Abstract
Background
The transition of dialysis modalities from continuous renal replacement therapy (CRRT) to intermittent hemodialysis (iHD) is frequently conducted during the recovery phase of critically ill patients with acute kidney injury. Herein, we addressed the occurrence of intradialytic hypotension (IDH) after this transition, and its association with the mortality risk.
Methods
A total of 541 patients with acute kidney injury who attempted to transition from CRRT to iHD at Seoul National University Hospital, Korea from 2010 to 2020 were retrospectively collected. IDH was defined as a discontinuation of dialysis because of hemodynamic instability plus a nadir systolic blood pressure <90 mm Hg or a decrease in systolic blood pressure ≥30 mm Hg during the first session of iHD. Odds ratios (ORs) of outcomes, such as in-hospital mortality and weaning from RRT, were measured using a logistic regression model after adjusting for multiple variables.
Results
IDH occurred in 197 patients (36%), and their mortality rate (44%) was higher than that of those without IDH (19%; OR, 2.64; 95% confidence interval [CI], 1.70–4.08). For patients exhibiting IDH, the iHD sessions delayed successful weaning from RRT (OR, 0.62; 95% CI, 0.43–0.90) compared with sessions on those without IDH. Factors such as low blood pressure, high pulse rate, low urine output, use of mechanical ventilations and vasopressors, and hypoalbuminemia were associated with IDH risk.
Acute kidney injury (AKI) requiring renal replacement therapy (RRT) is a common and serious condition that is associated with poor outcomes [1]. The incidence of RRT-requiring AKI has increased by 10% per year from 2000 to 2009 [2], and its death rate is over 50% [3,4]. Even for survivors, RRT-requiring AKI carries the risk of several complications, such as progression to chronic kidney disease [5,6] and cardiovascular events, including myocardial infarction and congestive heart failure [7,8].
Modalities of RRT in the setting of AKI include continuous renal replacement therapy (CRRT) and intermittent hemodialysis (iHD). Whereas iHD achieves a rapid clearance of small uremic toxins within a short time, CRRT provides osmotic stability by the gradual elimination of fluid and toxins over a long period [9]. Although previous studies have not demonstrated the difference in survival or renal recovery outcome between the two modalities, CRRT is recommended as an initial modality for patients with hemodynamic instability [10-13].
Once patients with AKI achieve hemodynamic stability and fluid balance with CRRT, clinicians may consider a transition to iHD [10,14]. Sometimes, the unaffordable cost of CRRT or the need for patient mobilization and rehabilitation demands the transition to iHD [15,16]. Although RRT modality transitions are a common occurrence in the intensive care unit (ICU), data regarding adverse events, such as intradialytic hypotension (IDH) or arrhythmia after transition, are still limited.
IDH is attributable to overzealous ultrafiltration and impaired vascular resistance in decreased cardiovascular reserves [17]. IDH can occur in 10%-80% of patients with AKI, which may be dependent on the definition [18-20]. IDH is associated with high mortality in patients with AKI regardless of its modalities [19,21]. However, it remains undetermined whether IDH after a transition from CRRT to iHD is associated with adverse outcomes in patients with AKI. The present study addressed this issue using a cohort undergoing a transition and identified risk factors related to IDH occurrence.
The study was approved by the Institutional Review Board of the Seoul National University Hospital (No. H-2110-085-1262) and complied with the Declaration of Helsinki. The requirement for informed consent was waived by the Review Board.
The study was retrospective in nature using a cohort of 2,397 patients who underwent CRRT due to AKI at Seoul National University Hospital from June 2010 to December 2020. A total of 1,108 patients underwent a transition from CRRT to iHD because of the recovery phase in patient status. When the volume control became easy due to resolved oliguria and need of vasoactive agents was reduced, the transition between modalities was recommended according to the Kidney Disease Improving Global guideline [10]. Patients who were under 18 years old (n=11) and patients without information on iHD (n=556) were excluded. Accordingly, 541 patients were analyzed in the present study (Figure 1).
