Abstract
Background
Tuberculosis caused by Mycobacterium tuberculosis (MTB) remains a major health problem worldwide. Nontuberculous mycobacteria (NTM) infection is the primary cause of pulmonary disease. Currently, for early diagnosis of mycobacterial infection, direct PCR detection in clinical specimens is used. This study aimed to identify factors affecting the direct PCR detection of mycobacteria from clinical specimens.
Methods
Records of mycobacterial culture from October 2016 to July 2020 were retrospectively reviewed. Using culture as the reference method, the performance of direct PCR detection was calculated. Differences in analytical performances among mycobacteria species, specimen type, and acid-fast bacillus (AFB) staining were determined using chi-squared or Fisher’s exact test.
Results
Of the 27,267 culture datasets, 1,586 datasets were selected. The sensitivity of direct PCR detection for NTM was 27.6% (95% confidence interval [CI], 22.3–33.5) in sputum and 47.8% (95% CI, 37.3–58.5) in bronchial washing fluid (P<0.001). The sensitivity of direct PCR detection showed higher sensitivity in smear-positive AFB than in smear-negative AFB for both MTB (93.8% vs. 51.2%; P<0.001) and NTM (68.3% vs. 26.1%; P<0.001).
초록
배경
결핵균(Mycobacterium tuberculosis, MTB) 감염에 의한 결핵은 전 세계적으로 주요한 건강 문제로 남아있다. 비결핵항산균(nontuberculous mycobacteria, NTM) 감염은 폐 질환의 주요 원인이다. 이러한 마이코박테리아 감염의 조기진단을 위해 현재는 임상 검체에서 직접 PCR 검출 방법을 사용하고 있다. 본 연구에서는 임상 검체에서 마이코박테리아의 직접 PCR 검출에 영향을 미치는 요인을 알아보고자 한다.
방법
2016년 10월부터 2020년 7월까지의 마이코박테리아 배양 결과 데이터를 후향적으로 수집하였다. 배양 결과를 바탕으로 직접 PCR 검출의 성능을 분석했다. 마이코박테리아의 종류, 검체의 유형, 그리고 항산균 염색 간의 분석적 성능 차이는 chi-square 또는 Fisher’s exact test를 사용하여 검증하였다.
Tuberculosis caused by Mycobacterium tuberculosis (MTB) remains a major global health problem. The World Health Organization (WHO) estimates that approximately 10 million people had tuberculosis in 2020 worldwide and global tuberculosis deaths increased from 1.2 to 1.3 million among HIV-negative people between 2019 and 2020 [1]. Mycobacteria other than the MTB complex and Mycobacterium leprae are referred to as nontuberculous mycobacteria (NTM) [2, 3]. NTM infection is a primary cause of pulmonary disease with increased incidence in the Korean population [4-6]. Park et al. [6] reported that the annual incidence of pulmonary disease by NTM increased from 2.9 cases in 2008 to 12.3 cases in 2018 per 100,000 population.
The most effective protection approach for these infectious diseases is early diagnosis and treatment of mycobacterial infection [7]. Rapid and accurate diagnosis of mycobacteria is vital to reduce morbidity and mortality rates and the risk of person-to-person disease transmission [8]. Mycobacterium species can be detected using various methods, such as acid-fast bacillus (AFB) staining, culture, and PCR [9]. Specifically, molecular methods, using PCR-based techniques, can be used to obtain accurate results, and the advent of molecular methods for mycobacterial detection has reduced the time to diagnosis to a few days, whereas the diagnosis using conventional culture systems required several weeks [8, 10]. Recently, various PCR methods have been commonly used with conventional culture and microscopy-based methods [9, 11]. With the development of these technologies, direct detection using molecular genetic testing in clinical specimens, that is, direct PCR detection, is commonly used in laboratories currently. These direct PCR detection methods have also been introduced for the rapid diagnosis of mycobacterial infection.
In this study, the performance of direct PCR detection for mycobacteria was retrospectively reviewed based on the type of clinical specimen, AFB stain results, and type of Mycobacterium species by comparing mycobacterial culture as the reference method.
Records of mycobacterial culture-positive at Samsung Changwon Hospital from October 2016 to July 2020 were retrospectively reviewed. Data were collected by reviewing medical records: times and types of specimen collection for mycobacterial culture and PCR and laboratory data including AFB smear, mycobacterial culture, direct PCR detection, and NTM identification test. Data with the following conditions were excluded from the study: (1) incomplete data and (2) data that is difficult to confirm whether culture and direct PCR were performed using the same sample. This study was approved by the institutional review board of Samsung Changwon Hospital (SCMC 2022-08-007), and the requirement for informed consent was waived. A mycobacterial culture and direct PCR detection flowchart are shown in Figure 1.
