Abstract
Objective
The evidence on the accuracy of bite registration using intraoral scanners is sparse. This study aimed to develop a new method for evaluating bite registration accuracy using intraoral scanners.
Methods
Two different types of models were used; 10 stone models and 10 with acrylic resin teeth. A triangular frame with cylindrical posts at each apex (one anterior and two posteriors) was digitally designed and manufactured using three-dimensional (3D) printing. Such a structure was fitted in the lingual space of each maxillary and mandibular model so that, in occlusion, the posts would contact their opposing counterparts, enforcing a small interocclusal gap between the two arches. This ensured no tooth interference and full contact between opposing posts. Bite registration accuracy was evaluated by measuring the distance between opposing posts, with small values indicating high-accuracy. Three intraoral scanners were used Medit i500, Primescan, and Trios 4. Viewbox software was used to measure the distance between opposing posts and compute roll and pitch.
Results
The average maximum error in interocclusal registration exceeded 50 μm. Roll and pitch orientation errors ranged above 0.1 degrees, implying an additional interocclusal error of around 40 μm or more. The models with acrylic teeth exhibited higher errors.
Conclusions
A method that avoids the need for reference hardware and the imprecision of locating reference points on tooth surfaces, and offers simplicity in the assessment of bite registration with an intraoral scanner, was developed. These results suggest that intraoral scanners may exhibit clinically significant errors in reproducing the interocclusal relationships.
Intraoral scanning (IOS) is increasingly being used in several fields of dentistry, including the fabrication of conventional or implant-supported prostheses, guided implant placement, orthodontic diagnosis and treatment planning, fabrication of orthodontic appliances, and planning of orthognathic surgery cases.1
The accuracy of IOS has been well-established, based on a large number of, mainly, in vitro studies1-6 (here we use the ISO 5725-1 definition for ‘trueness,’ referring to “the closeness of agreement between the arithmetic mean of a large number of test results and the true or accepted reference value” and ‘precision,’ referring to “the closeness of agreement between test results”; ‘accuracy’ is used to refer to both trueness and precision7). Most intraoral scanners provide results comparable to high-accuracy impression materials such as polyether and polyvinyl siloxane when partial arch scanning is considered. Full arch scans seem to incur higher errors,2 raising concerns for implant-supported prostheses, but probably not for orthodontic treatment planning, where the required accuracy is less.5 In vivo studies are fewer and difficult to compare because of their different methodologies.8-11 Trueness has been reported from a low value of approximately 15 μm, for the anterior segment of the maxillary arch,9 to a high of approximately 50 μm or more, for scans extending to the premolar region.8 Errors exceeding 200 μm have also been reported but may be attributable to specific software settings.11
Evidence on the accuracy of bite registration using intraoral scanners is sparse and mainly restricted to in vitro studies. Some studies have used the transillumination method of bite impression materials or the T-scan system,12,13 whereas others have compared various intraoral scanners.14,15 Researchers have examined models with various occlusal schemes and edentulous areas, intentionally raised the bite to change the angle between the buccal surfaces of the upper and lower teeth, or placed solid abutment analogs to serve as stable points for the measurement of interocclusal discrepancies.14-16 Very few in vivo studies have examined bite registration with intraoral scanners by obtaining and comparing two different digital bite records or by comparing conventional polyvinyl siloxane records to digital ones.17,18
Several factors may affect the evaluation of bite-registration accuracy, such as the optical characteristics of scanned objects in in vitro studies and the oral environment in in vivo studies. Digital intraoral scanners use light to record the examined surfaces. The optical properties of the material being scanned determine how light is reflected and refracted. Higher translucency or more reflective materials such as ceramics, zirconia, resin, and metals may result in lower scanning accuracy.19,20 In in vitro studies, the difference in optical properties between the scanned materials, such as dental stone, composite, ceramic, and natural teeth should be considered.21,22 In in vivo studies, saliva can also affect the accuracy of the scanned surface owing to the refraction and reflection of light on saliva itself.23
Orthodontics is particularly interested in studies investigating the use of digital models for orthodontic diagnostic purposes,5 specifically focusing on the objectives set by the American Board of Orthodontics (ABO).24-27 These studies, which used desktop scanners, unanimously conclude that digital models for the ABO system cannot replace conventional models. The major factors that showed statistically significant differences between the digital and conventional methods were occlusal contact, overjet, and alignment. These differences were mostly attributed to incorrect articulation of the digital casts by the scanner, as well as to the difficulty in landmark identification and the software algorithm.24-27
A major difficulty in studies investigating bite registration is establishing the gold standard, i.e., the occlusal contacts that the scanner should detect. This is hampered by the complexity of the occluding tooth surfaces, the multitude of contacts, and the existence of areas that are in close proximity but not touching. This study aimed to develop a method for evaluating the trueness and precision of bite registration using intraoral scanners, potentially avoiding the limitations of the present methods.
The main difficulty in evaluating the accuracy of bite registration lies in the complex morphology of the occluding tooth surfaces, which prevents accurate identification of the contact points. To avoid this problem, we designed two opposing structures with flat contact surfaces and placed them in the lingual space of the articulating models (Figure 1). Their heights were adjusted so that only they would come into contact, enforcing a small interocclusal gap between the teeth of the opposing arches (Figure 2). The surfaces, which are flat with definite contact areas, enable easy and accurate measurement of the distance between them. Because the surfaces are physically touching, this distance should be zero on the scanned models. Any discrepancy, either positive (gap) or negative (interpenetration), would reveal errors in bite registration.
