Abstract
The three-dimensional (3D) printing itself is not a novel technology, it is more than 30 years old. Stereolithographic (SLA) technology has been used as the first and popular technology for medical application of 3D printing. Since 1991 Radiology and Plastic Surgery have published articles about SLA for rapid prototyping anatomical 3D models. Medical applications of 3D printing have been popularizing and stabilizing so far. Implantable medical devices such as metal or absorbable implants, surgical guide systems, prosthesis and orthosis, and 3D anatomical models for normal or diseased anatomy have been developing and expanding its markets so far. There are many obstacles, such as insurance, authorization as a medical device, and lack of standards technology for further expansion of medical applications. Many technical specifications and guidelines for authorization as medical device have been published by regulatory bodies from many countries. Even though international standards for 3D printing have been developing more and more, there have been few standards for medical application of 3D printing. In this harsh environment academia, company, research institute, regulatory bodies, and government have been doing good job for the development of 3D printing industry.
Medical applications of three-dimensional (3D) printing seem to be doing quite a bit reliably. From surgical explanations for patients or their family using 3D models, which were performed in various ways in the early stages, to surgical rehearsals, etc., are becoming stable. Accordingly, neurosurgery, orthopedics, plastic surgery, and dental applications using 3D-printed metal parts are very active and have become one of the options for patient treatment. Since Ashley [1] introduced rapid prototyping (RP) systems in 1991, RP by stereolithographic (SLA) technology has been popularizing and common technology in medical applications of 3D printing [23]. For medical 3D printing, the starting point is medical images from CT/MRI by Digital Imaging and Communication in Medicine (DICOM) formats. Since the nature of image processing, radiology experts have been the leader of medical 3D printing. There is Special Interest Group for 3D printing (3D printing SIG) within Radiological Society of North America (RSNA). They are very active group and have been trying to establish standard medical practice based on 3D printing. And also 3D printing SIG has been communicating with regulatory bodies such as US Food and Drug Administration (US FDA) and medical insurance for authorization and reimbursement [4]. In neurosurgery, the process of verifying the plan using a 3D model and verifying the plan through virtual surgery is established and carried out for pediatric craniofacial deformities. Accordingly, it is common to manufacture a surgical guide and precisely apply the surgical plan to the patient. So far presurgical planning and simulation surgery using RP 3D models have been established and become standard practice [567]. Bone defects occur in various situations, and reconstructing them is a long-cherished dream for neurosurgeons. At the beginning of 3D printing for skull defects, indirect manufacturing of metal plates for cranioplasty was used by pre-surgical bending and customization from pre-made titanium plates [8]. Various materials and various methods have been attempted for complicated and complex large-sized defects, but reconstruction using metal 3D printing has significantly overcome these difficulties [68910]. Metal 3D printing enables the production of prostheses in a shape and structure that is easy to insert to fit the bone defect, and allows the prosthesis to be produced according to the surgeon’s intention so that it can be optimized for the surgery [6910].
Although it has emerged as a new technology for more than 30 years, it has been fully applied to medical care within 10 years, so there are various problems with technical guidelines and medical device licensing, and cost problems still remain. International Organization for Standardization (ISO), International Electrotechnical Commission (IEC), Joint Technical Committee 1 (JTC1), International Medical Device Regulatory Forum (IMDRF), and other international standards and medical device regulatory organizations are working to establish related standards, and the Korean Ministry of Food and Drug Safety (MFDS) is making great efforts based on them in Korea. 3D printing, medical devices, medical application, and international standard are mainly used to search literatures and references for this article.
The term of “3D printing” is defined as “fabrication of objects through the deposition of a material using a print head, nozzle or another printer technology” by ISO/ASTM 52900: 2021(en) [11].
3D printing, also known as additive manufacturing (AM), is defined as “a process of joining materials to make parts from 3D model data, usually layer upon layer, as opposed to subtractive manufacturing and formative manufacturing methodologies” by ISO/ASTM 52900:2021(en). Historical terms include additive fabrication, additive processes, additive techniques, additive layer manufacturing, layer manufacturing, solid freeform fabrication, and freeform fabrication.
