Abstract
Diffuse intrinsic pontine gliomas (DIPGs) account for 10%–20% of all central nervous system tumors in children and are the leading cause of death in children with brain tumors. Although many clinical trials have been conducted over the past decades, the survival outcome has remained unchanged. Over 90% of children die within 2 years of the diagnosis, and radiotherapy remains the standard treatment to date. To improve the prognosis, hyperfractionated and hypofractionated radiotherapy and/or addition of radiosensitizers have been investigated. However, none of the radiotherapy approaches have shown a survival benefit, and the overall survival of patients with DIPG is approximately 11 months. Here, we comprehensively review the management of DIPG with focus on radiotherapy.
Diffuse intrinsic pontine glioma (DIPG) is the most aggressive primary central nervous system malignancy in children, accounting for 10%–20% of all childhood brain tumors [12]. It originates in the pons and commonly infiltrates other regions of the brain stem, further invading into the cerebellum and thalamus via the white matter tracts, and are occasionally accompanied by leptomeningeal dissemination. Together with recent advances in molecular profiling, DIPG has been classified as a new pathologic entity called “diffuse midline glioma, H3K27M-altered” by the World Health Organization [34].
Due to the anatomical complexity and high risk of complications following biopsy, DIPG is usually diagnosed based on characteristic radiographic findings and clinical signs and symptoms. Surgical resection is hindered by its location and infiltrative nature of the tumor. Without any treatment, the prognosis is very dismal with a median survival of approximately 4 months [56]. Radiotherapy is effective as a palliative treatment, providing transient symptomatic improvement, and improves the overall survival (OS) duration by several months [67]. To date, radiotherapy has been considered as the mainstay of treatment for DIPG. Clinical trials of combined chemotherapy provided to improve the prognosis of DIPG have failed to demonstrate a meaningful survival benefit.
Although symptomatic and radiologic responses are achieved with radiotherapy, subsequent tumor progression is almost universal, with a median survival period of 8–11 months in patients with DIPG [18]. To further improve clinical outcomes, the role of alternative radiotherapy fractionation regimens has been investigated. In this review, we summarize the role of radiotherapy and various radiotherapy approaches in the treatment of DIPG.
The current standard of treatment for DIPG consists of external beam radiotherapy at a dose of 54–60 Gy with conventional fractionation (1.8–2.0 Gy daily, 5 days/week) [1]. This approach has not changed over the decades. Similar to the current recommendation, the Children’s Cancer Study Group study performed in 1977 recommended radiotherapy to be administered 5 times a week at 8–9 Gy/week up to 50–60 Gy [9]. A Korean Radiation Oncology Group (KROG) study reported that the radiotherapy dose did not change over the last 20 years in clinical practice in Korea, and most patients received 54 Gy of radiation with conventional fractionation [10].
Upfront radiotherapy results in a worthwhile, albeit temporary, improvement in neurologic function, although the overall prognosis remains dismal [111213]. The median OS is approximately 12 months after 54 Gy of conventional fractionation radiation. A systematic review of 61 studies involving 1,620 patients treated with upfront conventional radiotherapy with or without systemic therapy showed a median OS of 11.4 months [14]. Similarly, a multicenter study involving 162 patients treated with radiotherapy in Korea for 20 years showed a median OS of 13.1 months and a 2-year OS rate of 19% [10]. OS improved significantly over time, even after adjusting for other prognostic factors, to median OS of 11.4, 13.5, and 17.6 months for patients treated before 2010, during 2010–2015, and after 2015, respectively. The reason may be the improvement in socioeconomic conditions and medical insurance reimbursement because clinical practice of radiotherapy did not change during the period. Age ≤3 or >10 years and longer symptom duration at presentation are prognostic factors associated with a favorable survival outcome [815]. A Korean study [10] also confirmed these significant prognostic factors.
After an upfront radiotherapy, 70%–80% of patients respond to radiotherapy with transient improvement in neurological symptoms, which leads to improvement of quality-of-life. However, this is not sustainable in most of the patients and worsen again with progression of tumor after approximately 6 months [216]. A longitudinal study prospectively evaluating neuropsychological assessments and daily functioning of children with DIPG before and after radiotherapy showed that they exhibited short-term improvements immediately post-radiotherapy in performance-based attention tests and parent-reported behavior, including attention, hyperactivity, behavioral regulation, and executive function. However, these improvements did not persist and significant decline was documented by 6 months [17].