A tissue grinder was used for the homogenization of biopsy specimens. When body fluid or abscess volume was ≥10 mL, centrifugation was performed at 3,000×g for 15 minutes. For decontamination, respiratory specimens of the sputum, bronchial washing fluid (BWF), and contaminated specimens (abscesses, gastric aspirates, and urine) were treated with 2% N-acetyl-L-cysteine–sodium hydroxide and centrifugation at 3,000×g was performed for 20 minutes. Non-contaminated samples, such as body fluids (cerebrospinal [CSF], joint, and pleural fluids), blood, bone marrow, and biopsy specimens, were not decontaminated.
For most specimens with or without decontamination, the AFB smear was stained using the auramine-rhodamine fluorescence and confirmed using the Ziehl–Neelsen method based on guidelines provided by the American Thoracic Society [12]. A smear-positive had a grade higher than +1. All specimens were cultured in liquid (MGIT960 system; Becton Dickinson, Sparks, MD, USA) and solid (3% Ogawa agar; Shenyang, Seoul, Korea) media for a maximum of 6 weeks. When a positive signal was shown in the MGIT or colonies were observed on the Ogawa media, AFB stain and nested PCR assay were routinely performed for detection of MTB. Subcultures were performed in Ogawa medium using aliquots from MGIT only if they showed positivity. The nested PCR assay was conducted using an MTB (II) PCR kit (Biosewoom, Seoul, Korea) according to the manufacturer’s instructions. Briefly, a 5 μL aliquot of the PCR product was placed on 1.5% agarose gel. The products were visualized by ultraviolet transillumination and compared to the positive control. The first PCR band was revealed at 256 bp and the second PCR band was revealed at 181 bp. If nested PCR showed equivocal results, a real-time PCR assay was also performed. The equivocal results are as follows: no PCR band, first PCR band visible, or faint PCR band (Fig. 2).
Real-time PCR was conducted using the AdvanSure tuberculosis/nontuberculous mycobacteria (TB/NTM) real-time PCR kit (AdvanSure TB/NTM kit; LG Life Sciences, Seoul, Korea). The real-time PCR allows the identification of clinically important MTB and NTM [8, 13]. Specimens were mixed with 50-μL DNA extraction solution and heated at 100°C for 20 minutes. In the PCR tube including the PCR mixture, 5-μL primer/probe mixture and 5-μL template DNA were mixed and spun down. Assays were analyzed using a SLAN-96P real-time PCR machine (LG Life Sciences, Seoul, Korea). Positive and negative controls were included in each analysis. Positive MTB and NTM results from the AdvanSure TB/NTM kit were determined when the cycle threshold (Ct) value was <35.
Using culture results as the reference method, mycobacterial PCR performances on clinical specimens were calculated in accuracy, sensitivity, specificity, positive predictive value (PPV), and negative predictive value (NPV) with a 95% confidence interval (CI). Differences in analytical performances between MTB and NTM were determined using chi-squared or Fisher’s exact tests. Stata software (version 14.0; Stata Corporation, College Station, TX, USA) and the IBM statistical package for the social sciences (version 20; SPSS Inc., Chicago, IL, USA) were used for analysis. A P value of <0.05 was considered statistically significant.
Among the 27,267 culture datasets collected, 1,586 data (516 tissues, 462 sputa, 263 BWF, 250 abscesses, 28 joint fluid specimens, 22 pleural fluid specimens, 8 CSF, 5 pericardial effusion, 3 urine specimens, and 29 other fluid specimens) of 1,252 patients were selected. Of the 1,586 datasets, 677 (42.7%) were culture-positive and of that, 315 (46.5%) and 362 (53.5%) showed MTB and NTM isolates, respectively.
The proportion of mycobacteria among all positive mycobacterial cultures decreased from 66.0% in 2016 to 38.0% in 2020 for MTB and increased from 34.0% in 2016 to 62.0% in 2020 for NTM (P=0.001, chi-square test for trend). The average annual smear-positive AFB rate was 43.6% and 34.8% for MTB and NTM, respectively (P=0.019). The average annual direct PCR detection rate based on culture was 62.8% and 31.4% for MTB and NTM, respectively (P<0.001).
The direct PCR detection rate based on culture-positive was 64.4% (203/315) and 33.1% (120/362) for MTB and NTM, respectively (Table 1). Of the 1,586 data, 373 (23.5%) discrepancies were observed between detections by culture and direct PCR. Of this data, 348 (93.3%) were mycobacteria in culture alone (Culture-only); 18 (4.8%) were direct PCR alone (PCR-only); 7 (1.9%) showed detection of different mycobacteria between culture and direct PCR (Mycobacteria-mismatch). Among the Culture-only, the smear-positive rate was 6.6% (23/348), which was lower than that when the same mycobacteria were detected by both culture and direct PCR [41.5% (134/323)]. The 18 PCR-only data resulted from DNA extracted from one sputum and 17 non-respiratory specimens (12 tissues, four abscesses, and one pleural fluid). PCR-only case (No. 1058) from sputum had extra-pulmonary tuberculosis and MTB was detected in both culture and direct PCR in pleural fluid and pericardial effusion collected with sputum. Of the seven Mycobacteria-mismatch cases, two (No. 239 and 717) had NTM lung disease with latent tuberculosis; four cases (No. 11, 247, 256, and 695) had pulmonary tuberculosis and NTM grew in only one of two or more cultures. The Mycobacteria-mismatch data are summarized in Supplementary Table S1.