It involves using a computer-controlled machine to build objects layer by layer, using materials such as plastic, metal, ceramic, and other suitable materials. The process starts with a digital 3D model of the object, which is created using computer-aided design (CAD) software or scanned using a 3D scanner. The digital model is then sliced into thin layers by slicing software, and the 3D printer or AM machine builds the object by depositing the material in these layers according to the instructions in the digital 3D model.
There are seven process categories used in 3D printing, including binder jetting, directed energy deposition, material extrusion, material jetting, powder bed fusion, sheet lamination, and vat photopolymerization by ISO/ASTM 52900:2021(en). Each of these technologies has its own advantages and limitations and is suitable for different types of objects and materials. 3D printing is used in a wide range of applications, including prototyping, manufacturing, and custom fabrication of products. It has the potential to revolutionize many industries by enabling the rapid and cost-effective production of custom, complex parts and products.
The applications of 3D printing are many positive and negative aspects. High versatility of design, customization which has been impossible to be mass manufacturing, highly efficient manufacturing which has no way to be possible so far, and simplified and regional manufacturing are advantages of 3D printing. There are still many limitations such as not easy to reach higher level of designing and quality control, not suitable for mass production, limitation of size and speed of manufacturing, and limitation of materials. Table 1 shows summary of its applications and most used 3D printing technologies and materials.
Trauma, infection, and tumor surgery are major causes of a cranial bone defect and result in aesthetic and functional deficiencies [121314]. Depressed scalp and inappropriate cerebrospinal fluid circulation will affect the brain function and also asymmetry of calvaria can affect social relationship. Syndrome of trephined is well known neurologic symptoms like dizziness, irritability, anxiety, and intolerance [1314]. Calvarial reconstruction should cerebral protection, restoration of the cerebrospinal fluid dynamics, provide biomechanical stability and cranial contour for cosmetic appearance. While autologous bone is most widely used today, 30–90 days after craniectomy is the time limitation of re-use of autologous bone. Bi-halving of autologous bone is not suitable for significantly large defects and requires synthetic materials such as inert metals, ceramics, plastics, and absorbable polymers. Medical image-based modeling, CAD software, and 3D printing technology can customize patient specific or matched implant to the shape of individual defects and its purpose [910].
The patient-matched medical device and custom-made medical device are different by definition of International Medical Device Regulatory Forum (IMDRF; IMDRF/PMD WG/N49 FINAL:2018 Definitions for Personalized Medical Devices; https://www.imdrf.org/documents/definitions-personalized-medical-devices). The custom-made medical device is specifically made in accordance with a written request of an authorized healthcare professional, which gives, under their responsibility, specific design characteristics and is intended to address the specific anatomo-physiological features or pathological condition of the individual for whom it is intended. The patient-matched medical device is matched to a patient’s anatomy within a specified design envelope using techniques such as scaling of the device based on anatomic references, or by using the full anatomic features from patient imaging, and it is designed and produced under the responsibility of a manufacturer even though the design may be developed in consultation with an authorized healthcare professional.
The processes for manufacturing 3D printing implant for medical applications consist of image acquisition phase, segmentation phase, 3D modeling phase, 3D printing phase, post-processing phase, quality control phase, clinical application and review phase, and post-market phase (ISO/IEC DIS 3532-1:2021(E)) (Fig. 1) [15].
The titanium alloy is the most used material for manufacturing 3D printing implant for skeletal reconstruction and powder bed fusion technology using laser or electron beam are mostly used as well. The titanium implants for skull defects are manufactured by using 3D CT data, medical image processing software, and an electron beam melting machine. For each patient and calvarial defect, the engineer and surgeon shall try several different implant designs against 3D-printed skull models. Design factors (thickness, fixation type, porosity, augmentation or reduction of contour, etc.) should be considered before this step. The final implant design will incorporate 2 mm thickness implants for bone-like rigidity. After manufacturing the 3D implant, the operation is simulated with it to the patient’s RP model [6910]. It takes at least 5 days to manufacture the 3D printing skull implant from 3D CT. The required time for manufacturing depends on the complexity of the implant and combining surgical guides. It takes more than 2 weeks for complex and complicated pelvic surgery to manufacture 3D printing implants and surgical guides.
