Abstract
Patients with diabetes mellitus are highly susceptible to cardiovascular complications, which are directly correlated with cardiovascular morbidity and mortality. In addition to coronary artery disease, there is growing awareness of the risk and prevalence of heart failure (HF) in patients with diabetes. Echocardiography is an essential diagnostic modality commonly performed in patients with symptoms suggestive of cardiovascular diseases (CVD), such as dyspnea or chest pain, to establish or rule out the cause of symptoms. Conventional echocardiographic parameters, such as left ventricular ejection fraction, are helpful not only for diagnosing CVD but also for determining severity, treatment strategy, prognosis, and response to treatment. Echocardiographic myocardial strain, a novel echocardiographic technique, enables the detection of early changes in ventricular dysfunction before HF symptoms develop. This article aims to review the role of echocardiography in evaluating CVD in patients with diabetes mellitus and how to use it in patients with suspected cardiac diseases.
Prolonged hyperglycemia in individuals with diabetes mellitus (DM) is linked to chronic organ damage. DM constitutes a significant risk factor for the onset of atherosclerotic cardiovascular diseases (CVD), including peripheral artery disease, cerebrovascular disease, and coronary artery disease (CAD) [1]. Recently, there has been growing interest in heart failure (HF) as an overlooked cardiovascular complication in patients with diabetes [2].
Echocardiography is the most effective imaging modality for the assessment of patients exhibiting cardiac symptoms such as dyspnea or chest pain. It plays a pivotal role in identifying or ruling out cardiogenic dyspnea and other causes. In patients with CVD, echocardiography provides important information on the severity of the disease, the decision-making process for treatment strategies, prognosis prediction, and treatment response evaluation. Although conventional echocardiography provides various parameters regarding systolic and diastolic functions, it cannot yield information about myocardial deformation [3]. Recently, with the technological advancements of echocardiographic equipment, the incorporation of novel echocardiographic techniques like myocardial strain imaging and three-dimensional (3D) echocardiography has overcome the limitations of conventional echocardiography, such as operator dependency, inability to assess myocardial deformation, and lack of reproducibility [4-6].
In addition to conventional echocardiography, exercise and pharmacologic stress tests can also be utilized to detect the existence of CAD or asymptomatic HF [7]. It is crucial for clinicians to recognize and manage CVDs in patients with DM, as prompt detection of CVDs can significantly reduce the likelihood of future adverse cardiovascular outcomes. This review article aims to evaluate the role of echocardiography in patients with DM and suspected CVDs. Since the majority of patients with diabetes have type 2 diabetes mellitus (T2DM), this review article primarily applies to T2DM.
DM is frequently accompanied by additional cardiovascular risk factors and nearly doubles the risk of CVDs independent of other risk factors [8,9]. The underlying mechanism of CAD in patients with DM is accelerated atherosclerosis, and the prevalence of DM among patients with established CAD in Western countries exceeds 30% [10]. Additionally, nearly 20% of CAD patients have undiagnosed DM, and about 25% have prediabetes or impaired glucose tolerance [10]. CAD is the leading cause of morbidity and mortality in patients with DM [11]. The risk of cardiovascular mortality is approximately 1.7 times higher in adults with DM than in non-diabetic adults in 2014 [11]. In Korea, CVDs accounted for 20.8% of death in patients with diabetes in 2015 [1].
Although mortality from CVDs remains high in patients with DM, cardiovascular mortality has decreased over time, potentially due to the increased use of pharmacotherapy for cardiovascular risk factors [1,12,13]. Therefore, early detection of CVDs in patients with diabetes can impede disease progression and prevent future adverse cardiovascular events.
Patients with DM and CAD may present with chronic or acute coronary syndromes with typical angina, but some DM patients with CAD may not experience typical chest pain. They may only complain of dyspnea or may be asymptomatic and present with electrocardiographic (ECG) abnormalities and/or elevated cardiac biomarkers [14]. This phenomenon, so called silent myocardial ischemia or infarction, is often due to diabetic neuropathy and loss of pain sensation [15,16].
According to the American College of Cardiology/American Heart Association treatment guidelines for HF [17], HF can be classified into four stages. Stage A, at-risk for HF, includes individuals with cardiovascular risk factors for developing HF such as DM, hypertension, obesity, and metabolic syndrome, but without structural heart disease or HF symptoms. Stage B, pre-HF, means the presence of structural heart disease without HF symptoms. Diabetic patients without HF symptoms can be classified as stage A or B HF, and therapeutic interventions are recommended to prevent progression of HF [17,18]. Stage C and D HF refer to symptomatic and advanced HF, respectively. HF can be classified into three types based on the left ventricular ejection fraction (LVEF): HF with reduced ejection fraction (HFrEF; LVEF <40%), HF with mildly reduced ejection fraction (HFmrEF, LVEF=40% to 49%), and HF with preserved ejection fraction (HFpEF; LVEF ≥50%) [18].
DM and HF have a bidirectional relationship, and patients with diabetes have a 2-to 5-fold increased risk of incident HF [19-21], and in Korea, 67.6% of HF patients have DM [22]. In one clinical trial with antidiabetic medication, HF was present in 23.6% of patients with T2DM [23]. The prevalence of prediabetes or diabetes was 34.4% among patients with symptomatic HF who participated in an HF trial comparing sacubitril/valsartan versus ramipril [24].
In patients with diabetes, HF can be caused by ischemic or nonischemic cardiomyopathies [21]. Myocardial ischemia is one of the possible causes of HF associated with CAD [9]. Diabetic patients with CAD have a 3.3-fold increased risk of developing HF [21].
Diabetic cardiomyopathy (DiaCM) is a form of nonischemic HF in patients with diabetes, and it is defined as structural or functional changes in the myocardium that arise from longstanding DM without any specific cause of ventricular dysfunction, such as uncontrolled hypertension, significant valvular heart disease (VHD), or CAD [25]. In the early stages of DiaCM, left ventricular (LV) hypertrophy, myocardial fibrosis, and abnormal cell signaling can be found without symptoms of HF [26]. The estimated prevalence of LV hypertrophy is approximately 70% in adult patients with DM [27]. Chronic hyperglycemia, insulin resistance, hyperinsulinemia, impaired insulin metabolic signaling, increased oxidative stress, metabolic imbalance, inflammation, inadequate immune response, microvascular dysfunction, activation of renin-angiotensin-aldosterone system, and sympathetic nervous system, and accumulation of advanced glycation end-products can all adversely affect cardiac function, resulting in DiaCM [21,26,28]. Abnormal LV remodeling is associated with longstanding glycemic abnormalities, and the longer duration of abnormal glucose control, the worse the degree of LV hypertrophy and diastolic dysfunction [29]. The prevalence of diastolic dysfunction is about 20% to 60%, depending on the diagnostic criteria and study populations [30,31].
Traditionally, DiaCM has been considered a progressive disease with two successive stages: an early concentric phenotype with diastolic dysfunction, and a late fibrotic, dilated phenotype with systolic and advanced diastolic dysfunction (Fig. 1) [25,32]. Recently, Seferovic and Paulus [33] proposed two distinct subtypes of DiaCM: dilated and restrictive phenotypes. The dilated phenotype is characterized pathologically by apoptosis and necrosis of cardiomyocytes and subsequent replacement fibrosis, and clinically presents as HFrEF. The restrictive phenotype is characterized pathologically by cardiomyocyte hypertrophy and reactive interstitial fibrosis, functionally by increased myocardial stiffness, and clinically by HFpEF.
Echocardiography is a medical imaging technique that uses ultrasound with frequencies greater than 20,000 Hz to examine the structure and function of the heart. The conventional modalities of echocardiography include two-dimensional (2D) echocardiography, M-mode, and Doppler echocardiography (Table 1). Due to its benefits in diagnosis, management, and follow-up, echocardiography has become the most commonly used imaging modality for many CVDs (Fig. 2).
However, conventional echocardiography has limitations. In response, researchers have developed several echocardiographic modalities to overcome these limitations. One such modality is strain echocardiography, which can measure intrinsic myocardial deformation by tracking myocardial speckles generated by ultrasound-myocardium interactions. This technique is known as speckle-tracking echocardiography (STE), which uses optimal 2D echocardiographic images with an adequate frame rate. Analyzing myocardial strain with STE allows us to obtain global and regional myocardial strain values, and some algorithms generate a bull’s eye mapping that shows the values of the LV segments (Fig. 3).
In assessing LV systolic function, left ventricular global longitudinal strain (LVGLS) is the most commonly used parameter. Strain is expressed as a percentage change in myocardial length, showing the myocardial deformation during the cardiac cycle [34]. A negative value indicates systolic shortening, and a higher absolute value indicates better LV systolic function. In one study, normal Koreans were found to have an LVGLS of –20.4%±2.2% (95% confidence interval, –25.4% to –16.7%) [35]. The interobserver variability in the measurement of LVGLS ranges from 5.4% to 8.6%, and the intraobserver variability ranges from 4.9% to 7.3%. These variabilities are superior or at least comparable to those of LVEF measurements (which are 10.1% and 7.9%, respectively). Thus, LVGLS can be used as a good objective marker of LV systolic function and has been used in clinical practice [3]. With the Bull’s eye view, cardiologists can detect segments of decreased strain suggesting obstructive CAD (Fig. 3C).
Stress echocardiography can be performed with exercise and pharmacologic stresses. It can reveal obstructive CAD by demonstrating worsening regional wall motion abnormality (RWMA) with increasing cardiac contractility under stress. Additionally, subclinical HFpEF can be detected with the stress echocardiography by demonstrating increased pulmonary arterial pressure with stress.
Echocardiography plays a crucial role in the diagnosis of CAD and HF. In addition to echocardiographic examination, ECG, other imaging modalities, and stress tests are also used for confirming the diagnosis.
Dyspnea, shortness of breath, is a common and nonspecific symptom that can be caused by diverse causes. Since patients with diabetes are at risk of developing HF and may have dyspnea instead of chest pain in cases with CAD, cardiogenic dyspnea should be included as one of the differential diagnoses (Fig. 4). If a diabetic patient presents acute dyspnea, physicians should rule out acute myocardial infarction (AMI) and acute pulmonary embolism (PE). Patients with AMI might experience sweating, indigestion, nausea, and pain or discomfort in the chest, shoulders, jaw, or back in addition to acute dyspnea. Patients with acute PE can present with acute chest pain, exacerbated by inspiration or cough, as well as unilateral leg swelling.
Chronic dyspnea is one of the most common symptoms encountered in daily clinical practice, and the two most common causes are respiratory and cardiac diseases [36]. The symptoms and signs of respiratory diseases include cough, sputum, and abnormal breathing sounds. Patients with significant CAD or HF can have chronic dyspnea which worsens during exercise. Orthopnea, i.e., dyspnea that worsens as the patient lays down, paroxysmal nocturnal dyspnea, and nocturnal cough can occur in HF patients.
