Abstract
Ankylosing spondylitis (AS) is an autoinflammatory disease that manifests with the unique feature of enthesitis. Gut microbiota, HLA-B*27, and biomechanical stress mutually influence and interact resulting in setting off a flame of inflammation. In the HLA-B*27 positive group, dysbiosis in the gut environment disrupts the barrier to exogenous bacteria or viruses. Additionally, biomechanical stress induces inflammation through enthesial resident or gut-origin immune cells. On this basis, innate and adaptive immunity can propagate inflammation and lead to chronic disease. Finally, bone homeostasis is regulated by cytokines, by which the inflamed region is substituted into new bone. Agents that block cytokines are constantly being developed to provide diverse therapeutic options for preventing the progression of inflammation. In addition, some antibodies have been shown to distinguish disease selectively, which support the involvement of autoimmune immunity in AS. In this review, we critically analyze the complexity and uniqueness of the pathogenesis with updates on the findings of immunity and provide new information about biologics and biomarkers.
Ankylosing spondylitis (AS) is a radiographic axial spondyloarthritis (axSpA), mainly involving the spine and sacroiliac joint, and characterized by inflammation leading to ankylosis. AS is a chronic inflammatory disease with a critical gene, HLA-B*27, associated with environmental factors such as infections or injury due to high mechanical stress [1-3]. AS also manifests with peripheral involvement including enthesitis, dactylitis, arthritis, and extra-articular symptoms such as uveitis, psoriasis, and inflammatory bowel disease (IBD). Enthesitis, inflammation of the tendons and ligaments attached to bone, is a distinctive feature that plays a central role in the pathophysiology of AS [4]. Osteogenesis occurs at enthesial sites, and there is evidence that fatty degeneration in combination with inflammation could contribute to new bone formation in patients with AS [5-7].
Unlike other rheumatic diseases, AS is generally perceived as an autoinflammatory disease that is induced by the innate immune system. However, it remains unclear whether autoantibodies or autoantigen-specific T cells are involved in AS pathogenesis. Thus, AS has been regarded as a more autoinflammatory disease than autoimmune diseases such as other rheumatic diseases. Studies on the pathogenesis of AS are still underway, and evolving proteogenomics brings adaptive immunity to the surface. Currently, AS is suggested as an assembly of autoinflammation and autoimmunity, with growing evidence of autoimmune responses in patients with AS [2].
In this review, we have discussed the new mechanisms of pathogenesis, newly detected antibodies, and additionally verified immune reactions.
Microbiota is a general term for a community of microorganisms themselves [8-10]. The human gastrointestinal tract contains 100 trillion microorganisms, most of which colonize bacteria with viruses, fungi, and protozoa. The microbiome is a collective genome of microorganisms in a particular environment and considered one of the body organs. This microbial organ mutually affects the host and influences the host immune system through metabolites. There are two concepts regarding the interaction mechanism between HLA-B*27 and the gut microbiota. First, HLA-B*27 exhibits molecular mimicry of some bacterial antigen components, which could increase the number of cells producing interferon (IFN)-γ [11]. Another theory is that the misfolding of HLA-B*27 in endoplasmic reticulum (ER) could cause ER stress, inducing an unfolded protein response or autophagy [12]. Microorganisms could be pathogenic in patients with AS, because molecular mimicry causes autoimmunity and immunological cross-reactivity [13,14]. Among the bacteria, Klebsiella pneumoniae in human feces was associated with AS and stronger in patients with active AS. In addition, there was an overlap of homologous peptides in HLA-B*27.1. Moreover, K. pneumoniae nitrogenase reductase was revealed to have six consecutive amino acids [11]. AS and rheumatoid arthritis (RA) have distinct gut microbiome features. However, it is unclear whether these differences are the cause or result of HLA alleles. Asquith et al. [15] examined intestinal tissues and stools in patients with AS and RA and found that the microbial composition was associated with HLA alleles. This supports the hypothesis that HLA-B*27 could affect the gut microbiota. Microbiota in patients with AS was found to be reduced in diversity, whereas taxonomic diversity in the mucosal-associated microbiota was increased [16-19]. In a recent study, healthy controls with HLA-B*27 showed reduced microbiota, similar to patients with SpA [20]. This study used DNA shotgun sequencing of the microbiome in human stool, which is superior to metagenomic analysis. It verified that the disease activity in patients with AS was associated with a lesser extent of dysbiosis, whereas Ruminococcus gnavus, Erysipelatoclostridium ramosum, Clostridium symbiosum, and Clostridium bolteae were enriched in those with severe disease. Inflammasome activation has been evaluated in peripheral blood monocytes and the gut of AS rodent models [21]. Although there is no evident cause of inflammasome activation, microorganisms could induce immune genetic factors.
Gut dysbiosis increases the incidence of AS by draining lymph nodes from the gastrointestinal tract near the pelvic area where the sacroiliac joint is located. RNA-seq assists in identifying gut-origin trafficking molecules to verify the effect of the gut on arthritis. It was found in the synovial fluid from patients with AS that potential pathogenic CD8+T cell subsets had gut-associated trafficking molecules, CD103+β7+CD29+CD49a+, and distinct transcriptional profiles, unlike other joint-derived CD8+T cells with cytotoxic and regulatory transcriptomic effects [22]. CD103+β7+ binds to human intestinal microvascular endothelia cells [23] and CD49+CD29+, or VLA-1, is found on 48% of gut-resident CD8+T cells [24].
The link between the gut microbiome and joint inflammation can be explained by the metabolites produced by the gut microbiome. Metabolites from the gut microbiome containing short-chain fatty acids, tryptophan metabolites, amino acids, trimethylamine, and B vitamins have been found to modulate disease by balancing gut barriers, T cell regulation, and cytokines [10]. Compared to the indole produced by tryptophanase, which is exclusive to bacteria, indole-3-acetate and indole-3-acetaldehyde are increased in axSpA, irrespective of bowel inflammation [25].
Potential pathogens other than the gut microbiota affect the occurrence of AS. Unlike previous studies, the incidence of AS increased 6 years after Candida infection [26]. Human papilloma virus (HPV) infection had 1.348 times greater risk of AS compared with the non-HPV cohort and has the potential to trigger systemic lupus erythematosus [27,28]. Compared with other autoimmune diseases among human immunodeficiency virus (HIV)-positive population, AS showed the strongest association with HIV with adjusted hazard ratio 1.82 (1.03~3.21) [29].
The gut microbiota has received attention with increasing evidence of a connection between the gut environment and AS. Technological developments have enabled the verification of the personal gut microbiome. Specific flora can be used for diagnosis and treatment tailored to individuals, such as dietary supplements.
HLA-B*27 is the most critical genetic component in patients with AS. There is clear evidence that the pathogenic properties of HLA-B*27 depend on the subtype. Only subtle changes in the variation of a single amino acid in the HLA-B*27 molecule could induce a different tendency in the dimerization assembly [30]. In addition, pathogenic types such as HLA-B*27:05 showed prolonged binding to ERp57 and immunoglobulin protein compared with HLA-B*27:06, which is less associated with AS. A recent study has confirmed an association between the major histocompatibility complex (MHC) and amino acids in East Asians. HLA-C*15 showed the strongest risk association with AS, followed by HLA-B*40, under controlled HLA-B*27 in East Asians [31]. At the amino acid level, histidine at position 114 in HLA-B in uncontrolled analysis and lysine at position 70, followed by asparagine at position 97 in controlled HLA-B*27, showed the strongest association with AS. In a large cohort, there was a positive association between HLA-A*29,B*38,B*49,B*52,DRB1*11,andDPB1*03:01, and negative associations withHLA-B*07, B*57, DRB1*15:01, DQB1*02:01, and DQB1*06:02 in HLA-B*27 negative AS [32].
Although approximately 90% of patients with AS carry HLA-B*27, positivity for HLA-B*27 is not directly related to the manifestation of the disease, indicating that genes other than MHC are necessary for developing AS [33]. Endoplasmic reticulum aminopeptidases (ERAP)-1 and ERAP-2 are important for trimming peptides present in MHC class I molecules in the ER [34,35]. Aberrant function of ERAP-1 and ERAP-2 leads to increased ER stress and autophagy activation [36]. ERAP-1 highly interacts with HLA-B*27 [37] and HLA-B*40 [38] in patients with AS, and ERAP-2 is significantly associated with AS in patients who are either HLA-B*27 positive or HLA-B*27 negative [39]. The percentage of ERAP-1/ERAP-2 heterodimers was found to be 10%~30%, which means that ERAP-1 needs ERAP-2 for trimming longer amino acids, while the majority of ERAP1 and ERAP2 exist in the monomeric form [40]. Phenotypes of ERAP-1/ERAP-2 are diverse and act in distinct ways [41]. High-activity ERAP-1 is related to increased nanomers more than longer ligands compared with low-activity ERAP-2, while cells expressing ERAP-2 and ERAP-2 positivity in low-activity ERAP-1 were associated with lower amounts of peptides compared with ERAP-2 negativity in high-activity ERAP-1. RNA-seq validated the ERAP-1 and ERAP-2 polymorphisms are associated with gene expression, which influences protein expression levels. In addition, disease-associated isoforms of ERAP-1, ENST00000443439, and ENST00000296754 are regulated by genetic splice-interfering variant [42]. Thus, the function of ERAP-1 and ERAP-2 is influenced by their alleles and play pivotal roles in AS pathogenesis.
Inflammation is a discriminating feature of AS onset [4]. The enthesis is the area of tendons or ligaments attached to bones, and its role is to maintain stability and transduce mechanical forces [43]. Similar to human gait in the orthograde posture, sustained mechanical stress is imposed on the lumbar spine and lower extremities, which are susceptible to microdamage. Tenocytes are mainly composed of entheseal cells that transform into round chondroid cells near the bone and regulate mechanotransduction pathways to cope with biological stress [44]. When the enthesis is under mechanical stress, transforming growth factor-β (TGFβ) is induced and high concentration of TGFβ leads to tenocyte cell death [45]. Cell deaths generate toll-like receptor-danger-associated molecular patterns (DAMPs) and IL-1β activated by inflammasomes [46,47]. High-mobility group protein B1 acting as a DAMP was detected in inflamed tendon leading to production of IL-1β, IL-6, IL-33, CCL2, and CXCL12. IL-1β is a proinflammatory cytokine that initiates the molecular cascade and propagate inflammation by inhibiting tenogenic potential of in tendon progenitor cells [48,49]. Recent studies have revealed the existence of cytokine-producing cells in the entheseal zone of human tissues. In normal human enthesis and perientheseal bone, resident CD14+ myeloid cells are main producer of IL-23, IL-1β, tumor necrosis factor (TNF), and CCL20 in entheses [50]. In addition, γδT cells are found in human enthesis and perientheseal bone and produce IL-17A independently to IL-23 [51]. IL-23 is suggested to initiate AS because a rat experiment demonstrated that an IL-23 blocker completely inhibited the evolution of spondylitis and arthritis [52]. The population of resident endothelial cells may connect microdamage to the propagation of inflammation in patients with AS. Although the origin of immune cells is still debated, enthesial cells are suggested to be conductors that recruit cells from the inflamed gut or skin, resulting in the propagation of inflammation.
The proinflammatory cytokines IL-23 and IL-17 play key roles in host defense and function as barriers to mucosal surfaces [53,54]. Evidence of the association and dysregulation of the IL-23/IL-17 pathway with AS pathogenesis has been identified. Serum levels of IL-23 and IL-17 were significantly elevated in the peripheral blood of patients with AS, strongly associated with AS [55,56]. IL-23 is a cytokine consisting of subunits p19 and p40 produced by antigen-presenting cells, which induces IL-17 production by Th17 cells [57,58]. In addition to entheseal dwelling cells, which produce IL-23, resident Paneth cells are a crucial source of IL-23 because IL-23 expression is upregulated in the terminal ileum in patients with AS [59]. IL-23 could play a key role in the initiation of reactive arthritis, as early inhibition of IL-23p19 blocked arthritis in SKG mice infected with Chlamydia muridarum, but not in the late stage [60]. Since macrophages carry C. muridarum to the joint, depletion of macrophages reduced the dissemination of bacteria and development of arthritis, and inhibition of TNF at infection or the beginning of arthritis decreased joint inflammation, suggesting that macrophages from SKG mice could induce TNF production for constant inflammation of joints.
IL-17 is a signature cytokine secreted by Th17 cells [61]. Up-regulation of the IL-23 receptor with high expression of E receptor type 4 (EP4), a prostaglandin E2 receptor, promotes Th17 cell recruitment. Overexpression of EP4 in Th17cells is unique compared with RA or psoriatic arthritis (PsA) [62]. However, Th17 cells secreting IL-17 and contribution of IL-23 remain controversial [63]. IL-23 inhibition has less effect on AS. Thus, IL-23 independent production of IL-17 is thought to be an important pathway in the pathogenesis of AS [64]. In addition, there are several other cells producing IL-17: γδT cells [65], mucosal-associated invariant (MAIT) cells [66,67], type 3 innate lymphoid cells (ILC3) [68], CD4+ T cells [69], CD8+ T cells [70], CD3-CD56+ natural killer (NK) cells [71], and mast cells [72]. Cuthbert et al. [51] examined γδT cells and found that Vδ1 subset of γδT cells could produce IL-17 independent to IL-23 while Vδ2 subset expresses IL-23/IL-17 axis cytokine signaling. In this analysis, γδT cells were substantial source of IL-17A with IL-22 or TNF-α in addition to Th17cells. IL-17A production from MAIT cells was significantly higher than that of CD4+T cell, γδT cells, and CD8+T cells while expression of IL-17A by neutrophils could not reach the detection limit [73]. Upon induction with a combination of IL-7, IL-18, and anti-CD3/anti-CD28 antibodies, MAIT cells produced IL-17A, which was strongly independent of IL-23. Retinoid-related orphan receptor gamma (RORγt)-expressing γT cells produce IL-17 [74]. Oral RORγt inhibitor (PF-06747711) suppressing type-17 signaling pathway was shown to be effective in clinical manifestations such as bone loss, bone erosions, dermatitis, colitis, and weight loss in IL-23 overexpression mice [75].
In inflammation, IL-17 promotes neutrophilic inflammation by elevating expression of proinflammatory molecules such as TNF, IL-1α, IL-1β, IL-6, and IL-8. IL-17 also promotes neutrophil survival by producing granulocyte-colony stimulating factor (G-CSF) and granulocyte macrophage-CSF from IL-17-activated endothelial cells within microvessel wall [76,77]. In collaboration with TNF-α, IL-17A showed synergistic increase in neutrophil recruitment and endothelial activation [78]. IL-17 also induces inflammation by targeting fibroblasts and macrophages. In bone metabolism, IL-17 promotes osteoclast differentiation from its osteoclast precursor by direct stimulation of osteoclast and elevated expression of macrophage-colony stimulating factor (M-CSF) and receptor activator of nuclear factors κB ligand (RANKL) for osteoclast survival and differentiation [79]. Osteogenic differentiation and proliferation of mesenchymal stem cells (MSCs) are enhanced by IL-17 [80]. Thus, IL-17 is a crucial cytokine in AS progression.
Evidence of pathogenesis is largely associated with autoinflammation, depending on the genetic background. Although there is limited support for adaptive immunity in AS pathogenesis, evidence of adaptive immunity has accumulated in harmony with innate immunity.