In planning radiotherapy, radiation oncologists determine the optimal target volume and protect the normal organs close to the tumor. However, the optimal margin for the target volume is undefined yet. Most clinical trials recommended a 1–2-cm margin in addition to the gross tumor volume (GTV) for the clinical target volume (CTV) [18192021]. GTV includes contrast-enhanced lesions on magnetic resonance imaging and hyperintense lesions on T2-weighted and fluid-attenuated inversion recovery images. An additional margin of 3–5 mm is added to obtain the planning target volume (PTV). Tinkle et al. [22] evaluated failure patterns of patients with DIPG treated with standard (1 cm) or extended (2–3 cm) margins. Among 93 patients who underwent radiographic evaluation for progression, 87% showed local progression. Central failure (Vprogression95% ≥95%) accounted for 99% of all failures. The failure patterns, progression-free survival (PFS), or OS did not differ significantly with the CTV margin. These findings suggested that an extended margin over 1 cm is not recommended, considering no apparent benefit in tumor control and the high risk of organ damage from the large target volume. Similarly, a multicenter KROG study showed that the infield failure rate was comparable among different CTV margin groups, since most failures represented infield progression (78.6% for no margin, 75.9% for <1 cm of margin, and 86% for 1–2 cm of margin; p=0.160) [10].
Over the decades, numerous trials of the alternative fractionation approach have been performed to overcome the poor prognosis of DIPG (Table 1). The principal focus of these trials has been the use of hyperfractionated radiotherapy, considering the predominantly local pattern of failure and the dose–response relationship in malignant gliomas [23]. The late-responding tissues, such as the normal brain tissues, may be spared from the effects of radiation relative to the tumor with a decreasing dose per fraction [24], which has been the theoretical basis of studies on hyperfractionated radiotherapy.
In the 1980s, the Pediatric Oncology Group (POG) performed a phase I/II trial of the efficacy and toxicity of hyperfractionated radiotherapy administered twice daily in children with brainstem glioma. Survival tended to increase with an increase in the radiation dose from 66 Gy to 72 Gy but did not change with an increase in the radiation dose from 72 Gy to 75.6 Gy [25]. To further address this issue, the POG 92-39, a phase III prospective randomized controlled trial, comparing hyperfractionated (1.17 Gy/fraction twice daily up to 70.2 Gy) and conventional (1.8 Gy/fraction once daily up to 54 Gy) radiotherapy with cisplatin showed no improvement with either approach in progression or survival [26]. A Children’s Cancer Group (CCG) phase I/II trial of the safety and efficacy of hyperfractionated radiotherapy with a dose of 78 Gy also showed no improvement in objective responses with hyperfractionated radiotherapy compared to conventional fractionation and accompanying possible radiation-associated normal tissue damage with a higher dose of radiation [27].
Gallitto et al. [14] performed a systematic review to identify all available studies until 2018 involving patients with DIPG treated with radiotherapy. Of all eligible studies, 49 (1,286 patients) used conventional fractionated radiotherapy and 8 (348 patients) used hyperfractionated radiotherapy. The median OS was 12.0 and 10.2 months in patients who received conventional and hyperfractionated radiotherapy, respectively. In the Cochrane Database of Systematic Review, a review compared hyperfractionated and conventional radiotherapy in 2016 and revealed no clear evidence of the effect on OS or event-free survival (EFS) on patients receiving hyperfractionated radiotherapy compared to conventional radiotherapy (OS: hazard ratio [HR]=1.07, 95% confidence interval [CI]: 0.75 to 1.53; EFS: HR=1.26, 95% CI: 0.83 to 1.90) [28].
Considering the paucity of data in favor of hyperfractionation radiotherapy, hyperfractionation radiotherapy is not promising in clinical practice and undesirable because of the discomfort of two sessions per day and possible damage to the healthy organs caused by higher doses.
Considering that the median survival of patients with DPIG is approximately 12 months, hypofractionated radiotherapy has been evaluated to shorten the treatment period and reduce hospital visits. Negretti et al. [19] reported a single institution’s experience with 22 patients treated with hypofractionated radiotherapy with 3 Gy/fraction up to 45 Gy over 3 weeks (Table 1). The median OS was 7.6 months, similar to that in other previous studies using conventional fractionation. However, the daily fraction was modified to 2 Gy because of intolerance in 5 cases, and one patient died from serious intracranial hypertension. A matched cohort analysis by Janssens et al. [18] compared outcomes of hypofractionated (44.8 Gy in 16 fractions or 39 Gy in 13 fractions) and conventional fractionated radiotherapy, which revealed that hypofractionated radiotherapy provided equivalent survival outcomes with shortening of the overall treatment time. Zaghloul et al. [29] performed the first related prospective randomized controlled trial involving 35 patients receiving hypofractionated radiotherapy (3 Gy/fraction up to 39 Gy) and 36 patients receiving conventional fractionation (1.8 Gy/fraction up to 54 Gy). The non-inferiority trial revealed median OS of 7.8 and 9.5 months in the hypofractionated and conventional groups, respectively, showing no significant differences, thereby failing to fulfill the non-inferiority assumption.