Metal-based implantable devices are not always suitable for reconstruction. Biodegradable 3D printing refers to the use of 3D printing technology to create objects that are made from biodegradable materials (polylactic acid [PLA], polycaprolactone [PCL], polycaprolactone/beta-tricalcium phosphate [PCL/β-TCP], etc.) that can be broken down and absorbed by the body over time. Craniofacial reconstruction and regeneration have been tried with these novel technologies in Korea [161718]. Biodegradable materials have several advantages over non-biodegradable materials in the production of medical devices. They can be safely absorbed by the body, reducing the risk of infection or rejection. They also reduce the need for surgical removal of the device, which can be costly and inconvenient for the patient. The combination of 3D-printed scaffold and a tissue-engineering technique with an optimal cell culture have been researched to obtain a precise and delicate cartilage implant without donor-site morbidity or major complications [1920]. Metal implant can be a replacement or cover defects and repair deformities. Bio 3D printing can be a regenerative or restoring technology for anatomical defects and functional disabilities.
It is very important for limb assistant devices to fit patient’s body parts. 3D printing technology will be the most suitable technique for this and can be made to be lightweight and comfortable for the user. 3D printing can create custom-fit prosthetics for limbs (the leg, foot, arm, and hand) including partial upper or lower limb prosthetics, ankle-foot prosthetics, and knee-ankle-foot prosthetics. These devices can be designed to mimic the appearance and function of the missing limb. Custom-fit craniofacial prosthetics for the face and head, including ears, nose, and eyes can be created by 3D printing technology. Orthopedic braces for the knees, ankles, wrists, and other joints can be manufactured by 3D printing with custom-fit braces. These devices can be designed to provide support and stability to the joint. Spinal custom-fit orthotics (scoliosis braces and spinal fusion cages) can also be created. These devices can be designed to support the spine and correct any abnormalities. Custom-fit orthotics for the feet, including insoles and arch supports can be designed to provide support and comfort for the feet. 3D scanning is also critical technology for custom-fit prosthesis and orthosis. Classical manufacturing of these devices is done by cast and mold technique. 3D scanning can obtain 3D surface data directly from patient and process within image processing software to produce 3D rendering image of patient. The prosthesis or orthosis can be designed to anatomically and functionally fit individual patients.
For complex and complicated bone surgery such as pelvic bone tumors wide resection and effective reconstruction should be critical for patient’s survival and functional recovery. If it is possible to be reconstructed as it was, the wide resection with enough safe margin around the tumor will be done. To ensure postoperative function of the salvaged limb joints, normal bone stock, and important ligaments and tendons should be maximally preserved. To achieve a wide bone margin and maximize limb function after limb salvage surgery, various techniques have been attempted such as a fluoroscopic radiologic imaging system, a navigation system [161821], an augmented reality system [22], and PSI, including a 3D-printed patient-specific bone tumor resection guide (PS-BTRG) [232425]. 3D printing technology has been used in the production of surgical guides, which are devices that are used to assist surgeons in performing complex procedures. Surgical guides can be used to provide precise guidance for the placement of implants or other devices, or to help surgeons navigate through the body during surgery. CT images of the affected bone are used to identify the bony structure for the cutting guide design. MRI is utilized to evaluate the tumor boundary. MRI is especially useful when a tumor formed an intramedullary lesion without definite bone destruction or a wide tumor reactive zone is planned for resection. In most cases, both CT and MRI are used in the initial design process [24]. The surgeon performing the excision shall plan and decide the surgical approach, preservation of critical structures, the safe cutting level with sufficient margin, the appropriate trajectory of a bone cutting saw, and the reconstruction option in designing the 3D-printed PS-BTRG. The surgeon and engineer must communicate each other thoroughly to make the decision for the final version of the 3D-printed PS-BTRG. The surgical guides are usually manufactured by biocompatible resins such as MED610 which is medically approved and rigid enough to hold the surgical instruments.
