cells.41,42 RANKL expressions may induce indirectly due to the application of low-magnitude high-frequency vibrations via the signaling pathway concerning PGE2 in the PDL cells.42 PGE2 has been shown to have autocrine and paracrine effects that help activate receptors of RANKL expression, which causes bone resorption.41,42
Runx2, a transcription factor, is crucial for osteoblast differentiation and bone resorption.43 PDLSCs have been shown to generate Runx2 in response to LMHF vibration.44
Yamasaki et al.45 stated that the amount of tooth movement was increased when prostaglandins were administered locally together with OTM, which was in accordance with the study done by Cağlaroğlu and Erdem.46 Exogenous PGE2 injections for a longer period of time in rats did increase the amount of tooth movement, according to research by Leiker et al.47 on the long-term effects of different dosages and frequency of injectable, exogenous PGE2. In their study on the effects of exogenous PGE2 treatment in young rabbits, Valiathan and Dhar48 noted a clinical increase in the rate of tooth movement and a microscopically observed increase in the number of osteoclasts and resorption lacunae. According to Reitan,49 PGE2 elevated blood calcium levels during OTM, which significantly impacted the cellular response. In addition, Yang et al.50 reported that PGE2 influenced bone resorption via elevating cAMP and calcium levels. The administration of PGE2 accelerated the OTM.
This research study sheds light on the effects of the mechanical vibration of different intensities on the periodontal tissue cells and the mechanism through which levels of PGE2 get affected due to these vibrations. GCF is sensitive to plaque and saliva contamination, and this bias should be considered. Considering the wide range of interfering factors and biological response in both GCF and periodontal ligament, the number of subjects participating in this study may be limiting.
The rate of OTM and levels of PGE2 were highest in patients who used an electric toothbrush with 150 Hz mechanical vibration compared to those who used an electric toothbrush with 125 Hz mechanical vibration. The rate of OTM and the levels of PGE2 were least in patients who did not use the electric toothbrush.
Mechanical vibration led to an increase in the levels of PGE2 and accelerated the OTM.
Notes
REFERENCES
1. Piccioni MA, Campos EA, Saad JR, Andrade MF, Galvão MR, Rached AA. 2013; Application of the finite element method in dentistry. RSBO. 10:369–77. https://doi.org/10.21726/rsbo.v10i4.948. DOI: 10.21726/rsbo.v10i4.948.


2. Sarmah A, Mathur AK, Gupta V, Pai VS, Nandini S. 2011; Finite element analysis of dental implant as orthodontic anchorage. J Contemp Dent Pract. 12:259–64. https://doi.org/10.5005/jp-journals-10024-1044. DOI: 10.5005/jp-journals-10024-1044. PMID: 22186860.


3. Singh JR, Kambalyal P, Jain M, Khandelwal P. 2016; Revolution in orthodontics: finite element analysis. J Int Soc Prev Community Dent. 6:110–4. https://doi.org/10.4103/2231-0762.178743. DOI: 10.4103/2231-0762.178743. PMID: 27114948. PMCID: PMC4820568.


4. Ansari TA, Mascarenhas R, Husain A, Salim M. 2011; Evaluation of the power arm in bringing about bodily movement using finite element analysis. Orthodontics (Chic. ). 12:318–29. https://pubmed.ncbi.nlm.nih.gov/22299105/. DOI: 10.1201/b15951-28.
5. Bica C, Brezeanu L, Bica D, Suciu M. 2015; Biomechanical reactions due to orthodontic forces. a finite element study. Procedia Technol. 19:895–900. https://doi.org/10.1016/j.protcy.2015.02.128. DOI: 10.1016/j.protcy.2015.02.128.


6. Hamanaka R, Yamaoka S, Anh TN, Tominaga JY, Koga Y, Yoshida N. 2017; Numeric simulation model for long-term orthodontic tooth movement with contact boundary conditions using the finite element method. Am J Orthod Dentofacial Orthop. 152:601–12. https://doi.org/10.1016/j.ajodo.2017.03.021. DOI: 10.1016/j.ajodo.2017.03.021. PMID: 29103438.


7. Takano-Yamamoto T, Sasaki K, Fatemeh G, Fukunaga T, Seiryu M, Daimaruya T, et al. 2017; Synergistic acceleration of experimental tooth movement by supplementary high-frequency vibration applied with a static force in rats. Sci Rep. 7:13969. https://doi.org/10.1038/s41598-017-13541-7. DOI: 10.1038/s41598-017-13541-7. PMID: 29070874. PMCID: PMC5656656.


8. Klein DC, Raisz LG. 1970; Prostaglandins: stimulation of bone resorption in tissue culture. Endocrinology. 86:1436–40. https://doi.org/10.1210/endo-86-6-1436. DOI: 10.1210/endo-86-6-1436. PMID: 4315103.


9. Alfaqeeh SA, Anil S. 2014; Gingival crevicular fluid flow rate and alkaline phosphatase level as potential marker of active tooth movement. Oral Health Dent Manag. 13:458–63. https://pubmed.ncbi.nlm.nih.gov/24984665/.
10. Almeida RC, Capelli J Jr, Teles RP. 2015; Levels of gingival crevicular fluid matrix metalloproteinases in periodontally compromised teeth under orthodontic forces. Angle Orthod. 85:1009–14. https://doi.org/10.2319/101714-744.1. DOI: 10.2319/101714-744.1. PMID: 25751014. PMCID: PMC8612058.