The T-cell receptor (TCR) recognizes the peptides of antigens presented by MHC molecules, and this immune response mediates adaptive immunity. Complementary determining region 3 (CDR3) is responsible for antigen recognition [81]. CDR3a and CDR3b loops give a diversity of TCRβ as it is encoded with variable (V), diversity (D), and joining (J) genes. Based on this mechanism, immunosequencing of TCRβ diversity with peripheral blood mononuclear cells was performed in patients with AS with HLA-B27+ compared with healthy controls with HLA-B27+ [82]. AS has a higher diversity of TCR repertoire in CD4+ and CD8+ T cell populations, with a global reduction in peripheral T cell clonal expansion. In functional aspect, production of IFN-γ or TNF is reduced in CD4+ or CD8+ T cells, implicating disruption of TCR signaling cascade. In addition, expansion of the viral (Epstein-Barr virus and cytomegalovirus)-specific CD8 clonotype and public CD8 clonotype in AS with HLA-B27+ was detected, suggesting insufficient pathogen control due to an imbalanced regulatory mechanism [83]. TCR Rep-seq and CDR3 motif analyses showed that CD4+ and CD8+ T cells share the same CDR3 amino acid sequence, which could be explained by antigen stimulation [84]. During high disease activity in patients with AS, the diversity of terminally differentiated effector cells decreases and is inversely correlated with the disease activity. Thus, it could be deduced that disease progression could be accompanied by autoantigens.
There is a new clue for the recruitment of cytotoxic CD8+T cells to the joints as production of granzyme A, granzyme B, and granulysin was increased, while a decrease in perforin 1 production was incompatible with serum with reduced CD8+T cells in the blood. However, elevated cytotoxic T cells in synovial fluid implicate that these cells are important for joint inflammation in patients with AS [85].
NK cells are involved in the inflammatory process from initiation to remission. NK cells in autoimmune diseases play an important role in bridging innate and adaptive immunity [86]. There are altered subsets of AS. There was a significant reduction in CD3-CD56+NK cells in the peripheral blood mononuclear cells in patients with AS compared with those in the control group (4.95% versus 10.53%, p<0.001) [87]. Among NK subsets, the CD56dimNK cells were diminished which has killing capacity while CD56brightNK cells regulating lymphocytes were increased. In addition, cytotoxicity-related molecules, such as granzymes and GZMB+NK cells, were significantly reduced. In a previous study, expression of A20 which has anti-nuclear factor-κB effect was dramatically reduced in CD56brightNK cells in patients, whereas expression of A20 in CD56dimsubset had no difference compared with healthy control group [88]. Biologics in rheumatic diseases affect NK cell activity. TNF inhibitors (TNFi) showed good response rates in CD8+NK cell subsets [89]. However, treatment with secukinumab showed no change in NK cells in patients with AS [90]. An altered NK cell population could be a cell-based parameter for the selection of therapeutic agents.
Factors contributing to new bone formation have been recognized and may help identify new therapeutic targets. However, seizing the progression of new bone formation using current medications remains challenging. Inflammation of the sacroiliac joints and the spine leads to new bone formation and abnormalities that restrict spinal mobility. Resolution of inflammation is replaced by fat metaplasia, which was associated with ankylosis on T1-weighted magnetic resonance imaging (MRI) [91]. The fatty signal on MRI corresponds to adipocytes, which was found in 90.5% of patients with AS [92]. Vertebral corner inflammation and fat deposition are strongly associated with new bone formation on MRI [6]. However, 40% to 66% of new bone could develop without the detection of MRI inflammation or fat degeneration. A study showed that the subchondral bone plate was invaded by fibrous tissue originating from the bone marrow, resulting in thinning and degeneration of the cartilage and subchondral bone, while the trabecular bone was unaffected in patients with AS [93]. This fibrous tissue could play a critical role in bone remodeling. The same study group found that granulation tissue in the subchondral bone marrow could carry capabilities of bone formation, as osteoblasts are found in the lining of granulation tissue near the cartilage in the facet joints [94].
IL-22 is directly induced by IL-23 from Th17 cells, DCs, Th22 cells, γδT cells, and ILC3s and is associated with AS [95,96]. IL-22 in combination with IFN-γ and TNF promotes MSCs to activate bone formation via maintenance, proliferation and migration of MSCs and this process consequently results in new bone formation [7]. In vitro, MSCs treated with IL-22 produced more calcium than did the untreated MSCs. IL-22 produced by entheseal resident cells activates signal transducer and activator of transcription 3 (STAT3)-dependent osteoblast-mediated bone remodeling [96]. Thus, IL-22 plays a key role in new bone formation in patients with AS, as it affects the enthesis.
The IL-23/IL-17 pathway is influenced by the Janus kinase (JAK)/STAT pathway, and STAT3 mediates intracellular signaling in osteoblasts and osteoclast [97-99]. In a mouse model, a STAT3 inhibitor suppressed new bone formation comparable to that of an IL-17A blocker [100]. Osteogenesis is reduced in the spinal enthesis cells. Thus, STAT3 inhibitors are promising agents for targeting new bone formation and inflammation. Resident MSCs in the normal spinal enthesis promotes osteogenesis induced by IL-17A and TNF [101].
TNF is the important target in patients with AS and RA even though the immunological role of TNF is not fully elucidated. Unlike erosive arthritis in patients with RA, AS has distinctive features of new bone formation and TNF mechanism. The Relationship between osteoproliferation and TNF is still not fully understood. In AS synovial fluid, transmembrane TNF (tmTNF) was increased, while soluble TNF (sTNF) was decreased compared with RA synovial fluid, with decreased activity of A disintegrin and metalloproteinase or TNF-α converting enzyme [102]. Mice overexpressing tmTNF manifested spondylitis and peripheral arthritis with synovitis, enthesitis, and osteitis. In addition, TNF-receptor II could be essential for osteoproliferation in tmTNF signaling as tmTNF and sTNF have different affinity for subsets of TNF-receptors. Alkaline phosphatase and collagen type I expression in fibroblasts from tmTNF transgenic mice were significantly increased, and excessive mineralization was observed in the presence of IL-17A. Thus, tmTNF contributes to new bone formation in patients with AS and to the clinical features of the SpA family.
Macrophage migration inhibitory factor (MIF) is a proinflammatory cytokine that binds to its high-affinity receptor, CD74, on antigen-presenting cells, and the serum level of MIF is increased in patients with AS [103,104]. MIF was not affected by TNFi, and radiographic progression was associated with serum MIF levels (p=0.031) [105]. MIF signaling activates Wnt/β-catenin pathway by triggering stabilization of β-catenin, a key regulator of osteoblast differentiation and maturation. Wnt signaling is blocked by dickkof-related protein 1 (DKK1) and sclerostin. DKK1 is negatively associated with the formation of osteophytes and spinal ligament ossification, and sclerostin levels are lowered in patients with AS [106-108]. RANKL is essential for osteoclast differentiation and maturation of monocytes and macrophages regulated by CD16+ monocytes. It was found that the levels of CD16+ monocytes and osteoclasts decreased, which was associated with lower RANKL/osteoprotegerin (OPG) ratios in patients with AS [109]. RANKL SNPs were also associated with syndesmophyte formation in patients with AS [110]. Osteoimmunology, which refers to bone homeostasis affected by cytokines and immune system signaling pathways, is important for new bone formation in patients with AS [111].
TNFi have been successfully used over the past two decades as the mainstay treatment of AS. TNFi, including etanercept, infliximab, adalimumab, and golimumab showed similar response rates of approximately 60% according to the Assessment in Ankylosing Spondylitis Response Criteria(ASAS) 20 and 40% according to ASAS 40 [112-115]. All TNFi treatments improve pain, function, stiffness, and global well-being [116]. Spinal and sacroiliac joint inflammation detected on MRI also improved in the TNFi group. In cases of uveitis and IBD, TNF-α monoclonal antibodies are recommended over other biologic drugs because they have a lower incidence of disease recurrence and exacerbation [117]. However, it has been reported that TNFi induced psoriasis and paradoxical arthritis in IBD by dysregulating type I IFN [118,119]. Although there are no evident therapeutic options for new bone formation, TNFi can inhibit spinal radiographic progression if used for more than 4 years [120]. Unfortunately, it was not possible to demonstrate the effect of TNFi initiation in the advanced stages [121].
IL-23 blockers showed a lack of efficacy in patents with AS, whereas these drugs were beneficial for psoriasis [122,123]. Ustekinumab is a human monoclonal antibody that binds to the p40 subunit of IL-12 and IL-23. Clinical trials of AS failed to demonstrate improvements in symptoms and were discontinued [124,125]. According to a rat experiment, an IL-23 blocker was capable of suppressing disease onset, with no effect on inflammation [60]. In addition, the production of IL-17A is not solely dependent on the IL-23/IL-17A axis. Thus, blocking IL-23 may not inhibit the inflammation which is provoked through IL-17. Unlike AS, IL-23 blockers showed good efficacy in peripheral synovitis and enthesitis in psoriasis, and there was an improvement in spinal domain pain in PsA [126,127]. Whether there is efficacy in peripheral arthritis in patients with AS and the initiation of axial manifestations in PsA is still questionable.
Secukinumab was the first recombinant human monoclonal antibody that directly blocked IL-17A from binding to the IL-17 receptor [128]. Secukinumab achieved approximately 40% ASAS 40 and lowered C-reactive protein (CRP), SIJ edema scores on MRI, and quality of life at week 16 [129]. Histopathological examination of synovial inflammation showed that CD15+ neutrophils and CD56+ macrophages decreased from baseline 12weeks after beginning of secukinumab 300 mg [130]. Ixekizumab showed effects on joint improvement comparable to those of adalimumab [131]. In an extended study on PsA, ixekizumab showed persistent efficacy, safety, and inhibition of radiographic progression [132]. In addition to skeletal symptoms, IL-17A blockers showed improvement in the skin. However, there was a lack of efficacy in gut symptoms with aggravation or new-onset IBD [133]. In addition, secukinumab has an advantage in tuberculosis infections because there was no evidence of the incidence or reactivation of tuberculosis [134]. Brodalumab, an anti-IL-17 receptor A monoclonal antibody, showed ASAS 40/20 response rates of 43.8% and 67.5%, respectively, at week 16 in patients with active axSpA in a phase 3 trial [135]. In the extension trial, in which the placebo group switched to brodalumab at week 16, the placebo group did catch up with the brodalumab group in efficacy, with a sustained effect and clinical index. In addition, MRI showed an improvement in the Berlin Spine Score in both groups at week 68 [136]. Serious drug- and treatment-related adverse event included one case each of herpes zoster oticus, acute myocardial infarction, cellulitis, appendicitis, and diverticulitis. A study of monoclonal antibody neutralizing IL-17A and IL-17F, bimekizumab, showed that 46% of patients administered 160 mg and 320 mg every 4 weeks achieved ASAS 40 at week 12, and approximately 60% of patients responded at week 48 in phase IIb [137]. In the non-responder imputation analysis, the ratio of ASAS 40 and partial remission was 53.7% and 28.0%, respectively, with a gradual reduction in ASDAS at week 156 [138].
JAK inhibitors, tofacitinib and upadacitinib have been approved for AS by U.S. Food and Drug Administration. Upadacitinib, a selective JAK1 inhibitor, administered at 15 mg once daily was effective in AS refractory to a previous history of biologic disease-modifying antirheumatic drugs with ASAS 40 at 45% at week 14 in a phase 3 trial [139]. The incidence rate of serious infections was 2.4% consisting of 5 cases; 4 cases were coronavirus disease 2019-related infections and one case was uveitis. The zoster infection rate was 0.9%. Tofacitinib is a first-generation JAK inhibitor targeting JAK3, JAK1, and JAK2 [140]. Tofacitinib had response rate of 40.6% in the ASAS 40 at week 14 [141]. There were 2.3% non-serious herpes zoster cases and 0.8% serious infections. In the two studies on upadacitinib and tofacitinib, there were no major adverse cardiovascular events, thromboembolic events, malignancies, or deaths while there are still concerns about risks of major adverse cardiovascular events and cancer in RA.
Granulocyte-macrophage colony stimulating factor (GM-CSF) is a cytokine that could result in joint damage by inducing matrix metalloproteinases and osteoclasts and showed improved signs and symptoms of RA in a phase II trial [142,143]. Namilumab is a monoclonal antibody targeting GM-CSF, and a phase IIa study of namilumab with primary endpoint of ASAS 20 at week 12 is currently being assessed [144].
The neutrophil-to-lymphocyte ratio (NLR) and platelet-to-lymphocyte ratio (PLR) have a high possibility of utility, similar to the existing biomarkers erythrocyte sedimentation rate (ESR) and CRP [145]. The cutoff values were 3.669 for NLR and 242.466 for PLR, while the ESR was 22 mm/h and 1.980 mg/dL for CRP. Each parameter had a good predictive value for radiographic sacroiliitis, and NLR or PLR with existing inflammatory markers showed better outcomes for the effect of TNFi treatment. In addition, the lymphocyte-to-monocyte ratio, with a cutoff value of 4.26 showed a good predictor of radiologic progression in patients with AS (sensitivity, 94.9%; sensitivity, 97.4%; AUC, 0.975) [146].
Although HLA-B*27 has been widely used for the detection of AS, negative HLA-B*27 results can delay diagnosis and treatment initiation. Proteomic analysis could aid in identifying new targets for the early detection of the disease. In 2020, it was reported that eight AS-specific proteins were upregulated (>1.5-fold) in the synovial fluid of Korean patients with AS: HP, MMP1, MMP3, serum amyloid P-component, complement factor H-related protein 5 (CFHR5), mannose-binding lectin 2, complement component 9 (C9), and complement C4-A [147]. Among these, MMP3, C9, and CFHR5 have been identified as potential markers.
Autoantibodies are useful tools for distinguishing autoimmune diseases and verifying their disease activity. AS is considered an autoinflammatory disease; thus, it is difficult to classify patients with AS based on nonspecific chronic low back pain in the early disease phase. Novel antibodies and biomarkers have been explored for early detection of AS. Recently, antibodies against AS were identified. Three antibodies against axSpA peptides have been detected in the axSpA cohort at Hasselt University [148]. The three novel antibodies were tested in patients with axial SpA from the University Hospital Leuven (Bio)SPAR cohort. The other antibody is against CD74. The immunoglobulin (Ig) A anti-CD74 antibody is associated with radiographic changes in AS because CD74, an MHC class II invariant chain, leads to cell proliferation and TNFα production [149]. Anti-CD74 IgA showed potential diagnostic value, as the area under the ROC curve for the diagnosis of axSpA compared with fibromyalgia was 0.705, while there was no correlation with gut inflammation [150]. In addition, the anti-CD74 IgG antibody was associated with disease activity, whereas there was no difference between the ratios of early and late phases of the disease [151]. Thus, anti-CD74 antibodies may serve as good detectors with high specificity [152,153].
The latest evidence on the pathogenesis of AS provides new perceptions and clues into the unexplained mechanisms. AS is a complex network between the autoinflammatory and autoimmune systems (Figure 1). HLA-B*27 creates an environment susceptible to various stimuli such as infection and trauma. Consequently, the IL-23/IL-17 pathway is activated, and immune cells contribute to the perpetuation of joint inflammation. The cytokines generated by these mechanisms are involved in bone homeostasis, resulting in new bone formation, which is an authentic feature of AS. New therapeutic choices and diagnostic biomarkers are emerging against the background of new discoveries, and we look forward to personalized treatments for better outcomes.
REFERENCES
1. Generali E, Bose T, Selmi C, Voncken JW, Damoiseaux JGMC. 2018; Nature versus nurture in the spectrum of rheumatic diseases: classification of spondyloarthritis as autoimmune or autoinflammatory. Autoimmun Rev. 17:935–41. DOI: 10.1016/j.autrev.2018.04.002. PMID: 30005857.