The aforementioned structure comprised two identical triangular frames with cylindrical posts at each apex (one anterior and two posteriors) on either side (Figure 1). Six rectangular slabs were added to one of the frames for accurate alignment between them. The frames were digitally designed and three-dimensional (3D)-printed on a liquid-crystal display (LCD) printer (Phrozen Shuffle; Phrozen Technology Co., Ltd., Hsinchu, Taiwan) using photosensitive liquid resin (Phrozen Standard Resin Gray; Phrozen Technology Co., Ltd.). The printed structure was fitted in the lingual space of each maxillary and mandibular model (Figure 2). Thus, in occlusion, the posts would contact their opposing counterparts, enforcing an interocclusal gap between the two arches. This ensured no tooth interference and full contact between opposing posts, which could be visually confirmed during the scanning procedure. In this way, the usual process of scanning and bite registration could occur, during which the posts were not visible to the scanner and did not influence the procedure.
Two groups of models were used for demonstrating and testing the method. The first group consisted of 10 dental stone casts derived from successfully treated orthodontic cases, and the second group consisted of 10 models with acrylic resin teeth (New Ace, medium size, A3 shade) arranged on pink dental wax bases (Figure 3). The second model group aimed to imitate the optical properties of natural teeth more closely. Moreover, all models in the second category exhibited different orthodontic malocclusion types, such as anterior or posterior open bite, deep bite, crowding, increased overjet, and Class II or III dental occlusion. Each model resembled an actual patient to evaluate the effects of a wide range of malocclusions.
Scanning commenced from the right second molar, following the occlusal surface up to the left second molar, including a portion of the buccal surface. It continued onto the lingual surface of the left second molar to the right second molar, including a portion of the occlusal surface. Finally, structures with posts on the lingual/palatal surfaces of the models were scanned. All the maxillary and mandibular models were scanned in an identical manner. Bite registration was obtained by scanning the buccal surfaces from the first molar to the canine on both sides. During bite registration, the researcher confirmed that all three posts were in contact with their opposing counterparts. Three different intraoral 3D scanners were evaluated: the Trios® 4th generation (Trios 4; 3Shape Dental Systems, Copenhagen, Denmark), the Medit™ i500 (Medit; MEDIT Co., Seoul, Korea), and the Primescan Dentsply Sirona (Primescan; Sirona Dental Systems, Bensheim, Germany). The Trios 4 (3Shape Dental Systems) and Primescan (Sirona Dental Systems) scanners were used for scanning both the dental casts and models with the acrylic resin teeth; however, the Medit (MEDIT Co.) scanner was used to digitize only the stone models because it could not reliably scan the acrylic teeth and wax base. All scans were performed by a single researcher (LK). Intra-examiner reliability was tested by scanning 10 dental casts twice with the Medit intraoral scanner. A second researcher (DJH) scanned 10 dental casts using the Medit intraoral scanner to assess the inter-examiner reliability.
Errors in bite registration could arise from errors during the scanning of the two individual arches, from errors during the scanning of the left and right interocclusal records when the models are in occlusion, and from the alignment of these four virtual objects to each other (two arches and two interocclusal records). Alignment is achieved in the software. Details are proprietary; however, all scanners probably use a version of the iterative closest point algorithm.28 In our attempt to evaluate the error due to bite registration alone, we applied the following procedure to the group of stone models: First, we scanned the upper and lower jaws of each case without performing bite registration. We then duplicated the models of each case using the cloning function of the scanner software and finally obtained a separate occlusal recording for each clone. This procedure was carried out with the Trios 4 and Medit scanners only, as duplicating a model with Primescan software independently of bite registration was not possible. After the scanning procedure was completed, articulated virtual casts were exported in a standard tessellation language (STL) file format.
In our project, the cylindrical posts of the triangular frames were in physical contact with their opposing counterparts during scanning. Therefore, any error in bite registration should be manifested in the contact area of the posts as the distance between the opposing posts on the digital casts. This distance could be positive, which corresponds to a gap between the posts, or negative, which is visualized as penetration (Figure 4).
To evaluate the accuracy of bite registration, the virtual models were loaded into the Viewbox software (dHal Software, Kifissia, Greece), and the distance between opposing posts was measured. Three measurements (one for the anterior and two for the posterior posts) were obtained for each model and scanner. The distances were not measured directly to minimize errors; however, 10 points were digitized on the surface of each post in the lower and upper jaws. The average of these 10 points was calculated using the software, and the vertical distance perpendicular to the contact plane of the three posts between the average points of the opposing posts was computed (Figure 5).
Errors in bite registration could be similar across all three posts, in which case the models would be at the wrong distance to each other but similarly apart over the whole dental arch, or there could be different errors between the three positions, implying errors in the “pitch” or “roll” orientation between the arches (Figure 6). The roll was computed as the angle between the upper and lower posterior frame bars on the frontal plane and pitch as the angle between the upper and lower midsagittal axes of the frames on the sagittal plane. The roll and pitch were computed using trigonometric functions for the measurements of the three posts. We also computed the average of the three post-measurements for each model (AveErr) and the maximum absolute value of the three measurements (MaxErr).
Inter- and intra-examiner agreements were evaluated using Bland–Altman analysis. A one-sample t-test was performed to test for systematic errors in each scanner from correct registration (zero roll, pitch). One-factor analysis of variance (ANOVA) was used to test for differences between the scanners, and Levene’s test, based on the median, was used to evaluate differences in the variance between the scanners. The Bland–Altman analysis was used to evaluate the agreement between the two registrations with the cloned models.
Statistical significance was set at p = 0.05. All tests were performed using IBM SPSS Statistics version 26 (IBM Co., Armonk, NY, USA).
Paired t-tests showed no statistically significant differences between examiners or between the repeated measures of the first examiner, except for the intra-examiner AveErr (Table 1). The 95% limits of agreement (LoA) reached almost 0.7 degrees for pitch, less for roll, and approximately 200 μm for MaxErr. Inter- and intra-examiner errors, as reported here, include errors due to both the examiner and the scanner.
