Abstract
Purpose
Materials and Methods
Results
Electronic Supplementary Material
Notes
Ethical Statement
The institutional review board of Samsung Medical Center approved the study protocol (IRB No. SMC 2020-11-048). The need for informed consent was waived as this study was in retrospective nature. All data were anonymized and de-identified before analysis.
Author Contributions
Conceived and designed the analysis: Choi Y, Noh JM, Jeong BH.
Collected the data: Choi Y, Noh JM, Jeong BH.
Contributed data or analysis tools: Choi Y, Noh JM, Shin SH, Lee K, Um SW, Kim H, Pyo H, Ahn YC, Jeong BH.
Performed the analysis: Choi Y, Noh JM.
Wrote the paper: Choi Y, Noh JM.
Supervision: Shin SH, Lee K, Um SW, Kim H, Pyo H, Ahn YC.
Writing - review and editing: Pyo H, Ahn YC, Jeong BH.
Acknowledgments
References


















Fig. 1
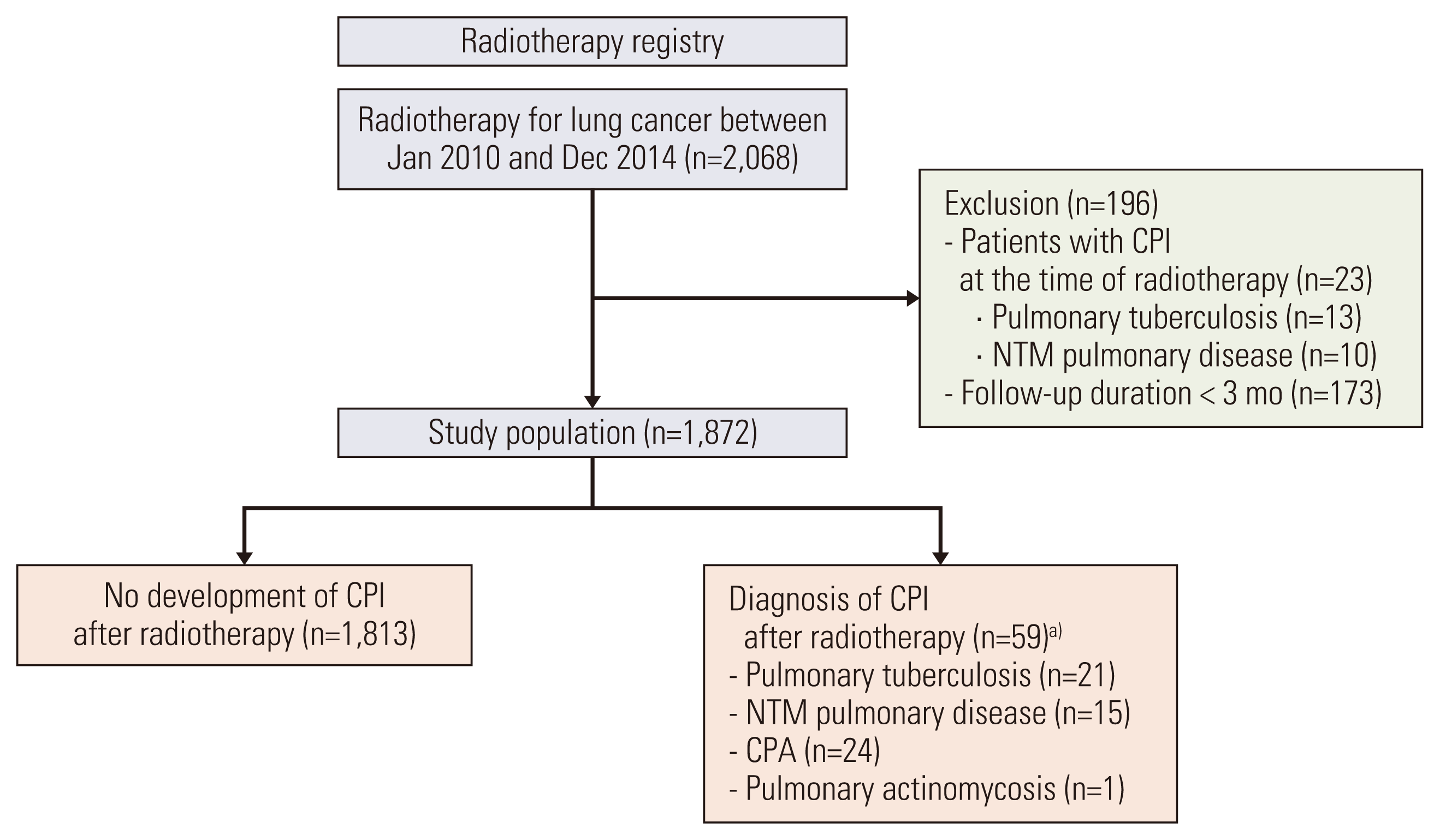
Fig. 2
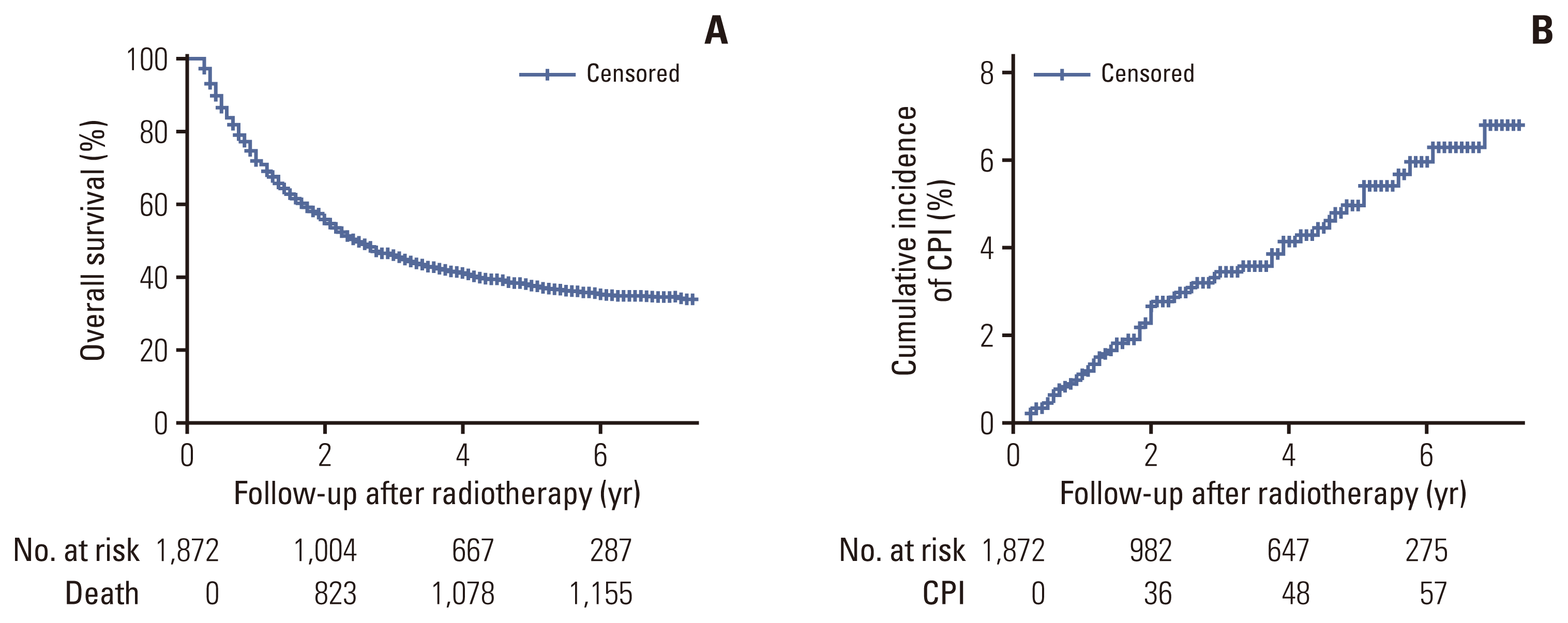
Fig. 3
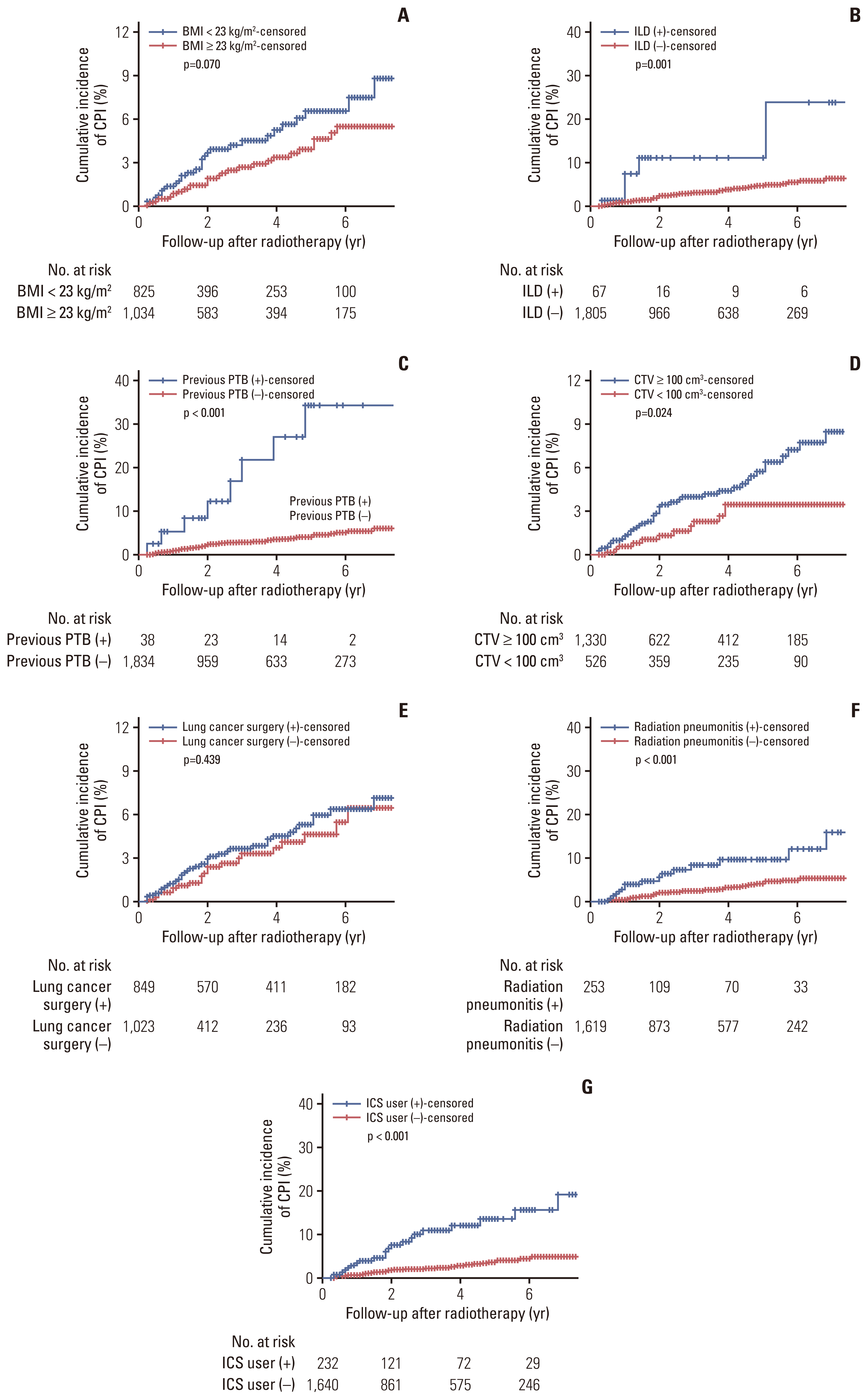
Table 1
Variable | Total (n=1,872) | CPI (−) (n=1,813) | CPI (+) (n=59) | p-value |
---|---|---|---|---|
Age (yr) | 63 (56–70) | 63 (56–70) | 65 (58–69) | 0.609 |
Male | 1,465 (78.3) | 1,415 (78.0) | 50 (84.7) | 0.220 |
BMI (kg/m2) (n=1,859) | 23.4 (21.4–25.4) | 23.4 (21.4–25.4) | 22.7 (20.5–25.2) | 0.229 |
Smoking status (n=1,863) | ||||
Never | 406 (21.8) | 398 (22.0) | 10 (16.9) | 0.575 |
Ex-smoker | 883 (47.4) | 855 (47.4) | 28 (47.5) | |
Current smoker | 574 (30.8) | 553 (30.7) | 21 (35.6) | |
Comorbidity | ||||