Another phase II randomized controlled trial from India aimed to determine whether or not hypofractionated radiotherapy (39 Gy in 13 fractions) with 75 mg/m2 body surface area of concurrent temozolomide followed by adjuvant temozolomide for 6 cycles improved the prognosis better than conventional fractionated radiotherapy (60 Gy in 30 fractions) [30]. Hypofractionated radiotherapy with temozolomide did not improve survival outcomes and accompanied higher hematologic toxicity compared to conventional fractionated radiotherapy. A recently published meta-analysis performed to overcome the small sample size of previous studies included 4 articles, involving 88 patients undergoing hypofractionated radiotherapy and 96 patients undergoing conventional fractionation radiotherapy [31]. It revealed that hypofractionated and conventional fractionation radiotherapy provided comparable OS (HR=1.07, 95% CI: 0.77–1.47) and PFS (HR= 1.04, 95% CI: 0.75–1.45) and had similar toxicity profiles. However, this result cannot change the current mainstay of conventional radiotherapy in patients with DIPG because of the small number of patients and retrospective design of some included studies. To overcome this limitation, a phase III non-inferiority randomized trial comparing hypofractionated (39 Gy in 13 fractions and 45 Gy in 15 fractions) and conventional fractionated (54 Gy in 30 fractions) radiotherapy was performed and recently published [32]. They randomized 253 patients into 3 arms of radiation regimens. The results revealed median OS of 9.6, 8.2, and 8.7 months in the 39 Gy in 13 fractions, 45 Gy in 15 fractions, and conventional groups, respectively, fulfilling their non-inferiority assumption (noninferiority margin of 15%) at 18 months OS. Younger patients (2–5 years of age) had better OS, excepting for those in 45 Gy in 15 fractions group, which may seem that the higher radiation dose with higher biologically effective dose deprived the younger patients of their inherited superior survivals.
Based on the results so far, hypofractionation radiotherapy can be performed with a dose of 39 Gy in 13 fractions in patients with DIPG according to the clinician’s discretion considering the patient’s condition and tumor location.
In all aforementioned studies, radiation was delivered as photon beams, which is the basis of external beam radiation and the treatment adopted worldwide. Proton therapy is a type of particle radiotherapy with relatively constant energy deposition up to the Bragg peak and a subsequent drop because of the depth–dose distribution of particles. It has the advantage of protecting the surrounding healthy organs by reducing the radiation dose behind the Bragg peak. Although proton therapy is theoretically useful in reducing the radiation dose to healthy brain tissue, studies investigating proton therapy in patients with DIPG scarce.
A small retrospective study from Japan reported clinical outcomes of 12 children who received proton therapy for DIPG at the University of Tsukuba Hospital [33]. The target volume was defined as similar typical radiotherapy volumes: CTV as the GTV plus a 5–10-mm margin and PTV as the CTV plus 2–3-mm margin. A total dose of 54 Gy (relative biological effectiveness [RBE]) in 30 fractions was delivered to the PTV during proton therapy.
Median PFS and OS were 5 and 9 months, respectively, showing no significant difference from the conventional photon radiotherapy group in which the median PFS and OS were 5 and 11 months, respectively. Proton therapy was well tolerated and caused a grade 3 low neutrophil count in only one patient; however, the proton therapy was not safer or more effective treatment compared to conventional photon radiotherapy.
Although proton therapy is a priority in pediatric low-grade glioma [34], it may be contraindicated in high-grade gliomas, including DIPG, because of poor outcomes and a short survival prognosis which render patients unable to benefit from the advantages of proton therapy. In addition, uncommon but serious morbidities, including symptomatic brainstem injury after proton beam radiation, have been reported [353637]. Unlike photons, protons differ in linear energy transfer (LET) and RBE, which are increased within the distal segments of the spread-out Bragg peak (SOBP). This can lead to unexpected brainstem injury. Caution should be exercised to limit brainstem exposure to the distal or lateral aspects of SOBP. Further, brainstem tolerance doses should be reduced, and RBE and LET should be accounted for [38].