Using culture data as the reference results, the performance of direct PCR detection showed higher sensitivity (64.4% vs. 33.2%), NPV (91.8% vs. 83.4%), and accuracy (92.1% vs. 84.0%) for MTB than for NTM (each, P<0.001) (Table 2). The performance of direct PCR detection for MTB showed no significant differences between the sputum and BWF (65.5% vs. 64.3%). The performance of direct PCR detection for NTM showed higher sensitivity (47.8% vs. 27.6%), NPV (78.1% vs. 52.4%), and accuracy (81.7% vs. 59.7%) in BWF than in sputum (each, P<0.001).
The sensitivity, specificity, PPV, and NPV of direct PCR detection based on AFB staining results are shown in Table 3. The performance of direct PCR detection showed a higher sensitivity for MTB (93.8% vs. 51.2%) and NTM (68.3% vs. 26.1%) with smear-positive than that with smear-negative (each, P<0.001). However, the specificity with smear-positive was lower than that with smear-negative for MTB (95.5% vs. 99.1%; P=0.033) and NTM (97.0% vs. 99.4%; P=0.042).
Pulmonary tuberculosis remains a major global health problem, and the prevalence of NTM lung disease has increased worldwide [1, 14-17]. The annual rate of NTM isolates significantly increased during the study period. The performance of direct PCR detection generally showed lower sensitivity in NTM than in MTB (33.2% vs. 64.4%, Table 2). Compared with previous studies [8, 9, 14, 18], the sensitivity and specificity of direct PCR detection for MTB were similar (median 72.3% [range 63.2–87.5] for sensitivity and median 99.0% [range 97.5–100] for specificity). However, for NTM, medians of 73.9% and 99.4–100% for sensitivity and specificity were reported in a study that included 17 NTM-positive isolates [9], and 23.2% and 99.7% in a study that included 69 NTM-positive isolates, respectively [18]. In the present study, the sensitivity and specificity were 33.2% and 99.1%, respectively, which was a statistical result of 362 NTM-positive isolates; therefore, it is considered more reliable than those of the previous studies.
In the performance evaluation based on specimen types, the difference in performing direct PCR detection between sputum and BWF was identified (Table 2). The sensitivity of direct PCR detection for NTM was higher in BWF than in sputum (47.8% vs. 27.6%; P<0.001). The specificity of the method used for NTM was 100% in sputum and BWF. To the best of our knowledge, previous studies on direct PCR detection for NTM have not reported the differences between sputum and BWF. Conversely, the performance of direct PCR detection for MTB did not show a significant difference between respiratory specimens of sputum and BWF (65.5% vs. 64.3% for sensitivity and 97.9% vs. 99.3% for specificity). Cho et al. [8] reported a sensitivity of 70.9% (95% CI, 67.4–74.2) and a specificity of 98.2% (95% CI, 97.9–98.4).
The performance evaluation of direct PCR detection based on the results of AFB staining showed better sensitivity in smear-positive than in smear-negative (93.8% vs. 51.2% [P<0.001] for MTB; 68.3% vs. 26.1% [P<0.001] for NTM) (Table 3). Several studies reported that the sensitivity had a median of 93.2% (range 54.5–100%) in the smear-positive and a median of 49.5% (range 7.3–100%) in the smear-negative [8, 9, 14, 18]. As previously mentioned, sensitivity for NTM was higher in BWF than in sputum. In 297 cases, excluding discrepancy cases between culture and direct PCR detection, additional analysis was conducted to determine the relationship between the type of respiratory specimen and the results of AFB staining. We observed that the grade of AFB staining in sputum was higher than in BWF (Supplementary Table S2). Therefore, the high sensitivity of NTM in BWF was not related to the grade of AFB staining. Additionally, the area under the receiver operating characteristic curve (AUROC) for direct PCR detection for mycobacteria was analyzed using AFB staining for each specimen type (Table 4). The AUROC for MTB was highest in the smear-positive sputum and lowest in the smear-negative sputum (0.941 vs. 0.718). The AUROC for NTM was highest in the smear-positive BWF and lowest in the smear-negative sputum (0.938 vs. 0.588).