Any | 903 (48.2) | 862 (47.5) | 41 (69.5) | 0.001 |
COPD/Asthma | 241 (12.9) | 228 (12.6) | 13 (22.0) | 0.033 |
Interstitial lung disease | 67 (3.6) | 62 (3.4) | 5 (8.5) | 0.057 |
Previous PTB | 38 (2.0) | 30 (1.7) | 8 (13.6) | < 0.001 |
Diabetes mellitus | 372 (19.9) | 354 (19.5) | 18 (30.5) | 0.037 |
Chronic heart disease | 370 (19.8) | 350 (19.3) | 20 (33.9) | 0.006 |
Cerebrovascular disease | 138 (7.4) | 134 (7.4) | 4 (6.8) | > 0.99 |
Chronic liver disease | 66 (3.5) | 64 (3.5) | 2 (3.4) | > 0.99 |
Chronic kidney disease | 56 (3.0) | 55 (3.0) | 1 (1.7) | > 0.99 |
Tumor histology | ||||
NSCLC | 1,548 (82.7) | 1,495 (82.5) | 53 (89.8) | 0.141 |
Adenocarcinoma | 746 (39.9) | 721 (39.8) | 25 (42.4) | |
Squamous cell carcinoma | 651 (34.8) | 627 (34.6) | 24 (40.7) | |
NSCLC, NOS | 98 (5.2) | 95 (5.2) | 3 (5.1) | |
NSCLC, othera) | 42 (2.2) | 41 (2.3) | 1 (1.7) | |
Salivary-gland type carcinomasb) | 11 (0.6) | 11 (0.6) | 0 | |
SCLC | 300 (16.0) | 294 (16.2) | 6 (10.2) | 0.213 |
Sarcoma | 3 (0.2) | 3 (0.2) | 0 | - |
Not proven | 21 (1.1) | 21 (1.2) | 0 | - |
Clinical stagec) | ||||
NSCLC | 1,548 (83.8) | 1,495 (83.6) | 53 (89.8) | |
Stage I | 189 (10.2) | 184 (10.3) | 5 (8.5) | 0.046d) |
Stage II | 166 (9.0) | 160 (8.9) | 6 (10.2) | |
Stage III | 933 (50.5) | 893 (49.9) | 40 (67.8) | |
Stage IV/Recurrent | 260 (14.1) | 258 (14.4) | 2 (3.4) | |
SCLC | 300 (16.2) | 294 (16.4) | 6 (10.2) | |
LD | 241 (13.0) | 235 (13.1) | 6 (10.2) | 0.602d) |
ED/Recurrent | 59 (3.2) | 59 (3.3) | 0 |