Re-irradiation for patients with DIPG is an emerging salvage treatment as it can alleviate the symptoms and improve the prognosis. Fractionated re-irradiation may be considered at the time of progression, although it may increase the risk of toxicity. A few studies have been reported to date; however, a conclusive implication of re-irradiation is difficult to draw because of the small number of patients included in each study (Table 2) [39404142434445]. The first and only prospective study was a phase I/II trial conducted to determine the optimal dose of reirradiation for patients with DIPG. Three dose levels (24 Gy in 12 fractions, 26.4 Gy in 12 fractions, and 30.8 Gy in 14 fractions) were evaluated in 12 patients, and the regimen of 24 Gy in 12 fractions was preferred, considering its safety profiles and efficacy in clinical outcomes. Clinical improvement was observed in all but one patient, and quality of life was improved in almost two-thirds of the patients with a median OS of 19.5 months and median PFS of 4.5 months from the start of reirradiation. Other retrospective studies published before this study usually used a regimen of 18–20 Gy in 10 fractions and reported a median OS of 3–7 months after re-irradiation with an acceptable tolerability [3940414344]. The largest retrospective study was a matched cohort analysis performed by the European Society for Paediatric Oncology (SIOPE) high-grade glioma (HGG)/DIPG working group. It involved 31 children who underwent re-irradiation for DIPG and 39 children as the matched cohort [39]. It showed a survival benefit with re-irradiation (median OS: 13.7 months for re-irradiation vs. 10.3 months for control; p=0.04). The survival benefit was prominent with a longer interval between end-of-radiotherapy and first progression, and clinical improvement was observed in 77% of patients. Lassaletta et al. [43] published a Canadian multicenter series of 16 patients treated with re-irradiation at a dose of 21.6–36 Gy (median: 30.6 Gy) for recurrent DIPG. The median survival from progression to death was 92 days in patients without re-irradiation and 218 days in patients with re-irradiation (p=0.0001). Although the results are not conclusive, we could consider re-irradiation with a dose of 30.6–36.0 Gy in 17–20 fractions as a salvage treatment, particularly when the interval from upfront radiotherapy is long. Recently, Krishnatry and Manjali [46] reported the largest single institution series from Tata Memorial Center involving 20 patients treated with higher dose of re-irradiation (median 41.4 Gy, range 33.8–43.2 Gy) according to a response-based dose escalation approach. Clinical outcomes for all patients were comparable to previously reported studies with a median OS of 5.5 months after re-irradiation and 16.6 months from initial diagnosis, respectively. Notably, they evaluated the median OS according to the radiation dose groups (5.8 months for 39.6–41.4 Gy, 7 months for 43.2 Gy, and 5.3 months for 45 Gy group), and suggested there may be a dose-response benefit although statistical analysis was not performed due to the small sample size.
All studies reported to date have included only a small number of patients, except for the study from the SIOPE HGG/DIPG working group. To offset the limitation driven from the small cohort of each study, a systematic review and meta-analysis of seven studies involving 90 patients was recently reported by Lu et al. [47]. In this review, the pooled incidences of clinical improvement and acute serious toxicity following reirradiation were 87% and 0%, respectively. Pooled PFS and OS from the time of re-irradiation were 4.2 and 6.2 months, respectively. The pooled median OS in the re-radiation group was 18.0 months, implicating that re-irradiation may be an effective therapeutic intervention in select patients with DIPG. A non-randomized phase II trial of the efficacy of conventional fractionation re-irradiation with a dose of 30.6 Gy or 36 Gy in recurrent or progressive DIPG is underway (NCT03126266) [17].
Despite recent advances in medical technology, including molecular, imaging, and therapeutic aspects, pediatric DIPG remains an unsolved problem. To date, numerous clinical trials have explored potential therapeutic challenges in DIPG and showed no improvement in survival. Conventional radiotherapy with a dose of 54 Gy remains the only proven therapeutic option. Recent advances in molecular profiling has ushered in a new era of DIPG, leading to the development of new targeted approaches to treat DIPG. While clinical studies are required before application in clinical practice, the results of these studies may serve as a basis to discover more effective treatment options for DIPG.
Availability of Data and Material
Data sharing not applicable to this article as no datasets were generated or analyzed during the study.
References
1. Hargrave D, Bartels U, Bouffet E. Diffuse brainstem glioma in children: critical review of clinical trials. Lancet Oncol. 2006; 7:241–248. PMID: 16510333.
2. Jansen MH, van Vuurden DG, Vandertop WP, Kaspers GJ. Diffuse intrinsic pontine gliomas: a systematic update on clinical trials and biology. Cancer Treat Rev. 2012; 38:27–35. PMID: 21764221.
3. Louis DN, Perry A, Reifenberger G, von Deimling A, Figarella-Branger D, Cavenee WK, et al. The 2016 World Health Organization classification of tumors of the central nervous system: a summary. Acta Neuropathol. 2016; 131:803–820. PMID: 27157931.
4. Louis DN, Perry A, Wesseling P, Brat DJ, Cree IA, Figarella-Branger D, et al. The 2021 WHO classification of tumors of the central nervous system: a summary. Neuro Oncol. 2021; 23:1231–1251. PMID: 34185076.
5. Lassman LP, Arjona VE. Pontine gliomas of childhood. Lancet. 1967; 1:913–915. PMID: 4164397.
6. Langmoen IA, Lundar T, Storm-Mathisen I, Lie SO, Hovind KH. Management of pediatric pontine gliomas. Childs Nerv Syst. 1991; 7:13–15. PMID: 2054800.
7. Frazier JL, Lee J, Thomale UW, Noggle JC, Cohen KJ, Jallo GI. Treatment of diffuse intrinsic brainstem gliomas: failed approaches and future strategies. J Neurosurg Pediatr. 2009; 3:259–269. PMID: 19338403.