2. Mauro D, Thomas R, Guggino G, Lories R, Brown MA, Ciccia F. 2021; Ankylosing spondylitis: an autoimmune or autoinflammatory disease? Nat Rev Rheumatol. 17:387–404. DOI: 10.1038/s41584-021-00625-y. PMID: 34113018.


3. Xiong Y, Cai M, Xu Y, Dong P, Chen H, He W, et al. 2022; Joint together: the etiology and pathogenesis of ankylosing spondylitis. Front Immunol. 13:996103. DOI: 10.3389/fimmu.2022.996103. PMID: 36325352. PMCID: PMC9619093.


4. Schett G, Lories RJ, D'Agostino MA, Elewaut D, Kirkham B, Soriano ER, et al. 2017; Enthesitis: from pathophysiology to treatment. Nat Rev Rheumatol. 13:731–41. DOI: 10.1038/nrrheum.2017.188. PMID: 29158573.


5. Baraliakos X, Heldmann F, Callhoff J, Listing J, Appelboom T, Brandt J, et al. 2014; Which spinal lesions are associated with new bone formation in patients with ankylosing spondylitis treated with anti-TNF agents? A long-term observational study using MRI and conventional radiography. Ann Rheum Dis. 73:1819–25. DOI: 10.1136/annrheumdis-2013-203425. PMID: 23852807.


6. Machado PM, Baraliakos X, van der Heijde D, Braun J, Landewé R. 2016; MRI vertebral corner inflammation followed by fat deposition is the strongest contributor to the development of new bone at the same vertebral corner: a multilevel longitudinal analysis in patients with ankylosing spondylitis. Ann Rheum Dis. 75:1486–93. DOI: 10.1136/annrheumdis-2015-208011. PMID: 26462728.


7. El-Zayadi AA, Jones EA, Churchman SM, Baboolal TG, Cuthbert RJ, El-Jawhari JJ, et al. 2017; Interleukin-22 drives the proliferation, migration and osteogenic differentiation of mesenchymal stem cells: a novel cytokine that could contribute to new bone formation in spondyloarthropathies. Rheumatology (Oxford). 56:488–93. DOI: 10.1093/rheumatology/kew384. PMID: 27940584.