This study had some limitations. First, because this is a retrospective study the data for this study were not obtained from a controlled environment; thus, unexpected environmental variables may have affected the results. However, these unexpected variables may exist in the actual laboratory; therefore, the results of this study will still be considered significant. Second, no medical history such as pulmonary disease or radiologic findings was included in this study. However, given that the statistics are somewhat similar to those of previous reports, results can be considered significant even after excluding this clinical data. Third, to identify a factor associated with direct PCR detection rate between NTM species, further studies are required to analyze the larger dataset.
In summary, we identified factors affecting the direct PCR detection of mycobacteria in a retrospective study. The AFB staining results were related with the direct PCR detection of MTB and NTM, and the type of respiratory specimen was related with the direct PCR detection of NTM.
REFERENCES
1. World Health Organization. Global tuberculosis report 2021. https://apps.who.int/iris/rest/bitstreams/1379788/retrieve. Last accessed on August 2022.
2. Daley CL, Iaccarino JM, Lange C, Cambau E, Wallace RJ, Andrejak C, et al. 2020; Treatment of nontuberculous mycobacterial pulmonary disease: an official ATS/ERS/ESCMID/IDSA clinical practice guideline. Clin Infect Dis. 71:905–13. DOI: 10.1093/cid/ciaa1125. PMID: 32797222. PMCID: PMC7768745.
3. Griffith DE, Aksamit T, Brown-Elliott BA, Catanzaro A, Daley C, Gordin F, et al. 2007; An official ATS/IDSA statement: diagnosis, treatment, and prevention of nontuberculous mycobacterial diseases. Am J Respir Crit Care Med. 175:367–416. DOI: 10.1164/rccm.200604-571ST. PMID: 17277290.
4. Marras TK, Vinnard C, Zhang Q, Hamilton K, Adjemian J, Eagle G, et al. 2018; Relative risk of all-cause mortality in patients with nontuberculous mycobacterial lung disease in a US managed care population. Respir Med. 145:80–8. DOI: 10.1016/j.rmed.2018.10.022. PMID: 30509721. PMCID: PMC6283283.
5. Park YS, Lee CH, Lee SM, Yang SC, Yoo CG, Kim YW, et al. 2010; Rapid increase of non-tuberculous mycobacterial lung diseases at a tertiary referral hospital in South Korea. Int J Tuberc Lung Dis. 14:1069–71.
6. Park JH, Shin S, Kim TS, Park H. 2022; Clinically refined epidemiology of nontuberculous mycobacterial pulmonary disease in South Korea: overestimation when relying only on diagnostic codes. BMC Pulm Med. 22:195. DOI: 10.1186/s12890-022-01993-1. PMID: 35562714. PMCID: PMC9107265.
7. Wang HY, Kim H, Kim S, Kim DK, Cho SN, Lee H. 2015; Performance of a real-time PCR assay for the rapid identification of Mycobacterium species. J Microbiol. 53:38–46. DOI: 10.1007/s12275-015-4495-8. PMID: 25557479.
8. Cho WH, Won EJ, Choi HJ, Kee SJ, Shin JH, Ryang DW, et al. 2015; Comparison of AdvanSure TB/NTM PCR and COBAS TaqMan MTB PCR for detection of Mycobacterium tuberculosis complex in routine clinical practice. Ann Lab Med. 35:356–61. DOI: 10.3343/alm.2015.35.3.356. PMID: 25932446. PMCID: PMC4390706.
9. Kim J, Choi Q, Kim JW, Kim SY, Kim HJ, Park Y, et al. 2020; Comparison of the Genedia MTB/NTM Detection Kit and Anyplex plus MTB/NTM Detection Kit for detection of Mycobacterium tuberculosis complex and nontuberculous mycobacteria in clinical specimens. J Clin Lab Anal. 34:e23021. DOI: 10.1002/jcla.23021.
10. Cheng VC, Yew WW, Yuen KY. 2005; Molecular diagnostics in tuberculosis. Eur J Clin Microbiol Infect Dis. 24:711–20. DOI: 10.1007/s10096-005-0039-1. PMID: 16283213.
11. Drobniewski FA, Caws M, Gibson A, Young D. 2003; Modern laboratory diagnosis of tuberculosis. Lancet Infect Dis. 3:141–7. DOI: 10.1016/S1473-3099(03)00544-9. PMID: 12614730.
12. Diagnostic Standards and Classification of Tuberculosis in Adults and Children. Am J Respir Crit Care Med. 2000; 161:1376–95. DOI: 10.1164/ajrccm.161.4.16141. PMID: 10764337.
13. Kim YJ, Park MY, Kim SY, Cho SA, Hwang SH, Kim HH, et al. 2008; Evaluation of the performances of AdvanSure TB/NTM real time PCR kit for detection of mycobacteria in respiratory specimens. Korean J Lab Med. 28:34–8. DOI: 10.3343/kjlm.2008.28.1.34. PMID: 18309253.