8. Jansen MH, Veldhuijzen van Zanten SE, Sanchez Aliaga E, Heymans MW, Warmuth-Metz M, Hargrave D, et al. Survival prediction model of children with diffuse intrinsic pontine glioma based on clinical and radiological criteria. Neuro Oncol. 2015; 17:160–166. PMID: 24903904.
9. Jenkin RD, Boesel C, Ertel I, Evans A, Hittle R, Ortega J, et al. Brainstem tumors in childhood: a prospective randomized trial of irradiation with and without adjuvant CCNU, VCR, and prednisone. A report of the childrens cancer study group. J Neurosurg. 1987; 66:227–233. PMID: 3806204.
10. Kim HJ, Lee JH, Kim Y, Lim DH, Park SH, Ahn SD, et al. Suggestions for escaping the dark ages for pediatric diffuse intrinsic pontine glioma treated with radiotherapy: analysis of prognostic factors from the national multicenter study. Cancer Res Treat. 2022; 03. 04. DOI: 10.4143/crt.2021.1514. [Epub].
11. Halperin EC. Pediatric brain stem tumors: patterns of treatment failure and their implications for radiotherapy. Int J Radiat Oncol Biol Phys. 1985; 11:1293–1298. PMID: 2989230.
12. Massimino M, Spreafico F, Biassoni V, Simonetti F, Riva D, Trecate G, et al. Diffuse pontine gliomas in children: changing strategies, changing results? A mono-institutional 20-year experience. J Neurooncol. 2008; 87:355–361. PMID: 18217208.
13. Chassot A, Canale S, Varlet P, Puget S, Roujeau T, Negretti L, et al. Radiotherapy with concurrent and adjuvant temozolomide in children with newly diagnosed diffuse intrinsic pontine glioma. J Neurooncol. 2012; 106:399–407. PMID: 21858607.
14. Gallitto M, Lazarev S, Wasserman I, Stafford JM, Wolden SL, Terezakis SA, et al. Role of radiation therapy in the management of diffuse intrinsic pontine glioma: a systematic review. Adv Radiat Oncol. 2019; 4:520–531. PMID: 31360809.
15. Hoffman LM, Veldhuijzen van Zanten SEM, Colditz N, Baugh J, Chaney B, et al. Clinical, radiologic, pathologic, and molecular characteristics of long-term survivors of diffuse intrinsic pontine glioma (DIPG): a collaborative report from the International and European Society for pediatric oncology DIPG registries. J Clin Oncol. 2018; 36:1963–1972. PMID: 29746225.
16. Robison NJ, Kieran MW. Diffuse intrinsic pontine glioma: a reassessment. J Neurooncol. 2014; 119:7–15. PMID: 24792486.
17. Rhodes A, Martin S, Toledo-Tamula MA, Loucas C, Glod J, Warren KE, et al. The neuropsychological profile of children with diffuse intrinsic pontine glioma (DIPG) before and after radiation therapy: a prospective longitudinal study. Child Neuropsychol. 2022; 11. 11. DOI: 10.1080/09297049.2022.2144189. [Epub].
18. Janssens GO, Jansen MH, Lauwers SJ, Nowak PJ, Oldenburger FR, Bouffet E, et al. Hypofractionation vs conventional radiation therapy for newly diagnosed diffuse intrinsic pontine glioma: a matched-cohort analysis. Int J Radiat Oncol Biol Phys. 2013; 85:315–320. PMID: 22682807.
19. Negretti L, Bouchireb K, Levy-Piedbois C, Habrand JL, Dhermain F, Kalifa C, et al. Hypofractionated radiotherapy in the treatment of diffuse intrinsic pontine glioma in children: a single institution’s experience. J Neurooncol. 2011; 104:773–777. PMID: 21327862.
20. Cohen KJ, Heideman RL, Zhou T, Holmes EJ, Lavey RS, Bouffet E, et al. Temozolomide in the treatment of children with newly diagnosed diffuse intrinsic pontine gliomas: a report from the children’s oncology group. Neuro Oncol. 2011; 13:410–416. PMID: 21345842.
21. Baxter PA, Su JM, Onar-Thomas A, Billups CA, Li XN, Poussaint TY, et al. A phase I/II study of veliparib (ABT-888) with radiation and temozolomide in newly diagnosed diffuse pontine glioma: a pediatric brain tumor consortium study. Neuro Oncol. 2020; 22:875–885. PMID: 32009149.
22. Tinkle CL, Simone B, Chiang J, Li X, Campbell K, Han Y, et al. Defining optimal target volumes of conformal radiation therapy for diffuse intrinsic pontine glioma. Int J Radiat Oncol Biol Phys. 2020; 106:838–847. PMID: 31785339.
23. Salazar OM, Rubin P, Feldstein ML, Pizzutiello R. High dose radiation therapy in the treatment of malignant gliomas: final report. Int J Radiat Oncol Biol Phys. 1979; 5:1733–1740. PMID: 231023.