Descriptive statistics for the two groups of models are shown in Table 2, and the univariate plots are shown in Figure 7. None of the roll and pitch variables were, on average, significantly different from zero, except for the pitch of the Trios 4 scanner on the acrylic teeth (one-sample t-test; t = –3.10, p = 0.013). An ANOVA test on the first group (stone models) showed that the Medit scanner had significantly higher AveErr (F = 11.03, p < 0.001) and MaxErr (F = 7.91, p = 0.002) values than the other two scanners, with significantly higher variance in MaxErr (Levene’s test; F = 8.25, p = 0.002). A comparison between the two groups of models (stone vs. acrylic) showed that the roll had a significantly higher variance in the second group (Levene’s test; Trios 4: F = 4.52, p = 0.048; Primescan: F = 4.38, p = 0.051). The same was true for MaxErr, albeit only marginally so for the Trios 4 scanner (Levene’s test; Trios 4: F = 4.15, p = 0.057, Primescan: F = 4.76, p = 0.043). Overall, the range of errors (MaxErr and AveErr) was higher for the acrylic teeth for both Primescan and Trios 4. The maximum MaxErr for the acrylic models reached 220 μm, in contrast to the stone models, where the maximum MaxErr was 97 μm. The AveErr ranged between –94 μm and 147 μm for acrylic teeth with Primescan, whereas the corresponding range for stone models was from –21 μm to 52 μm.
This study aimed to develop a method for testing the accuracy of bite registration using intraoral scanners. We aimed to develop a method suitable for orthodontics, i.e. using complete dental scans and dual bite registrations (left and right sides), which would not require additional hardware, such as a high-accuracy reference scanner or a coordinate measuring machine (CMM), to avoid the imprecision of locating reference points on tooth surfaces and could measure the error in the relative position and orientation of the two dental models, instead of being limited to a color-coded error map.
The simplicity of the method lies in the design of the triangular structures. These do not affect the bite registration scans in any way because they are hidden from the scanner in the interior model space. They enforce a small interocclusal gap between the two arches, which can be adjusted as desired, ensuring that the only surfaces in contact are the three cylindrical posts. The posts’ contact areas can be visually checked during scanning, and they provide flat surfaces at three standard locations to easily measure registration discrepancies. Thus, this method can be used with models of various malocclusions and different materials.
Industrial reference scanners and CMMs are not required to avoid the time-consuming steps of locating landmarks and obtaining reference measurements. The only additional requirements are the triangular structures, which can be easily constructed via 3D printing, and software to measure the distances between two surfaces. For 3D printing, we used a resin LCD printer, which ensured a flat-top surface, and a gray resin material, which was easily captured by the scanners. To increase the accuracy of measuring the inter-post gap distance, we used software configured to calculate the average of several landmarks on each post surface and then computed the distance between two averages (upper and lower posts) in the direction perpendicular to the plane of the surfaces (Figure 5). Because the location of the posts is standard among all models, it is easy to compute the roll and pitch from measurements using basic trigonometric functions.
In addition to errors in the average gap between the models, expressed here as AveErr, our results revealed bite registration errors in pitch and roll orientation, implying that the models were not aligned over the entire dental arch. Every 0.1 degree of roll will result in 38 μm of interocclusal error at the posterior teeth assuming an average adult mandibular arch of approximately 44 mm intermolar distance.29 This compounds the overall error (AveErr), positively or negatively, on either side of the dental arch. Similarly, pitch affects the registration of the overbite at the anterior part.
A crucial part of this investigation was to isolate the error derived from the bite registration per se from the errors in scanning the models. To this end, we cloned the maxillary and mandibular models and obtained two different bite recordings for the replicated models, similar to Park et al.15; however, only for two of the scanners, as the Primescan software would not allow independent bite recordings. If the bite registration error was minimal, the discrepancy between the two bite recordings in the cloned models approached zero. As expected, the 95% LoA were smaller than the LoA of the intra-observer repeats, where the entire procedure was repeated (see Tables 1 and 3). However, significant discrepancies were observed. The LoA of the cloned models were approximately 40%, 65%, 82%, and 53% of the intra-examiner LoA, for roll, pitch, AveErr, and MaxErr, respectively. Therefore, more than half of the total error could be attributed to the bite registration procedure.
Previous approaches to evaluating bite registration have varied. Iwaki et al.30 placed reference markers on buccal tooth surfaces and measured the distance between the maxillary and mandibular markers. Whether the presence of such markers affects bite registration remains unclear, as they were visible and registered by the scanner. Furthermore, a high-accuracy CMM is required to establish the reference position to assess the trueness. Wong et al.16 employed a similar method, including a CMM as the gold standard; however, the markers were larger and positioned high in the apical area of the models, presumably to avoid including them in bite registration scans, although this was not clearly stated. As in the previous study, the markers were made of metal and appeared highly reflective, making them difficult to image using a scanner. Edher et al.12 used the transillumination method to evaluate the contact areas, limiting the results to color-coded distance maps. Unilateral bite registration, contrary to common clinical practice in orthodontics, was employed by Park et al.,15 in addition to a high-accuracy industrial scanner, to test trueness. Gintaute et al.14 used a local superimposition method on tooth surfaces to accurately locate corresponding points; therefore, avoiding errors in point identification; however, they did not extend their method to assess trueness.
To demonstrate the proposed method, we used two different types of models and three scanners. The models with acrylic teeth showed higher errors; however, whether this was due to the tooth material or wax bases remains unclear. Another causative factor may be that the stone models were derived from successfully treated orthodontic cases, whereas models with acrylic resin teeth represent different types of orthodontic malocclusion. Differences between scanners probably arise because of different 3D acquisition technologies. The Medit scanner showed a bias towards positive interocclusal distances; all scanners had maximum errors that equaled or exceeded 50 μm on average at the posts, suggesting even larger values for the teeth. Wong et al.16 measured interocclusal distortion values from 26.3 to –184.9 μm. In the study of Park et al.,15 the deviations exhibited great variation, and some exceeded 400 μm. Patients can perceive an interference of 20 μm between their natural teeth and 64 μm when two implants oppose each other.31,32 Studies using conventional materials for bite registration have considered contact areas with a thickness of 50 μm or smaller33,34 and sites in close proximity to areas where the material of the interocclusal record had a thickness of less than 100 μm.12,14 Several of our measurements were above these thresholds, and some distances reached extreme values above 200 μm. Extreme values may cause significant problems in clinical dental practice, particularly in prosthodontics. Research areas in orthodontics, such as the investigation of posttreatment occlusal settling, also require high-accuracy, which current scanning systems do not seem to provide.