11. Collins DA, Chambers TJ. 1992; Prostaglandin E2 promotes osteoclast formation in murine hematopoietic cultures through an action on hematopoietic cells. J Bone Miner Res. 7:555–61. https://doi.org/10.1002/jbmr.5650070512. DOI: 10.1002/jbmr.5650070512. PMID: 1615762.


12. Chaudhari VC, Suchita MT. 2015; Comparison of rate of retraction and anchorage loss using nickel titanium closed coil springs and elastomeric chain during the en-masse retraction: a clinical study. J Orthod Res. 3:129–33. https://doi.org/10.4103/2321-3825.150582. DOI: 10.4103/2321-3825.150582.


13. Suamphan S. 2010. Change in rate of orthodontic tooth movement and interleukin-1 beta level in gingival crevicular fluid in response to mechanical vibratory stimulation from electrical toothbrush [Master's Thesis]. Prince of Songkla University;Songkhla: http://kb.psu.ac.th:8080/psukb/bitstream/2010/8860/1/345954.pdf.
14. Limpanichkul W, Godfrey K, Srisuk N, Rattanayatikul C. 2006; Effects of low-level laser therapy on the rate of orthodontic tooth movement. Orthod Craniofac Res. 9:38–43. https://doi.org/10.1111/j.1601-6343.2006.00338.x. DOI: 10.1111/j.1601-6343.2006.00338.x. PMID: 16420273.


15. Fenol A, Sasidharan RK, Krishnan S. 2014; Levels of interleukin-10 in gingival crevicular fluid and its role in the initiation and progression of gingivitis to periodontitis. J Oral Hyg Health. 2:135. https://doi.org/10.4172/2332-0702.1000135. DOI: 10.4172/2332-0702.1000135.


16. Jamesha FI, Maradi AP, Chithresan K, Janakiram S, Maddur PK, Rangaraju R. 2018; Comparison of gingival crevicular fluid periostin levels in healthy, chronic periodontitis, and aggressive periodontitis. J Indian Soc Periodontol. 22:480–6. https://doi.org/10.4103/jisp.jisp_266_18. DOI: 10.4103/jisp.jisp_266_18. PMID: 30631225. PMCID: PMC6305088.


17. Gujar AN, Baeshen HA, Alhazmi A, Bhandi S, Raj AT, Patil S, et al. 2019; Cytokine levels in gingival crevicular fluid during orthodontic treatment with aligners compared to conventional labial fixed appliances: a 3-week clinical study. Acta Odontol Scand. 77:474–81. https://doi.org/10.1080/00016357.2019.1607548. DOI: 10.1080/00016357.2019.1607548. PMID: 31027423.


18. Nishimura M, Chiba M, Ohashi T, Sato M, Shimizu Y, Igarashi K, et al. 2008; Periodontal tissue activation by vibration: intermittent stimulation by resonance vibration accelerates experimental tooth movement in rats. Am J Orthod Dentofacial Orthop. 133:572–83. https://doi.org/10.1016/j.ajodo.2006.01.046. DOI: 10.1016/j.ajodo.2006.01.046. PMID: 18405822.


19. Yadav S, Dobie T, Assefnia A, Gupta H, Kalajzic Z, Nanda R. 2015; Effect of low-frequency mechanical vibration on orthodontic tooth movement. Am J Orthod Dentofacial Orthop. 148:440–9. https://doi.org/10.1016/j.ajodo.2015.03.031. DOI: 10.1016/j.ajodo.2015.03.031. PMID: 26321342.


20. Gujar AN, Shivamurthy PG, Sabrish S. 2021; Effect of 125-150 Hz vibrational frequency electric toothbrush on teeth and supporting structures: a finite element method study. J Contemp Dent Pract. 22:1150–9. https://doi.org/10.5005/jp-journals-10024-3202. DOI: 10.5005/jp-journals-10024-3202.


21. Slot DE, Wiggelinkhuizen L, Rosema NA, Van der Weijden GA. 2012; The efficacy of manual toothbrushes following a brushing exercise: a systematic review. Int J Dent Hyg. 10:187–97. https://doi.org/10.1111/j.1601-5037.2012.00557.x. DOI: 10.1111/j.1601-5037.2012.00557.x. PMID: 22672101.


22. Bahammam S, Chen CY, Ishida Y, Hayashi A, Ikeda Y, Ishii H, et al. 2021; Electric and manual oral hygiene routines affect plaque index score differently. Int J Environ Res Public Health. 18:13123. https://doi.org/10.3390/ijerph182413123. DOI: 10.3390/ijerph182413123. PMID: 34948732. PMCID: PMC8701503.


23. Raisz LG. 1999; Prostaglandins and bone: physiology and pathophysiology. Osteoarthritis Cartilage. 7:419–21. https://doi.org/10.1053/joca.1998.0230. DOI: 10.1053/joca.1998.0230. PMID: 10419786.