24. Thames HD Jr, Withers HR, Peters LJ, Fletcher GH. Changes in early and late radiation responses with altered dose fractionation: implications for dose-survival relationships. Int J Radiat Oncol Biol Phys. 1982; 8:219–226. PMID: 7085377.
25. Freeman CR, Krischer J, Sanford RA, Burger PC, Cohen M, Norris D. Hyperfractionated radiotherapy in brain stem tumors: results of a pediatric oncology group study. Int J Radiat Oncol Biol Phys. 1988; 15:311–318. PMID: 2841262.
26. Mandell LR, Kadota R, Freeman C, Douglass EC, Fontanesi J, Cohen ME, et al. There is no role for hyperfractionated radiotherapy in the management of children with newly diagnosed diffuse intrinsic brainstem tumors: results of a pediatric oncology group phase III trial comparing conventional vs. hyperfractionated radiotherapy. Int J Radiat Oncol Biol Phys. 1999; 43:959–964. PMID: 10192340.
27. Packer RJ, Boyett JM, Zimmerman RA, Albright AL, Kaplan AM, Rorke LB, et al. Outcome of children with brain stem gliomas after treatment with 7800 cGy of hyperfractionated radiotherapy. A childrens cancer group phase 1/11 trial. Cancer. 1994; 74:1827–1834. PMID: 8082086.
28. Hu X, Fang Y, Hui X, Jv Y, You C. Radiotherapy for diffuse brainstem glioma in children and young adults. Cochrane Database Syst Rev. 2016; 2016:CD010439. PMID: 27378212.
29. Zaghloul MS, Eldebawy E, Ahmed S, Mousa AG, Amin A, Refaat A, et al. Hypofractionated conformal radiotherapy for pediatric diffuse intrinsic pontine glioma (DIPG): a randomized controlled trial. Radiother Oncol. 2014; 111:35–40. PMID: 24560760.
30. Izzuddeen Y, Gupta S, Haresh KP, Sharma D, Giridhar P, Rath GK. Hypofractionated radiotherapy with temozolomide in diffuse intrinsic pontine gliomas: a randomized controlled trial. J Neurooncol. 2020; 146:91–95. PMID: 31728883.
31. Park J, Yea JW, Park JW. Hypofractionated radiotherapy versus conventional radiotherapy for diffuse intrinsic pontine glioma: a systematic review and meta-analysis. Medicine (Baltimore). 2020; 99:e22721. PMID: 33080729.
32. Zaghloul MS, Nasr A, Tolba M, Refaat A, Youssef A, Mosaab A, et al. Hypofractionated radiation therapy for diffuse intrinsic pontine glioma: a noninferiority randomized study including 253 children. Int J Radiat Oncol Biol Phys. 2022; 113:360–368. PMID: 35150788.
33. Muroi A, Mizumoto M, Ishikawa E, Ihara S, Fukushima H, Tsurubuchi T, et al. Proton therapy for newly diagnosed pediatric diffuse intrinsic pontine glioma. Childs Nerv Syst. 2020; 36:507–512. PMID: 31728705.
34. Indelicato DJ, Rotondo RL, Uezono H, Sandler ES, Aldana PR, Ranalli NJ, et al. Outcomes following proton therapy for pediatric low-grade glioma. Int J Radiat Oncol Biol Phys. 2019; 104:149–156. PMID: 30684665.
35. Indelicato DJ, Flampouri S, Rotondo RL, Bradley JA, Morris CG, Aldana PR, et al. Incidence and dosimetric parameters of pediatric brainstem toxicity following proton therapy. Acta Oncol. 2014; 53:1298–1304. PMID: 25279957.
36. Upadhyay R, Liao K, Grosshans DR, McGovern SL, Frances McAleer M, Zaky W, et al. Quantifying the risk and dosimetric variables of symptomatic brainstem injury after proton beam radiation in pediatric brain tumors. Neuro Oncol. 2022; 24:1571–1581. PMID: 35157767.
37. Yock TI, Constine LS, Mahajan A. Protons, the brainstem, and toxicity: ingredients for an emerging dialectic. Acta Oncol. 2014; 53:1279–1282. PMID: 25327262.
38. Haas-Kogan D, Indelicato D, Paganetti H, Esiashvili N, Mahajan A, Yock T, et al. National Cancer Institute workshop on proton therapy for children: considerations regarding brainstem injury. Int J Radiat Oncol Biol Phys. 2018; 101:152–168. PMID: 29619963.
39. Janssens GO, Gandola L, Bolle S, Mandeville H, Ramos-Albiac M, van Beek K, et al. Survival benefit for patients with diffuse intrinsic pontine glioma (DIPG) undergoing re-irradiation at first progression: a matched-cohort analysis on behalf of the SIOP-E-HGG/DIPG working group. Eur J Cancer. 2017; 73:38–47. PMID: 28161497.