Values are presented as median (interquartile range) or number (%). BMI, body mass index; COPD, chronic obstructive pulmonary disease; CPI, chronic pulmonary infection; ED, extensive disease; LD, limited disease; NOS, not otherwise specified; NSCLC, non–small-cell lung cancer; PTB, pulmonary tuberculosis; SCLC, small-cell lung cancer.
a) Large-cell neuroendocrine carcinoma (n=21), adenosquamous cell carcinoma (n=10), sarcomatoid carcinoma (n=10), and carcinoid tumor (n=1),
b) Adenoid cystic carcinoma (n=8), epithelial–myoepithelial carcinoma (n=2), and mucoepidermoid carcinoma (n=1),
c) Excluded patients with sarcoma and histologically not proven tumors. If they were assessed by NSCLC stage, three patients with pulmonary sarcoma were stage III and 21 patients with histologically not-proven tumors were stage I (n=18), stage II (n=2), stage III (n=3), or had recurrent tumors (n=1), respectively,
Table 2
Variable | Total (n=1,872) | CPI (−) (n=1,813) | CPI (+) (n=59) | p-value |
---|---|---|---|---|
Indications of radiotherapy | ||||
Definitive | 849 (45.4) | 826 (45.6) | 23 (39.0) | < 0.001 |
Early stage | 138 (7.4) | 135 (7.4) | 3 (5.1) | |
Locally advanced stage | 711 (38.0) | 691 (38.1) | 20 (33.9) | |
Preoperative | 423 (22.6) | 396 (21.8) | 27 (45.8) | |
Postoperative | 275 (14.7) | 268 (14.8) | 7 (11.9) | |
Salvage | 116 (6.2) | 114 (6.3) | 2 (3.4) | |
Palliative | 209 (11.2) | 209 (11.5) | 0 | |
Radiotherapy technique | ||||