14. Kim JU, Ryu DS, Cha CH, Park SH. 2018; Paradigm for diagnosing mycobacterial disease: direct detection and differentiation of Mycobacterium tuberculosis complex and non-tuberculous mycobacteria in clinical specimens using multiplex real-time PCR. J Clin Pathol. 71:774–80. DOI: 10.1136/jclinpath-2017-204945. PMID: 29559518.
15. Yoo JW, Jo KW, Kim MN, Lee SD, Kim WS, Kim DS, et al. 2012; Increasing trend of isolation of non-tuberculous mycobacteria in a tertiary university hospital in South Korea. Tuberc Respir Dis (Seoul). 72:409–15. DOI: 10.4046/trd.2012.72.5.409. PMID: 23101005. PMCID: PMC3475469.
16. Lee SK, Lee EJ, Kim SK, Chang J, Jeong SH, Kang YA. 2012; Changing epidemiology of nontuberculous mycobacterial lung disease in South Korea. Scand J Infect Dis. 44:733–8. DOI: 10.3109/00365548.2012.681695. PMID: 22720876.
17. Weiss CH, Glassroth J. 2012; Pulmonary disease caused by nontuberculous mycobacteria. Expert Rev Respir Med. 6:597–612. DOI: 10.1586/ers.12.58. PMID: 23234447.
18. Shin S, Yoo IY, Shim HJ, Kang OK, Jhun BW, Koh WJ, et al. 2020; Diagnostic performance of the GENEDIA MTB/NTM Detection Kit for detecting Mycobacterium tuberculosis and nontuberculous mycobacteria with sputum specimens. Ann Lab Med. 40:169–73. DOI: 10.3343/alm.2020.40.2.169. PMID: 31650734. PMCID: PMC6822004.
Fig. 1
A flowchart for the detection of MTB and NTM from cultured and uncultured specimens between October 2016 and July 2020. Decontamination was performed on respiratory specimens.
Abbreviations: AFB, acid-fast bacilli; MTB, Mycobacterium tuberculosis; NTM, nontuberculous mycobacteria.
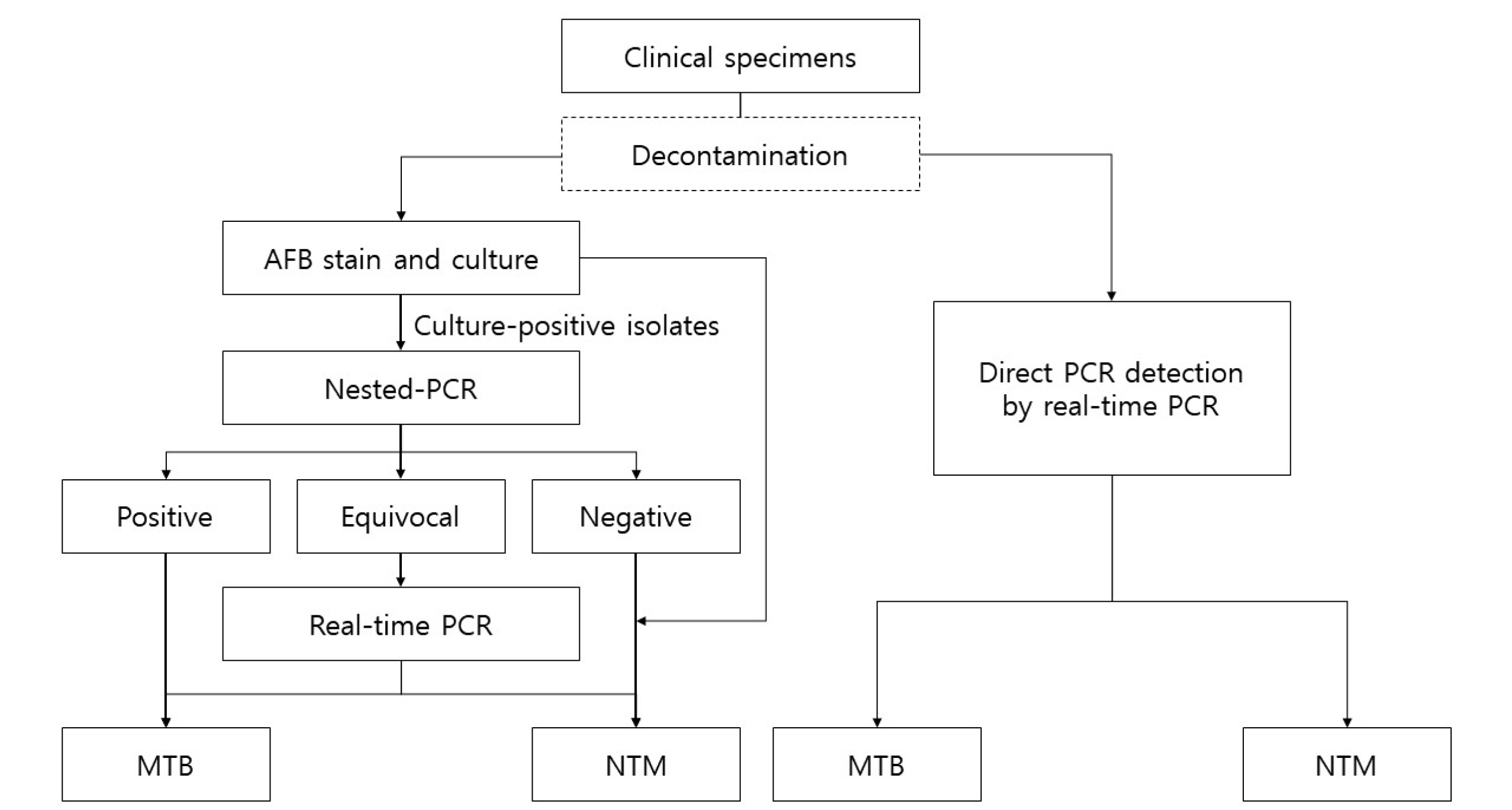
Fig. 2