24. Bowman SJ. 2014; The effect of vibration on the rate of leveling and alignment. J Clin Orthod. 48:678–88. https://pubmed.ncbi.nlm.nih.gov/25707947/.
25. Kau CH, Nguyen JT, English JD. 2010; The clinical evaluation of a novel cyclical force generating device in orthodontics. Orthod Pract US. 1:10–5. https://cdn.vivarep.com/contrib/va/documents/al_lib_66.2013103193933523.pdf.
26. Kau CH. 2011; A radiographic analysis of tooth morphology following the use of a novel cyclical force device in orthodontics. Head Face Med. 7:14. https://doi.org/10.1186/1746-160X-7-14. DOI: 10.1186/1746-160X-7-14. PMID: 21827655. PMCID: PMC3162905. PMID: 1475646d1c5e4b348c245bfaa140d2f4.


27. Drummond S, Canavarro C, Perinetti G, Teles R, Capelli J Jr. 2012; The monitoring of gingival crevicular fluid volume during orthodontic treatment: a longitudinal randomized split-mouth study. Eur J Orthod. 34:109–13. https://doi.org/10.1093/ejo/cjq172. DOI: 10.1093/ejo/cjq172. PMID: 21273285.


28. Alikhani M, Khoo E, Alyami B, Raptis M, Salgueiro JM, Oliveira SM, et al. 2012; Osteogenic effect of high-frequency acceleration on alveolar bone. J Dent Res. 91:413–9. https://doi.org/10.1177/0022034512438590. DOI: 10.1177/0022034512438590. PMID: 22337699. PMCID: PMC3310758.


29. Dinarello CA. 1988; Biology of interleukin 1. FASEB J. 2:108–15. https://doi.org/10.1096/fasebj.2.2.3277884. DOI: 10.1096/fasebj.2.2.3277884. PMID: 3277884.


30. Raisz LG, Sandberg AL, Goodson JM, Simmons HA, Mergenhagen SE. 1974; Complement-dependent stimulation of prostaglandin synthesis and bone resorption. Science. 185:789–91. https://doi.org/10.1126/science.185.4153.789. DOI: 10.1126/science.185.4153.789. PMID: 4843377.


31. Dowsett M, Eastman AR, Easty DM, Easty GC, Powles TJ, Neville AM. 1976; Prostaglandin mediation of collagenase-induced bone resorption. Nature. 263:72–4. https://doi.org/10.1038/263072a0. DOI: 10.1038/263072a0. PMID: 183146.


32. Leiker BJ, Nanda RS, Currier GF, Howes RI, Sinha PK. 1995; The effects of exogenous prostaglandins on orthodontic tooth movement in rats. Am J Orthod Dentofacial Orthop. 108:380–8. https://doi.org/10.1016/s0889-5406(95)70035-8. DOI: 10.1016/S0889-5406(95)70035-8. PMID: 7572849.


33. Yamasaki K, Miura F, Suda T. 1980; Prostaglandin as a mediator of bone resorption induced by experimental tooth movement in rats. J Dent Res. 59:1635–42. https://doi.org/10.1177/00220345800590101301. DOI: 10.1177/00220345800590101301. PMID: 6932420.


34. Davidovitch Z, Nicolay OF, Ngan PW, Shanfeld JL. 1988; Neurotransmitters, cytokines, and the control of alveolar bone remodeling in orthodontics. Dent Clin North Am. 32:411–35. https://doi.org/10.1016/S0011-8532(22)00320-2. DOI: 10.1016/S0011-8532(22)00320-2. PMID: 2900159.


35. Yamasaki K. 1983; The role of cyclic AMP, calcium, and prostaglandins in the induction of osteoclastic bone resorption associated with experimental tooth movement. J Dent Res. 62:877–81. https://doi.org/10.1177/00220345830620080501. DOI: 10.1177/00220345830620080501. PMID: 6306082.


36. Benjakul S, Jitpukdeebodintra S, Leethanakul C. 2018; Effects of low magnitude high frequency mechanical vibration combined with compressive force on human periodontal ligament cells in vitro. Eur J Orthod. 40:356–63. https://doi.org/10.1093/ejo/cjx062. DOI: 10.1093/ejo/cjx062. PMID: 29016746.


37. Leethanakul C, Suamphan S, Jitpukdeebodintra S, Thongudomporn U, Charoemratrote C. 2016; Vibratory stimulation increases interleukin-1 beta secretion during orthodontic tooth movement. Angle Orthod. 86:74–80. https://doi.org/10.2319/111914-830.1. DOI: 10.2319/111914-830.1. PMID: 25811245. PMCID: PMC8603964.


38. Zhang C, Li J, Zhang L, Zhou Y, Hou W, Quan H, et al. 2012; Effects of mechanical vibration on proliferation and osteogenic differentiation of human periodontal ligament stem cells. Arch Oral Biol. 57:1395–407. https://doi.org/10.1016/j.archoralbio.2012.04.010. DOI: 10.1016/j.archoralbio.2012.04.010. PMID: 22595622.


39. Fukushima H, Jimi E, Okamoto F, Motokawa W, Okabe K. 2005; IL-1-induced receptor activator of NF-kappa B ligand in human periodontal ligament cells involves ERK-dependent PGE2 production. Bone. 36:267–75. https://doi.org/10.1016/j.bone.2004.09.011. DOI: 10.1016/j.bone.2004.09.011. PMID: 15780952.