8. Fan Y, Pedersen O. 2021; Gut microbiota in human metabolic health and disease. Nat Rev Microbiol. 19:55–71. DOI: 10.1038/s41579-020-0433-9. PMID: 32887946.


9. Valdes AM, Walter J, Segal E, Spector TD. 2018; Role of the gut microbiota in nutrition and health. BMJ. 361:k2179. DOI: 10.1136/bmj.k2179. PMID: 29899036. PMCID: PMC6000740.


10. Song ZY, Yuan D, Zhang SX. 2022; Role of the microbiome and its metabolites in ankylosing spondylitis. Front Immunol. 13:1010572. DOI: 10.3389/fimmu.2022.1010572. PMID: 36311749. PMCID: PMC9608452.


11. Ewing C, Ebringer R, Tribbick G, Geysen HM. 1990; Antibody activity in ankylosing spondylitis sera to two sites on HLA B27.1 at the MHC groove region (within sequence 65-85), and to a Klebsiella pneumoniae nitrogenase reductase peptide (within sequence 181-199). J Exp Med. 171:1635–47. DOI: 10.1084/jem.171.5.1635. PMID: 2185331. PMCID: PMC2187896.


12. Goodall JC, Wu C, Zhang Y, McNeill L, Ellis L, Saudek V, et al. 2010; Endoplasmic reticulum stress-induced transcription factor, CHOP, is crucial for dendritic cell IL-23 expression. Proc Natl Acad Sci U S A. 107:17698–703. DOI: 10.1073/pnas.1011736107. PMID: 20876114. PMCID: PMC2955096.


13. Long F, Wang T, Li Q, Xiong Y, Zeng Y. 2022; Association between Klebsiella pneumoniae and ankylosing spondylitis: a systematic review and meta-analysis. Int J Rheum Dis. 25:422–32. DOI: 10.1111/1756-185X.14283. PMID: 35019225.
14. Schwimmbeck PL, Oldstone MB. 1988; Molecular mimicry between human leukocyte antigen B27 and Klebsiella. Consequences for spondyloarthropathies. Am J Med. 85(6A):51–3. DOI: 10.1016/0002-9343(88)90385-3. PMID: 2462350.
15. Asquith M, Sternes PR, Costello ME, Karstens L, Diamond S, Martin TM, et al. 2019; HLA alleles associated with risk of ankylosing spondylitis and rheumatoid arthritis influence the gut microbiome. Arthritis Rheumatol. 71:1642–50. DOI: 10.1002/art.40917. PMID: 31038287.


16. Costello ME, Ciccia F, Willner D, Warrington N, Robinson PC, Gardiner B, et al. 2015; Brief report: intestinal dysbiosis in ankylosing spondylitis. Arthritis Rheumatol. 67:686–91. DOI: 10.1002/art.38967. PMID: 25417597.


17. Tito RY, Cypers H, Joossens M, Varkas G, Van Praet L, Glorieus E, et al. 2017; Brief report: Dialister as a microbial marker of disease activity in spondyloarthritis. Arthritis Rheumatol. 69:114–21. DOI: 10.1002/art.39802. PMID: 27390077.
18. Breban M, Tap J, Leboime A, Said-Nahal R, Langella P, Chiocchia G, et al. 2017; Faecal microbiota study reveals specific dysbiosis in spondyloarthritis. Ann Rheum Dis. 76:1614–22. DOI: 10.1136/annrheumdis-2016-211064. PMID: 28606969.


19. Zhou C, Zhao H, Xiao XY, Chen BD, Guo RJ, Wang Q, et al. 2020; Metagenomic profiling of the pro-inflammatory gut microbiota in ankylosing spondylitis. J Autoimmun. 107:102360. DOI: 10.1016/j.jaut.2019.102360. PMID: 31806420.


20. Berland M, Meslier V, Berreira Ibraim S, Le Chatelier E, Pons N, Maziers N, et al. 2023; Both disease activity and HLA-B27 status are associated with gut microbiome dysbiosis in spondyloarthritis patients. Arthritis Rheumatol. 75:41–52. DOI: 10.1002/art.42289. PMID: 35818337. PMCID: PMC10099252.


21. Guggino G, Mauro D, Rizzo A, Alessandro R, Raimondo S, Bergot AS, et al. 2021; Inflammasome activation in ankylosing spondylitis is associated with gut dysbiosis. Arthritis Rheumatol. 73:1189–99. DOI: 10.1002/art.41644. PMID: 33452867.


22. Qaiyum Z, Gracey E, Yao Y, Inman RD. 2019; Integrin and transcriptomic profiles identify a distinctive synovial CD8+ T cell subpopulation in spondyloarthritis. Ann Rheum Dis. 78:1566–75. DOI: 10.1136/annrheumdis-2019-215349. PMID: 31471299.


23. Strauch UG, Mueller RC, Li XY, Cernadas M, Higgins JM, Binion DG, et al. 2001; Integrin alpha E(CD103)beta 7 mediates adhesion to intestinal microvascular endothelial cell lines via an E-cadherin-independent interaction. J Immunol. 166:3506–14. DOI: 10.4049/jimmunol.166.5.3506. PMID: 11207310.
24. Purwar R, Campbell J, Murphy G, Richards WG, Clark RA, Kupper TS. 2011; Resident memory T cells (T(RM)) are abundant in human lung: diversity, function, and antigen specificity. PLoS One. 6:e16245. DOI: 10.1371/journal.pone.0016245. PMID: 21298112. PMCID: PMC3027667.


25. Berlinberg AJ, Regner EH, Stahly A, Brar A, Reisz JA, Gerich ME, et al. 2021; Multi 'omics analysis of intestinal tissue in ankylosing spondylitis identifies alterations in the tryptophan metabolism pathway. Front Immunol. 12:587119. DOI: 10.3389/fimmu.2021.587119. PMID: 33746944. PMCID: PMC7966505.


26. Wei JC, Chou MC, Huang JY, Chang R, Hung YM. 2020; The association between Candida infection and ankylosing spondylitis: a population-based matched cohort study. Curr Med Res Opin. 36:2063–9. DOI: 10.1080/03007995.2020.1838460. PMID: 33066709.
27. Wei CY, Lin JY, Wang YT, Huang JY, Wei JC, Chiou JY. 2020; Risk of ankylosing spondylitis following human papillomavirus infection: a nationwide, population-based, cohort study. J Autoimmun. 113:102482. DOI: 10.1016/j.jaut.2020.102482. PMID: 32417193.


28. Segal Y, Calabrò M, Kanduc D, Shoenfeld Y. 2017; Human papilloma virus and lupus: the virus, the vaccine and the disease. Curr Opin Rheumatol. 29:331–42. DOI: 10.1097/BOR.0000000000000398. PMID: 28394823.


29. Damba JJ, Laskine M, Jin Y, Sinyavskaya L, Durand M. 2021; Incidence of autoimmune diseases in people living with HIV compared to a matched population: a cohort study. Clin Rheumatol. 40:2439–45. DOI: 10.1007/s10067-020-05500-x. PMID: 33230683.


30. Guiliano DB, North H, Panayoitou E, Campbell EC, McHugh K, Cooke FG, et al. 2017; Polymorphisms in the F pocket of HLA-B27 subtypes strongly affect assembly, chaperone interactions, and heavy-chain misfolding. Arthritis Rheumatol. 69:610–21. DOI: 10.1002/art.39948. PMID: 27723268.


31. Wang G, Kim TH, Li Z, Cortes A, Kim K, Bang SY, et al. 2020; MHC associations of ankylosing spondylitis in East Asians are complex and involve non-HLA-B27 HLA contributions. Arthritis Res Ther. 22:74. DOI: 10.1186/s13075-020-02148-5. PMID: 32272966. PMCID: PMC7146985.


32. Reveille JD, Zhou X, Lee M, Weisman MH, Yi L, Gensler LS, et al. 2019; HLA class I and II alleles in susceptibility to ankylosing spondylitis. Ann Rheum Dis. 78:66–73. DOI: 10.1136/annrheumdis-2018-213779. PMID: 30341055. PMCID: PMC6982366.


33. Reveille JD. 2006; The genetic basis of ankylosing spondylitis. Curr Opin Rheumatol. 18:332–41. DOI: 10.1097/01.bor.0000231899.81677.04. PMID: 16763451.


34. York IA, Chang SC, Saric T, Keys JA, Favreau JM, Goldberg AL, et al. 2002; The ER aminopeptidase ERAP1 enhances or limits antigen presentation by trimming epitopes to 8-9 residues. Nat Immunol. 3:1177–84. DOI: 10.1038/ni860. PMID: 12436110.


35. Tsui FW, Haroon N, Reveille JD, Rahman P, Chiu B, Tsui HW, et al. 2010; Association of an ERAP1 ERAP2 haplotype with familial ankylosing spondylitis. Ann Rheum Dis. 69:733–6. DOI: 10.1136/ard.2008.103804. PMID: 19433412.


36. Nakamura A, Boroojeni SF, Haroon N. 2021; Aberrant antigen processing and presentation: key pathogenic factors leading to immune activation in Ankylosing spondylitis. Semin Immunopathol. 43:245–53. DOI: 10.1007/s00281-020-00833-w. PMID: 33532928.


37. Evans DM, Spencer CC, Pointon JJ, Su Z, Harvey D, Kochan G, et al. 2011; Interaction between ERAP1 and HLA-B27 in ankylosing spondylitis implicates peptide handling in the mechanism for HLA-B27 in disease susceptibility. Nat Genet. 43:761–7. Erratum in: Nat Genet 2011;43:919. DOI: 10.1038/ng0911-919a. PMID: 21743469. PMCID: PMC3640413.
38. Wei JC, Tsai WC, Lin HS, Tsai CY, Chou CT. 2004; HLA-B60 and B61 are strongly associated with ankylosing spondylitis in HLA-B27-negative Taiwan Chinese patients. Rheumatology (Oxford). 43:839–42. DOI: 10.1093/rheumatology/keh193. PMID: 15113995.


39. Robinson PC, Costello ME, Leo P, Bradbury LA, Hollis K, Cortes A, et al. 2015; ERAP2 is associated with ankylosing spondylitis in HLA-B27-positive and HLA-B27-negative patients. Ann Rheum Dis. 74:1627–9. DOI: 10.1136/annrheumdis-2015-207416. PMID: 25917849. PMCID: PMC4498996.
40. Saveanu L, Carroll O, Lindo V, Del Val M, Lopez D, Lepelletier Y, et al. 2005; Concerted peptide trimming by human ERAP1 and ERAP2 aminopeptidase complexes in the endoplasmic reticulum. Nat Immunol. 6:689–97. DOI: 10.1038/ni1208. PMID: 15908954.


41. Martín-Esteban A, Sanz-Bravo A, Guasp P, Barnea E, Admon A, López de Castro JA. 2017; Separate effects of the ankylosing spondylitis associated ERAP1 and ERAP2 aminopeptidases determine the influence of their combined phenotype on the HLA-B*27 peptidome. J Autoimmun. 79:28–38. DOI: 10.1016/j.jaut.2016.12.008. PMID: 28063628.