Chest pain is another common symptom, and pressure, heaviness, tightness, squeezing, or burning in the chest are regarded as angina-equivalent symptoms. The proposed diagnostic algorithm is summarized in Fig. 5. History taking is the most important step in the diagnosis of myocardial ischemia. Chest pain produced by myocardial ischemia is characterized by being centrally located, deep-sited, difficult to localize and diffuse (typical chest pain). If the chest pain is aggravated by exercise or emotional stress, and relieved by rest or sublingual nitroglycerin, the symptoms are highly suggestive of CVDs (typical chest pain). Typical chest pain is associated with obstructive CAD. In AMI, the chest pain usually lasts longer than 30 minutes and is not relieved by sublingual nitroglycerin. Pericarditis-related chest pain is worsened by a deep breathing or coughing. The pain of aortic dissection or aortic intramural hematoma is typically severe and occurs suddenly in the back or between the shoulder blades. The pain is frequently described as a tearing or ripping sensation that migrates upward or downward depending on the propagation of the lesion. Chest pain from acute PE occurs suddenly and is similar to pleuritic chest pain, which is common in pericarditis.
Along with history taking and physical examination, a chest radiograph, ECG, and routine blood test can all assist with the diagnosis of CVD. An echocardiographic demonstration of RWMA consistent with the coronary artery territory is a representative finding of CAD. Echocardiography can also detect other causes of chest pain or dyspnea, such as aortic dissection or PE [37,38]. Echocardiography in conjunction with exercise or pharmacologic stress can cause a mismatch in myocardial oxygen demand-supply, which allows for a provocative test in patients with stable effort-related chest pain and normal echocardiographic results at rest. Stress echocardiography is also an important supplemental diagnostic test for the evaluation of myocardial viability prior to coronary revascularization [7].
Since STE can identify myocardial segments with decreased myocardial strain suggestive of CAD, it can aid in the detection of significant CAD and eliminate the subjective view of the observer in the evaluation of RWMA associated with conventional echocardiography [3,5,39]. However, invasive coronary angiography remains the gold standard for the diagnosis of obstructive CAD (Fig. 3).
Similar to the evaluation of other CVDs, a detailed history taking, and physical examination are required. A chest X-ray, ECG, and routine blood tests are necessary to diagnose HF. The echocardiographic examination is a fundamental imaging method for the diagnosis of HF presenting with typical symptoms and elevated levels of brain natriuretic peptide (BNP) or N-terminal pro-brain natriuretic peptide (NT proBNP) [17]. HF guidelines recommend measuring natriuretic peptides as one of the initial diagnostic steps in cases of suspected HF [17,18]. A BNP <35 pg/mL or NT proBNP <125 pg/mL suggests that HF is unlikely, while elevated levels of BNP or NT proBNP support an HF diagnosis [17,18]. The existence of alternative potential cardiac and noncardiac causes aside from HF that trigger elevated concentrations of natriuretic peptides should be noted [40,41]. LVEF assessed by conventional echocardiography is used to classify types of HF (HFrEF, HFmrEF, and HFpEF). Echocardiography can also detect combined VHD, RWMA, and pericardial effusion, as well as provide information about prognosis and treatment response [17,18]. The traditional 2D echocardiographic technique is usually used to calculate LVEF. To supplement the 2D echocardiography, 3D measurements are recommended as they provide more accurate data for quantification of LV volume, despite the limitations of lower temporal resolution and higher dependency on image quality [42,43]. The 3D echocardiographic modality is also useful for the evaluation of right ventricular volume and function [44].
HFrEF and HFmrEF can be identified if the LVEF is less than 50%. However, HFpEF can be diagnosed when the LVEF greater than 50%, echocardiographic parameters of diastolic dysfunction are present, and BNP/NT proBNP level is elevated. HFpEF involves a wide range of proposed mechanisms, including diastolic dysfunction, left atriopathy, endothelial dysfunction, volume overload, and cardiometabolic abnormalities [26]. An elevated E/e’ (>9) and the presence of pulmonary hypertension (estimated pulmonary arterial systolic pressure [PASP] >35 mm Hg) are two parameters of H2FPEF score used to diagnose HFpEF [45]. An E/e’ is the ratio of mitral E velocity and mitral annular e’ velocity in early diastole measured by Doppler echocardiography and is associated with LV filling pressure [46]. Estimated PASP is calculated using the maximal velocity of tricuspid regurgitation (TR Vmax) by the continuous wave Doppler and right atrial pressure. H2FPEF scoring system is useful for diagnosing HFpEF, especially in euvolemic patients [47,48].
It was recently discovered that LVGLS can be used to supplement LVEF, particularly in patients with HFpEF [3,6,49]. Decreased LVGLS is a marker of myocardial dysfunction that indicates subclinical LV dysfunction before clinical symptoms develop [3,50,51]. Furthermore, strain echocardiography can detect early diastolic dysfunction by calculating left atrial strain [52,53]. Strain echocardiography, it has been suggested, can aid in the early detection of myocardial dysfunction in patients with DiaCM [54]. A study by Yang et al. [55] demonstrated that LVGLS was significantly impaired T2DM patients with microvascular complications, more so than in without complications, while no significant difference in LVEF was observed between the two groups. A recent meta-analysis showed that 3D echocardiography-measured LVGLS was reduced in asymptomatic patients with diabetes [56].
Additional imaging modalities such as cardiac magnetic resonance imaging and cardiac computed tomographic angiography can provide information if routine transthoracic echocardiography is unavailable or falls short in providing sufficient information [57-59]. If a patient with dyspnea only on exertion and suspected HFpEF has normal echocardiographic results at rest, exercise stress echocardiography may be warranted to uncover exercise-induced hemodynamic abnormalities such as diastolic dysfunction or pulmonary hypertension [60,61].
We recommend that echocardiography should be performed on patients with diabetes in the following situations. In all cases, a detailed history taking, physical examinations, ECGs, and measurements of BNP or NT proBNP levels also provide helpful information that should guide decisions (Fig. 6).
(1) Unquestionable signs and symptoms of HF, i.e., dyspnea, crackles or decreased breathing sounds on auscultations on bilateral lung fields, pulmonary congestion and/or bilateral pleural effusions on chest radiographs: Echocardiography should be performed to confirm the diagnosis of HF and to rule out other possible causes of the symptoms.
(2) Bilateral edema on lower legs: Clinicians should investigate the causes of edema, such as the patient’s use of calcium channel blockers, non-steroidal anti-inflammatory drugs, and glucoselowering agents such as thiazolidinediones, or the presence of systemic diseases like renal disease. Echocardiography can both assess right heart function and diagnose right-sided HF.
(3) Exertional dyspnea without signs of congestion: Echocardiography can be used to diagnose HF, VHD, or CAD. Strain echocardiography can detect HF in its early stages before clinical symptoms develop. Exercise or pharmacologic stress echocardiography can help determine the cause of dyspnea, such as CAD or pulmonary hypertension, or evaluate functional capacity.
(4) Obvious abnormal findings on ECG or chest X-ray: ECG findings that indicate heart diseases include arrhythmias such as atrial fibrillation, ventricular tachycardia, pathologic Q waves, ST-segment changes, and left bundle branch block. Cardiomegaly, increased pulmonary arterial size, pulmonary edema, and pleural effusions are chest X-ray findings that suggest the presence of CVD. Echocardiography should be performed to determine the presence and severity of CVD, even if the patient is asymptomatic.
(5) Poor functional capacity or inactivity with elevated BNP or NT proBNP levels: Echocardiography should be performed at least once to confirm the presence of HF or another CVD, particularly in patients with a high suspicion of HF.
(6) To check the efficacy of medications: Follow-up echocardiograms comparing parameters pre and post treatment can provide clinical clues as to whether the medications should be maintained or changed. For example, in patients with HFrEF, if LVEF re-measured after a certain period of medical treatment has not sufficiently improved, additional drugs may be required.
(7) To diagnose early-stage HF or check the effect of medications, such as sodium glucose cotransporter 2 inhibitor (SGLT2i) to prevent HF’s progression (usually for research purposes): Strain echocardiography can help detect early-stage HF before overt HF symptoms develop or structural changes occur.
While the prevalence of many CVDs is decreasing in patients with DM, HF is becoming more common. Unfortunately, to date less attention has been paid to HF than CAD as a cardiac complication in patients with diabetes [2,62]. Because asymptomatic patients with diabetes are regarded as having stage A and B HF, timely recognition of HF allows for treatment options. Recently, SGLT2is and glucagon-like peptide-1 receptor agonists (GLP-1RAs) have been in the limelight because these medications have been shown to significantly reduce atherosclerotic CVD, including myocardial infarction, stroke, and cardiovascular death [63]. Strain measurement of the LV can be useful for detecting stage A and B HF before associated HF symptoms develop. Strain measurement of the left atrium is also helpful to find atrial dysfunction in patients with HFpEF [52].
SGLT2is are at the center of interest in the management of HF regardless of the presence of DM [64]. Subsequent studies using SGLT2is have shown consistent results that all SGLT2is significantly reduce the risk of HF in patients with diabetes and are effective in both the secondary and primary prevention of HF [65,66]. Furthermore, SGLT2is effectively reduce the risk of cardiac death and hospitalization for HF, irrespective of the presence of diabetes or the type of HF based on the LVEF [67]. Large-scale studies demonstrating the beneficial effects of SGLT2i on the echocardiographic parameters, including strain measurements, will enable researchers a better understanding of the mechanism by which SGLT2is exert their preventive and therapeutic effects of on HF [68].
Although GLP-1RAs have been found to have a neutral effect on HF hospitalization [63,69], studies have demonstrated that GLP-1RA, alone or in combination with SGLT2i in patients with T2DM can effectively improve cardiac work (as assessed by strain echocardiography) more effectively than insulin treatment [70]. Unlike conventional echocardiography, strain measurement detects subtle changes in cardiac function and can thus be used to compare the effects of treatment.
Patients with diabetes are predisposed to vascular diseases such as CAD, stroke, and HF. DM is classified as stage A and B HF, even in the absence of HF symptoms. If a diabetic patient presents with dyspnea or chest pain, echocardiography should be performed as a part of a diagnostic process that also involves a detailed history taking, physical examinations, an assessment of biomarkers, an ECG, and chest radiographs. Since symptoms in diabetic patients with CVD may be ambiguous or absent, echocardiography and stress tests should also be considered for those who show signs of HF or CAD, even if they are otherwise asymptomatic. In short, echocardiography is an essential diagnostic test for determining the cause of cardiogenic dyspnea or chest pain.
ACKNOWLEDGMENTS
This paper was proofread by the Writing Center at Jeonbuk National University in February 2023.
The authors like to acknowledge Dr. Kyoung Ae Lee for her advice on this work.
REFERENCES
1. Park JH, Ha KH, Kim BY, Lee JH, Kim DJ. Trends in cardiovascular complications and mortality among patients with diabetes in South Korea. Diabetes Metab J. 2021; 45:120–4.