Baseline information at the time of transition was collected, such as age, sex, body mass index, cause of AKI (e.g., septic and nonseptic), the time interval between CRRT and iHD, systolic blood pressure, diastolic blood pressure, mean arterial pressure, pulse rate, duration of iHD, ultrafiltration volume, urine output, mechanical ventilation, and use of vasopressors. Blood pressures were measured using noninvasive blood pressure monitoring system. Sequential Organ Failure Assessment (SOFA) was calculated according to the original formula [22]. Dialysis settings, such as ultrafiltration target, blood flow rate, and session time, could be different between patient according to their status. All patients performed high-flux hemodialysis. Either unfractionated heparin or nafamostat was used as an anticoagulant during CRRT. The Charlson comorbidity index was calculated to consider the effect of various comorbidities, such as acute myocardial infarction, congestive heart failure, peripheral vascular disease, cerebral vascular accident, dementia, pulmonary disease, connective tissue disorder, peptic ulcer, liver disease, diabetes, paraplegia, chronic kidney disease, malignancy, and human immunodeficiency virus infection [23].
The patients were categorized into two groups according to the presence of IDH during the 1st session of iHD. Because there is no consensus on defining IDH when patients have AKI rather than end-stage kidney disease, we referred to methods used in previous studies as follows [24]: discontinuation of dialysis as a result of hemodynamic instability plus a nadir systolic blood pressure <90 mm Hg (termed IDH-A) or a decrease in systolic blood pressure ≥30 mm Hg (termed IDH-B).
The primary outcome was all-cause mortality after transition until hospital discharge or death. Additionally, weaning from RRT as a cessation of RRT for >7 days was evaluated during the above timeframe.
Categorical and continuous variables are expressed as proportions and means±standard deviations when they were normally distributed and as medians with interquartile ranges when they were not normally distributed, respectively. The normality of the distribution was analyzed using the Kolmogorov–Smirnov test. The chi-square test or Fisher’s exact test was used to compare categorical variables. The Student t test or the Mann-Whitney U-test was used for continuous variables with or without a normal distribution. Kaplan-Meier survival curves were drawn and compared between groups using a log-rank test. The odds ratios (ORs) and confidence intervals for mortality and kidney outcomes were calculated using logistic regression model. The adjusted variables were selected based on their significant association with mortality. To identify risk factors for IDH, backward stepwise selection was further applied. All statistical analyses were performed using IBM SPSS ver. 27 (IBM Corp.) and R software (ver. 3.5.1; R core team). A P-value of less than 0.05 was considered statistically significant.
The mean patient age was 64±15 years, and 39.5% of the patients were female. The proportion of patients with septic AKI was 50.6%. IDH occurred in 197 patients (36.4%). Among them, 123 patients (22.7%) had IDH-A alone, 138 patients (25.5%) had IDH-B alone, and 64 patients (11.8%) had both. The IDH prevalence was different according to the ICU subtypes: 39.0% in medical ICU, 28.5% in surgical ICU, 35.6% in cardiopulmonary ICU, and 38.2% in emergency ICU.
The IDH group was more likely to have low systolic blood pressure, high pulse rate, low urine output, high SOFA score and the need for mechanical ventilation and vasopressors than the non-IDH group. Before hemodialysis, 22.0% of patients and 9.2% after hemodialysis used vasopressors, respectively. Other baseline characteristics are shown in Table 1.
During a median follow-up period of 25 days (interquartile range, 13–54 days), 160 patients (28.3%) died. The mortality incidence was 5.7 deaths per 1,000 person-days. When a univariate Cox regression model was applied, several variables, such as cause of AKI, pulse rate, SOFA score, platelet count, and total bilirubin, were associated with in-hospital mortality in the present cohort (Table 2), and these were included in subsequent multivariate regression models as adjusting factors. Figure 2 shows Kaplan-Meier survival curves according to IDH occurrence; the groups with IDH-A, IDH-B, or both had lower survival rates than the non-IDH group. The low survival rates in patients with IDH compared to those without IDH remained consistent after adjusting for multiple variables (Table 3).
Figure 3 depicts curves that show weaning from RRT between the groups with and without IDH, and the weaning rate was higher in the non-IDH group than in the IDH group (P<0.001). In a subgroup analysis, the association between IDH and low weaning from RRT was prominent when patients were male and had septic AKI or high SOFA scores (Figure 4). When logistic regression was applied, the iHD sessions delayed successful weaning from RRT (OR, 0.62; 95% confidence interval, 0.43–0.90) compared with sessions on those without IDH.
When a multivariate logistic regression model was applied, several factors were associated with IDH occurrence, such as low systolic blood pressure, high pulse rate, low urine output, and the use of mechanical ventilation and vasopressors. Regarding IDH-A, hypoalbuminemia was further noted to correlate with IDH occurrence (Table 4).