3D-CRT | 1,600 (85.5) | 1,545 (85.2)a) | 55 (93.2) | 0.234 |
IMRT | 162 (8.7) | 161 (8.9)b) | 1 (1.7) | |
SBRT | 94 (5.0) | 91 (5.0) | 3 (5.1) | |
2D-RT | 16 (0.9) | 16 (0.9) | 0 | |
Radiotherapy parameter | ||||
Total dose (Gy) | 52.5 (44.0–60.0) | 52.5 (44.0–60.0) | 50.0 (44.0–60.0) | 0.186 |
BED10 (Gy) | 63.5 (52.8–79.2) | 63.5 (52.8–79.2) | 60.0 (52.8–78.0) | 0.185 |
CTV (cm3) (n=1,856)c) | 167 (88–281) | 167 (87–281) | 163 (112–256) | 0.649 |
Reirradiation | 270 (14.4) | 258 (14.2) | 12 (20.3) | 0.189 |
Concurrent chemoradiation | 1,252 (66.9) | 1,206 (66.5) | 46 (78.0) | 0.066 |
History of lung cancer surgery | 849 (45.4) | 813 (44.8) | 36 (61.0) | 0.014 |
History of radiation pneumonitis | 253 (13.5) | 237 (13.1) | 16 (27.1) | 0.002 |
Medications | ||||
Systemic corticosteroid user | 1,320 (70.5) | 1,274 (70.3) | 46 (78.0) | 0.202 |
Inhaled corticosteroids user | 232 (12.4) | 211 (11.6) | 21 (35.6) | < 0.001 |
Values are presented as median (interquartile range) or number (%). BED10, biologically effective dose with an α/β ratio of 10; CPI, chronic pulmonary infection; CTV, clinical target volume; IMRT, intensity-modulated radiation therapy; SBRT, stereotactic body radiation therapy; 2D-RT, two-dimensional radiation therapy; 3D-CRT, three-dimensional conformal external beam radiation therapy.
Table 3
Variable | Univariable | Multivariable | ||
---|---|---|---|---|
HR (95% CI) | p-value | HR (95% CI) | p-value | |
Age (yr) | 1.02 (0.99–1.05) | 0.137 | - | - |
Male sex | 1.93 (0.95–3.92) | 0.070 | - | - |