Examples of 1.5% agarose gel analysis of PCR products generated by conventional PCR. The black arrow indicates the internal control band (483 bp); the white arrow indicates the second PCR band (181 bp). Lanes 1, 8, 9, and 10 showed equivocal results and were identified as NTM, Mixed, and MTB using real-time PCR, respectively.
Abbreviations: MTB, Mycobacterium tuberculosis; NTM, nontuberculous mycobacteria; Mixed, MTB, and NTM.
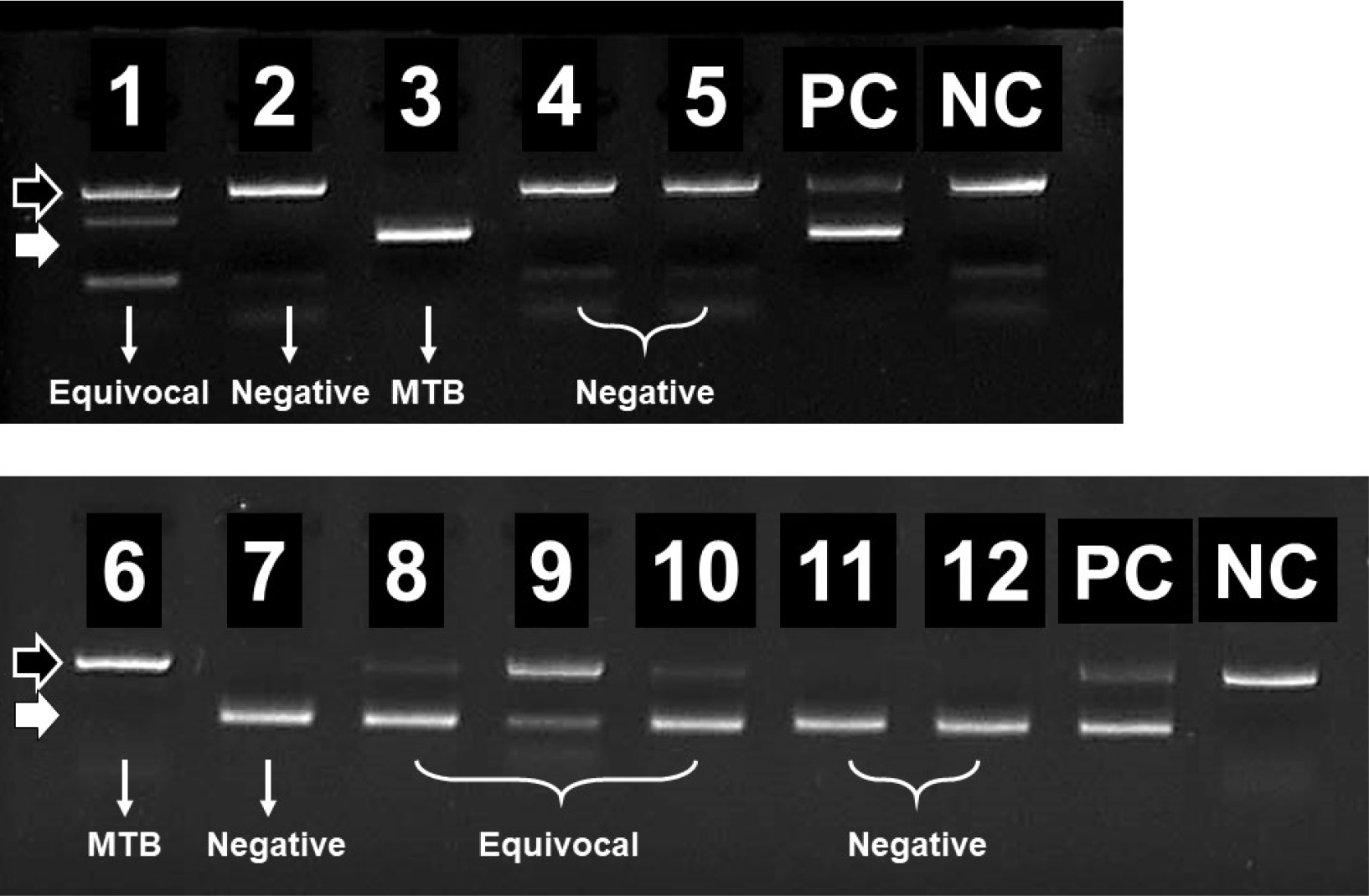
Table 1
Correlations between direct PCR detection and culture from clinical specimens
Methods | Direct PCR detection* | |||
---|---|---|---|---|
|
||||
MTB | NTM | Negative | Total | |
Culture-positive | ||||
MTB | 203† | 1‡ | 112§ | 315 |
AFB stain grading | ||||
0 | 111 | 1 | 106 | 217 |
+1 | 30 | 0 | 4 | 34 |
+2 | 28 | 0 | 1 | 29 |
+3 | 18 | 0 | 0 | 19 |
+4 | 15 | 0 | 1 | 17 |
NTM | 6‡ | 120† | 236§ | 362 |
AFB stain grading | ||||
0 | 3 | 77 | 215 | 295 |
+1 | 3 | 19 | 13 | 35 |
+2 | 0 | 10 | 4 | 14 |
+3 | 0 | 7 | 0 | 7 |
+4 | 0 | 7 | 0 | 7 |
Culture-negative | 8ll | 10ll | 891 | 909 |
AFB stain grading | ||||
0 | 8 | 6 | 887 | 901 |
+1 | 0 | 3 | 0 | 3 |
Total | 217 | 131 | 1,239 | 1,586 |
1,586*Cases in which MTB and NTM were simultaneously detected by real-time PCR were included. †The direct PCR detection rate based on culture-positive was 64.4% (203/315) for MTB and 33.1% (120/362) for NTM. Of the 1,586 data, 373 (23.5%) discrepancies were observed between culture and direct PCR detections, consisting of 7 (1.9%) Mycobacteria-mismatch‡, 348 (93.3%) Culture-only§, and 18 (4.8%) PCR-onlyll cases.
Table 2
Performance of direct PCR detection in different specimen types based on culture
Types of specimens | TP | FP | TN | FN | Sensitivity | Specificity | PPV | NPV | Accuracy | |||||
---|---|---|---|---|---|---|---|---|---|---|---|---|---|---|
|
|
|
|
|
||||||||||
% | 95% CI | % | 95% CI | % | 95% CI | % | 95% CI | % | 95% CI | |||||