It is well known that IDH occurrence confers a high risk of mortality in patients with end-stage kidney disease; however, this relationship has not been well established for AKI patients requiring iHD. The present study used a cohort of patients with AKI who needed a transition from CRRT to iHD. IDH occurrence after transition was associated with subsequent high risks of mortality and nonweaning from RRT. Several factors were identified to be associated with IDH occurrence, and the findings will help clinicians identify patients at risk of hemodynamic instability, such as IDH after the transition to iHD.
The prevalence of IDH on severe AKI varied from 30% to 90% depending on the definition of AKI and its timing [19,21,25]. Several factors may further increase the risk of IDH in patients with AKI receiving iHD compared to those with end-stage kidney disease receiving maintenance iHD. These include fluid overload due to hemodynamic instability, nutritional support, and intravenous antibiotics. Furthermore, IDH can occur because of an impaired response to the physiological stress of RRT, such as increased vascular resistance or cardiac reserve due to critical illness [26]. The occurrence of IDH might be related to the delivered dose of RRT and fluid removal being insufficient, all of which influence patient outcomes.
A previous prospective cohort study of patients with AKI requiring RRT, wherein IDH was defined for each session of RRT as either the occurrence of mean arterial pressure <65 mm Hg or a reduction of ≥20% from the starting mean arterial pressure, demonstrated that more frequent exposure to IDH led to a higher risk of mortality outcome regardless of RRT modality [19]. Another observational study with CRRT alone showed that IDH occurring within the first hour of CRRT initiation, defined as a decline in blood pressure from baseline, was associated with an increased risk of mortality [21]. The present study reconfirmed the association of patient outcome with IDH, although the definition and timing of IDH differ between studies.
One previous study suggested a high risk of mortality when IDH occurs during the transition period (n=213) [25]. The present study expanded the sample size and added sufficient laboratory data at the time of iHD initiation to the analyses. Furthermore, the present study considered an RRT weaning outcome. Baseline hypoalbuminemia indicated the risk of IDH, which might reflect pathological conditions, such as organ failure, nutritional deficiency, and inflammation, in critically ill patients [27,28]. Because serum albumin primarily determines osmotic pressure and has a decisive effect on intravascular volume, hypoalbuminemia would result in hemodynamic instability while on iHD. In a prospective randomized controlled trial, the administration of albumin during iHD resulted in few episodes of IDH and improved the fluid removal rate compared to the nonadministration of albumin [29].
The occurrence of IDH delayed RRT weaning and thus prolonged the dependence of patients with ischemic AKI on RRT [30]. Hot lesions in ischemic tubules were further observed in kidney biopsies of patients dependent on RRT, which suggests that IDH would cause an additional ischemic injury [31]. In a randomized controlled trial comparing CRRT and iHD after AKI, IDH was associated with mortality and a lower probability of renal recovery for both modalities [32].
Although the study is informative, there are certain limitations to be addressed. Because of the retrospective design, unmeasured bias and confounders could have interfered with the present analyses. Continuous fluctuations in biochemical parameters and alterations during practice could be related to mortality but were not considered in the study. The present study did not categorize nonseptic patients into specific causes, which could further segment risk factors of IDH. The cause of death was not taken into consideration, which could affect the results.
The occurrence of IDH after transitioning from CRRT is associated with an increased risk of hospital mortality and dependence on RRT. Certain factors, such as low blood pressure, high pulse rate, low urine output, use of mechanical ventilation and vasopressors, and hypoalbuminemia, are associated with IDH occurrence. The findings provide a basis for future studies to clarify the clinical implications of IDH and strategies to prevent it in the context of transitions between RRT modalities in patients with AKI.
ACKNOWLEDGMENTS
The results presented in this paper have not been published previously in whole or part, except in abstract format at The Korean Society of Nephrology (KSN) 2022.
REFERENCES
1. Cerdá J, Lameire N, Eggers P, Pannu N, Uchino S, Wang H, et al. Epidemiology of acute kidney injury. Clin J Am Soc Nephrol. 2008; 3:881–6.