40. Krishnan V, Davidovitch Z. 2006; Cellular, molecular, and tissue-level reactions to orthodontic force. Am J Orthod Dentofacial Orthop. 129:469.e1–32. https://doi.org/10.1016/j.ajodo.2005.10.007. DOI: 10.1016/j.ajodo.2005.10.007. PMID: 16627171.


41. Römer P, Köstler J, Koretsi V, Proff P. 2013; Endotoxins potentiate COX-2 and RANKL expression in compressed PDL cells. Clin Oral Investig. 17:2041–8. https://doi.org/10.1007/s00784-013-0928-0. DOI: 10.1007/s00784-013-0928-0. PMID: 23392729.


42. Nettelhoff L, Grimm S, Jacobs C, Walter C, Pabst AM, Goldschmitt J, et al. 2016; Influence of mechanical compression on human periodontal ligament fibroblasts and osteoblasts. Clin Oral Investig. 20:621–9. https://doi.org/10.1007/s00784-015-1542-0. DOI: 10.1007/s00784-015-1542-0. PMID: 26243456.


43. Marie PJ. 2008; Transcription factors controlling osteoblastogenesis. Arch Biochem Biophys. 473:98–105. https://doi.org/10.1016/j.abb.2008.02.030. DOI: 10.1016/j.abb.2008.02.030. PMID: 18331818.


44. Chen D, Kim S, Lee S, Lee JM, Choi YJ, Shin SJ, et al. 2021; The effect of mechanical vibration on osteogenesis of periodontal ligament stem cells. J Endod. 47:1767–74. https://doi.org/10.1016/j.joen.2021.08.014. DOI: 10.1016/j.joen.2021.08.014. PMID: 34492230.
45. Yamasaki K, Shibata Y, Imai S, Tani Y, Shibasaki Y, Fukuhara T. 1984; Clinical application of prostaglandin E1 (PGE1) upon orthodontic tooth movement. Am J Orthod. 85:508–18. https://doi.org/10.1016/0002-9416(84)90091-5. DOI: 10.1016/0002-9416(84)90091-5. PMID: 6587784.
46. Cağlaroğlu M, Erdem A. 2012; Histopathologic investigation of the effects of prostaglandin E2 administered by different methods on tooth movement and bone metabolism. Korean J Orthod. 42:118–28. https://doi.org/10.4041/kjod.2012.42.3.118. DOI: 10.4041/kjod.2012.42.3.118. PMID: 23112942. PMCID: PMC3481982.
47. Seifi M, Eslami B, Saffar AS. 2003; The effect of prostaglandin E2 and calcium gluconate on orthodontic tooth movement and root resorption in rats. Eur J Orthod. 25:199–204. https://doi.org/10.1093/ejo/25.2.199. DOI: 10.1093/ejo/25.2.199. PMID: 12737218.
48. Valiathan A, Dhar S. 2006; Prostaglandins and enhanced orthodontic tooth movement: in search of the silver bullet. Curr Sci. 90:311–3. https://www.jstor.org/stable/24091864.
49. Reitan K. 1967; Clinical and histologic observations on tooth movement during and after orthodontic treatment. Am J Orthod. 53:721–45. https://doi.org/10.1016/0002-9416(67)90118-2. DOI: 10.1016/0002-9416(67)90118-2. PMID: 5233926.


50. Yang RS, Fu WM, Wang SM, Lu KS, Liu TK, Lin-Shiau SY. 1998; Morphological changes induced by prostaglandin E in cultured rat osteoblasts. Bone. 22:629–36. https://doi.org/10.1016/s8756-3282(98)00055-6. DOI: 10.1016/S8756-3282(98)00055-6. PMID: 9626401.