2. Pop-Busui R, Januzzi JL, Bruemmer D, Butalia S, Green JB, Horton WB, et al. Heart failure: an underappreciated complication of diabetes. A consensus report of the American Diabetes Association. Diabetes Care. 2022; 45:1670–90.


3. Park JH. Two-dimensional echocardiographic assessment of myocardial strain: important echocardiographic parameter readily useful in clinical field. Korean Circ J. 2019; 49:908–31.


4. Choi W, Kim CH, Hwang IC, Yoon CH, Choi HM, Yoon YE, et al. Three-dimensional myocardial strain for the prediction of clinical events in patients with ST-segment elevation myocardial infarction. J Cardiovasc Imaging. 2022; 30:185–96.


5. Malagoli A, Fanti D, Albini A, Rossi A, Ribichini FL, Benfari G. Echocardiographic strain imaging in coronary artery disease: the added value of a quantitative approach. Cardiol Clin. 2020; 38:517–26.
6. Marwick TH. Ejection fraction pros and cons: JACC state-of-the-art review. J Am Coll Cardiol. 2018; 72:2360–79.
7. Guerreiro RA, Fazendas P, Pereira AR, Marques A, Pais J, Alegria S, et al. Clinical and echocardiographic characterization of false-positive results from stress echocardiography. J Cardiovasc Imaging. 2020; 28:123–33.


8. Kim HC, Lee H, Lee HH, Seo E, Kim E, Han J, et al. Korea hypertension fact sheet 2021: analysis of nationwide population-based data with special focus on hypertension in women. Clin Hypertens. 2022; 28:1.


9. Al Backr HB, Albacker TB, Elshaer F, Asfina N, AlSubaie FA, Ullah A, et al. Ischemic cardiomyopathy versus non-ischemic cardiomyopathy in diabetic patients: clinical characteristics, management, and long-term outcomes. Am J Cardiovasc Dis. 2022; 12:56–66.
10. Standl E, Khunti K, Hansen TB, Schnell O. The global epidemics of diabetes in the 21st century: current situation and perspectives. Eur J Prev Cardiol. 2019; 26(2 Suppl):7–14.


11. Leon BM, Maddox TM. Diabetes and cardiovascular disease: epidemiology, biological mechanisms, treatment recommendations and future research. World J Diabetes. 2015; 6:1246–58.
12. Rawshani A, Rawshani A, Gudbjornsdottir S. Mortality and cardiovascular disease in type 1 and type 2 diabetes. N Engl J Med. 2017; 377:300–1.