2. Hsu RK, McCulloch CE, Dudley RA, Lo LJ, Hsu CY. Temporal changes in incidence of dialysis-requiring AKI. J Am Soc Nephrol. 2013; 24:37–42.


3. Uchino S, Kellum JA, Bellomo R, Doig GS, Morimatsu H, Morgera S, et al. Acute renal failure in critically ill patients: a multinational, multicenter study. JAMA. 2005; 294:813–8.


4. Tolwani A. Continuous renal-replacement therapy for acute kidney injury. N Engl J Med. 2012; 367:2505–14.


5. Lo LJ, Go AS, Chertow GM, McCulloch CE, Fan D, Ordoñez JD, et al. Dialysis-requiring acute renal failure increases the risk of progressive chronic kidney disease. Kidney Int. 2009; 76:893–9.


6. Duran PA, Concepcion LA. Survival after acute kidney injury requiring dialysis: long-term follow up. Hemodial Int. 2014; 18 Suppl 1:S1–6.


7. Chawla LS, Amdur RL, Shaw AD, Faselis C, Palant CE, Kimmel PL. Association between AKI and long-term renal and cardiovascular outcomes in United States veterans. Clin J Am Soc Nephrol. 2014; 9:448–56.


8. Wu VC, Wu CH, Huang TM, Wang CY, Lai CF, Shiao CC, et al. Long-term risk of coronary events after AKI. J Am Soc Nephrol. 2014; 25:595–605.


9. Bellomo R, Kellum JA, La Manna G, Ronco C. 40 Years of continuous renal replacement therapy. Karger;2018.
10. Khwaja A. KDIGO clinical practice guidelines for acute kidney injury. Nephron Clin Pract. 2012; 120:c179–84.


11. Schefold JC, von Haehling S, Pschowski R, Bender T, Berkmann C, Briegel S, et al. The effect of continuous versus intermittent renal replacement therapy on the outcome of critically ill patients with acute renal failure (CONVINT): a prospective randomized controlled trial. Crit Care. 2014; 18:R11.


12. Chawla LS, Bellomo R, Bihorac A, Goldstein SL, Siew ED, Bagshaw SM, et al. Acute kidney disease and renal recovery: consensus report of the Acute Disease Quality Initiative (ADQI) 16 Workgroup. Nat Rev Nephrol. 2017; 13:241–57.


13. Nash DM, Przech S, Wald R, O’Reilly D. Systematic review and meta-analysis of renal replacement therapy modalities for acute kidney injury in the intensive care unit. J Crit Care. 2017; 41:138–44.


14. Karkar A, Ronco C. Prescription of CRRT: a pathway to optimize therapy. Ann Intensive Care. 2020; 10:32.


15. Brownback CA, Fletcher P, Pierce LN, Klaus S. Early mobility activities during continuous renal replacement therapy. Am J Crit Care. 2014; 23:348–51.


16. Srisawat N, Lawsin L, Uchino S, Bellomo R, Kellum JA, BEST Kidney Investigators. Cost of acute renal replacement therapy in the intensive care unit: results from The Beginning and Ending Supportive Therapy for the Kidney (BEST Kidney) study. Crit Care. 2010; 14:R46.


17. Sars B, van der Sande FM, Kooman JP. Intradialytic hypotension: mechanisms and outcome. Blood Purif. 2020; 49:158–67.


18. VA/NIH Acute Renal Failure Trial Network, Palevsky PM, Zhang JH, O’Connor TZ, Chertow GM, Crowley ST, et al. Intensity of renal support in critically ill patients with acute kidney injury. N Engl J Med. 2008; 359:7–20.


19. Silversides JA, Pinto R, Kuint R, Wald R, Hladunewich MA, Lapinsky SE, et al. Fluid balance, intradialytic hypotension, and outcomes in critically ill patients undergoing renal replacement therapy: a cohort study. Crit Care. 2014; 18:624.