Figure 4
Comparison of the mean rate of orthodontic tooth movement (mm) on the right side between 3 groups at different time intervals. The dot in the graph is the outlier, its a part of box plot graph.
b/w, between; T0, retraction; T1, on the 30th day; T2, on the 60th day; T3, on the 90th day.
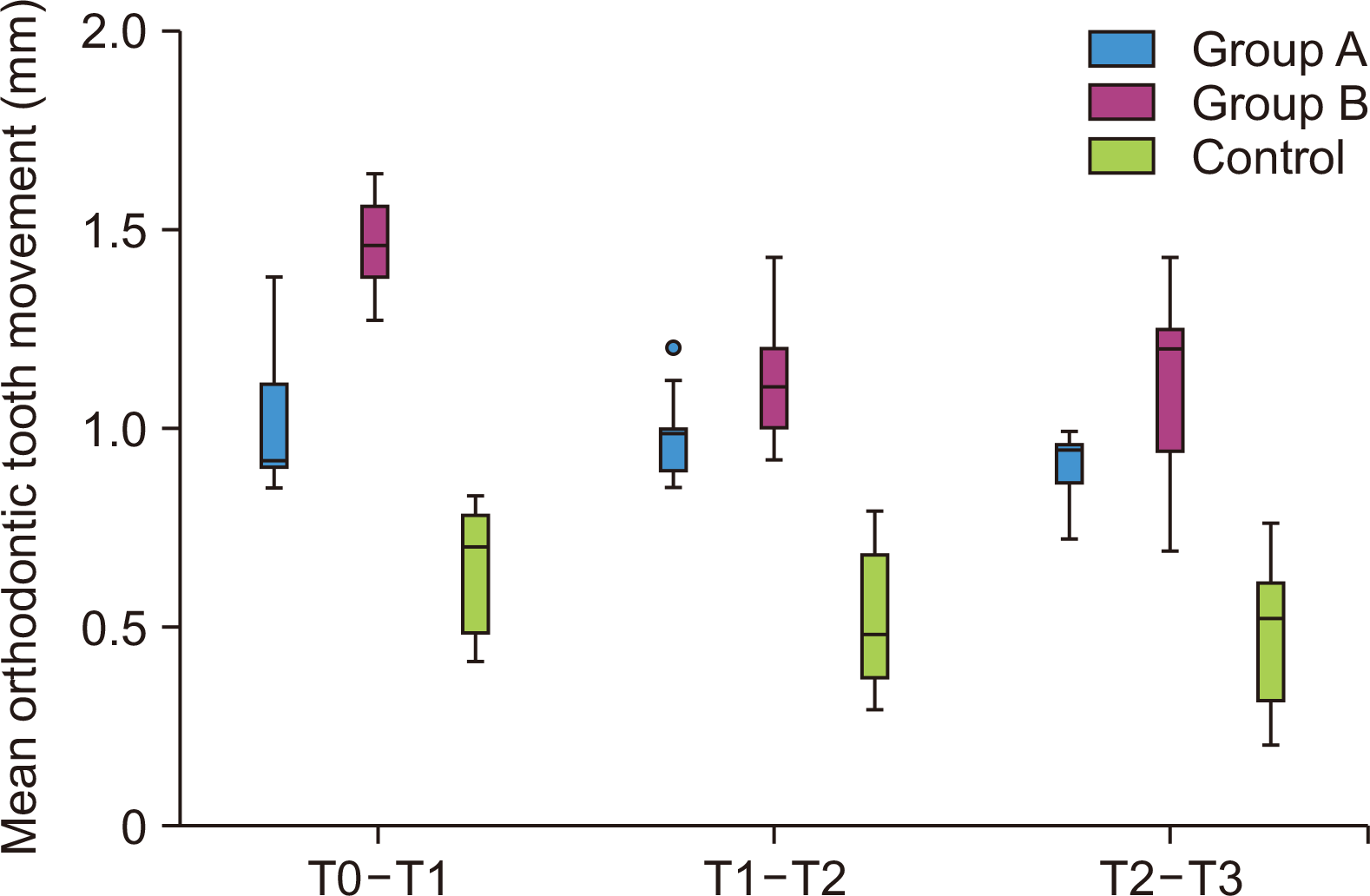
Figure 5
Comparison of the mean rate of orthodontic tooth movement (mm) on the left side between 3 groups at different time intervals. The dot in the graph is the outlier, its a part of box plot graph.
b/w, between; T0, retraction; T1, on the 30th day; T2, on the 60th day; T3, on the 90th day.
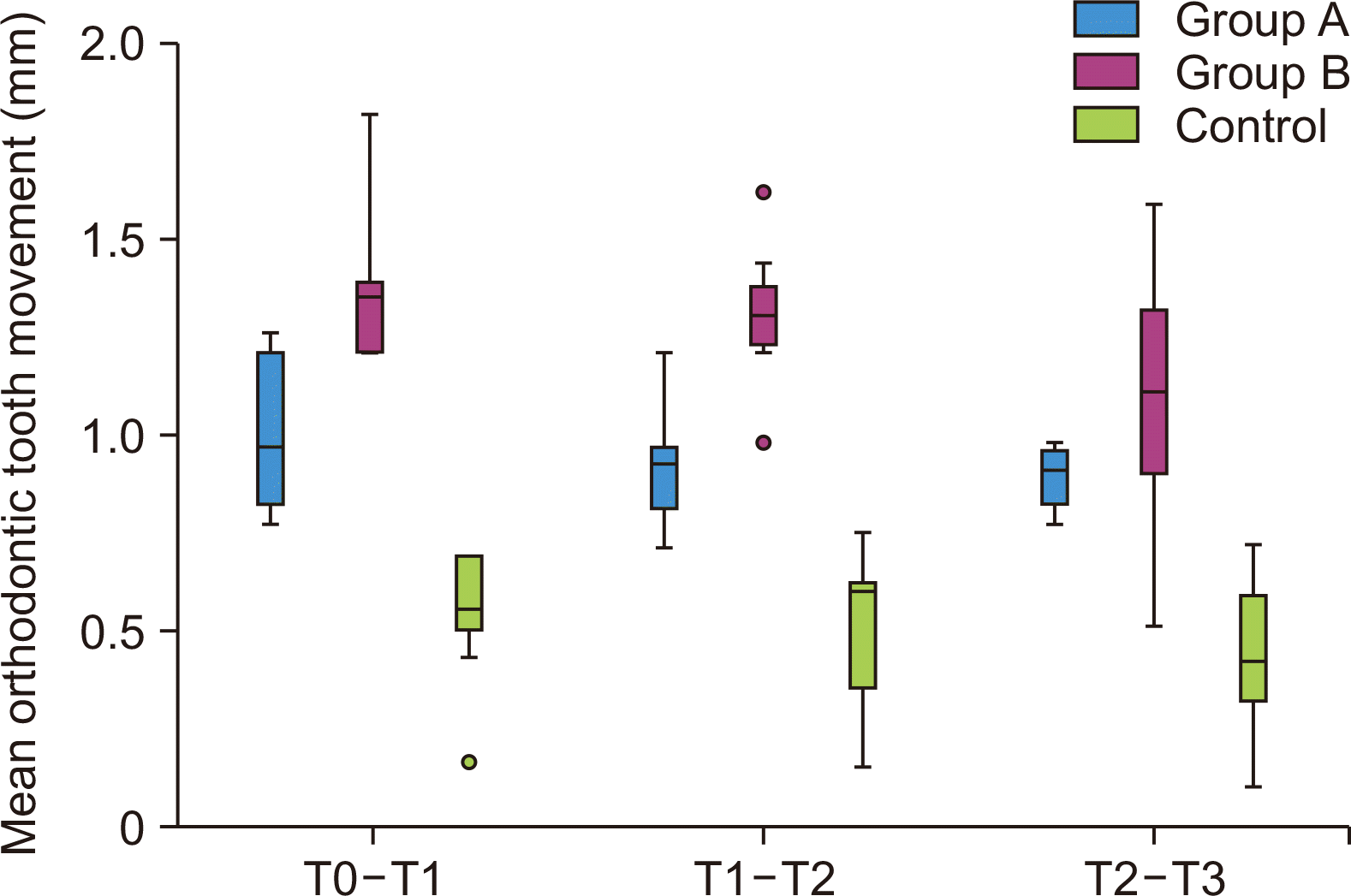
Figure 6
Comparison of mean prostaglandin E2 (PGE2) levels (pg/mL) between 3 groups at different time intervals.
T0, retraction; T1, on the 30th day; T2, on the 60th day; T3, on the 90th day.
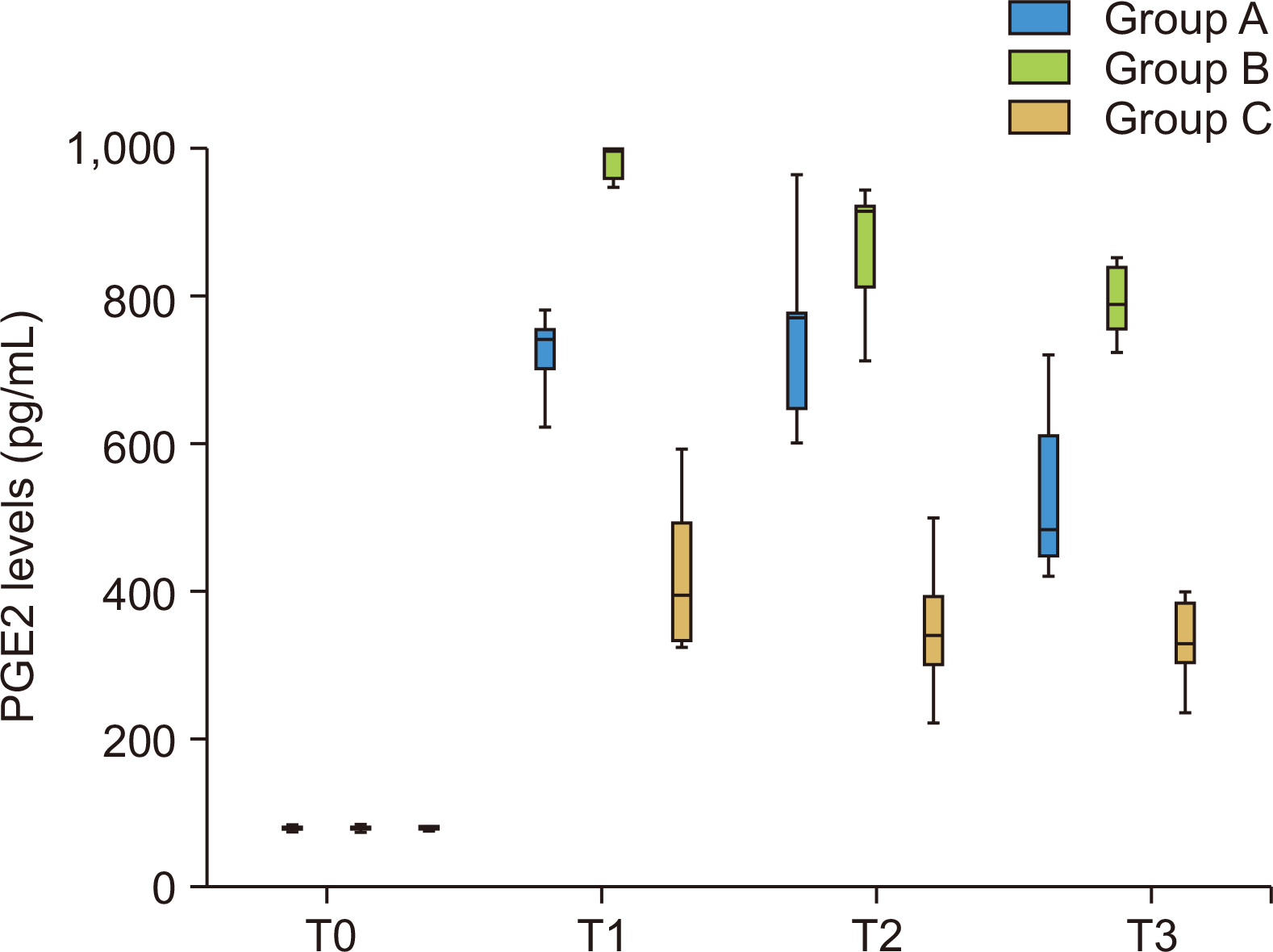
Figure 7
Comparison of mean prostaglandin E2 (PGE2) levels (pg/mL) between different time intervals in each group.
T0, retraction; T1, on the 30th day; T2, on the 60th day; T3, on the 90th day.
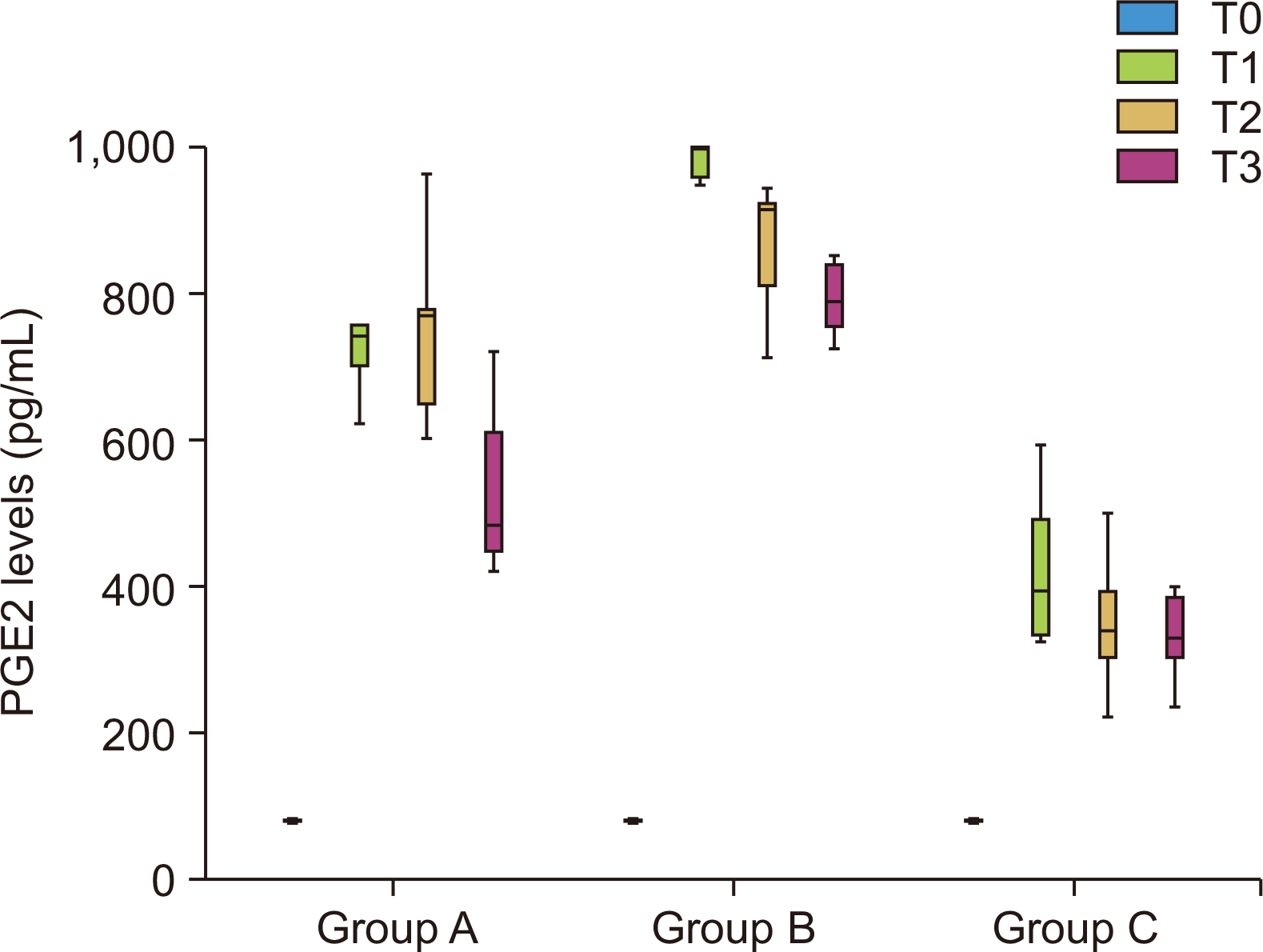
Table 1
Comparison of the mean rate of orthodontic tooth movement (in mm) on the right side between 3 groups at different time intervals