Sputum | ||||||||||||||
MTB | 112 | 6 | 285 | 59 | 65.5 | 57.9-72.6 | 97.9 | 95.6-99.2 | 94.9 | 89.4-97.7 | 82.8 | 79.7-85.6 | 85.9 | 82.4-89.0 |
NTM | 71 | 0 | 205 | 186 | 27.6* | 22.3-33.5 | 100 | 98.2-100 | 100 | 100-100 | 52.4* | 50.5-54.3 | 59.7* | 55.1-64.3 |
BWF | ||||||||||||||
MTB | 72 | 1 | 150 | 40 | 64.3 | 54.7-73.1 | 99.3 | 96.4-99.9 | 98.6 | 91.0-99.8 | 78.9 | 74.5-82.8 | 84.4 | 79.5-88.6 |
NTM | 44 | 0 | 171 | 48 | 47.8* | 37.3-58.5 | 100 | 97.9-100 | 100 | 100-100 | 78.1* | 74.6-81.3 | 81.7* | 79.5-86.2 |
Non-respiratory | ||||||||||||||
MTB | 19 | 7 | 822 | 13 | 59.4 | 40.6-76.3 | 99.2 | 98.3-99.7 | 73.1 | 55.2-85.7 | 98.4 | 97.7-99.0 | 97.7 | 96.4-98.6 |
NTM | 5 | 11 | 837 | 8 | 38.5 | 13.9-68.4 | 98.7 | 97.7-99.4 | 31.3 | 15.5-52.9 | 99.1 | 98.6-99.4 | 97.8 | 96.6-98.7 |
Total | ||||||||||||||
MTB | 203 | 14 | 1,257 | 112 | 64.4 | 58.6-69.4 | 98.9 | 98.2-99.4 | 93.6 | 89.5-96.1 | 91.8 | 90.6-92.9 | 92.1 | 90.6-93.3 |
NTM | 120 | 11 | 1,213 | 242 | 33.2 | 28.3-38.3 | 99.1 | 98.4-99.6 | 91.6 | 85.6-95.2 | 83.4 | 82.3-84.4 | 84.0 | 82.2-85.8 |
Table 3
Performance of direct PCR detection based on culture with AFB stain in clinical specimens
AFB smear | TP | FP | TN | FN | Sensitivity | Specificity | PPV | NPV | Accuracy | |||||
---|---|---|---|---|---|---|---|---|---|---|---|---|---|---|
|
|
|
|
|
||||||||||
% | 95% CI | % | 95% CI | % | 95% CI | % | 95% CI | % | 95% CI | |||||
M. tuberculosis | ||||||||||||||
Smear-positive | 91 | 3 | 63 | 6 | 93.8* | 87.0-97.7 | 95.5 | 87.3-99.1 | 96.8 | 90.9-98.9 | 91.3 | 82.8-95.8 | 94.5 | 89.8-97.4 |
Sputum | 67 | 2 | 53 | 6 | 91.8 | 83.0-96.9 | 96.4 | 87.5-99.6 | 97.1 | 89.6-99.2 | 89.8 | 80.4-95.0 | 93.8 | 88.1-97.3 |
BWF | 17 | 1 | 7 | 0 | 100 | 80.5-100.0 | 87.5 | 47.4-99.7 | 94.4 | 73.1-99.1 | 100 | 100.0-100.0 | 96.0 | 79.7-99.9 |