20. Tonelli M, Astephen P, Andreou P, Beed S, Lundrigan P, Jindal K. Blood volume monitoring in intermittent hemodialysis for acute renal failure. Kidney Int. 2002; 62:1075–80.
21. Shawwa K, Kompotiatis P, Jentzer JC, Wiley BM, Williams AW, Dillon JJ, et al. Hypotension within one-hour from starting CRRT is associated with in-hospital mortality. J Crit Care. 2019; 54:7–13.
22. Jones AE, Trzeciak S, Kline JA. The Sequential Organ Failure Assessment score for predicting outcome in patients with severe sepsis and evidence of hypoperfusion at the time of emergency department presentation. Crit Care Med. 2009; 37:1649–54.
23. Sundararajan V, Henderson T, Perry C, Muggivan A, Quan H, Ghali WA. New ICD-10 version of the Charlson comorbidity index predicted in-hospital mortality. J Clin Epidemiol. 2004; 57:1288–94.
24. Flythe JE, Xue H, Lynch KE, Curhan GC, Brunelli SM. Association of mortality risk with various definitions of intradialytic hypotension. J Am Soc Nephrol. 2015; 26:724–34.
25. Beaubien-Souligny W, Yang Y, Burns KE, Friedrich JO, Meraz-Muñoz A, Clark EG, et al. Intra-dialytic hypotension following the transition from continuous to intermittent renal replacement therapy. Ann Intensive Care. 2021; 11:96.
26. Douvris A, Zeid K, Hiremath S, Bagshaw SM, Wald R, Beaubien-Souligny W, et al. Mechanisms for hemodynamic instability related to renal replacement therapy: a narrative review. Intensive Care Med. 2019; 45:1333–46.