Time | Groups | N | Mean | SD | Minimum | Maximum | P value | Sig. Diff | P value |
---|---|---|---|---|---|---|---|---|---|
T0–T1 | Group A | 10 | 1.020 | 0.178 | 0.85 | 1.38 | < 0.001* | A vs. B | < 0.001* |
Group B | 10 | 1.463 | 0.115 | 1.27 | 1.64 | A vs. C | < 0.001* | ||
Control | 10 | 0.644 | 0.155 | 0.41 | 0.83 | B vs. C | < 0.001* | ||
T1–T2 | Group A | 10 | 0.982 | 0.110 | 0.85 | 1.20 | < 0.001* | A vs. B | 0.129 |
Group B | 10 | 1.117 | 0.153 | 0.92 | 1.43 | A vs. C | < 0.001* | ||
Control | 10 | 0.521 | 0.179 | 0.29 | 0.79 | B vs. C | < 0.001* | ||
T2–T3 | Group A | 10 | 0.909 | 0.087 | 0.72 | 0.99 | < 0.001* | A vs. B | 0.031 |
Group B | 10 | 1.119 | 0.221 | 0.69 | 1.43 | A vs. C | < 0.001* | ||
Control | 10 | 0.473 | 0.185 | 0.20 | 0.76 | B vs. C | < 0.001* |
Table 2
Comparison of the mean rate of orthodontic tooth movement (in mm) on the left side between 3 groups at different time intervals
Time | Groups | N | Mean | SD | Minimum | Maximum | P value | Sig. Diff | P value |
---|---|---|---|---|---|---|---|---|---|
T0–T1 | Group A | 10 | 1.007 | 0.181 | 0.77 | 1.26 | < 0.001* | A vs. B | < 0.001* |
Group B | 10 | 1.424 | 0.220 | 1.21 | 1.82 | A vs. C | < 0.001* | ||