As this was an in vitro study, several factors that may affect the scanning accuracy, such as patient movement, saliva control, the extent of mouth opening, and bite force when closing, could not be investigated. Another crucial factor is the optical characteristics of the object being scanned. Although we included a set of models with acrylic teeth, these did not have optical properties identical to those of natural teeth. A comparison of the results between the plaster and acrylic tooth models showed some significant differences; however, further studies using natural materials are needed. An additional limitation of the method is that yaw discrepancies cannot be measured; they are only assessed on virtual models by visual inspection of the posts for any misalignment between them.
Notes
REFERENCES
1. Mangano F, Gandolfi A, Luongo G, Logozzo S. 2017; Intraoral scanners in dentistry: a review of the current literature. BMC Oral Health. 17:149. https://doi.org/10.1186/s12903-017-0442-x. DOI: 10.1186/s12903-017-0442-x. PMID: 29233132. PMCID: PMC5727697. PMID: 7b629d06d1744e679aa8123ce1b01b24.


2. Abduo J, Elseyoufi M. 2018; Accuracy of intraoral scanners: a systematic review of influencing factors. Eur J Prosthodont Restor Dent. 26:101–21. https://pubmed.ncbi.nlm.nih.gov/29989757/. DOI: 10.1922/EJPRD_01752Abduo21. PMID: 29989757.
3. Kachhara S, Nallaswamy D, Ganapathy DM, Sivaswamy V, Rajaraman V. 2020; Assessment of intraoral scanning technology for multiple implant impressions- a systematic review and meta-analysis. J Indian Prosthodont Soc. 20:141–52. https://doi.org/10.4103/jips.jips_379_19. DOI: 10.4103/jips.jips_379_19. PMID: 32655218. PMCID: PMC7335030.


4. Goracci C, Franchi L, Vichi A, Ferrari M. 2016; Accuracy, reliability, and efficiency of intraoral scanners for full-arch impressions: a systematic review of the clinical evidence. Eur J Orthod. 38:422–8. https://doi.org/10.1093/ejo/cjv077. DOI: 10.1093/ejo/cjv077. PMID: 26487391.


5. Rossini G, Parrini S, Castroflorio T, Deregibus A, Debernardi CL. 2016; Diagnostic accuracy and measurement sensitivity of digital models for orthodontic purposes: a systematic review. Am J Orthod Dentofacial Orthop. 149:161–70. https://doi.org/10.1016/j.ajodo.2015.06.029. DOI: 10.1016/j.ajodo.2015.06.029. PMID: 26827972.


6. Ahlholm P, Sipilä K, Vallittu P, Jakonen M, Kotiranta U. 2018; Digital versus conventional impressions in fixed prosthodontics: a review. J Prosthodont. 27:35–41. https://doi.org/10.1111/jopr.12527. DOI: 10.1111/jopr.12527. PMID: 27483210.


7. International Organization for Standardization (ISO) 5725-1:1994. 1994. Accuracy (trueness and precision) of measurement methods and results - part 1: general principles and definitions [Internet]. ISO;Geneva: Available from: https://www.iso.org/standard/11833.html. cited 2022 Mar 20.
8. Nedelcu R, Olsson P, Nyström I, Rydén J, Thor A. 2018; Accuracy and precision of 3 intraoral scanners and accuracy of conventional impressions: a novel in vivo analysis method. J Dent. 69:110–8. https://doi.org/10.1016/j.jdent.2017.12.006. DOI: 10.1016/j.jdent.2017.12.006. PMID: 29246490.
9. Winkler J, Gkantidis N. 2020; Trueness and precision of intraoral scanners in the maxillary dental arch: an in vivo analysis. Sci Rep. 10:1172. https://doi.org/10.1038/s41598-020-58075-7. DOI: 10.1038/s41598-020-58075-7. PMID: 31980724. PMCID: PMC6981254.
10. Ender A, Attin T, Mehl A. 2016; In vivo precision of conventional and digital methods of obtaining complete-arch dental impressions. J Prosthet Dent. 115:313–20. https://doi.org/10.1016/j.prosdent.2015.09.011. DOI: 10.1016/j.prosdent.2015.09.011. PMID: 26548890.
11. Kuhr F, Schmidt A, Rehmann P, Wöstmann B. 2016; A new method for assessing the accuracy of full arch impressions in patients. J Dent. 55:68–74. https://doi.org/10.1016/j.jdent.2016.10.002. DOI: 10.1016/j.jdent.2016.10.002. PMID: 27717754.
12. Edher F, Hannam AG, Tobias DL, Wyatt CCL. 2018; The accuracy of virtual interocclusal registration during intraoral scanning. J Prosthet Dent. 120:904–12. https://doi.org/10.1016/j.prosdent.2018.01.024. DOI: 10.1016/j.prosdent.2018.01.024. PMID: 29961618.
13. Ayuso-Montero R, Mariano-Hernandez Y, Khoury-Ribas L, Rovira-Lastra B, Willaert E, Martinez-Gomis J. 2020; Reliability and validity of T-scan and 3D intraoral scanning for measuring the occlusal contact area. J Prosthodont. 29:19–25. https://doi.org/10.1111/jopr.13096. DOI: 10.1111/jopr.13096. PMID: 31270888.
14. Gintaute A, Keeling AJ, Osnes CA, Zitzmann NU, Ferrari M, Joda T. 2020; Precision of maxillo-mandibular registration with intraoral scanners in vitro. J Prosthodont Res. 64:114–9. https://doi.org/10.1016/j.jpor.2019.05.006. DOI: 10.1016/j.jpor.2019.05.006. PMID: 31387847.