27. Kaysen GA, Dubin JA, Müller HG, Rosales L, Levin NW, Mitch WE, et al. Inflammation and reduced albumin synthesis associated with stable decline in serum albumin in hemodialysis patients. Kidney Int. 2004; 65:1408–15.
28. Gabay C, Kushner I. Acute-phase proteins and other systemic responses to inflammation. N Engl J Med. 1999; 340:448–54.
29. Macedo E, Karl B, Lee E, Mehta RL. A randomized trial of albumin infusion to prevent intradialytic hypotension in hospitalized hypoalbuminemic patients. Crit Care. 2021; 25:18.
30. Manns M, Sigler MH, Teehan BP. Intradialytic renal haemodynamics: potential consequences for the management of the patient with acute renal failure. Nephrol Dial Transplant. 1997; 12:870–2.
31. Kelleher SP, Robinette JB, Miller F, Conger JD. Effect of hemorrhagic reduction in blood pressure on recovery from acute renal failure. Kidney Int. 1987; 31:725–30.
32. Augustine JJ, Sandy D, Seifert TH, Paganini EP. A randomized controlled trial comparing intermittent with continuous dialysis in patients with ARF. Am J Kidney Dis. 2004; 44:1000–7.
Figure 1.
Flowchart of selection criteria. CRRT: continuous renal replacement therapy; AKI: acute kidney injury; iHD: intermittent hemodialysis.
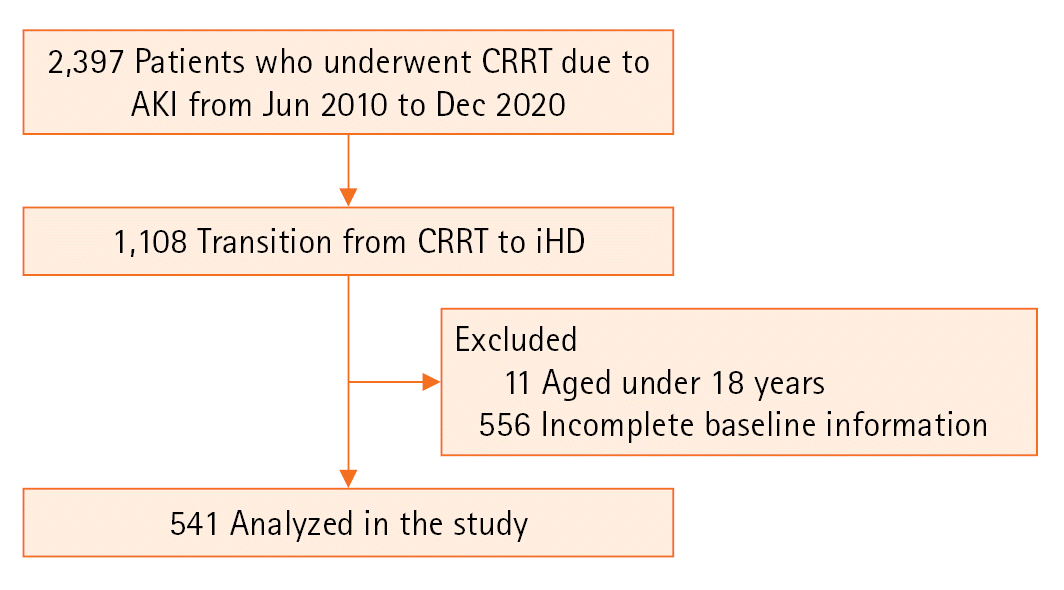
Figure 2.
