Abstract
Background
Methods
Results
Notes
DATA AVAILABILITY STATEMENT
The datasets generated during and/or analyzed during the current study are available from the corresponding author on reasonable request.
AUTHOR CONTRIBUTIONS
Conceptualization: Ha Yeon Park, Junyong In. Data curation: Ha Yeon Park, Hey Ran Choi, Yong Beom Kim, Seok Kyeong Oh, Taehoon Kim, Hong Seuk Yang, Junyong In. Formal analysis: Ha Yeon Park, Junyong In. Methodology: Ha Yeon Park. Project administration: Ha Yeon Park, Junyong In. Visualization: Ha Yeon Park, Junyong In. Writing - original draft: Ha Yeon Park, Junyong In. Writing - review & editing: Ha Yeon Park, Hey Ran Choi, Yong Beom Kim, Seok Kyeong Oh, Taehoon Kim, Hong Seuk Yang, Junyong In. Investigation: Ha Yeon Park, Junyong In. Supervision: Junyong In.
ACKNOWLEDGMENTS
REFERENCES






















Fig. 1.
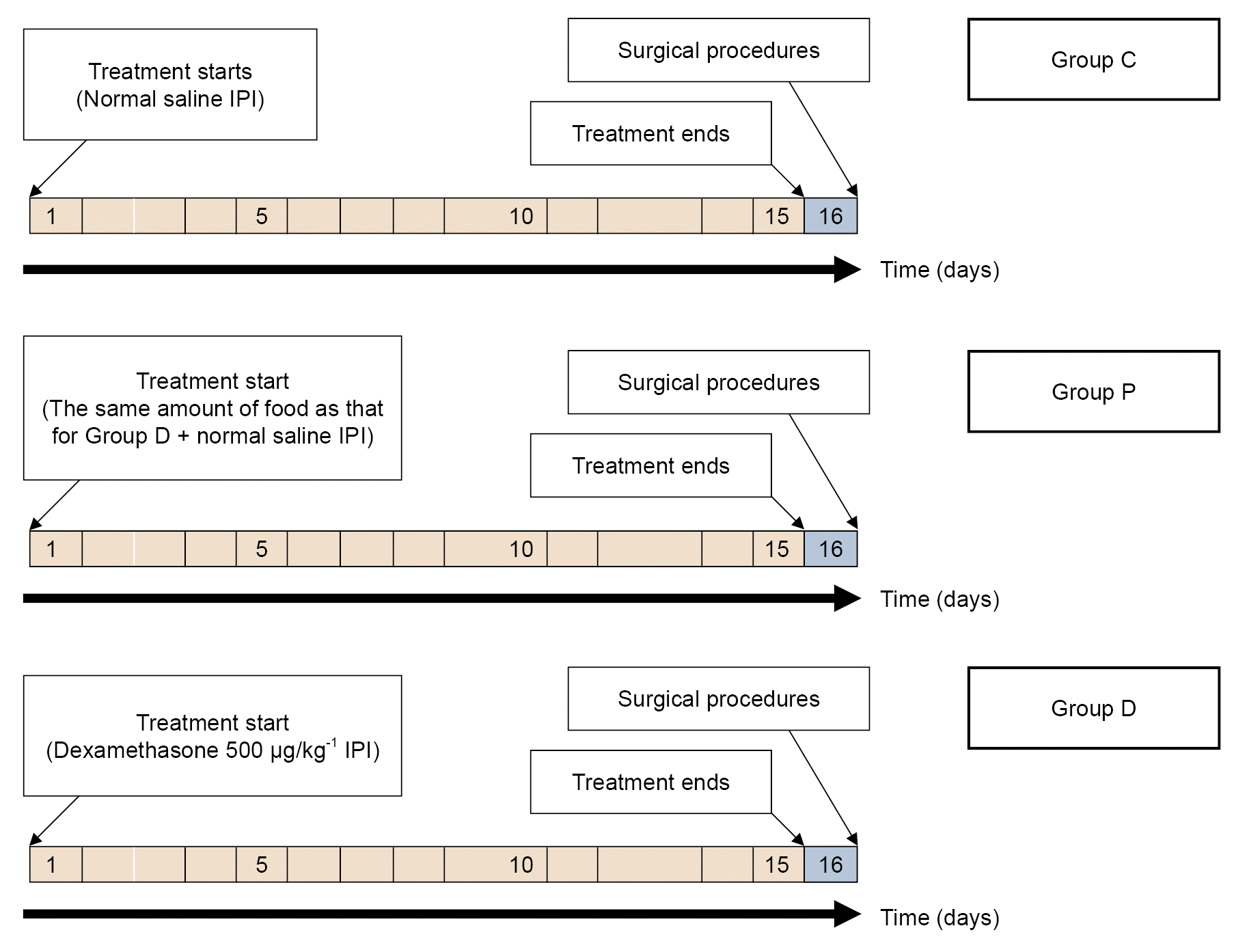
Fig. 2.