15. Park JM, Jeon J, Heo SJ. 2018; Accuracy comparison of buccal bite scans by five intra-oral scanners. J Dent Rehabil Appl Sci. 34:17–31. https://doi.org/10.14368/jdras.2018.34.1.17. DOI: 10.14368/jdras.2018.34.1.17.
16. Wong KY, Esguerra RJ, Chia VAP, Tan YH, Tan KBC. 2018; Three-dimensional accuracy of digital static interocclusal registration by three intraoral scanner systems. J Prosthodont. 27:120–8. https://doi.org/10.1111/jopr.12714. DOI: 10.1111/jopr.12714. PMID: 29160904.
17. Botsford KP, Frazier MC, Ghoneima AAM, Utreja A, Bhamidipalli SS, Stewart KT. 2019; Precision of the virtual occlusal record. Angle Orthod. 89:751–7. https://doi.org/10.2319/092018-684.1. DOI: 10.2319/092018-684.1. PMID: 30920871. PMCID: PMC8111830.
18. Abdulateef S, Edher F, Hannam AG, Tobias DL, Wyatt CCL. 2020; Clinical accuracy and reproducibility of virtual interocclusal records. J Prosthet Dent. 124:667–73. https://doi.org/10.1016/j.prosdent.2019.11.014. DOI: 10.1016/j.prosdent.2019.11.014. PMID: 32014284.
19. Li H, Lyu P, Wang Y, Sun Y. 2017; Influence of object translucency on the scanning accuracy of a powder-free intraoral scanner: a laboratory study. J Prosthet Dent. 117:93–101. https://doi.org/10.1016/j.prosdent.2016.04.008. DOI: 10.1016/j.prosdent.2016.04.008. PMID: 27460324.
20. Song J, Kim M. 2020; Accuracy on scanned images of full arch models with orthodontic brackets by various intraoral scanners in the presence of artificial saliva. Biomed Res Int. 2020:2920804. https://doi.org/10.1155/2020/2920804. DOI: 10.1155/2020/2920804. PMID: 32185200. PMCID: PMC7063212.
21. Ryan EA, Tam LE, McComb D. 2010; Comparative translucency of esthetic composite resin restorative materials. J Can Dent Assoc. 76:a84. https://pubmed.ncbi.nlm.nih.gov/20719098/. PMID: 20719098.
22. Nogueira AD, Della Bona A. 2013; The effect of a coupling medium on color and translucency of CAD-CAM ceramics. J Dent. 41 Suppl 3:e18–23. https://doi.org/10.1016/j.jdent.2013.02.005. DOI: 10.1016/j.jdent.2013.02.005. PMID: 23438417.
23. Kurz M, Attin T, Mehl A. 2015; Influence of material surface on the scanning error of a powder-free 3D measuring system. Clin Oral Investig. 19:2035–43. https://doi.org/10.1007/s00784-015-1440-5. DOI: 10.1007/s00784-015-1440-5. PMID: 25743568.
24. Im J, Cha JY, Lee KJ, Yu HS, Hwang CJ. 2014; Comparison of virtual and manual tooth setups with digital and plaster models in extraction cases. Am J Orthod Dentofacial Orthop. 145:434–42. https://doi.org/10.1016/j.ajodo.2013.12.014. DOI: 10.1016/j.ajodo.2013.12.014. PMID: 24703281.
25. Hildebrand JC, Palomo JM, Palomo L, Sivik M, Hans M. 2008; Evaluation of a software program for applying the American Board of Orthodontics objective grading system to digital casts. Am J Orthod Dentofacial Orthop. 133:283–9. https://doi.org/10.1016/j.ajodo.2006.03.035. DOI: 10.1016/j.ajodo.2006.03.035. PMID: 18249296.
26. Costalos PA, Sarraf K, Cangialosi TJ, Efstratiadis S. 2005; Evaluation of the accuracy of digital model analysis for the American Board of Orthodontics objective grading system for dental casts. Am J Orthod Dentofacial Orthop. 128:624–9. https://doi.org/10.1016/j.ajodo.2004.08.017. DOI: 10.1016/j.ajodo.2004.08.017. PMID: 16286210.
27. Okunami TR, Kusnoto B, BeGole E, Evans CA, Sadowsky C, Fadavi S. 2007; Assessing the American Board of Orthodontics objective grading system: digital vs plaster dental casts. Am J Orthod Dentofacial Orthop. 131:51–6. https://doi.org/10.1016/j.ajodo.2005.04.042. DOI: 10.1016/j.ajodo.2005.04.042. PMID: 17208106.
28. Besl PJ, McKay ND. 1992; A method for registration of 3-D shapes. IEEE Trans Pattern Anal Mach Intell. 14:239–56. https://doi.org/10.1109/34.121791. DOI: 10.1109/34.121791.
29. Rajbhoj AA, Parchake P, Begnoni G, Willems G, Cadenas de Llano-Pérula M. 2021; Dental changes in humans with untreated normal occlusion throughout lifetime: a systematic scoping review. Am J Orthod Dentofacial Orthop. 160:340–62.e3. https://doi.org/10.1016/j.ajodo.2021.02.014. DOI: 10.1016/j.ajodo.2021.02.014. PMID: 34456004.
30. Iwaki Y, Wakabayashi N, Igarashi Y. 2013; Dimensional accuracy of optical bite registration in single and multiple unit restorations. Oper Dent. 38:309–15. https://doi.org/10.2341/12-233-L. DOI: 10.2341/12-233-L. PMID: 23092147.
31. Resnik RR, Misch CE. 2018. Misch's avoiding complications in oral implantology. Mosby;St. Louis: DOI: 10.1016/B978-0-323-37580-1.00017-2.