Kaplan-Meier survival curves between patients with and without intradialytic hypotension (IDH). IDH was defined as a discontinuation of dialysis because of hemodynamic instability plus a nadir systolic blood pressure <90 mm Hg (IDH-A) or a decrease in systolic blood pressure ≥30 mm Hg (IDH-B).
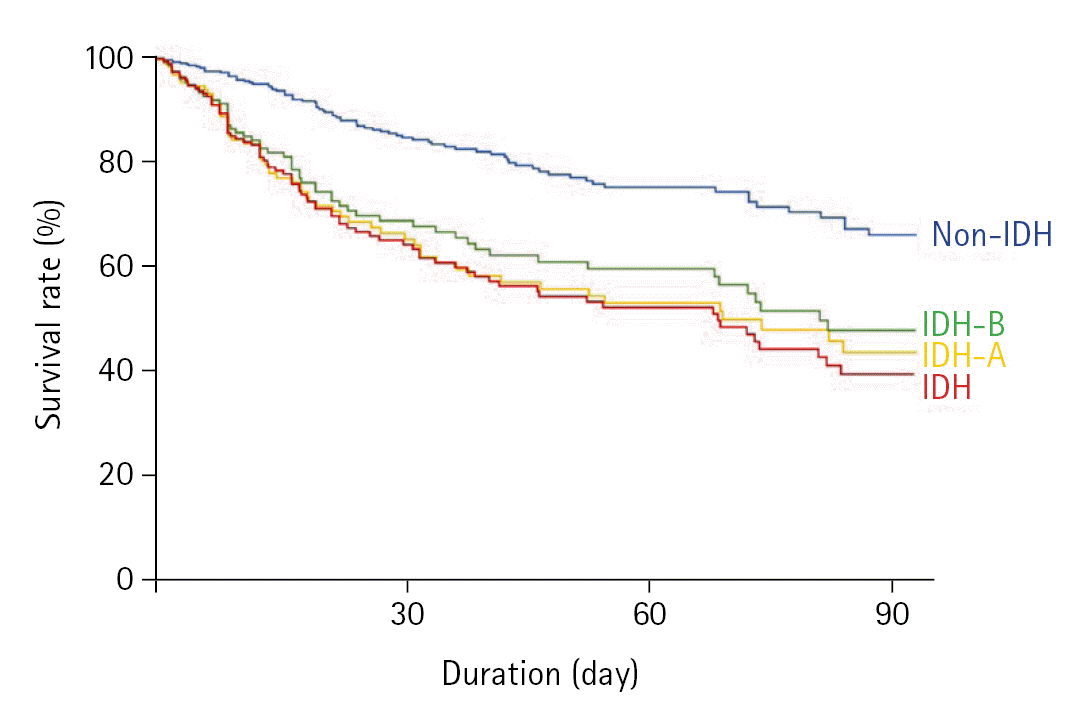
Figure 3.
Kaplan-Meier curves of the weaning rate from renal replacement therapy (RRT). IDH: intradialytic hypotension.
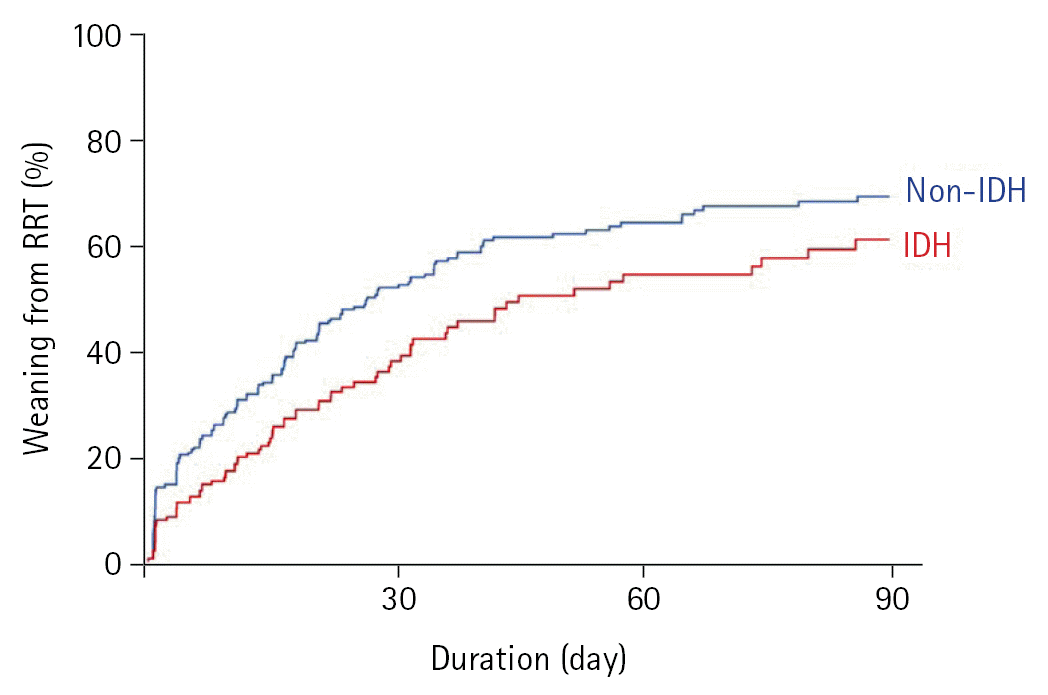
Figure 4.
Forest plot of subgroup analyses for the odds ratio (OR) of the renal replacement therapy weaning rate in the group with intradialytic hypotension compared with that of the group without intradialytic hypotension. CI: confidence interval; AKI: acute kidney injury; SOFA: Sequential Organ Failure Assessment.
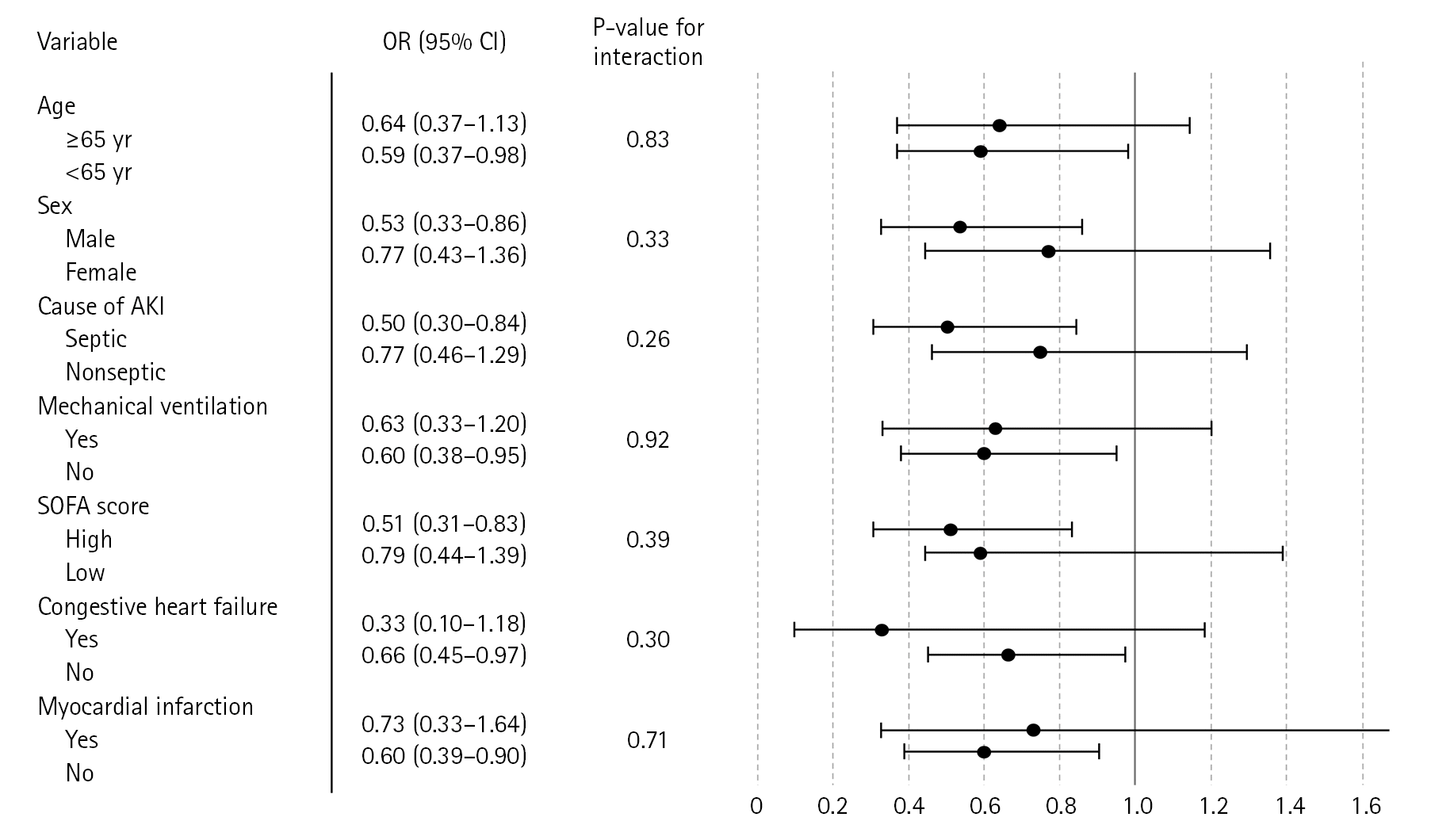
Table 1.
Baseline patient characteristics
Table 2.
Factors related to in-hospital mortality
Table 3.
Odds ratios of in-hospital mortality in patients with IDH compared with those without IDH
Outcome | Unadjusted OR (95% CI) | P-value | Adjusted OR (95% CI)a) | P-value |
---|---|---|---|---|
IDH | 3.33 (2.258–4.914) | <0.001 | 2.57 (1.632–4.040) | <0.001 |
IDH–Ab) | 3.33 (2.182–5.076) | <0.001 | 2.71 (1.600–4.643) | <0.001 |
IDH–Bb) | 2.46 (1.631–3.694) | <0.001 | 2.35 (1.443–3.834) | 0.001 |
IDH: intradialytic hypotension; OR: odds ratio; CI: confidence interval; SOFA: Sequential Organ Failure Assessment.
Table 4.
Factors associated with IDH in logistic regression with backward stepwise selection
Variable |
IDH |