13. Gyldenkerne C, Knudsen JS, Olesen KK, Sorensen HT, Botker HE, Thomsen RW, et al. Nationwide trends in cardiac risk and mortality in patients with incident type 2 diabetes: a Danish cohort study. Diabetes Care. 2021; dc210383.


14. Shimabukuro M, Saito T, Higa T, Nakamura K, Masuzaki H, Sata M, et al. Risk stratification of coronary artery disease in asymptomatic diabetic subjects using multidetector computed tomography. Circ J. 2015; 79:2422–9.


15. Marchant B, Umachandran V, Stevenson R, Kopelman PG, Timmis AD. Silent myocardial ischemia: role of subclinical neuropathy in patients with and without diabetes. J Am Coll Cardiol. 1993; 22:1433–7.


16. Duque A, Mediano MF, De Lorenzo A, Rodrigues LF Jr. Cardiovascular autonomic neuropathy in diabetes: pathophysiology, clinical assessment and implications. World J Diabetes. 2021; 12:855–67.


17. Heidenreich PA, Bozkurt B, Aguilar D, Allen LA, Byun JJ, Colvin MM, et al. 2022 AHA/ACC/HFSA guideline for the management of heart failure: a report of the American College of Cardiology/American Heart Association Joint Committee on Clinical Practice Guidelines. J Am Coll Cardiol. 2022; 79:e263–421.
18. McDonagh TA, Metra M, Adamo M, Gardner RS, Baumbach A, Bohm M, et al. 2021 ESC guidelines for the diagnosis and treatment of acute and chronic heart failure. Eur Heart J. 2021; 42:3599–726.


19. Lee HY. Heart failure and diabetes mellitus: dangerous liaisons. Int J Heart Fail. 2022; 4:163–74.


21. Dunlay SM, Givertz MM, Aguilar D, Allen LA, Chan M, Desai AS, et al. Type 2 diabetes mellitus and heart failure: a scientific statement from the American Heart Association and the Heart Failure Society of America. This statement does not represent an update of the 2017 ACC/AHA/HFSA heart failure guideline update. Circulation. 2019; 140:e294–324.


22. Park JJ, Lee CJ, Park SJ, Choi JO, Choi S, Park SM, et al. Heart failure statistics in Korea, 2020: a report from the Korean Society of Heart Failure. Int J Heart Fail. 2021; 3:224–36.


23. Marso SP, Bain SC, Consoli A, Eliaschewitz FG, Jodar E, Leiter LA, et al. Semaglutide and cardiovascular outcomes in patients with type 2 diabetes. N Engl J Med. 2016; 375:1834–44.


24. McMurray JJ, Packer M, Desai AS, Gong J, Lefkowitz MP, Rizkala AR, et al. Angiotensin-neprilysin inhibition versus enalapril in heart failure. N Engl J Med. 2014; 371:993–1004.


25. Paolillo S, Marsico F, Prastaro M, Renga F, Esposito L, De Martino F, et al. Diabetic cardiomyopathy: definition, diagnosis, and therapeutic implications. Heart Fail Clin. 2019; 15:341–7.
26. Jia G, Hill MA, Sowers JR. Diabetic cardiomyopathy: an update of mechanisms contributing to this clinical entity. Circ Res. 2018; 122:624–38.
27. Dawson A, Morris AD, Struthers AD. The epidemiology of left ventricular hypertrophy in type 2 diabetes mellitus. Diabetologia. 2005; 48:1971–9.


28. Urlic H, Kumric M, Vrdoljak J, Martinovic D, Dujic G, Vilovic M, et al. Role of echocardiography in diabetic cardiomyopathy: from mechanisms to clinical practice. J Cardiovasc Dev Dis. 2023; 10:46.


29. Kishi S, Gidding SS, Reis JP, Colangelo LA, Venkatesh BA, Armstrong AC, et al. Association of insulin resistance and glycemic metabolic abnormalities with LV structure and function in middle age: the CARDIA study. JACC Cardiovasc Imaging. 2017; 10:105–14.
30. Marwick TH, Ritchie R, Shaw JE, Kaye D. Implications of underlying mechanisms for the recognition and management of diabetic cardiomyopathy. J Am Coll Cardiol. 2018; 71:339–51.
31. From AM, Scott CG, Chen HH. The development of heart failure in patients with diabetes mellitus and pre-clinical diastolic dysfunction a population-based study. J Am Coll Cardiol. 2010; 55:300–5.
32. Murtaza G, Virk HU, Khalid M, Lavie CJ, Ventura H, Mukherjee D, et al. Diabetic cardiomyopathy: a comprehensive updated review. Prog Cardiovasc Dis. 2019; 62:315–26.
33. Seferovic PM, Paulus WJ. Clinical diabetic cardiomyopathy: a two-faced disease with restrictive and dilated phenotypes. Eur Heart J. 2015; 36:1718–27.


34. Berti M, Pernigo M, Dinatolo E, Cozza F, Cuccia C. Differentiating typical Tako-tsubo syndrome from extensive anterior STEMI: look behind the anterior wall. Echocardiography. 2022; 39:1299–306.


35. Farsalinos KE, Daraban AM, Unlu S, Thomas JD, Badano LP, Voigt JU. Head-to-head comparison of global longitudinal strain measurements among nine different vendors: the EACVI/ASE inter-vendor comparison study. J Am Soc Echocardiogr. 2015; 28:1171–81.
36. Ferry OR, Huang YC, Masel PJ, Hamilton M, Fong KM, Bowman RV, et al. Diagnostic approach to chronic dyspnoea in adults. J Thorac Dis. 2019; 11(Suppl 17):S2117–28.


37. Gulati M, Levy PD, Mukherjee D, Amsterdam E, Bhatt DL, Birtcher KK, et al. 2021 AHA/ACC/ASE/CHEST/SAEM/SCCT/SCMR guideline for the evaluation and diagnosis of chest pain: a report of the American College of Cardiology/American Heart Association Joint Committee on Clinical Practice Guidelines. Circulation. 2021; 144:e368–454.


38. Oh JK, Park JH. Role of echocardiography in acute pulmonary embolism. Korean J Intern Med. 2023; 38:456–70.


39. Biswas K, Mukherjee A, Nandi S, Khanra D, Sharma RK, Maji S. Utility of global longitudinal strain to detect significant coronary artery disease, its extent and severity in patients with stable ischemic heart disease. Echocardiography. 2020; 37:2000–9.


41. Zois NE, Bartels ED, Hunter I, Kousholt BS, Olsen LH, Goetze JP. Natriuretic peptides in cardiometabolic regulation and disease. Nat Rev Cardiol. 2014; 11:403–12.


42. Lang RM, Badano LP, Mor-Avi V, Afilalo J, Armstrong A, Ernande L, et al. Recommendations for cardiac chamber quantification by echocardiography in adults: an update from the American Society of Echocardiography and the European Association of Cardiovascular Imaging. J Am Soc Echocardiogr. 2015; 28:1–39.


43. Choi HJ. Multi-modality echocardiography for cardiac assessment in fetuses of pregnant women with diabetes mellitus. J Cardiovasc Imaging. 2022; 30:135–7.


44. Hamdy RM, Habib SA, Mohamed LA, Abd Elaziz OH. The usefulness of 4D echocardiographic modality for assessing RV affection in uncontrolled hypertensive patients. J Cardiovasc Imaging. 2022; 30:279–89.


45. Reddy YN, Carter RE, Obokata M, Redfield MM, Borlaug BA. A simple, evidence-based approach to help guide diagnosis of heart failure with preserved ejection fraction. Circulation. 2018; 138:861–70.


46. Nagueh SF, Smiseth OA, Appleton CP, Byrd BF 3rd, Dokainish H, Edvardsen T, et al. Recommendations for the evaluation of left ventricular diastolic function by echocardiography: an update from the American Society of Echocardiography and the European Association of Cardiovascular Imaging. J Am Soc Echocardiogr. 2016; 29:277–314.


47. Sueta D, Yamamoto E, Nishihara T, Tokitsu T, Fujisue K, Oike F, et al. H2FPEF score as a prognostic value in HFpEF patients. Am J Hypertens. 2019; 32:1082–90.


48. Tada A, Nagai T, Omote K, Iwano H, Tsujinaga S, Kamiya K, et al. Performance of the H2FPEF and the HFA-PEFF scores for the diagnosis of heart failure with preserved ejection fraction in Japanese patients: a report from the Japanese multicenter registry. Int J Cardiol. 2021; 342:43–8.