32. Kim Y, Oh TJ, Misch CE, Wang HL. 2005; Occlusal considerations in implant therapy: clinical guidelines with biomechanical rationale. Clin Oral Implants Res. 16:26–35. https://doi.org/10.1111/j.1600-0501.2004.01067.x. DOI: 10.1111/j.1600-0501.2004.01067.x. PMID: 15642028.
33. Owens S, Buschang PH, Throckmorton GS, Palmer L, English J. 2002; Masticatory performance and areas of occlusal contact and near contact in subjects with normal occlusion and malocclusion. Am J Orthod Dentofacial Orthop. 121:602–9. https://doi.org/10.1067/mod.2002.122829. DOI: 10.1067/mod.2002.122829. PMID: 12080313.
34. Sakaguchi RL, Anderson GC, DeLong R. 1994; Digital imaging of occlusal contacts in the intercuspal position. J Prosthodont. 3:193–7. https://doi.org/10.1111/j.1532-849x.1994.tb00154.x. DOI: 10.1111/j.1532-849X.1994.tb00154.x. PMID: 7866500.
Figure 1
Schematic and three-dimensional renderings of the triangular frames used for aligning and occluding the models; occlusal view and frames in ‘occlusion.’
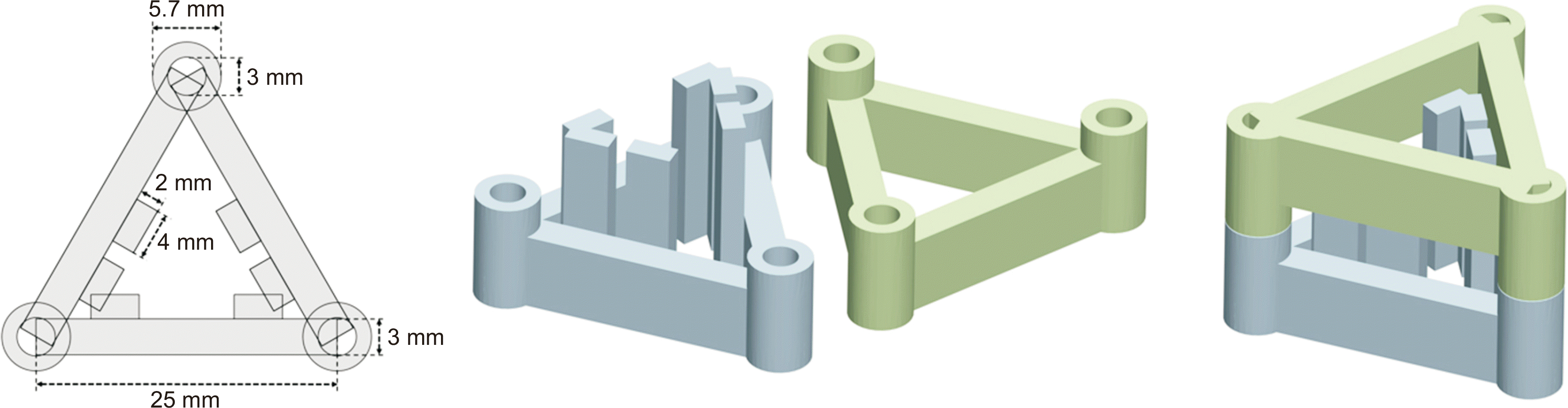
Figure 2
A, Digital models with frames in lingual and palatal space. B, Models in ‘occlusion’ showing interocclusal space.
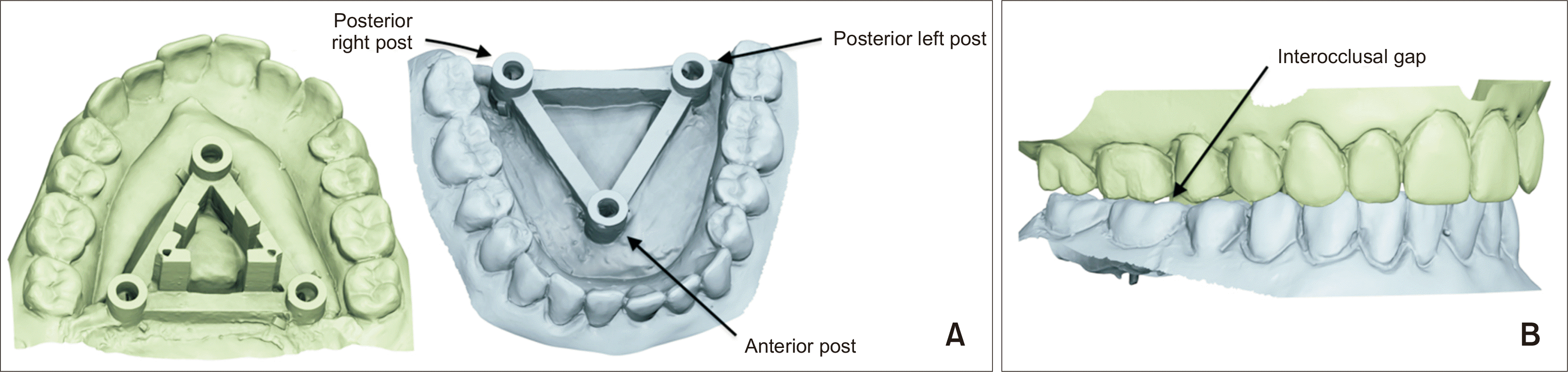
Figure 4
Posterior view of digital models, as aligned by intraoral scanner software. Inset shows space between the upper and lower posts (here approximately 0.2 mm), even though the posts were in full contact during scanning.