IDH–Aa) |
IDH–Ba) |
|||
---|---|---|---|---|---|---|
OR (95% CI) | P-value | OR (95% CI) | P-value | OR (95% CI) | P-value | |
Age (per 10 yr) | 1.10 (0.970–1.257) | 0.135 | 1.15 (0.973–1.350) | 0.103 | 1.12 (0.972–1.282) | 0.118 |
Female (vs. male) | 1.23 (0.836–1.802) | 0.295 | 1.25 (0.787–1.999) | 0.341 | 1.47 (0.972–2.213) | 0.068 |
Systolic blood pressure (per 10 mm Hg) | 0.92 (0.834–1.003) | 0.058 | 0.68 (0.594–0.768) | <0.001 | 1.15 (1.033–1.274) | 0.010 |
Pulse rate (per 10 beats/min) | 1.33 (1.191–1.485) | <0.001 | 1.35 (1.187–1.534) | <0.001 | 1.31 (1.170–1.475) | 0.001 |
Transition after 24 hr (vs. within 24 hr) | 1.13 (0.873–1.471) | 0.348 | 1.15 (0.826–1.556) | 0.397 | 0.99 (0.746–1.311) | 0.937 |
Hemodialysis duration (per 1 hr) | 0.61 (0.314–1.191) | 0.148 | 0.67 (0.298–1.526) | 0.355 | 0.63 (0.304–1.303) | 0.213 |
Ultrafiltration volume (per 1 L) | 1.10 (0.898–1.354) | 0.348 | 1.05 (0.831–1.336) | 0.665 | 0.99 (0.794–1.232) | 0.989 |
Urine output (per 100 ml/day) | 0.96 (0.921–0.998) | 0.038 | 0.97 (0.925–1.023) | 0.259 | 0.96 (0.964–1.007) | 0.096 |
Mechanical ventilation (%) | 1.71 (1.121–2.553) | 0.012 | 1.39 (0.848–2.284) | 0.191 | 1.40 (0.900–2.178) | 0.136 |
Use of vasopressor (vs. none) | 1.91 (1.212–3.015) | 0.005 | 2.84 (1.719–4.691) | <0.001 | 1.72 (1.053–2.794) | 0.030 |
Albumin (per 1 g/dl) | 0.71 (0.470–1.055) | 0.089 | 0.51 (0.311–0.845) | 0.009 | 1.00 (0.656–1.524) | 0.998 |