49. Park JJ, Park JB, Park JH, Cho GY. Global longitudinal strain to predict mortality in patients with acute heart failure. J Am Coll Cardiol. 2018; 71:1947–57.
50. Chaudhury A, Wanek A, Ponnalagu D, Singh H, Kohut A. Use of speckle tracking echocardiography to detect induced regional strain changes in the murine myocardium by acoustic radiation force. J Cardiovasc Imaging. 2021; 29:147–57.


51. Wang T, Li L, Huang J, Fan L. Assessment of subclinical left ventricle myocardial dysfunction using global myocardial work in type 2 diabetes mellitus patients with preserved left ventricle ejection fraction. Diabetol Metab Syndr. 2022; 14:17.
52. Sun BJ, Park JH. Echocardiographic measurement of left atrial strain: a key requirement in clinical practice. Circ J. 2021; 86:6–13.
53. Yoon SJ, Park S, Choi EY, Seo HS, Shim CY, Ahn CM, et al. Left atrial velocity vector imaging can assess early diastolic dysfunction in left ventricular hypertrophy and hypertrophic cardiomyopathy. J Cardiovasc Imaging. 2023; 31:41–8.
54. Lorenzo-Almoros A, Tunon J, Orejas M, Cortes M, Egido J, Lorenzo O. Diagnostic approaches for diabetic cardiomyopathy. Cardiovasc Diabetol. 2017; 16:28.
55. Yang QM, Fang JX, Chen XY, Lv H, Kang CS. The systolic and diastolic cardiac function of patients with type 2 diabetes mellitus: an evaluation of left ventricular strain and torsion using conventional and speckle tracking echocardiography. Front Physiol. 2022; 12:726719.
56. Ghoreyshi-Hefzabad SM, Jeyaprakash P, Gupta A, Vo HQ, Pathan F, Negishi K. Three-dimensional global left ventricular myocardial strain reduced in all directions in subclinical diabetic cardiomyopathy: a systematic review and meta-analysis. J Am Heart Assoc. 2021; 10:e020811.
57. Lee LE, Chandrasekar B, Yu P, Ma L. Quantification of myocardial fibrosis using noninvasive T2-mapping magnetic resonance imaging: preclinical models of aging and pressure overload. NMR Biomed. 2022; 35:e4641.


58. Kersten J, Hackenbroch C, Bouly M, Tyl B, Bernhardt P. What is normal for an aging heart?: a prospective CMR cohort study. J Cardiovasc Imaging. 2022; 30:202–11.


59. Hamdy RM, Osama H, Fereig HM. Evaluation of cardiac mechanical dyssynchrony in heart failure patients using current echo-doppler modalities. J Cardiovasc Imaging. 2022; 30:307–19.


60. Lancellotti P, Pellikka PA, Budts W, Chaudhry FA, Donal E, Dulgheru R, et al. The clinical use of stress echocardiography in non-ischaemic heart disease: recommendations from the European Association of Cardiovascular Imaging and the American Society of Echocardiography. Eur Heart J Cardiovasc Imaging. 2016; 17:1191–229.


61. Erdei T, Smiseth OA, Marino P, Fraser AG. A systematic review of diastolic stress tests in heart failure with preserved ejection fraction, with proposals from the EU-FP7 MEDIA study group. Eur J Heart Fail. 2014; 16:1345–61.


62. Ferrini M, Johansson I, Aboyans V. Heart failure and its complications in patients with diabetes: mounting evidence for a growing burden. Eur J Prev Cardiol. 2019; 26(2 Suppl):106–13.


63. Zelniker TA, Wiviott SD, Raz I, Im K, Goodrich EL, Furtado RH, et al. Comparison of the effects of glucagon-like peptide receptor agonists and sodium-glucose cotransporter 2 inhibitors for prevention of major adverse cardiovascular and renal outcomes in type 2 diabetes mellitus. Circulation. 2019; 139:2022–31.


64. Zinman B, Wanner C, Lachin JM, Fitchett D, Bluhmki E, Hantel S, et al. Empagliflozin, cardiovascular outcomes, and mortality in type 2 diabetes. N Engl J Med. 2015; 373:2117–28.


65. Neal B, Perkovic V, Mahaffey KW, de Zeeuw D, Fulcher G, Erondu N, et al. Canagliflozin and cardiovascular and renal events in type 2 diabetes. N Engl J Med. 2017; 377:644–57.


66. Giugliano D, Ceriello A, De Nicola L, Perrone-Filardi P, Cosentino F, Esposito K. Primary versus secondary cardiorenal prevention in type 2 diabetes: which newer anti-hyperglycaemic drug matters? Diabetes Obes Metab. 2020; 22:149–57.


67. Vaduganathan M, Docherty KF, Claggett BL, Jhund PS, de Boer RA, Hernandez AF, et al. SGLT-2 inhibitors in patients with heart failure: a comprehensive meta-analysis of five randomised controlled trials. Lancet. 2022; 400:757–67.


68. Wee CF, Teo YH, Teo YN, Syn NL, See RM, Leong S, et al. Effects of sodium/glucose cotransporter 2 (SGLT2) inhibitors on cardiac imaging parameters: a systematic review and metaanalysis of randomized controlled trials. J Cardiovasc Imaging. 2022; 30:153–68.


69. Seferovic PM, Coats AJ, Ponikowski P, Filippatos G, Huelsmann M, Jhund PS, et al. European Society of Cardiology/Heart Failure Association position paper on the role and safety of new glucose-lowering drugs in patients with heart failure. Eur J Heart Fail. 2020; 22:196–213.


70. Ikonomidis I, Pavlidis G, Thymis J, Birba D, Kalogeris A, Kousathana F, et al. Effects of glucagon-like peptide-1 receptor agonists, sodium-glucose cotransporter-2 inhibitors, and their combination on endothelial glycocalyx, arterial function, and myocardial work index in patients with type 2 diabetes mellitus after 12-month treatment. J Am Heart Assoc. 2020; 9:e015716.