42. Hanson AL, Cuddihy T, Haynes K, Loo D, Morton CJ, Oppermann U, et al. 2018; Genetic variants in ERAP1 and ERAP2 associated with immune-mediated diseases influence protein expression and the isoform profile. Arthritis Rheumatol. 70:255–65. DOI: 10.1002/art.40369. PMID: 29108111.
43. Gracey E, Burssens A, Cambré I, Schett G, Lories R, McInnes IB, et al. 2020; Tendon and ligament mechanical loading in the pathogenesis of inflammatory arthritis. Nat Rev Rheumatol. 16:193–207. DOI: 10.1038/s41584-019-0364-x. PMID: 32080619. PMCID: PMC7815340.


44. Lavagnino M, Wall ME, Little D, Banes AJ, Guilak F, Arnoczky SP. 2015; Tendon mechanobiology: current knowledge and future research opportunities. J Orthop Res. 33:813–22. DOI: 10.1002/jor.22871. PMID: 25763779. PMCID: PMC4524513.
45. Maeda T, Sakabe T, Sunaga A, Sakai K, Rivera AL, Keene DR, et al. 2011; Conversion of mechanical force into TGF-β-mediated biochemical signals. Curr Biol. 21:933–41. DOI: 10.1016/j.cub.2011.04.007. PMID: 21600772. PMCID: PMC3118584.


46. Bergsbaken T, Fink SL, Cookson BT. 2009; Pyroptosis: host cell death and inflammation. Nat Rev Microbiol. 7:99–109. DOI: 10.1038/nrmicro2070. PMID: 19148178. PMCID: PMC2910423.


47. Akbar M, Gilchrist DS, Kitson SM, Nelis B, Crowe LAN, Garcia-Melchor E, et al. 2017; Targeting danger molecules in tendinopathy: the HMGB1/TLR4 axis. RMD Open. 3:e000456. DOI: 10.1136/rmdopen-2017-000456. PMID: 28879051. PMCID: PMC5574425.


48. Zhang K, Asai S, Yu B, Enomoto-Iwamoto M. 2015; IL-1β irreversibly inhibits tenogenic differentiation and alters metabolism in injured tendon-derived progenitor cells in vitro. Biochem Biophys Res Commun. 463:667–72. DOI: 10.1016/j.bbrc.2015.05.122. PMID: 26051275. PMCID: PMC4496264.
49. Tsuzaki M, Guyton G, Garrett W, Archambault JM, Herzog W, Almekinders L, et al. 2003; IL-1 beta induces COX2, MMP-1, -3 and -13, ADAMTS-4, IL-1 beta and IL-6 in human tendon cells. J Orthop Res. 21:256–64. DOI: 10.1016/S0736-0266(02)00141-9. PMID: 12568957.
50. Bridgewood C, Watad A, Russell T, Palmer TM, Marzo-Ortega H, Khan A, et al. 2019; Identification of myeloid cells in the human enthesis as the main source of local IL-23 production. Ann Rheum Dis. 78:929–33. DOI: 10.1136/annrheumdis-2018-214944. PMID: 31018959. PMCID: PMC6585277.


51. Cuthbert RJ, Watad A, Fragkakis EM, Dunsmuir R, Loughenbury P, Khan A, et al. 2019; Evidence that tissue resident human enthesis γδT-cells can produce IL-17A independently of IL-23R transcript expression. Ann Rheum Dis. 78:1559–65. DOI: 10.1136/annrheumdis-2019-215210. PMID: 31530557. PMCID: PMC6837256.


52. van Tok MN, Na S, Lao CR, Alvi M, Pots D, van de Sande MGH, et al. 2018; The initiation, but not the persistence, of experimental spondyloarthritis is dependent on interleukin-23 signaling. Front Immunol. 9:1550. DOI: 10.3389/fimmu.2018.01550. PMID: 30038617. PMCID: PMC6046377.


53. Tsukazaki H, Kaito T. 2020; The role of the IL-23/IL-17 pathway in the pathogenesis of spondyloarthritis. Int J Mol Sci. 21:6401. DOI: 10.3390/ijms21176401. PMID: 32899140. PMCID: PMC7504446.


54. Hamada S, Umemura M, Shiono T, Tanaka K, Yahagi A, Begum MD, et al. 2008; IL-17A produced by gammadelta T cells plays a critical role in innate immunity against listeria monocytogenes infection in the liver. J Immunol. 181:3456–63. DOI: 10.4049/jimmunol.181.5.3456. PMID: 18714018. PMCID: PMC2859669.
55. Wang X, Lin Z, Wei Q, Jiang Y, Gu J. 2009; Expression of IL-23 and IL-17 and effect of IL-23 on IL-17 production in ankylosing spondylitis. Rheumatol Int. 29:1343–7. DOI: 10.1007/s00296-009-0883-x. PMID: 19247658.


56. Mei Y, Pan F, Gao J, Ge R, Duan Z, Zeng Z, et al. 2011; Increased serum IL-17 and IL-23 in the patient with ankylosing spondylitis. Clin Rheumatol. 30:269–73. DOI: 10.1007/s10067-010-1647-4. PMID: 21161669.


57. Aggarwal S, Ghilardi N, Xie MH, de Sauvage FJ, Gurney AL. 2003; Interleukin-23 promotes a distinct CD4 T cell activation state characterized by the production of interleukin-17. J Biol Chem. 278:1910–4. DOI: 10.1074/jbc.M207577200. PMID: 12417590.


58. Oppmann B, Lesley R, Blom B, Timans JC, Xu Y, Hunte B, et al. 2000; Novel p19 protein engages IL-12p40 to form a cytokine, IL-23, with biological activities similar as well as distinct from IL-12. Immunity. 13:715–25. DOI: 10.1016/S1074-7613(00)00070-4. PMID: 11114383.


59. Ciccia F, Bombardieri M, Principato A, Giardina A, Tripodo C, Porcasi R, et al. 2009; Overexpression of interleukin-23, but not interleukin-17, as an immunologic signature of subclinical intestinal inflammation in ankylosing spondylitis. Arthritis Rheum. 60:955–65. DOI: 10.1002/art.24389. PMID: 19333939.


60. Romand X, Liu X, Rahman MA, Bhuyan ZA, Douillard C, Kedia RA, et al. 2021; Mediation of interleukin-23 and tumor necrosis factor-driven reactive arthritis by Chlamydia-infected macrophages in SKG mice. Arthritis Rheumatol. 73:1200–10. DOI: 10.1002/art.41653. PMID: 33452873.
61. Harrington LE, Hatton RD, Mangan PR, Turner H, Murphy TL, Murphy KM, et al. 2005; Interleukin 17-producing CD4+ effector T cells develop via a lineage distinct from the T helper type 1 and 2 lineages. Nat Immunol. 6:1123–32. DOI: 10.1038/ni1254. PMID: 16200070.


62. Klasen C, Meyer A, Wittekind PS, Waqué I, Nabhani S, Kofler DM. 2019; Prostaglandin receptor EP4 expression by Th17 cells is associated with high disease activity in ankylosing spondylitis. Arthritis Res Ther. 21:159. DOI: 10.1186/s13075-019-1948-1. PMID: 31253169. PMCID: PMC6599260.


63. Schinocca C, Rizzo C, Fasano S, Grasso G, La Barbera L, Ciccia F, et al. 2021; Role of the IL-23/IL-17 pathway in rheumatic diseases: an overview. Front Immunol. 12:637829. DOI: 10.3389/fimmu.2021.637829. PMID: 33692806. PMCID: PMC7937623.


64. Baeten D, Adamopoulos IE. 2021; IL-23 inhibition in ankylosing spondylitis: where did it go wrong? Front Immunol. 11:623874. DOI: 10.3389/fimmu.2020.623874. PMID: 33679714. PMCID: PMC7935519.


65. Roark CL, Simonian PL, Fontenot AP, Born WK, O'Brien RL. 2008; gammadelta T cells: an important source of IL-17. Curr Opin Immunol. 20:353–7. DOI: 10.1016/j.coi.2008.03.006. PMID: 18439808. PMCID: PMC2601685.
66. Gracey E, Qaiyum Z, Almaghlouth I, Lawson D, Karki S, Avvaru N, et al. 2016; IL-7 primes IL-17 in mucosal-associated invariant T (MAIT) cells, which contribute to the Th17-axis in ankylosing spondylitis. Ann Rheum Dis. 75:2124–32. DOI: 10.1136/annrheumdis-2015-208902. PMID: 27165176.


67. Toussirot É, Laheurte C, Gaugler B, Gabriel D, Saas P. 2018; Increased IL-22- and IL-17A-producing mucosal-associated invariant T cells in the peripheral blood of patients with ankylosing spondylitis. Front Immunol. 9:1610. DOI: 10.3389/fimmu.2018.01610. PMID: 30057583. PMCID: PMC6053500.


68. Cuthbert RJ, Fragkakis EM, Dunsmuir R, Li Z, Coles M, Marzo-Ortega H, et al. 2017; Brief report: group 3 innate lymphoid cells in human enthesis. Arthritis Rheumatol. 69:1816–22. DOI: 10.1002/art.40150. PMID: 28511289.


69. Park H, Li Z, Yang XO, Chang SH, Nurieva R, Wang YH, et al. 2005; A distinct lineage of CD4 T cells regulates tissue inflammation by producing interleukin 17. Nat Immunol. 6:1133–41. DOI: 10.1038/ni1261. PMID: 16200068. PMCID: PMC1618871.


70. Wang C, Liao Q, Hu Y, Zhong D. 2015; T lymphocyte subset imbalances in patients contribute to ankylosing spondylitis. Exp Ther Med. 9:250–6. DOI: 10.3892/etm.2014.2046. PMID: 25452811. PMCID: PMC4247318.


71. Chowdhury AC, Chaurasia S, Mishra SK, Aggarwal A, Misra R. 2017; IL-17 and IFN-γ producing NK and γδ-T cells are preferentially expanded in synovial fluid of patients with reactive arthritis and undifferentiated spondyloarthritis. Clin Immunol. 183:207–12. DOI: 10.1016/j.clim.2017.03.016. PMID: 28390966.


72. Noordenbos T, Yeremenko N, Gofita I, van de Sande M, Tak PP, Caňete JD, et al. 2012; Interleukin-17-positive mast cells contribute to synovial inflammation in spondylarthritis. Arthritis Rheum. 64:99–109. DOI: 10.1002/art.33396. PMID: 21968742.


73. Rosine N, Rowe H, Koturan S, Yahia-Cherbal H, Leloup C, Watad A, et al. 2022; Characterization of blood mucosal-associated invariant T cells in patients with axial spondyloarthritis and of resident mucosal-associated invariant T cells from the axial entheses of non-axial spondyloarthritis control patients. Arthritis Rheumatol. 74:1786–95. DOI: 10.1002/art.42090. PMID: 35166073. PMCID: PMC9825958.
74. Venken K, Jacques P, Mortier C, Labadia ME, Decruy T, Coudenys J, et al. 2019; RORγt inhibition selectively targets IL-17 producing iNKT and γδ-T cells enriched in Spondyloarthritis patients. Nat Commun. 10:9. DOI: 10.1038/s41467-018-07911-6. PMID: 30602780. PMCID: PMC6315029.