40. Fontanilla HP, Pinnix CC, Ketonen LM, Woo SY, Vats TS, Rytting ME, et al. Palliative reirradiation for progressive diffuse intrinsic pontine glioma. Am J Clin Oncol. 2012; 35:51–57. PMID: 21297433.
41. Freese C, Takiar V, Fouladi M, DeWire M, Breneman J, Pater L. Radiation and subsequent reirradiation outcomes in the treatment of diffuse intrinsic pontine glioma and a systematic review of the reirradiation literature. Pract Radiat Oncol. 2017; 7:86–92. PMID: 28274399.
42. Kline C, Liu SJ, Duriseti S, Banerjee A, Nicolaides T, Raber S, et al. Reirradiation and PD-1 inhibition with nivolumab for the treatment of recurrent diffuse intrinsic pontine glioma: a single-institution experience. J Neurooncol. 2018; 140:629–638. PMID: 30206764.
43. Lassaletta A, Strother D, Laperriere N, Hukin J, Vanan MI, Goddard K, et al. Reirradiation in patients with diffuse intrinsic pontine gliomas: the Canadian experience. Pediatr Blood Cancer. 2018; 65:e26988. PMID: 29369515.
44. Massimino M, Biassoni V, Miceli R, Schiavello E, Warmuth-Metz M, Modena P, et al. Results of nimotuzumab and vinorelbine, radiation and re-irradiation for diffuse pontine glioma in childhood. J Neurooncol. 2014; 118:305–312. PMID: 24696052.
45. Amsbaugh MJ, Mahajan A, Thall PF, McAleer MF, Paulino AC, Grosshans D, et al. A phase 1/2 trial of reirradiation for diffuse intrinsic pontine glioma. Int J Radiat Oncol Biol Phys. 2019; 104:144–148. PMID: 30610915.
46. Krishnatry R, Manjali JJ. Clinical approach to re-irradiation for recurrent diffuse intrinsic pontine glioma. Jpn J Clin Oncol. 2021; 51:762–768. PMID: 33521824.
47. Lu VM, Welby JP, Mahajan A, Laack NN, Daniels DJ. Reirradiation for diffuse intrinsic pontine glioma: a systematic review and meta-analysis. Childs Nerv Syst. 2019; 35:739–746. PMID: 30879125.
48. Allen J, Siffert J, Donahue B, Nirenberg A, Jakacki R, Robertson P, et al. A phase I/II study of carboplatin combined with hyperfractionated radiotherapy for brainstem gliomas. Cancer. 1999; 86:1064–1069. PMID: 10491535.
49. Packer RJ, Zimmerman RA, Kaplan A, Wara WM, Rorke LB, Selch M, et al. Early cystic/necrotic changes after hyperfractionated radiation therapy in children with brain stem gliomas. Data from the Childrens Cancer Group. Cancer. 1993; 71:2666–2674. PMID: 8453590.
50. Freeman CR, Krischer JP, Sanford RA, Cohen ME, Burger PC, del Carpio R, et al. Final results of a study of escalating doses of hyperfractionated radiotherapy in brain stem tumors in children: a Pediatric Oncology Group study. Int J Radiat Oncol Biol Phys. 1993; 27:197–206. PMID: 8407392.
51. Kretschmar CS, Tarbell NJ, Barnes PD, Krischer JP, Burger PC, Kun L. Pre-irradiation chemotherapy and hyperfractionated radiation therapy 66 Gy for children with brain stem tumors. A phase II study of the Pediatric Oncology Group, Protocol 8833. Cancer. 1993; 72:1404–1413. PMID: 8339231.
52. Freeman CR, Krischer J, Sanford RA, Cohen ME, Burger PC, Kun L, et al. Hyperfractionated radiation therapy in brain stem tumors. Results of treatment at the 7020 cGy dose level of Pediatric Oncology Group study #8495. Cancer. 1991; 68:474–481. PMID: 2065266.
53. Hankinson TC, Patibandla MR, Green A, Hemenway M, Foreman N, Handler M, et al. Hypofractionated radiotherapy for children with diffuse intrinsic pontine gliomas. Pediatr Blood Cancer. 2016; 63:716–718. PMID: 26544789.
54. Zamora PL, Miller SR, Kovoor JJ. Single institution experience in reirradiation of biopsy-proven diffuse intrinsic pontine gliomas. Childs Nerv Syst. 2021; 37:2539–2543. PMID: 33973056.