Fig. 1.
There are two phenotypes of diabetic cardiomyopathy: the restrictive phenotype, characterized by concentric left ventricular (LV) hypertrophy and diastolic dysfunction; and the dilated phenotype, characterized by LV dilatation and both systolic and advanced diastolic dysfunction. It is important to note that some patients exhibiting a restrictive phenotype may transition to a dilated phenotype. RA, right atrium; Ao, aorta; LA, left atrium; RV, right ventricle; LVEDVi, indexed left ventricular end-diastolic volume; LVEF, left ventricular ejection fraction; HFpEF, heart failure with preserved ejection fraction; SGLT2i, sodium glucose cotransporter 2 inhibitor; HFrEF, heart failure with reduced ejection fraction; HFmrEF, heart failure with mildly reduced ejection fraction. aAngiotensin receptor-neprilysin inhibitor/angiotensin converting enzyme inhibitors/angiotensin receptor blockers, β-blockers, mineralocorticoid receptor antagonists, and ivabradine.
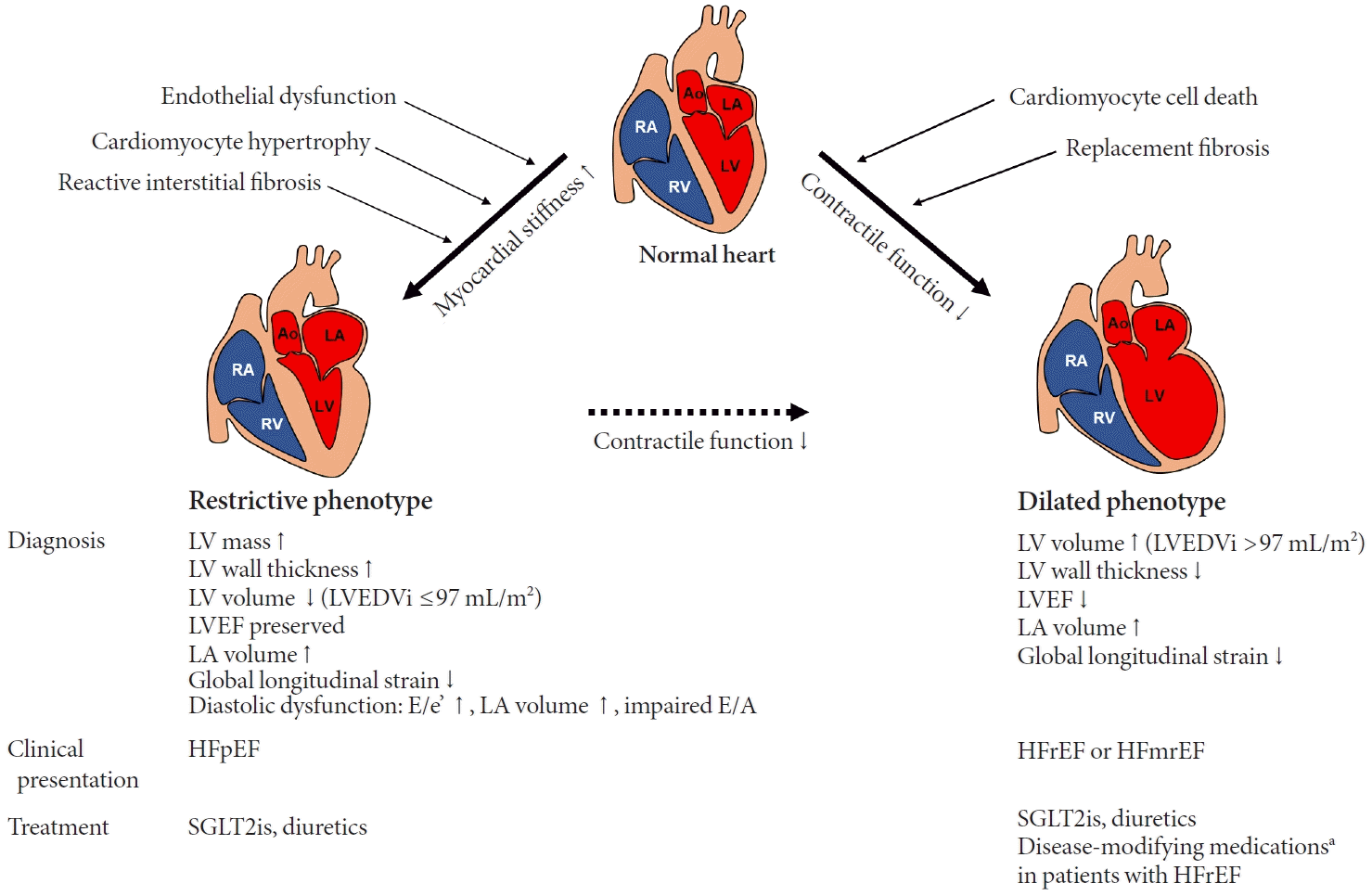
Fig. 2.
The use of conventional echocardiography for the assessment of cardiac function and structure. (A) Assessment of left ventricular (LV) geometry using two-dimensional (2D) and M-mode echocardiography, (B) assessment of LV systolic function using 2D echocardiography, (C) assessment of LV diastolic function using Doppler echocardiography and tissue Doppler imaging, (D) assessment of valvular function using 2D echocardiography and color Doppler imaging, (E) assessment of RV size and systolic function using 2D and M-mode echocardiography, and (F) assessment of pulmonary artery pressure using 2D and