There are some potential pros and cons of using 3D printing to produce surgical guides. Customization, precision, and speed are the advantages of 3D printing-based surgical guides. However, cost, material limitations, complexity, and sterilization are obstacles to the use of 3D printing-based surgical guides. The use of 3D printing in the production of surgical guides can provide many benefits.
3D printing can be used to create physical models of a patient’s anatomy, which can be used for surgical planning, simulations, and rehearsals. These models are created from medical imaging data, such as CT or MRI scans, and can provide a detailed, accurate representation of the patient’s anatomy and diseased status. There are several ways that 3D-printed models can be used in surgical planning and simulations. For pre-surgical planning surgeons can use 3D-printed models to plan and practice complex procedures before the surgery takes place. This can help the surgeon to understand the patient’s anatomy and identify any potential challenges that may arise during the surgery. 3D-printed models can be used to communicate surgical plans and procedures to other members of the surgical team, as well as to the patient and their family. This can help to ensure that everyone is on the same page and that the patient is fully informed about the procedure. 3D-printed models can be used to rehearse surgical procedures in a simulated environment, which can help the surgeon to become familiar with the patient’s anatomy and improve their skills. 3D-printed models can be used to train surgical residents and other medical professionals in the use of new technologies or procedures. Fig. 2 provides the typical workflow and clinical practice of pre-surgical planning and simulation surgery.
3D printing can be a valuable tool for education, as it allows students to create physical objects and prototypes that can help them to better understand complex concepts and ideas. 3D printing enables students to create physical objects, which can be a more engaging and interactive way to learn compared to traditional methods such as reading or listening to lectures. Hands-on learning can be achieved by creating physical models of objects or structures that are difficult or impossible to bring into the classroom, such as fossils, molecules, or historical landmarks. 3D printing allows students to design and print their own prototypes or models of objects, encouraging creativity and problem-solving skills and provides a hands-on, experiential learning opportunity for students to learn about the design and fabrication process. 3D printing also enables students to create custom parts or tools for projects or experiments, such as custom-fitted prosthetics or robotics components, and facilitates the creation of low-cost, customized learning materials, such as manipulatives or educational games. Students will be encouraged to perform collaboration and teamwork as students work together to design and print objects or solve problems using 3D printing technology. 3D printing can be used to create highly detailed and accurate models of the human body or diseased models, which can be used for educational purposes or for practicing medical procedures.
The MFDS has published several technical specifications, guidelines, and regulations for the authorization of 3D-printed medical devices (orthopedics, dentistry, bio-printing, etc.) which outline the requirements that must be met in order to obtain approval for these products. According to the MFDS guidelines, 3D-printed medical devices must meet the same safety and performance standards as other medical devices and must be subject to the same regulatory requirements. In order to obtain authorization, manufacturers of 3D-printed medical devices must submit a detailed application to the MFDS, including information on the design, materials, and intended use of the device, as well as data on its safety and effectiveness.
The MFDS also requires manufacturers of 3D-printed medical devices to maintain quality management systems (QMS) that meet the requirements of ISO 13485 which specifies the requirements for a QMS that is specifically designed for the manufacture of medical devices [26]. Good manufacturing practices (GMP) regulations for 3D printing medical devices are published recently by MFDS and specify the requirements for the manufacture of 3D-printed medical devices [27]. They cover areas such as medical image data processing, designing, manufacturing including post-processing, testing, and quality control.
US FDA has developed their guidance to provide the Agency’s initial thinking on technical considerations specific to devices using AM, the broad category of manufacturing encompassing 3D printing at 2017 [28]. This guidance is broadly organized into two topic areas: design and manufacturing considerations (Section V) and device testing considerations (Section VI).