Table 1
Clinical features of studies with hyperfractionated or hypofractionated RT in diffuse intrinsic pontine glioma
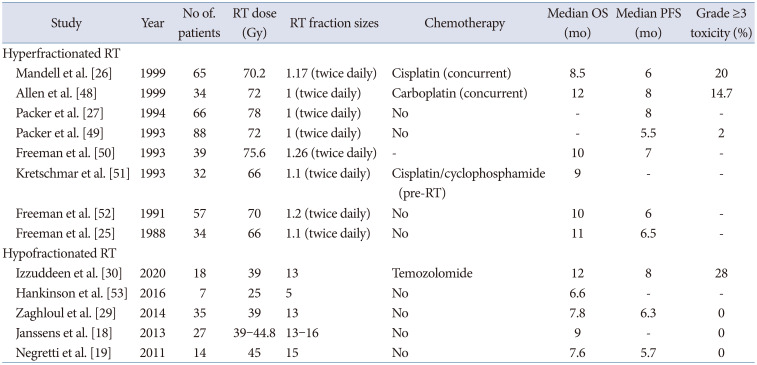
Study | Year | No of. patients | RT dose (Gy) | RT fraction sizes | Chemotherapy | Median OS (mo) | Median PFS (mo) | Grade ≥3 toxicity (%) | |
---|---|---|---|---|---|---|---|---|---|
Hyperfractionated RT | |||||||||
Mandell et al. [26] | 1999 | 65 | 70.2 | 1.17 (twice daily) | Cisplatin (concurrent) | 8.5 | 6 | 20 | |
Allen et al. [48] | 1999 | 34 | 72 | 1 (twice daily) | Carboplatin (concurrent) | 12 | 8 | 14.7 | |
Packer et al. [27] | 1994 | 66 | 78 | 1 (twice daily) | No | - | 8 | - | |
Packer et al. [49] | 1993 | 88 | 72 | 1 (twice daily) | No | - | 5.5 | 2 | |
Freeman et al. [50] | 1993 | 39 | 75.6 | 1.26 (twice daily) | - | 10 | 7 | - | |
Kretschmar et al. [51] | 1993 | 32 | 66 | 1.1 (twice daily) | Cisplatin/cyclophosphamide (pre-RT) | 9 | - | - | |
Freeman et al. [52] | 1991 | 57 | 70 | 1.2 (twice daily) | No | 10 | 6 | - | |
Freeman et al. [25] | 1988 | 34 | 66 | 1.1 (twice daily) | No | 11 | 6.5 | - | |
Hypofractionated RT | |||||||||
Izzuddeen et al. [30] | 2020 | 18 | 39 | 13 | Temozolomide | 12 | 8 | 28 | |
Hankinson et al. [53] | 2016 | 7 | 25 | 5 | No | 6.6 | - | - | |
Zaghloul et al. [29] | 2014 | 35 | 39 | 13 | No | 7.8 | 6.3 | 0 | |
Janssens et al. [18] | 2013 | 27 | 39–44.8 | 13–16 | No | 9 | - | 0 | |
Negretti et al. [19] | 2011 | 14 | 45 | 15 | No | 7.6 | 5.7 | 0 |
Table 2
Clinical features of studies with re-irradiation in diffuse intrinsic pontine glioma
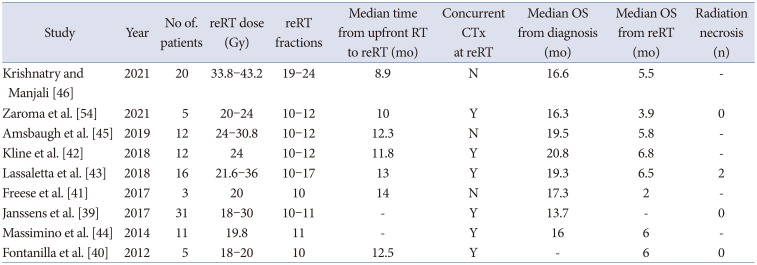
Study | Year | No of. patients | reRT dose (Gy) | reRT fractions | Median time from upfront RT to reRT (mo) | Concurrent CTx at reRT | Median OS from diagnosis (mo) | Median OS from reRT (mo) | Radiation necrosis (n) |
---|---|---|---|---|---|---|---|---|---|
Krishnatry and Manjali [46] | 2021 | 20 | 33.8–43.2 | 19–24 | 8.9 | N | 16.6 | 5.5 | - |
Zaroma et al. [54] | 2021 | 5 | 20–24 | 10–12 | 10 | Y | 16.3 | 3.9 | 0 |
Amsbaugh et al. [45] | 2019 | 12 | 24–30.8 | 10–12 | 12.3 | N | 19.5 | 5.8 | - |
Kline et al. [42] | 2018 | 12 | 24 | 10–12 | 11.8 | Y | 20.8 | 6.8 | - |
Lassaletta et al. [43] | 2018 | 16 | 21.6–36 | 10–17 | 13 | Y | 19.3 | 6.5 | 2 |
Freese et al. [41] | 2017 | 3 | 20 | 10 | 14 | N | 17.3 | 2 | - |
Janssens et al. [39] | 2017 | 31 | 18–30 | 10–11 | - | Y | 13.7 | - | 0 |
Massimino et al. [44] | 2014 | 11 | 19.8 | 11 | - | Y | 16 | 6 | - |
Fontanilla et al. [40] | 2012 | 5 | 18–20 | 10 | 12.5 | Y | - | 6 | 0 |