Control | 10 | 0.547 | 0.164 | 0.16 | 0.69 | B vs. C | < 0.001* | ||
T1–T2 | Group A | 10 | 0.913 | 0.159 | 0.71 | 1.21 | < 0.001* | A vs. B | < 0.001* |
Group B | 10 | 1.311 | 0.166 | 0.98 | 1.62 | A vs. C | < 0.001* | ||
Control | 10 | 0.517 | 0.191 | 0.15 | 0.75 | B vs. C | < 0.001* | ||
T2–T3 | Group A | 10 | 0.895 | 0.077 | 0.77 | 0.98 | < 0.001* | A vs. B | 0.094 |
Group B | 10 | 1.106 | 0.318 | 0.51 | 1.59 | A vs. C | < 0.001* | ||
Control | 10 | 0.428 | 0.186 | 0.10 | 0.72 | B vs. C | < 0.001* |
Table 3
Comparison of mean prostaglandin E2 levels (in pg/mL) between 3 groups at different time intervals
Time | Groups | N | Mean | SD | Minimum | Maximum | P value | Sig. Diff | P value |
---|---|---|---|---|---|---|---|---|---|
T0 | Group A | 10 | 78.001 | 3.104 | 72.94 | 82.79 | 0.890 | A vs. B | - |
Group B | 10 | 77.936 | 3.477 | 72.68 | 82.72 | A vs. C | - | ||
Control | 10 | 78.580 | 2.361 | 75.34 | 81.55 | B vs. C | - | ||
T1 | Group A | 10 | 763.136 | 121.335 | 621.65 | 993.57 | < 0.001* | A vs. B | 0.030* |
Group B | 10 | 982.831 | 22.873 | 947.90 | 1,000.00 | A vs. C | 0.007* | ||
Control | 10 | 421.457 | 98.206 | 323.34 | 593.13 | B vs. C | < 0.001* | ||
T2 | Group A | 10 | 750.752 | 109.410 | 601.61 | 964.60 | < 0.001* | A vs. B | 0.170 |