ISO/IEC JTC 1 for information technology is committed to developing, maintaining, promoting, and facilitating information technology (IT) standards required by global markets meeting business and user requirements. Over 2,000 experts from 163 countries develop mutually beneficial guidelines that enhance global trade while protecting intellectual property. ISO/IEC JTC 1/WG 12 is working group for 3D printing and scanning. ISO/IEC FDIS 3532-1 Information technology—Medical image-based modelling for 3D printing—Part 1: General requirements [15]; ISO/IEC DIS 3532-2 Information technology—Medical image-based modelling for 3D printing—Part 2: Segmentation [29]; ISO/IEC AWI 8803 Information Technology—3D Printing and Scanning—Accuracy and precision evaluation process for modeling from 3D scanned data [30]; ISO/IEC AWI 16466 Information Technology—3D Printing and scanning—Assessment methods of 3D scanned data for 3D printing model [31]; and ISO/IEC AWI 8801 Information Technology—3D Printing and Scanning—3D scanned and labeled data Standard Operating Procedure (SOP) for evaluation of modelling from 3D scanned data [32] are under development and related to medical applications. These five standards will provide requirements and guidelines for medical image data processing and assessments of its conformity for 3D printing and also they are applicable for medical applications of 3D printing.
International standardization activities for 3D printing/AM are mainly happening within ISO/TC 261 which is technical committee for AM. The scope of ISO/TC 261 is standardization in the field of AM concerning their processes, terms and definitions, process chains (hard- and software), test procedures, quality parameters, supply agreements, and all kinds of fundamentals. The technical report of ISO/ASTM TR 52916:2022 Additive manufacturing for medical—Data—Optimized medical image data has been published recently [11]. This document provides the review of the medical image data and its optimization. ISO/TC 150 is technical committee for implant for surgery. ISO/TC 261 and ISO/TC 150 formed joint working group of ISO/TC 150 JWG 1 which is Joint ISO/TC 150–ISO/TC 261 WG: Additive manufacturing in surgical implant applications. ISO/AWI 5092 Additive manufacturing for medical—General principles—Roadmap to safe and effective additively manufactured implants has been developed within this JWG 1 [33]. This document identifies factors that affect the safety and performance of surgical implants due to the fact these implants are manufactured additively. This document applies to non-active implants manufactured additively, including custom-made implants and patient-specific implants. This document does not apply to tissue-engineered implants manufactured by AM.
The 3D printing industry is expanding and extending its applications and markets. Certain areas of applications are stabilizing such as metal 3D printing implants and 3D printing model for simulation. However, there are still several obstacles to further advancement such as reimbursement, regulations and authorizations, and limited international standards or guidelines for quality control to be applicable to medical applications.
Availability of Data and Material
Data sharing not applicable to this article as no datasets were generated or analyzed during the study.
References
1. Ashley S. Rapid prototyping systems. Mech Eng. 1991; 113:34–43.
2. Barker TM, Earwaker WJ, Frost N, Wakeley G. Integration of 3-D medical imaging and rapid prototyping to create stereolithographic models. Australas Phys Eng Sci Med. 1993; 16:79–85. PMID: 8357307.
3. Mankovich N, Yue A, Ammirati M, Kioumehr F, Turner S. Solid models for CT/MR image display: accuracy and utility in surgical planning. In : Proceedings of Medical Imaging ‘91; 1991 Feb 24-28; San Jose, CA, USA. SPIE;1991.
4. Radiological Society of North America. 3D printing special interest group [Internet]. Oak Brook, IL: Radiological Society of North America;Accessed Dec 27, 2022. at: https://www.rsna.org/membership/involvement-opportunities/3d-printing-special-interest-group.
5. D’Urso PS, Atkinson RL, Lanigan MW, Earwaker WJ, Bruce IJ, Holmes A, et al. Stereolithographic (SL) biomodelling in craniofacial surgery. Br J Plast Surg. 1998; 51:522–530. PMID: 9924405.
6. Cho HR, Roh TS, Shim KW, Kim YO, Lew DH, Yun IS. Skull reconstruction with custom made three-dimensional titanium implant. Arch Craniofac Surg. 2015; 16:11–16. PMID: 28913212.
7. D’Urso PS, Earwaker WJ, Barker TM, Redmond MJ, Thompson RG, Effeney DJ, et al. Custom cranioplasty using stereolithography and acrylic. Br J Plast Surg. 2000; 53:200–204. PMID: 10738323.
8. Winder J, Cooke RS, Gray J, Fannin T, Fegan T. Medical rapid prototyping and 3D CT in the manufacture of custom made cranial titanium plates. J Med Eng Technol. 1999; 23:26–28. PMID: 10202700.