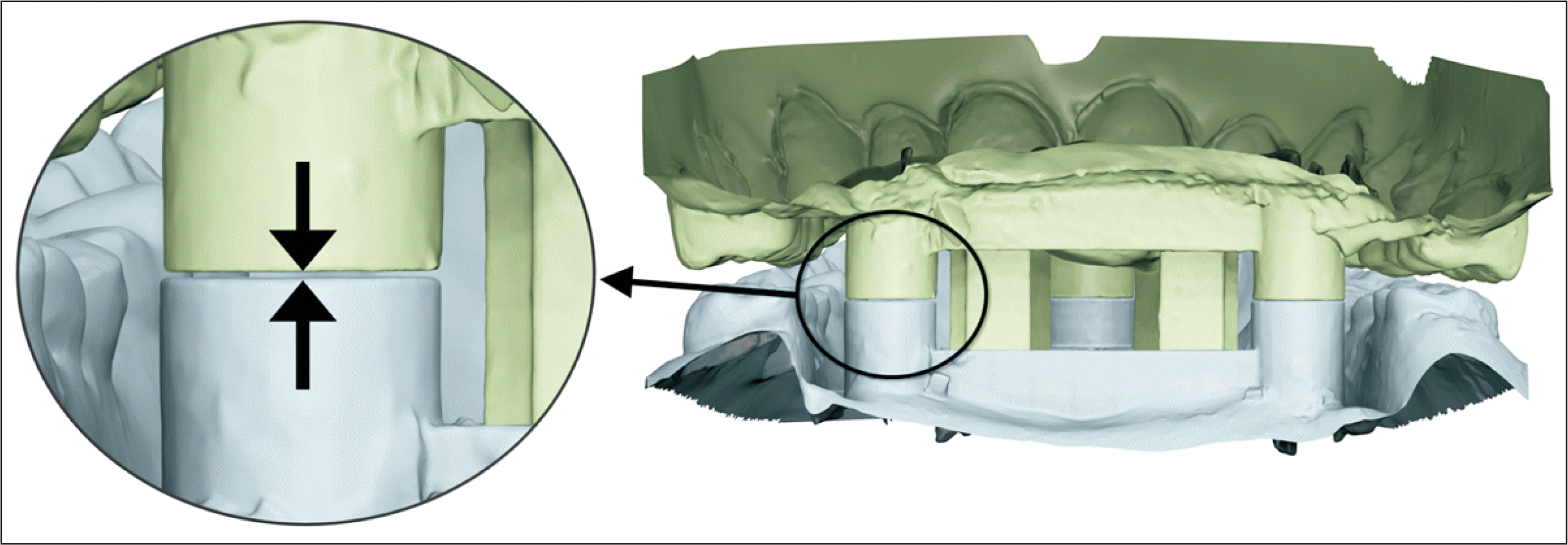
Figure 5
Measuring procedure. Ten points (orange) were digitized on the occluding surface of each post. The calculated average of these points (light and dark purple) was used to measure the inter-post distance, along a direction perpendicular to the plane of the 10 landmarks.
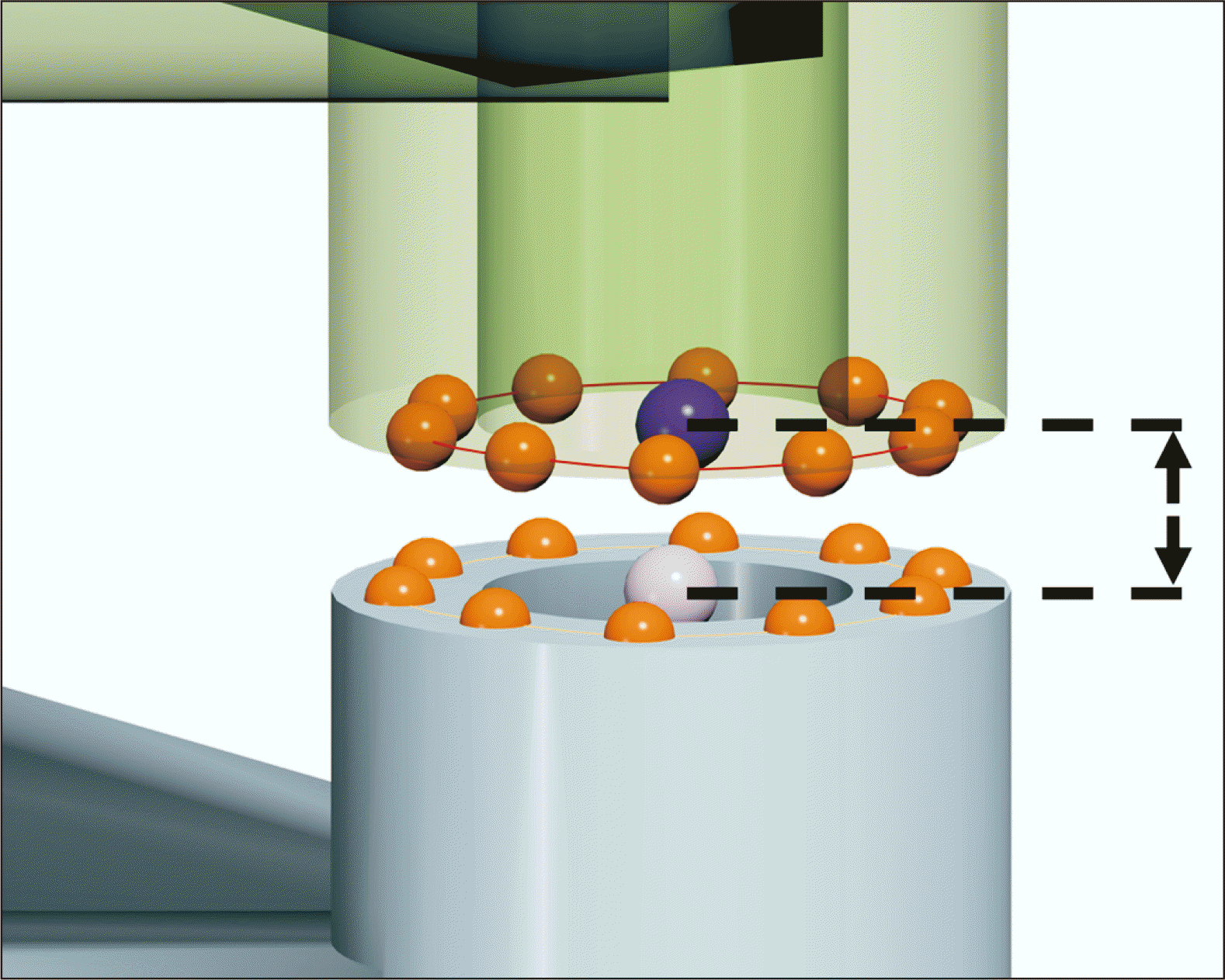
Figure 7
Univariate plots of A, roll, B, pitch, C, AveErr, and D, MaxErr. Group A: stone models; Group B: acrylic teeth on wax bases. Scanning of the acrylic teeth on wax bases (Group B) was not possible with the Medit (MEDIT Co., Seoul, Korea) scanner. AveErr, the average of the three post measurements of each model; MaxErr, the maximum absolute value of the three measurements of each model.