75. Mortier C, Gracey E, Coudenys J, Manuello T, Decruy T, Maelegheer M, et al. 2023; Jan. 20. RORγt inhibition ameliorates IL-23 driven experimental Psoriatic Arthritis by predominantly modulating γδ-T cells. Rheumatology (Oxford). [Epub]. DOI:10.1093/rheumatology/kead022. DOI: 10.1093/rheumatology/kead022. PMID: 36661300.


76. Liu R, Lauridsen HM, Amezquita RA, Pierce RW, Jane-Wit D, Fang C, et al. 2016; IL-17 promotes neutrophil-mediated immunity by activating microvascular pericytes and not endothelium. J Immunol. 197:2400–8. DOI: 10.4049/jimmunol.1600138. PMID: 27534549. PMCID: PMC5010945.


77. Lubberts E. 2015; The IL-23-IL-17 axis in inflammatory arthritis. Nat Rev Rheumatol. 11:415–29. Erratum in: Nat Rev Rheumatol 2015;11:562. DOI: 10.1038/nrrheum.2015.53. PMID: 25907700.


78. Griffin GK, Newton G, Tarrio ML, Bu DX, Maganto-Garcia E, Azcutia V, et al. 2012; IL-17 and TNF-α sustain neutrophil recruitment during inflammation through synergistic effects on endothelial activation. J Immunol. 188:6287–99. DOI: 10.4049/jimmunol.1200385. PMID: 22566565. PMCID: PMC3370121.


79. Sato K, Suematsu A, Okamoto K, Yamaguchi A, Morishita Y, Kadono Y, et al. 2006; Th17 functions as an osteoclastogenic helper T cell subset that links T cell activation and bone destruction. J Exp Med. 203:2673–82. DOI: 10.1084/jem.20061775. PMID: 17088434. PMCID: PMC2118166.


80. Huang H, Kim HJ, Chang EJ, Lee ZH, Hwang SJ, Kim HM, et al. 2009; IL-17 stimulates the proliferation and differentiation of human mesenchymal stem cells: implications for bone remodeling. Cell Death Differ. 16:1332–43. DOI: 10.1038/cdd.2009.74. PMID: 19543237.


81. Garcia KC, Gapin L, Adams JJ, Birnbaum ME, Scott-Browne JP, Kappler JW, et al. 2012; A closer look at TCR germline recognition. Immunity. 36:887–8. DOI: 10.1016/j.immuni.2012.05.018. PMID: 22705103. PMCID: PMC4237060.


82. Hanson AL, Nel HJ, Bradbury L, Phipps J, Thomas R, Lê Cao KA, et al. 2020; Altered repertoire diversity and disease-associated clonal expansions revealed by T cell receptor immunosequencing in ankylosing spondylitis patients. Arthritis Rheumatol. 72:1289–302. DOI: 10.1002/art.41252. PMID: 32162785.


83. Kok L, Masopust D, Schumacher TN. 2022; The precursors of CD8+ tissue resident memory T cells: from lymphoid organs to infected tissues. Nat Rev Immunol. 22:283–93. DOI: 10.1038/s41577-021-00590-3. PMID: 34480118. PMCID: PMC8415193.


84. Zheng M, Zhang X, Zhou Y, Tang J, Han Q, Zhang Y, et al. 2019; TCR repertoire and CDR3 motif analyses depict the role of αβ T cells in Ankylosing spondylitis. EBioMedicine. 47:414–26. DOI: 10.1016/j.ebiom.2019.07.032. PMID: 31477563. PMCID: PMC6796593.


85. Gracey E, Yao Y, Qaiyum Z, Lim M, Tang M, Inman RD. 2020; Altered cytotoxicity profile of CD8+ T cells in ankylosing spondylitis. Arthritis Rheumatol. 72:428–34. DOI: 10.1002/art.41129. PMID: 31599089.
86. Kucuksezer UC, Aktas Cetin E, Esen F, Tahrali I, Akdeniz N, Gelmez MY, et al. 2021; The role of natural killer cells in autoimmune diseases. Front Immunol. 12:622306. DOI: 10.3389/fimmu.2021.622306. PMID: 33717125. PMCID: PMC7947192.


87. Ren C, Li M, Zheng Y, Cai B, Du W, Zhang H, et al. 2022; Single-cell RNA-seq reveals altered NK cell subsets and reduced levels of cytotoxic molecules in patients with ankylosing spondylitis. J Cell Mol Med. 26:1071–82. DOI: 10.1111/jcmm.17159. PMID: 34994057. PMCID: PMC8831943.


88. Yang M, Zhou Y, Liu L, Wang S, Jiang J, Shang Q, et al. 2019; Decreased A20 expression on circulating CD56bright NK cells contributes to a worse disease status in patients with ankylosing spondylitis. Clin Exp Immunol. 198:1–10. DOI: 10.1111/cei.13341. PMID: 31206174. PMCID: PMC6718289.


89. Schulte-Wrede U, Sörensen T, Grün JR, Häupl T, Hirseland H, Steinbrich-Zöllner M, et al. 2018; An explorative study on deep profiling of peripheral leukocytes to identify predictors for responsiveness to anti-tumour necrosis factor alpha therapies in ankylosing spondylitis: natural killer cells in focus. Arthritis Res Ther. 20:191. DOI: 10.1186/s13075-018-1692-y. PMID: 30157966. PMCID: PMC6116509.


90. Jiang Y, Yang M, Zhang Y, Huang Y, Wu J, Xie Y, et al. 2021; Dynamics of adaptive immune cell and NK cell subsets in patients with ankylosing spondylitis after IL-17A inhibition by secukinumab. Front Pharmacol. 12:738316. DOI: 10.3389/fphar.2021.738316. PMID: 34721027. PMCID: PMC8551761.


91. Maksymowych WP, Wichuk S, Chiowchanwisawakit P, Lambert RG, Pedersen SJ. 2014; Fat metaplasia and backfill are key intermediaries in the development of sacroiliac joint ankylosis in patients with ankylosing spondylitis. Arthritis Rheumatol. 66:2958–67. DOI: 10.1002/art.38792. PMID: 25047851.


92. Baraliakos X, Boehm H, Bahrami R, Samir A, Schett G, Luber M, et al. 2019; What constitutes the fat signal detected by MRI in the spine of patients with ankylosing spondylitis? A prospective study based on biopsies obtained during planned spinal osteotomy to correct hyperkyphosis or spinal stenosis. Ann Rheum Dis. 78:1220–5. DOI: 10.1136/annrheumdis-2018-214983. PMID: 31122911.


93. Bleil J, Maier R, Hempfing A, Schlichting U, Appel H, Sieper J, et al. 2014; Histomorphologic and histomorphometric characteristics of zygapophyseal joint remodeling in ankylosing spondylitis. Arthritis Rheumatol. 66:1745–54. DOI: 10.1002/art.38404. PMID: 24574301.


94. Bleil J, Maier R, Hempfing A, Sieper J, Appel H, Syrbe U. 2016; Granulation tissue eroding the subchondral bone also promotes new bone formation in ankylosing spondylitis. Arthritis Rheumatol. 68:2456–65. DOI: 10.1002/art.39715. PMID: 27111225.


95. Rahman P, Inman RD, Gladman DD, Reeve JP, Peddle L, Maksymowych WP. 2008; Association of interleukin-23 receptor variants with ankylosing spondylitis. Arthritis Rheum. 58:1020–5. DOI: 10.1002/art.23389. PMID: 18383363.


96. Sherlock JP, Joyce-Shaikh B, Turner SP, Chao CC, Sathe M, Grein J, et al. 2012; IL-23 induces spondyloarthropathy by acting on ROR-γt+ CD3+CD4-CD8- entheseal resident T cells. Nat Med. 18:1069–76. DOI: 10.1038/nm.2817. PMID: 22772566.


97. Langrish CL, McKenzie BS, Wilson NJ, de Waal Malefyt R, Kastelein RA, Cua DJ. 2004; IL-12 and IL-23: master regulators of innate and adaptive immunity. Immunol Rev. 202:96–105. DOI: 10.1111/j.0105-2896.2004.00214.x. PMID: 15546388.


98. Veale DJ, McGonagle D, McInnes IB, Krueger JG, Ritchlin CT, Elewaut D, et al. 2019; The rationale for Janus kinase inhibitors for the treatment of spondyloarthritis. Rheumatology (Oxford). 58:197–205. DOI: 10.1093/rheumatology/key070. PMID: 29618084. PMCID: PMC6343466.


99. Li CH, Xu LL, Jian LL, Yu RH, Zhao JX, Sun L, et al. 2018; Stattic inhibits RANKL-mediated osteoclastogenesis by suppressing activation of STAT3 and NF-κB pathways. Int Immunopharmacol. 58:136–44. DOI: 10.1016/j.intimp.2018.03.021. PMID: 29587202.


100. Jo S, Won EJ, Kim MJ, Lee YJ, Jin SH, Park PR, et al. 2021; STAT3 phosphorylation inhibition for treating inflammation and new bone formation in ankylosing spondylitis. Rheumatology (Oxford). 60:3923–35. DOI: 10.1093/rheumatology/keaa846. PMID: 33237331.


101. Russell T, Watad A, Bridgewood C, Rowe H, Khan A, Rao A, et al. 2021; IL-17A and TNF modulate normal human spinal entheseal bone and soft tissue mesenchymal stem cell osteogenesis, adipogenesis, and stromal function. Cells. 10:341. DOI: 10.3390/cells10020341. PMID: 33562025. PMCID: PMC7915379.


102. Kaaij MH, van Tok MN, Blijdorp IC, Ambarus CA, Stock M, Pots D, et al. 2020; Transmembrane TNF drives osteoproliferative joint inflammation reminiscent of human spondyloarthritis. J Exp Med. 217:e20200288. DOI: 10.1084/jem.20200288. PMID: 32662821. PMCID: PMC7537402.


103. Leng L, Metz CN, Fang Y, Xu J, Donnelly S, Baugh J, et al. 2003; MIF signal transduction initiated by binding to CD74. J Exp Med. 197:1467–76. DOI: 10.1084/jem.20030286. PMID: 12782713. PMCID: PMC2193907.


104. Kozaci LD, Sari I, Alacacioglu A, Akar S, Akkoc N. 2010; Evaluation of inflammation and oxidative stress in ankylosing spondylitis: a role for macrophage migration inhibitory factor. Mod Rheumatol. 20:34–9. DOI: 10.3109/s10165-009-0230-9. PMID: 19787418.


105. Ranganathan V, Ciccia F, Zeng F, Sari I, Guggino G, Muralitharan J, et al. 2017; Macrophage migration inhibitory factor induces inflammation and predicts spinal progression in ankylosing spondylitis. Arthritis Rheumatol. 69:1796–806. DOI: 10.1002/art.40175. PMID: 28597514.


106. Heiland GR, Appel H, Poddubnyy D, Zwerina J, Hueber A, Haibel H, et al. 2012; High level of functional dickkopf-1 predicts protection from syndesmophyte formation in patients with ankylosing spondylitis. Ann Rheum Dis. 71:572–4. DOI: 10.1136/annrheumdis-2011-200216. PMID: 22186710.


107. Uderhardt S, Diarra D, Katzenbeisser J, David JP, Zwerina J, Richards W, et al. 2010; Blockade of Dickkopf (DKK)-1 induces fusion of sacroiliac joints. Ann Rheum Dis. 69:592–7. DOI: 10.1136/ard.2008.102046. PMID: 19304568.