9. Park EK, Lim JY, Yun IS, Kim JS, Woo SH, Kim DS, et al. Cranioplasty enhanced by three-dimensional printing: custom-made three-dimensional-printed titanium implants for skull defects. J Craniofac Surg. 2016; 27:943–949. PMID: 27192643.
10. Park SE, Park EK, Shim KW, Kim DS. Modified cranioplasty technique using 3-dimensional printed implants in preventing temporalis muscle hollowing. World Neurosurg. 2019; 126:e1160–e1168. PMID: 30880206.
11. ISO. ISO/ASTM 52900:2021. Additive manufacturing — General principles — Fundamentals and Vocabulary [Internet]. Geneva: ISO;Accessed Dec 27, 2022. at: https://www.iso.org/standard/74514.html.
12. Durand JL, Renier D, Marchac D. [The history of cranioplasty]. Ann Chir Plast Esthet. 1997; 42:75–83. French. PMID: 9768140.
13. Dujovny M, Aviles A, Agner C, Fernandez P, Charbel FT. Cranioplasty: cosmetic or therapeutic? Surg Neurol. 1997; 47:238–241. PMID: 9068693.
14. Dujovny M, Agner C, Aviles A. Syndrome of the trephined: theory and facts. Crit Rev Neurosurg. 1999; 9:271–278. PMID: 10525845.
15. ISO. ISO/IEC 3532-1:2023. Information technology — Medical image-based modelling for 3D printing — Part 1: general requirements [Internet]. Geneva: ISO;Accessed Dec 27, 2022. at: https://www.iso.org/standard/79624.html.
16. Han HH, Shim JH, Lee H, Kim BY, Lee JS, Jung JW, et al. Reconstruction of complex maxillary defects using patient-specific 3D-printed biodegradable scaffolds. Plast Reconstr Surg Glob Open. 2018; 6:e1975. PMID: 30881789.
17. Jung BK, Kim JY, Kim YS, Roh TS, Seo A, Park KH, et al. Ideal scaffold design for total ear reconstruction using a three-dimensional printing technique. J Biomed Mater Res B Appl Biomater. 2019; 107:1295–1303. PMID: 30261122.
18. Lee S, Choi D, Shim JH, Nam W. Efficacy of three-dimensionally printed polycaprolactone/beta tricalcium phosphate scaffold on mandibular reconstruction. Sci Rep. 2020; 10:4979. PMID: 32188900.
19. Lee JS, Kim BS, Seo D, Park JH, Cho DW. Three-dimensional cell printing of large-volume tissues: application to ear regeneration. Tissue Eng Part C Methods. 2017; 23:136–145. PMID: 28093047.
20. Lee JM, Sultan MT, Kim SH, Kumar V, Yeon YK, Lee OJ, et al. Artificial auricular cartilage using silk fibroin and polyvinyl alcohol hydrogel. Int J Mol Sci. 2017; 18:1707. PMID: 28777314.
21. Wong KC, Kumta SM. Computer-assisted tumor surgery in malignant bone tumors. Clin Orthop Relat Res. 2013; 471:750–761. PMID: 22948530.
22. Cho HS, Park YK, Gupta S, Yoon C, Han I, Kim HS, et al. Augmented reality in bone tumour resection: an experimental study. Bone Joint Res. 2017; 6:137–143. PMID: 28258117.
23. Wong KC, Kumta SM, Sze KY, Wong CM. Use of a patient-specific CAD/CAM surgical jig in extremity bone tumor resection and custom prosthetic reconstruction. Comput Aided Surg. 2012; 17:284–293. PMID: 23030839.
24. Park JW, Kang HG, Lim KM, Park DW, Kim JH, Kim HS. Bone tumor resection guide using three-dimensional printing for limb salvage surgery. J Surg Oncol. 2018; 118:898–905. PMID: 30261096.
25. Dong C, Beglinger I, Krieg AH. Personalized 3D-printed guide in malignant bone tumor resection and following reconstruction - 17 cases in pelvic and extremities. Surg Oncol. 2022; 42:101733. PMID: 35397377.