BMI (kg/m2) | 0.92 (0.84–1.01) | 0.064 | 0.89 (0.82–0.98) | 0.015 |
Former or current smoker | 1.75 (0.89–3.45) | 0.108 | - | - |
COPD/Asthma | 1.92 (1.04–3.56) | 0.037 | - | - |
Interstitial lung disease | 4.25 (1.70–10.67) | 0.002 | 3.53 (1.34–9.28) | 0.010 |
Previous PTB | 7.21 (3.42–15.22) | < 0.001 | 5.81 (2.61–12.94) | < 0.001 |
Diabetes mellitus | 1.93 (1.11–3.36) | 0.020 | - | - |
Chronic heart disease | 2.32 (1.35–3.98) | 0.002 | 1.70 (0.96–3.02) | 0.068 |
Cerebrovascular disease | 0.89 (0.32–2.45) | 0.820 | - | - |
Chronic liver disease | 0.99 (0.24–4.05) | 0.988 | - | - |
Chronic kidney disease | 0.46 (0.06–3.35) | 0.447 | - | - |
Tumor histology | ||||
Adenocarcinoma | Reference | - | - | |
Squamous cell carcinoma | 1.47 (0.84–2.57) | 0.181 | - | - |
SCLC | 0.85 (0.35–2.07) | 0.714 | - | - |
Others | 0.81 (0.28–2.33) | 0.697 | - | - |
Total dose (Gy) | 0.99 (0.96–1.01) | 0.254 | - | - |
BED10 (Gy) | 1.00 (0.98–1.01) | 0.489 | - | - |
CTV (cm3)a) | 1.01 (1.00–1.02) | 0.146 | 1.02 (1.00–1.03) | 0.047 |
Reirradiation | 1.20 (0.64–2.26) | 0.573 | - | - |
Concurrent chemoradiation | 1.12 (0.60–2.08) | 0.720 | - | - |
History of lung cancer surgery | 1.23 (0.73–2.09) | 0.439 | 1.80 (1.02–3.17) | 0.042 |
History of radiation pneumonitis | 2.79 (1.57–4.96) | < 0.001 | 2.87 (1.55–5.32) | 0.001 |
Systemic corticosteroid user | 1.58 (0.85–2.93) | 0.145 | - | - |
Inhaled corticosteroids user | 3.98 (2.34–6.79) | < 0.001 | 3.31 (1.90–5.77) | < 0.001 |