Others | 7 | 0 | 3 | 0 | 100 | 59.0-100.0 | 100 | 29.2-100.0 | 100 | 100.0-100.0 | 100 | 100.0-100.0 | 100 | 69.2-100.0 |
Smear-negative | 111 | 11 | 1,185 | 106 | 51.2* | 44.3-58.0 | 99.1 | 98.4-99.5 | 91.0 | 84.7-94.9 | 91.8 | 90.7-92.7 | 91.7 | 90.1-93.0 |
Sputum | 44 | 4 | 228 | 53 | 45.4 | 35.2-55.8 | 98.3 | 95.6-99.5 | 91.7 | 80.3-96.8 | 81.1 | 78.2-83.8 | 82.7 | 78.1-86.6 |
BWF | 55 | 0 | 143 | 40 | 57.9 | 47.3-68.0 | 100 | 97.5-100.0 | 100 | 100.0-100.0 | 78.1 | 73.9-81.9 | 83.2 | 77.8-87.7 |
Others | 12 | 7 | 814 | 13 | 48.0 | 27.8-68.7 | 99.2 | 98.3-99.7 | 63.2 | 42.5-79.9 | 98.4 | 97.7-98.9 | 97.6 | 96.4-98.6 |
NTM | ||||||||||||||
Smear-positive | 43 | 3 | 97 | 20 | 68.3† | 55.3-79.4 | 97.0 | 91.5-99.4 | 93.5 | 82.3-97.8 | 82.9 | 77.1-87.5 | 85.9 | 79.6-90.8 |
Sputum | 36 | 0 | 73 | 19 | 65.5 | 51.4-77.8 | 100 | 95.1-100.0 | 100 | 100.0-100.0 | 79.3 | 72.8-84.7 | 85.2 | 77.8-90.8 |
BWF | 7 | 0 | 17 | 1 | 87.5 | 47.4-99.7 | 100 | 80.5-100.0 | 100 | 100.0-100.0 | 94.4 | 73.1-99.1 | 96.0 | 79.7-99.9 |
Others | 0 | 3 | 7 | 0 | - | - | 70.0 | 34.8-93.3 | 0 | 0.0-30.9 | 100 | 100.0-100.0 | 70.0 | - |
Smear-negative | 77 | 7 | 1,111 | 218 | 26.1† | 21.2-31.5 | 99.4 | 98.7-99.8 | 91.7 | 83.7-95.9 | 83.6 | 82.6-84.5 | 84.1 | 82.1-86.0 |
Sputum | 35 | 0 | 131 | 163 | 17.7 | 12.6-23.7 | 100 | 97.2-100.0 | 100 | 100.0-100.0 | 44.6 | 43.0-46.2 | 50.5 | 44.9-56.0 |
BWF | 37 | 0 | 154 | 47 | 44.0 | 33.2-55.3 | 100 | 97.6-100.0 | 100 | 100.0-100.0 | 76.6 | 73.1-79.8 | 80.3 | 74.6-85.1 |
Others | 5 | 7 | 826 | 8 | 38.5 | 13.9-68.4 | 99.2 | 98.4-99.7 | 41.7 | 20.7-66.2 | 99.0 | 98.5-99.4 | 98.2 | 97.1-99.0 |
Table 4
AUROC and performance of direct PCR detection based on culture with AFB stain in respiratory specimens