26. ISO. ISO 13485:2016. Medical devices — Quality management systems — Requirements for regulatory purposes [Internet]. Geneva: ISO;Accessed Dec 27, 2022. at: https://www.iso.org/standard/59752.html.
27. Ministry of Food and Drug Safety. Good manufacturing practices (GMP) for custom made medical device manufactured by 3D printing [Internet]. Cheongju: Ministry of Food and Drug Safety;Accessed Dec 27, 2022. at: https://www.mfds.go.kr/brd/m_1060/view.do?seq=14360.
28. FDA. Technical considerations for additive manufactured medical devices [Internet]. Silver Spring, MD: FDA;Accessed Dec 27, 2022. at: https://www.fda.gov/regulatory-information/search-fda-guidance-documents/technical-considerations-additive-manufactured-medical-devices.
29. ISO. ISO/IEC DIS 3532-2. Information technology — Medical image-based modelling for 3D printing — Part 2: segmentation [Internet]. Geneva: ISO;Accessed Dec 27, 2022. at: https://www.iso.org/standard/79625.html.
30. ISO. ISO/IEC AWI 8803. Information technology — 3D printing and scanning — Accuracy and precision evaluation process for modeling from 3D scanned data [Internet]. Geneva: ISO;Accessed Dec 27, 2022. at: https://www.iso.org/standard/84598.html.
31. ISO. ISO/IEC AWI 16466. Information technology — 3D printing and scanning — Assessment methods of 3D scanned data for 3D printing model [Internet]. Geneva: ISO;Accessed Dec 27, 2022. at: https://www.iso.org/standard/84599.html.
32. ISO. ISO/IEC AWI 8801. Information technology — 3D printing and scanning— 3D scanned and labeled data standard operating procedure (SOP) for evaluation of modelling from 3D scanned data [Internet]. Geneva: ISO;Accessed Dec 27, 2022. at: https://www.iso.org/standard/84597.html.
33. ISO. ISO/AWI 5092. Additive manufacturing for medical — General principles — Roadmap to safe and effective additively manufactured implants [Internet]. Geneva: ISO;Accessed Dec 27, 2022. at: https://www.iso.org/standard/80762.html.
Fig. 2
Typical example for pre-surgical planning and simulation surgery. A: Two pieces of implants for fronto-orbital reconstruction. B: Orbital implant was tried on rapid prototyping (RP) model. C: Frontal implant was tried on frontal defect. D: Final simulated RP model with two pieces of implants. E: Surgical view.
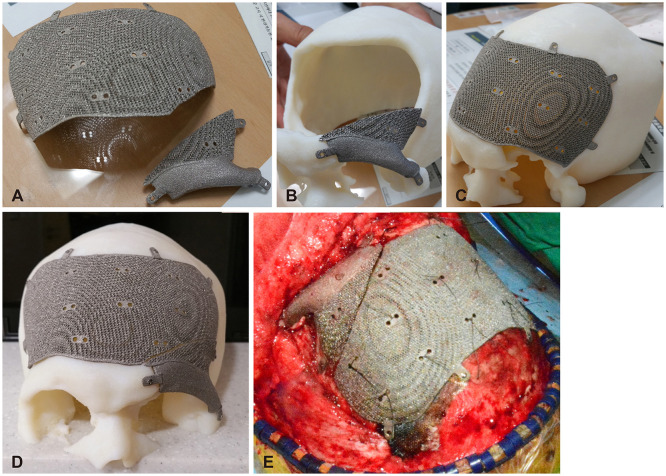
Table 1
Medical applications of 3D printing
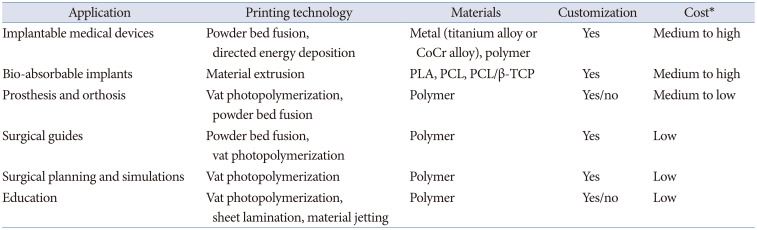