Doppler echocardiography. Ao, aorta; LA, left atrium; LVEF, left ventricular ejection fraction; TDI, tissue Doppler imaging; AV, aortic valve; MV, mitral valve; VHD, valvular heart disease; RV, right ventricle; RVFAC, right ventricular fractional area change; TAPSE, tricuspid annular plane systolic excursion; IVC, inferior vena cava; RA, right atrium; TR Vmax, maximal velocity of tricuspid regurgitation.
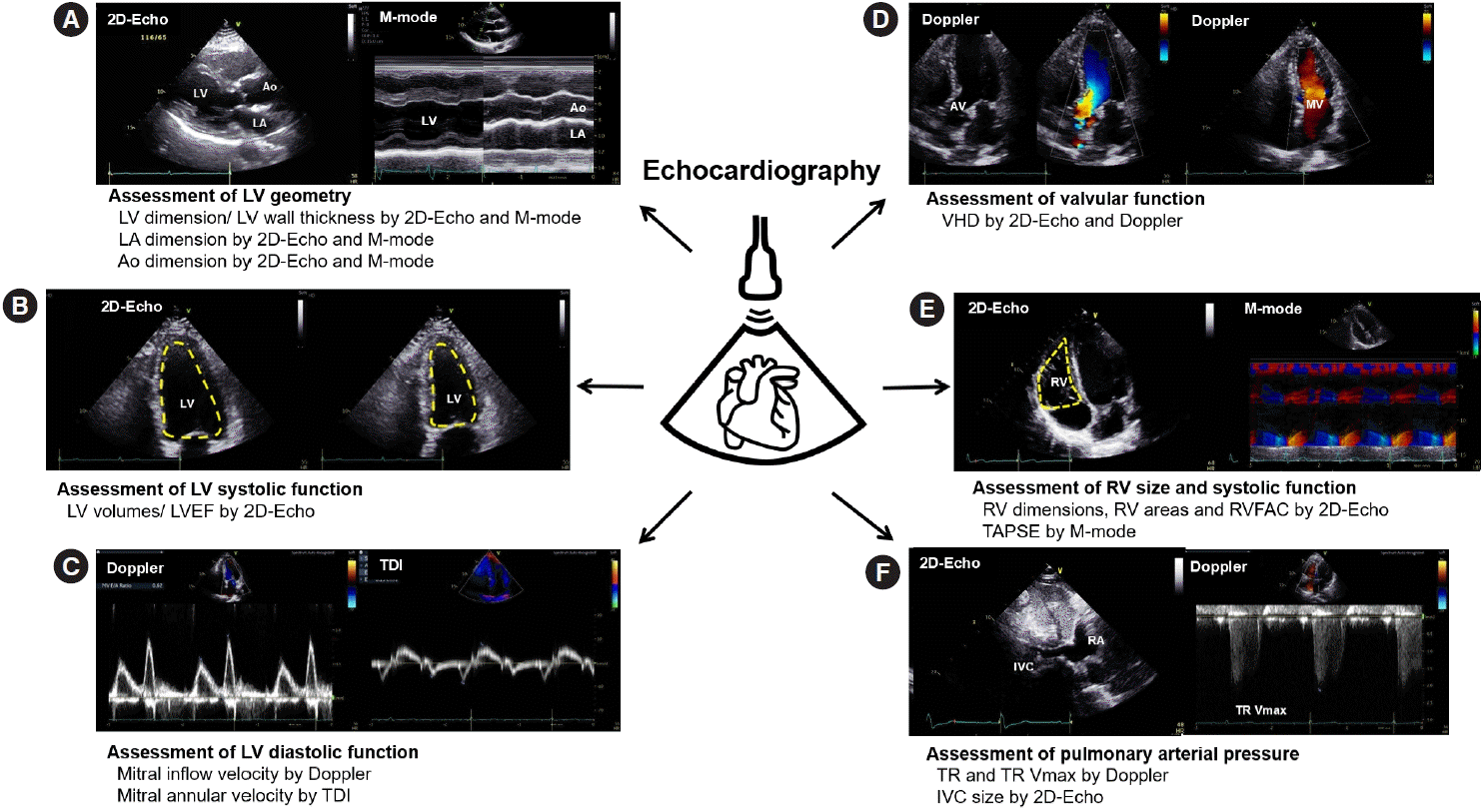
Fig. 3.
The demonstration of Bull’s eye maps using strain echocardiography. (A) A normal subject without cardiovascular risk factors and normal coronary angiography shows a normal Bull’s eye pattern with preserved left ventricular global longitudinal strain (LVGLS) value (–26.5%). (B) A patient with diabetes complaining of dyspnea can show a globally decreased LVGLS (–15.6%) in the Bull’s eye map. There was no significant obstructive coronary artery disease (CAD), as seen in the panel below. (C) In a patient with diabetes and obstructive CAD at the left anterior descending coronary artery (arrowheads), decreased regional strain values can be observed in the Bull’s eye map (arrows). A2C, apical 2-chamber; A3C, apical 3-chamber; A4C, apical 4-chamber; GLS, global longitudinal strain; RCA, right coronary artery; LCA, left coronary artery.
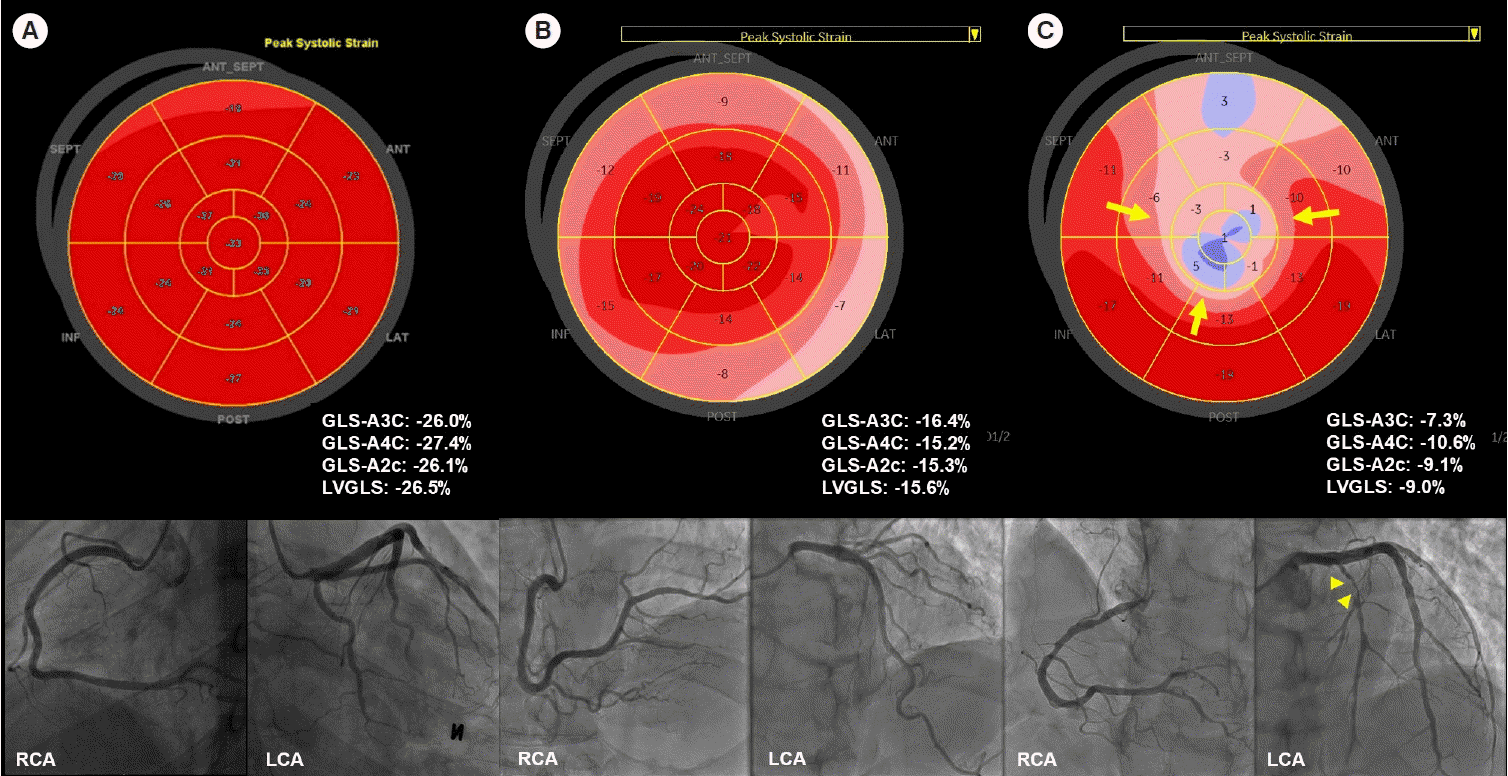
Fig. 4.
A diagnostic algorithm for the evaluation of dyspnea. ECG, electrocardiography; CVD, cardiovascular disease; COPD, chronic obstructive pulmonary disease; LVH, left ventricular hypertrophy; LAE, left atrial enlargement; LBBB, left bundle branch block; BNP, B type natriuretic peptide; NT proBNP, N-terminal pro-brain natriuretic peptide; CECT, contrast enhanced computed tomography; CMR, cardiac magnetic resonance imaging.