108. Aschermann S, Englbrecht M, Bergua A, Spriewald BM, Said-Nahal R, Breban M, et al. 2016; Presence of HLA-B27 is associated with changes of serum levels of mediators of the Wnt and hedgehog pathway. Joint Bone Spine. 83:43–6. DOI: 10.1016/j.jbspin.2015.03.019. PMID: 26494593.


109. Caparbo VF, Saad CGS, Moraes JC, de Brum-Fernandes AJ, Pereira RMR. 2018; Monocytes from male patients with ankylosing spondylitis display decreased osteoclastogenesis and decreased RANKL/OPG ratio. Osteoporos Int. 29:2565–73. DOI: 10.1007/s00198-018-4629-z. PMID: 30006885.


110. Wang CM, Tsai SC, Lin JC, Wu YJ, Wu J, Chen JY. 2019; Association of genetic variants of RANK, RANKL, and OPG with ankylosing spondylitis clinical features in Taiwanese. Mediators Inflamm. 2019:8029863. DOI: 10.1155/2019/8029863. PMID: 31015798. PMCID: PMC6446096.
111. Dar HY, Azam Z, Anupam R, Mondal RK, Srivastava RK. 2018; Osteoimmunology: the Nexus between bone and immune system. Front Biosci (Landmark Ed). 23:464–92. DOI: 10.2741/4600. PMID: 28930556.
112. Davis JC Jr, Van Der Heijde D, Braun J, Dougados M, Cush J, Clegg DO, et al. 2003; Recombinant human tumor necrosis factor receptor (etanercept) for treating ankylosing spondylitis: a randomized, controlled trial. Arthritis Rheum. 48:3230–6. DOI: 10.1002/art.11325. PMID: 14613288.


113. van der Heijde D, Dijkmans B, Geusens P, Sieper J, DeWoody K, Williamson P, et al. 2005; Efficacy and safety of infliximab in patients with ankylosing spondylitis: results of a randomized, placebo-controlled trial (ASSERT). Arthritis Rheum. 52:582–91. DOI: 10.1002/art.20852. PMID: 15692973.


114. van der Heijde D, Kivitz A, Schiff MH, Sieper J, Dijkmans BA, Braun J, et al. 2006; Efficacy and safety of adalimumab in patients with ankylosing spondylitis: results of a multicenter, randomized, double-blind, placebo-controlled trial. Arthritis Rheum. 54:2136–46. DOI: 10.1002/art.21913. PMID: 16802350.


115. Inman RD, Davis JC Jr, Heijde D, Diekman L, Sieper J, Kim SI, et al. 2008; Efficacy and safety of golimumab in patients with ankylosing spondylitis: results of a randomized, double-blind, placebo-controlled, phase III trial. Arthritis Rheum. 58:3402–12. DOI: 10.1002/art.23969. PMID: 18975305.


116. Maxwell LJ, Zochling J, Boonen A, Singh JA, Veras MM, Tanjong Ghogomu E, et al. 2014; TNF-alpha inhibitors for ankylosing spondylitis. Cochrane Database Syst Rev. (4):CD005468. DOI: 10.1002/14651858.CD005468.pub2. PMID: 25887212.


117. Ward MM, Deodhar A, Gensler LS, Dubreuil M, Yu D, Khan MA, et al. 2019; 2019 Update of the American College of Rheumatology/Spondylitis Association of America/Spondyloarthritis Research and Treatment Network recommendations for the treatment of ankylosing spondylitis and nonradiographic axial spondyloarthritis. Arthritis Rheumatol. 71:1599–613. DOI: 10.1002/art.41042. PMID: 31436036. PMCID: PMC6764882.


118. Ko JM, Gottlieb AB, Kerbleski JF. 2009; Induction and exacerbation of psoriasis with TNF-blockade therapy: a review and analysis of 127 cases. J Dermatolog Treat. 20:100–8. DOI: 10.1080/09546630802441234. PMID: 18923992.


119. Alivernini S, Pugliese D, Tolusso B, Bui L, Petricca L, Guidi L, et al. 2018; Paradoxical arthritis occurring during anti-TNF in patients with inflammatory bowel disease: histological and immunological features of a complex synovitis. RMD Open. 4:e000667. DOI: 10.1136/rmdopen-2018-000667. PMID: 29657833. PMCID: PMC5892785.


120. Karmacharya P, Duarte-Garcia A, Dubreuil M, Murad MH, Shahukhal R, Shrestha P, et al. 2020; Effect of therapy on radiographic progression in axial spondyloarthritis: a systematic review and meta-analysis. Arthritis Rheumatol. 72:733–49. DOI: 10.1002/art.41206. PMID: 31960614. PMCID: PMC7218689.


121. Zhang JR, Liu XJ, Xu WD, Dai SM. 2016; Effects of tumor necrosis factor-α inhibitors on new bone formation in ankylosing spondylitis. Joint Bone Spine. 83:257–64. DOI: 10.1016/j.jbspin.2015.06.013. PMID: 26678001.


122. McGonagle D, Watad A, Sharif K, Bridgewood C. 2021; Why inhibition of IL-23 lacked efficacy in ankylosing spondylitis. Front Immunol. 12:614255. DOI: 10.3389/fimmu.2021.614255. PMID: 33815371. PMCID: PMC8017223.


123. Lee YH. 2022; Comparative efficacy and safety of Janus kinase inhibitors and secukinumab in patients with active ankylosing spondylitis: a systematic review and meta-analysis. Pharmacology. 107:537–44. DOI: 10.1159/000525627. PMID: 35817017. PMCID: PMC9811419.


124. Deodhar A, Gensler LS, Sieper J, Clark M, Calderon C, Wang Y, et al. 2019; Three multicenter, randomized, double-blind, placebo-controlled studies evaluating the efficacy and safety of ustekinumab in axial spondyloarthritis. Arthritis Rheumatol. 71:258–70. DOI: 10.1002/art.40728. PMID: 30225992.


125. Kavanaugh A, Puig L, Gottlieb AB, Ritchlin C, You Y, Li S, et al. 2016; Efficacy and safety of ustekinumab in psoriatic arthritis patients with peripheral arthritis and physician-reported spondylitis: post-hoc analyses from two phase III, multicentre, double-blind, placebo-controlled studies (PSUMMIT-1/PSUMMIT-2). Ann Rheum Dis. 75:1984–8. DOI: 10.1136/annrheumdis-2015-209068. PMID: 27098404.


126. Savage L, Goodfield M, Horton L, Watad A, Hensor E, Emery P, et al. 2019; Regression of peripheral subclinical enthesopathy in therapy-naive patients treated with ustekinumab for moderate-to-severe chronic plaque psoriasis: a fifty-two-week, prospective, open-label feasibility study. Arthritis Rheumatol. 71:626–31. DOI: 10.1002/art.40778. PMID: 30468001.
127. Deodhar A, Helliwell PS, Boehncke WH, Kollmeier AP, Hsia EC, Subramanian RA, et al. 2020; Guselkumab in patients with active psoriatic arthritis who were biologic-naive or had previously received TNFα inhibitor treatment (DISCOVER-1): a double-blind, randomised, placebo-controlled phase 3 trial. Lancet. 395:1115–25. Erratum in: Lancet 2020;395:1114. DOI: 10.1016/S0140-6736(20)30265-8. PMID: 32178765.


128. Blair HA. 2019; Secukinumab: a review in ankylosing spondylitis. Drugs. 79:433–43. Erratum in: Drugs 2019;79:445. DOI: 10.1007/s40265-019-01075-3. PMID: 30793255. PMCID: PMC6422944.


129. Deodhar A, Blanco R, Dokoupilová E, Hall S, Kameda H, Kivitz AJ, et al. 2021; Improvement of signs and symptoms of nonradiographic axial spondyloarthritis in patients treated with secukinumab: primary results of a randomized, placebo-controlled phase III study. Arthritis Rheumatol. 73:110–20. DOI: 10.1002/art.41477. PMID: 32770640. PMCID: PMC7839589.


130. van Mens LJJ, van de Sande MGH, Menegatti S, Chen S, Blijdorp ICJ, de Jong HM, et al. 2018; Brief report: interleukin-17 blockade with secukinumab in peripheral spondyloarthritis impacts synovial immunopathology without compromising systemic immune responses. Arthritis Rheumatol. 70:1994–2002. DOI: 10.1002/art.40581. PMID: 29869838.


131. Mease PJ, Smolen JS, Behrens F, Nash P, Liu Leage S, Li L, et al. 2020; A head-to-head comparison of the efficacy and safety of ixekizumab and adalimumab in biological-naïve patients with active psoriatic arthritis: 24-week results of a randomised, open-label, blinded-assessor trial. Ann Rheum Dis. 79:123–31. DOI: 10.1136/annrheumdis-2019-215386. PMID: 31563894. PMCID: PMC6937408.


132. Chandran V, van der Heijde D, Fleischmann RM, Lespessailles E, Helliwell PS, Kameda H, et al. 2020; Ixekizumab treatment of biologic-naïve patients with active psoriatic arthritis: 3-year results from a phase III clinical trial (SPIRIT-P1). Rheumatology (Oxford). 59:2774–84. DOI: 10.1093/rheumatology/kez684. PMID: 32031665. PMCID: PMC7516094.


133. Hohenberger M, Cardwell LA, Oussedik E, Feldman SR. 2018; Interleukin-17 inhibition: role in psoriasis and inflammatory bowel disease. J Dermatolog Treat. 29:13–8. DOI: 10.1080/09546634.2017.1329511. PMID: 28521565.


134. Kammüller M, Tsai TF, Griffiths CE, Kapoor N, Kolattukudy PE, Brees D, et al. 2017; Inhibition of IL-17A by secukinumab shows no evidence of increased Mycobacterium tuberculosis infections. Clin Transl Immunology. 6:e152. DOI: 10.1038/cti.2017.34. PMID: 28868144. PMCID: PMC5579471.


135. Wei JC, Kim TH, Kishimoto M, Ogusu N, Jeong H, Kobayashi S. 2021; Efficacy and safety of brodalumab, an anti-IL17RA monoclonal antibody, in patients with axial spondyloarthritis: 16-week results from a randomised, placebo-controlled, phase 3 trial. Ann Rheum Dis. 80:1014–21. DOI: 10.1136/annrheumdis-2020-219406. PMID: 33827787. PMCID: PMC8292606.


136. Kim TH, Kishimoto M, Wei JC, Jeong H, Nozaki A, Kobayashi S. 2023; Brodalumab, an anti-interleukin-17 receptor A monoclonal antibody, in axial spondyloarthritis: 68-week results from a phase 3 study. Rheumatology (Oxford). 62:1851–9. DOI: 10.1093/rheumatology/keac522. PMID: 36130275. PMCID: PMC10152297.


137. van der Heijde D, Gensler LS, Deodhar A, Baraliakos X, Poddubnyy D, Kivitz A, et al. 2020; Dual neutralisation of interleukin-17A and interleukin-17F with bimekizumab in patients with active ankylosing spondylitis: results from a 48-week phase IIb, randomised, double-blind, placebo-controlled, dose-ranging study. Ann Rheum Dis. 79:595–604. Erratum in: Ann Rheum Dis 2021;80:e186. DOI: 10.1136/annrheumdis-2020-216980. PMID: 32253184. PMCID: PMC7213320.