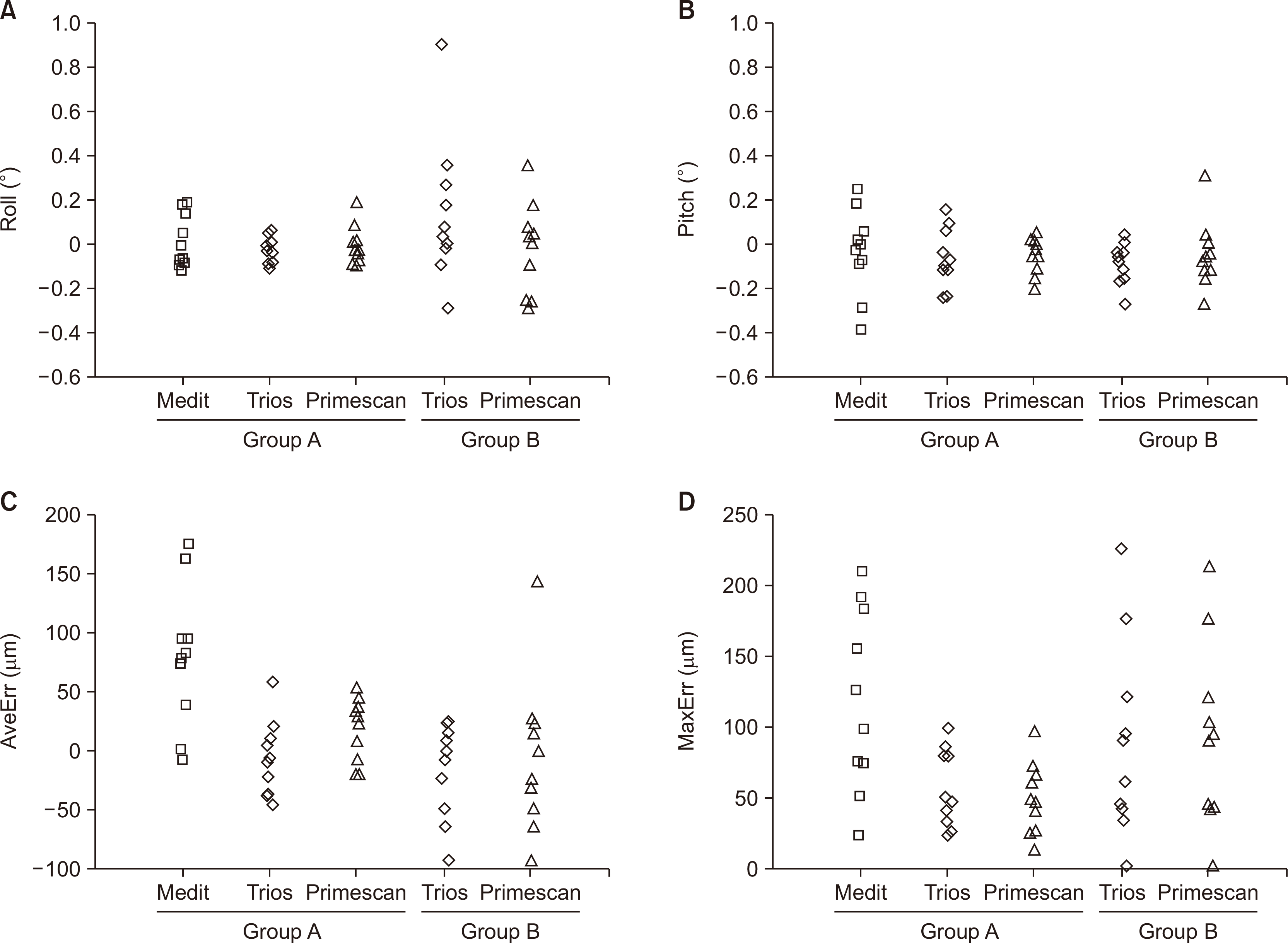
Table 1
Bland–Altman 95% limits of agreement and mean values for inter- and intra-examiner repeated measurements using the Medit (MEDIT Co., Seoul, Korea) scanner on 10 stone models
Error | Roll (degrees) | Pitch (degrees) | AveErr (μm) | MaxErr (μm) |
---|---|---|---|---|
Inter-examiner | ||||
95% LoA | −0.24 to 0.40 | −0.66 to 0.40 | −136.2 to 155.0 | −151.4 to 179.3 |
Mean (p-value) | 0.08 (0.170) | −0.13 (0.161) | 9.4 (0.699) | 13.9 (0.615) |
Intra-examiner | ||||
95% LoA | −0.50 to 0.44 | −0.64 to 0.49 | −71.2 to 156.8 | −176.6 to 199.5 |
Mean (p-value) | −0.03 (0.708) | −0.07 (0.438) | 42.8 (0.045*) | 11.4 (0.715) |
Table 2
Descriptive statistics for roll, pitch, AveErr, and MaxErr