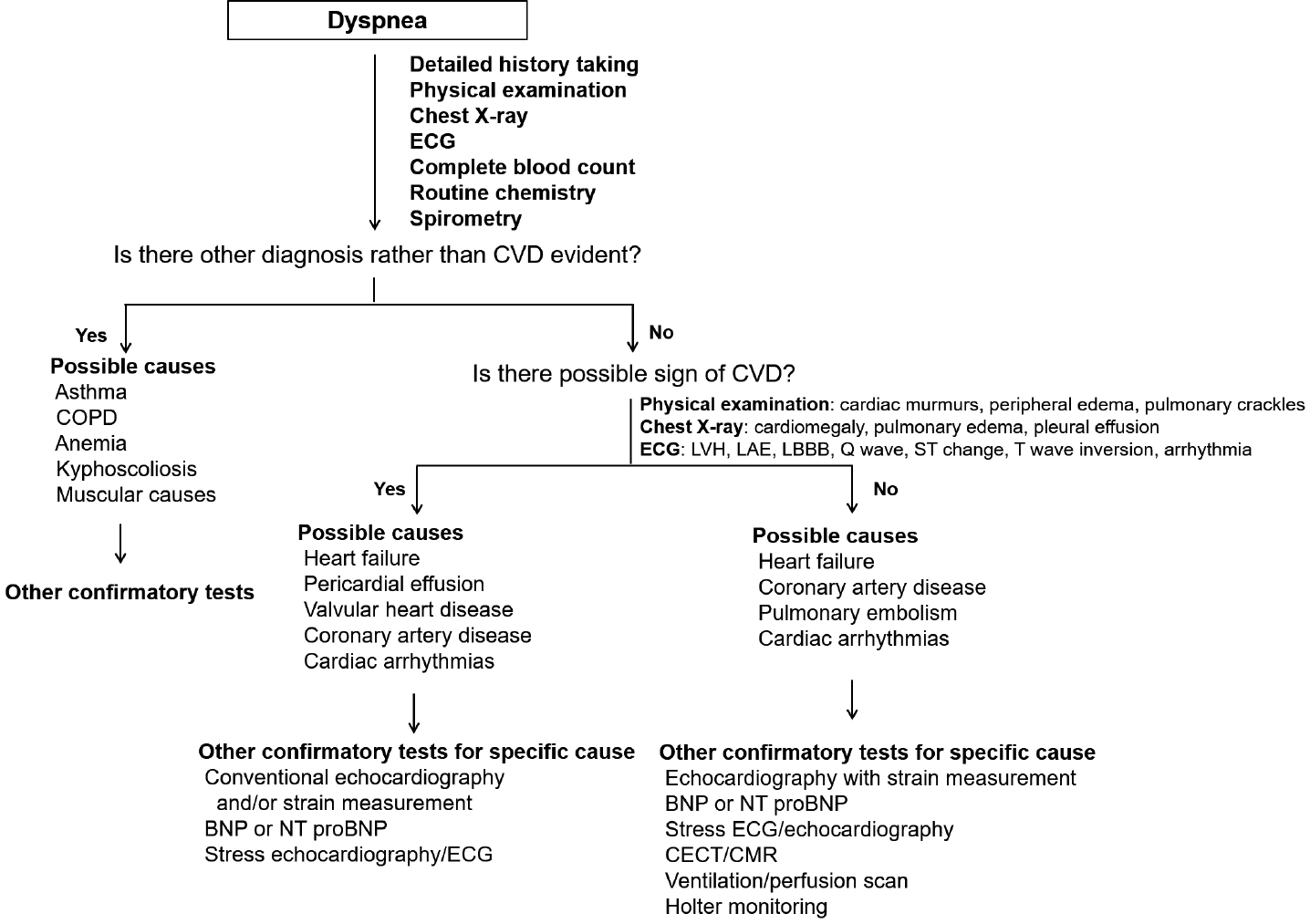
Fig. 5.
A diagnostic algorithm for the evaluation of chest pain. ECG, electrocardiography; CVD, cardiovascular disease; LVH, left ventricular hypertrophy; LAE, left atrial enlargement; LBBB, left bundle branch block; CECT, contrast enhanced computed tomography; CMR, cardiac magnetic resonance imaging.
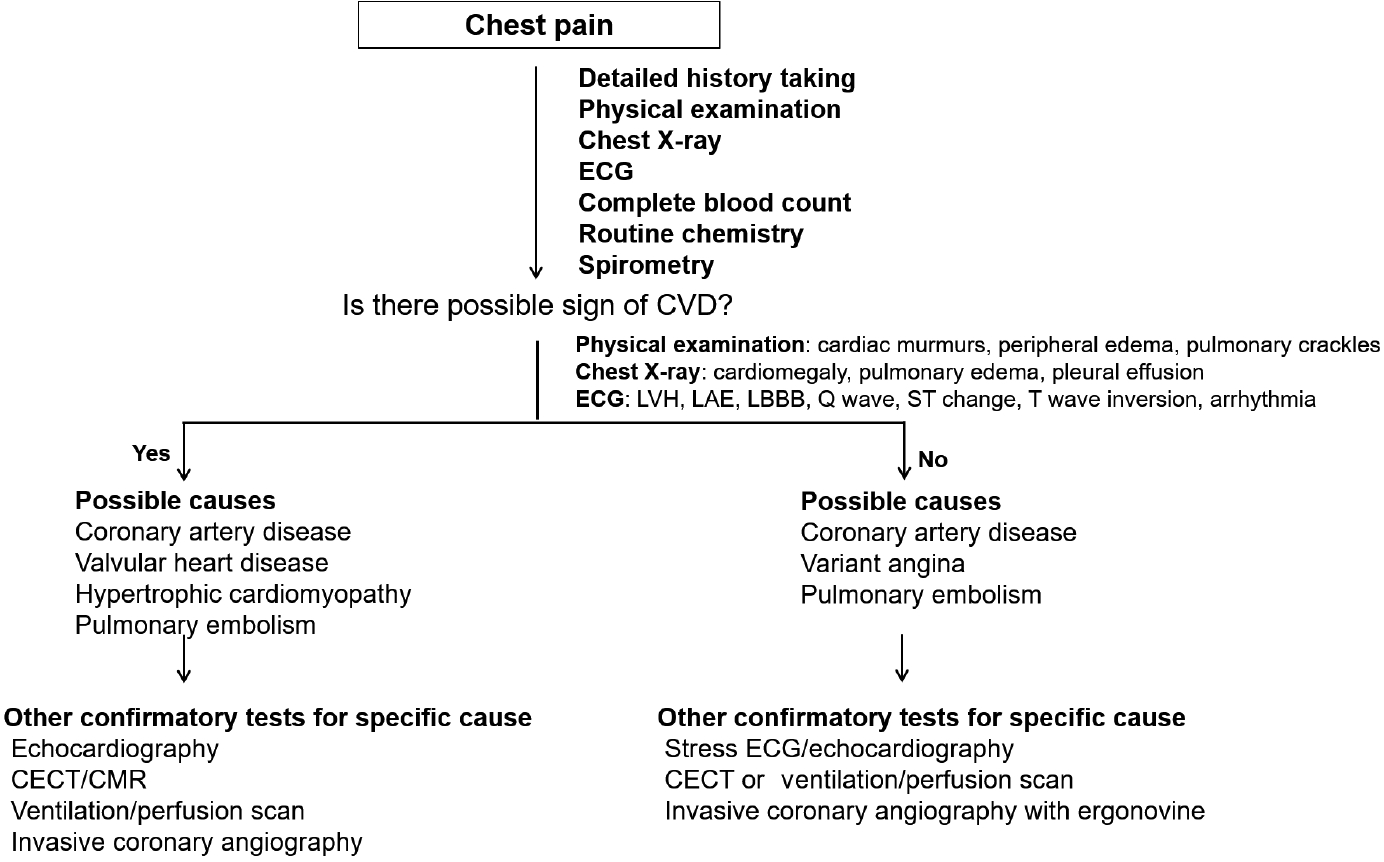
Fig. 6.
The role of echocardiography in evaluating cardiovascular diseases in patients with diabetes mellitus. PEx, physical examination; CXR, chest X-ray; ECG, electrocardiography; CBC, complete blood count; CVD, cardiovascular disease; BNP, brain natriuretic peptide; NT proBNP, N-terminal pro-brain natriuretic peptide; CAD, coronary artery disease; DiaCM, diabetic cardiomyopathy; HFpEF, heart failure with preserved ejection fraction.
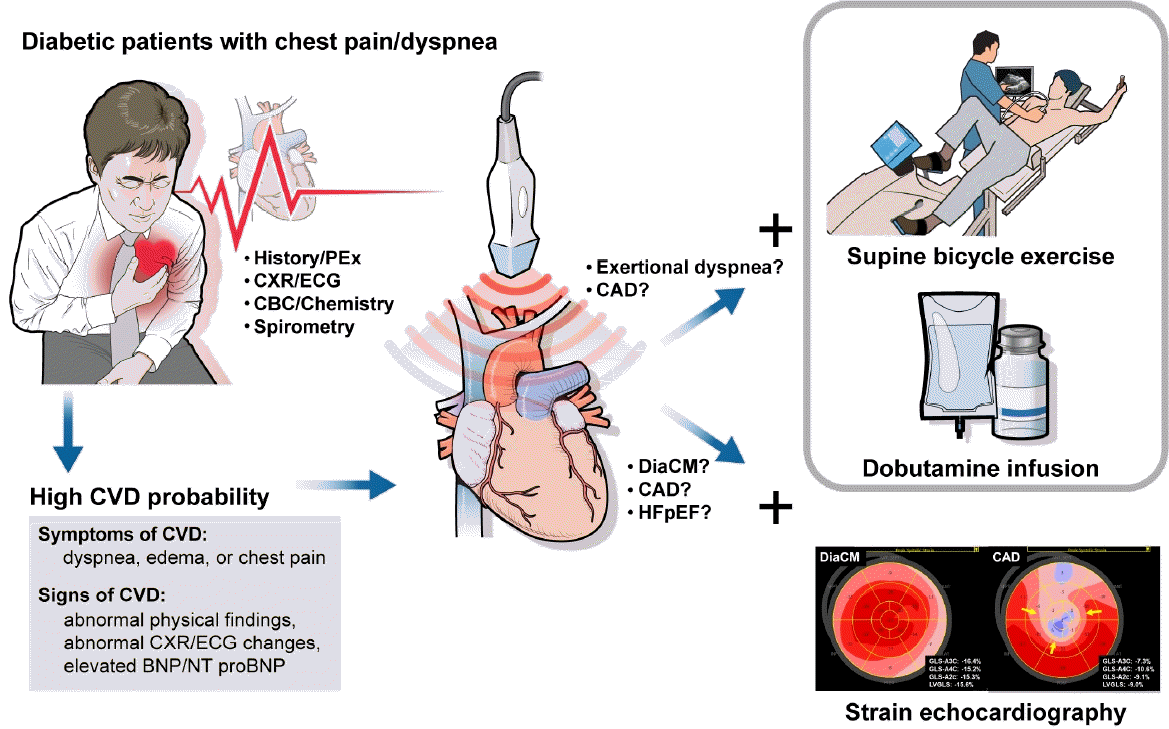
Table 1.
The characteristics of echocardiographic modalities used for evaluating cardiovascular diseases
LV, left ventricle; LVEF, left ventricular ejection fraction; LA, left atrium; 2D, two-dimensional; HF, heart failure; HFrEF, heart failure with reduced ejection fraction; HFmrEF, heart failure with mildly reduced ejection fraction; HFpEF, heart failure with preserved ejection fraction; VHD, valvular heart disease; TR Vmax, maximal velocity of tricuspid regurgitation; 3D, three-dimensional; TDI, tissue Doppler imaging; LVGLS, left ventricular global longitudinal strain; CAD, coronary artery disease.