138. Baraliakos X, Deodhar A, Dougados M, Gensler LS, Molto A, Ramiro S, et al. 2022; Safety and efficacy of bimekizumab in patients with active ankylosing spondylitis: three-year results from a phase IIb randomized controlled trial and its open-label extension study. Arthritis Rheumatol. 74:1943–58. DOI: 10.1002/art.42282. PMID: 35829672.
139. van der Heijde D, Baraliakos X, Sieper J, Deodhar A, Inman RD, Kameda H, et al. 2022; Efficacy and safety of upadacitinib for active ankylosing spondylitis refractory to biological therapy: a double-blind, randomised, placebo-controlled phase 3 trial. Ann Rheum Dis. 81:1515–23. DOI: 10.1136/ard-2022-222608. PMID: 35788492. PMCID: PMC9606523.


140. Schwartz DM, Kanno Y, Villarino A, Ward M, Gadina M, O'Shea JJ. 2017; JAK inhibition as a therapeutic strategy for immune and inflammatory diseases. Nat Rev Drug Discov. 16:843–62. Erratum in: Nat Rev Drug Discov 2017;17:78. DOI: 10.1038/nrd.2017.201. PMID: 29104284. PMCID: PMC6168198.


141. Deodhar A, Sliwinska-Stanczyk P, Xu H, Baraliakos X, Gensler LS, Fleishaker D, et al. 2021; Tofacitinib for the treatment of ankylosing spondylitis: a phase III, randomised, double-blind, placebo-controlled study. Ann Rheum Dis. 80:1004–13. DOI: 10.1136/annrheumdis-2020-219601. PMID: 33906853. PMCID: PMC8292568.


142. Taylor PC, Saurigny D, Vencovsky J, Takeuchi T, Nakamura T, Matsievskaia G, et al. 2019; Efficacy and safety of namilumab, a human monoclonal antibody against granulocyte-macrophage colony-stimulating factor (GM-CSF) ligand in patients with rheumatoid arthritis (RA) with either an inadequate response to background methotrexate therapy or an inadequate response or intolerance to an anti-TNF (tumour necrosis factor) biologic therapy: a randomized, controlled trial. Arthritis Res Ther. 21:101. DOI: 10.1186/s13075-019-1879-x. PMID: 30999929. PMCID: PMC6471864.


143. Perrotta FM, Scriffignano S, Ciccia F, Lubrano E. 2022; Therapeutic targets for ankylosing spondylitis - recent insights and future prospects. Open Access Rheumatol. 14:57–66. DOI: 10.2147/OARRR.S295033. PMID: 35469137. PMCID: PMC9034883.


144. Izana Bioscience Ltd. 2022. Mar. 8. Efficacy and safety of namilumab for moderate-to-severe axial spondyloarthritis (NAMASTE) [Internet]. ClinicalTrials.gov;Bethesda (MD): https://classic.clinicaltrials.gov/ct2/show/NCT03622658. cited 2023 Jul 13.
145. Sen R, Kim E, Napier RJ, Cheng E, Fernandez A, Manning ES, et al. 2023; Neutrophil-to-lymphocyte ratio and platelet-to-lymphocyte ratio as biomarkers in axial spondyloarthritis: observational studies from the Program to Understand the Longterm Outcomes in Spondyloarthritis registry. Arthritis Rheumatol. 75:232–41. DOI: 10.1002/art.42333. PMID: 36053919. PMCID: PMC9892177.
146. Wang J, Su J, Yuan Y, Jin X, Shen B, Lu G. 2021; The role of lymphocyte-monocyte ratio on axial spondyloarthritis diagnosis and sacroiliitis staging. BMC Musculoskelet Disord. 22:86. DOI: 10.1186/s12891-021-03973-8. PMID: 33453722. PMCID: PMC7811735.


147. Lee JH, Jung JH, Kim J, Baek WK, Rhee J, Kim TH, et al. 2020; Proteomic analysis of human synovial fluid reveals potential diagnostic biomarkers for ankylosing spondylitis. Clin Proteomics. 17:20. DOI: 10.1186/s12014-020-09281-y. PMID: 32518534. PMCID: PMC7269004.


148. Quaden D, Vandormael P, Ruytinx P, Geusens P, Corten K, Vanhoof J, et al. 2020; Antibodies against three novel peptides in early axial spondyloarthritis patients from two independent cohorts. Arthritis Rheumatol. 72:2094–105. DOI: 10.1002/art.41427. PMID: 32638516.


149. Do L, Granåsen G, Hellman U, Lejon K, Geijer M, Baraliakos X, et al. 2021; Anti-CD74 IgA autoantibodies in radiographic axial spondyloarthritis: a longitudinal Swedish study. Rheumatology (Oxford). 60:4085–93. DOI: 10.1093/rheumatology/keaa882. PMID: 33369649. PMCID: PMC8410007.


150. De Craemer AS, Witte T, Lobaton Ortega T, Hoorens A, De Vos M, Cuvelier C, et al. 2023; Anti-CD74 IgA antibodies show diagnostic potential for axial spondyloarthritis but are not associated with microscopic gut inflammation. Rheumatology (Oxford). 62:984–90. DOI: 10.1093/rheumatology/keac384. PMID: 35781486.


151. Abdelaziz MM, Gamal RM, Ismail NM, Lafy RA, Hetta HF. 2021; Diagnostic value of anti-CD74 antibodies in early and late axial spondyloarthritis and its relationship to disease activity. Rheumatology (Oxford). 60:263–8. DOI: 10.1093/rheumatology/keaa292. PMID: 32710117.


152. Baraliakos X, Baerlecken N, Witte T, Heldmann F, Braun J. 2014; High prevalence of anti-CD74 antibodies specific for the HLA class II-associated invariant chain peptide (CLIP) in patients with axial spondyloarthritis. Ann Rheum Dis. 73:1079–82. DOI: 10.1136/annrheumdis-2012-202177. PMID: 23644552.


153. Riechers E, Baerlecken N, Baraliakos X, Achilles-Mehr Bakhsh K, Aries P, Bannert B, et al. 2019; Sensitivity and specificity of autoantibodies against CD74 in nonradiographic axial spondyloarthritis. Arthritis Rheumatol. 71:729–35. DOI: 10.1002/art.40777. PMID: 30418704.
Fig. 1
Pathogenesis of ankylosing spondylitis. Gut microbiome produces short-chain fatty acids, tryptophan metabolites, and amino acids. Also, Paneth cells are source of IL-23 in the terminal ileum. HLA-B*27 provides arthritogenic peptide to TCR, and misfolded HLA-B*27 and accumulated protein induce UPR resulting in production of IL-23. Mechanical stress in enthesis induce DAMPs and IL-1β. As a result, produced IL-23 plays a pivotal role in initiating ankylosing spondylitis. Immune cells are involved to progress the disease by inducing IL-17, IL-22, and TNF-α. Bone remodeling is activated, as a result, new bone formation and bone resorption are promoted through cytokines. TCR: T-cell receptor, UPR: unfolded protein response, DAMPs: danger-associated molecular pattern, IL: interleukin, TNF: tumor necrosis factor, NK: natural killer, ERAP: endoplasmic reticulum aminopeptidases.
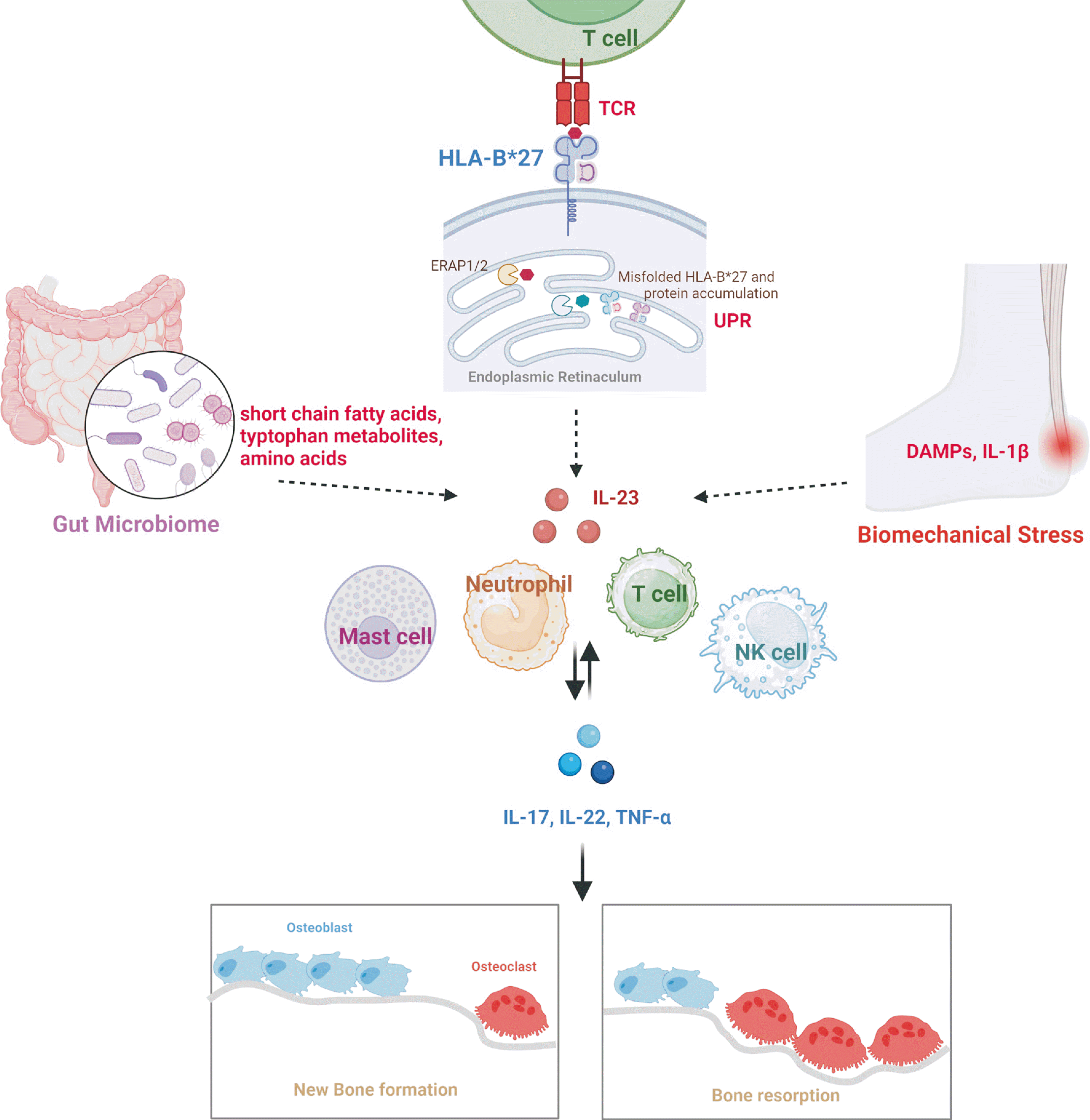