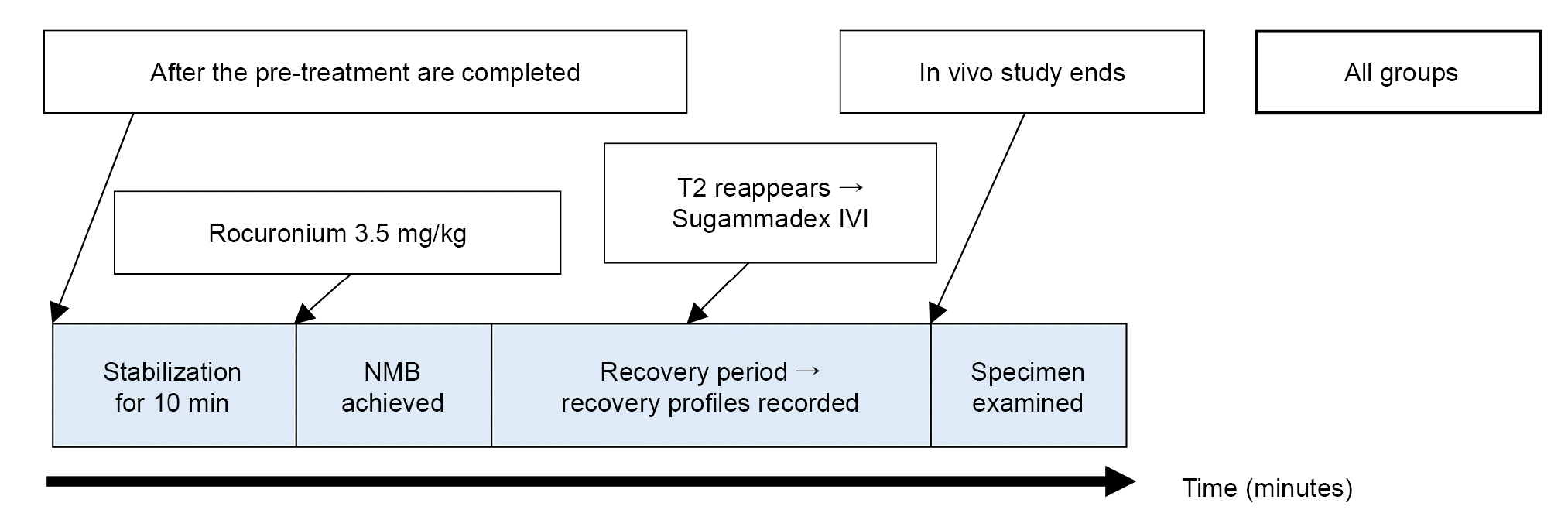
Fig. 3.
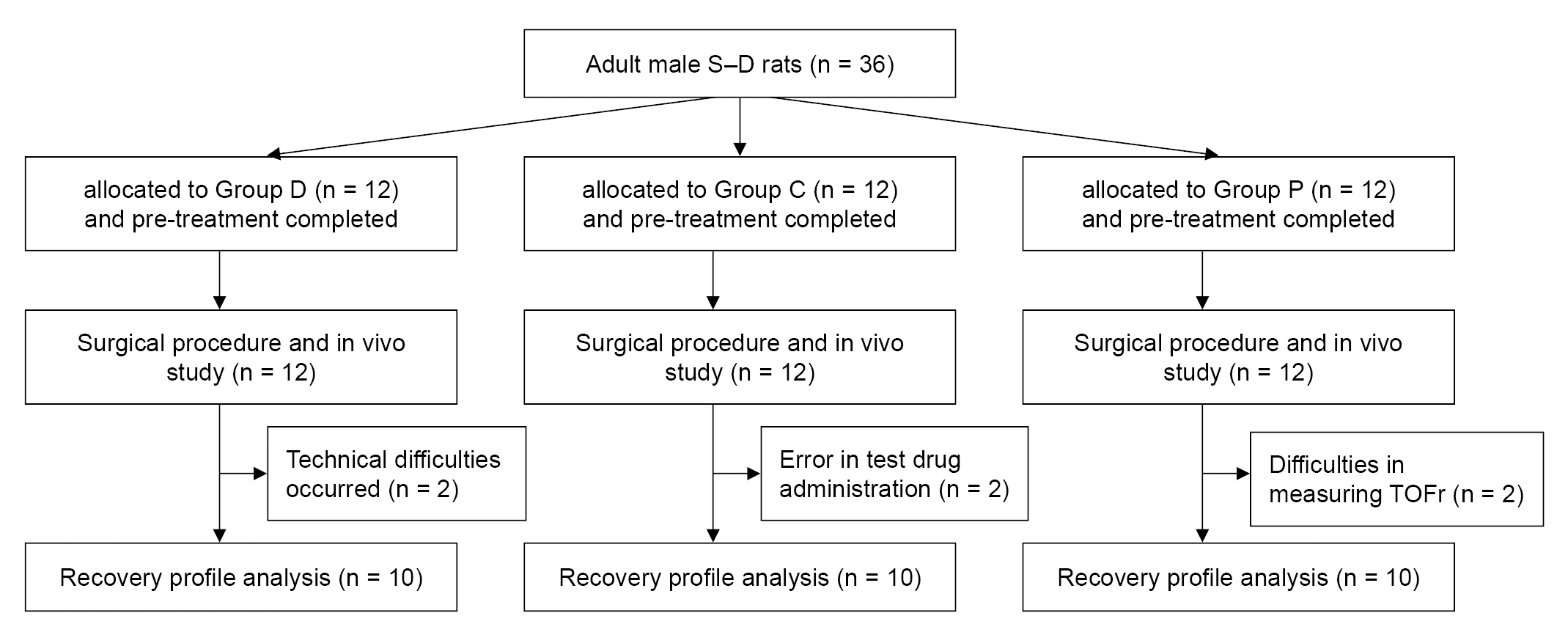
Table 1.
Factors |
Condition |
||
---|---|---|---|
Group C (n = 10) | Group D (n = 10) | Group P (n = 10) | |
Weight (g) | |||
Initial | 241 ± 7 | 232 ± 19 | 236 ± 17 |
Final | 392 ± 15 | 219 ± 17*,† | 322 ± 18* |
Temperature (°C) | 37.6 ± 0.1 | 37.6 ± 0.2 | 37.5 ± 0.2 |
Anterior tibialis muscle | |||
Weight (mg) | 687 ± 65 | 365 ± 48*,† | 565 ± 21* |
Length (mm) | 22 (21, 22) | 19 (18, 21) | 21 (21, 21) |
Width (mm) | 11 (11, 12) | 8 (8, 9) | 10 (9, 10) |
Values are presented as mean ± SD or median (1Q, 3Q). Group C: control group, Group D: dexamethasone group, and Group P: pair-fed group. Group C received the same amount of 0.9% saline daily as Group D. Group D received a daily intraperitoneal injection of 500 μg/kg dexamethasone suspended in 1 ml of 0.9% saline. Group P was fed with the same amount of food as Group D.
Table 2.
Factors |
Condition |
||
---|---|---|---|
Group C (n = 10) | Group D (n = 10) | Group P (n = 10) | |
Duration of neuromuscular blockade | |||
TT2 (min) | 5.0 ± 1.1 | 2.9 ± 1.0*,† | 5.1 ± 1.4 |
Recovery profiles after sugammadex administration | |||
TTOFr (min) | 4.3 ± 2.3 | 3.1 ± 1.8 | 4.0 ± 3.0 |
TT1 (min) | 4.2 ± 2.2 | 3.6 ± 2.1 | 4.4 ± 2.5 |
RI (min) | 1.5 ± 0.6 | 1.3 ± 0.6 | 2.2 ± 1.8 |
Values are presented as mean ± SD. Group C: control group, Group D: dexamethasone group, Group P: pair-fed group. Group C received only 0.9% saline, whereas Group D received saline daily. Group D received a daily intraperitoneal injection of 500 μg/kg dexamethasone suspended in 1 ml of 0.9% saline. Group P was fed daily with the same amount of food that was consumed by Group D. Time and recovery indices are presented as min; TOF: train-of-four, T1: the first twitch of train-of-four, T2: the second twitch of train-of-four, TT2: the time from rocuronium injection to appearance of T2, TTOFr: time taken for the TOF ratio to recover to 0.9 or higher after injection of sugammadex, TT1: the time taken for T1 to recover to 95% of the baseline T1 after the injection of sugammadex, RI: recovery index (the time taken from T1 of 25% of the baseline T1 to T1 of 75% of the baseline T1).