Group B | 10 | 862.605 | 88.645 | 712.86 | 943.88 | A vs. C | 0.002* | ||
Control | 10 | 371.078 | 117.873 | 219.88 | 632.97 | B vs. C | < 0.001* | ||
T3 | Group A | 10 | 531.810 | 107.364 | 420.35 | 720.35 | < 0.001* | A vs. B | 0.006* |
Group B | 10 | 805.595 | 78.472 | 724.29 | 995.00 | A vs. C | 0.040* | ||
Control | 10 | 355.732 | 129.759 | 234.23 | 693.13 | B vs. C | < 0.001* |
Table 4
Comparison of mean prostaglandin E2 levels (in pg/mL) between different time intervals in each group
Group | Time | N | Mean | SD | Minimum | Maximum | P value |
---|---|---|---|---|---|---|---|
Group A | T0 | 10 | 78.0006 | 3.10436 | 72.94 | 82.79 | < 0.001* |
T1 | 10 | 763.136 | 121.335 | 621.65 | 993.57 | ||
T2 | 10 | 750.752 | 109.41 | 601.61 | 964.60 | ||
T3 | 10 | 531.81 | 107.364 | 420.35 | 720.35 | ||
Group B | T0 | 10 | 77.936 | 3.47686 | 72.68 | 82.72 | < 0.001* |
T1 | 10 | 982.831 | 22.8729 | 947.90 | 1,000.00 | ||
T2 | 10 | 862.605 | 88.645 | 712.86 | 943.88 | ||
T3 | 10 | 805.595 | 78.4719 | 724.29 | 995.00 | ||
Group C | T0 | 10 | 78.5801 | 2.36103 | 75.34 | 81.55 | < 0.001* |
T1 | 10 | 421.457 | 98.2062 | 323.34 | 593.13 | ||
T2 | 10 | 371.078 | 117.873 | 219.88 | 632.97 | ||
T3 | 10 | 355.732 | 129.759 | 234.23 | 693.13 |