HUMAN ENTEROVIRUS
The enterovirus belonging to the Picornaviridae includes poliovirus, coxsackieviruses, echovirus, numbered enterovirus, and rhinoviruses (1). Most of the recent enterovirus infections associated with severe neurological changes are due to the occurrence of non-polio enterovirus (NPEV), which constantly threaten public health, as poliovirus, is on the verge of being eradicated by extensive vaccination (2, 3). NPEV is an infectious pathogen common in poor hygiene environments, and the infection route is fecal-oral, and oral transmission through contaminated water and soil. Viruses in feces discharged from infected people flow into groundwater, rivers, and seawater through sewage or wastewater and are transmitted back to humans, but rarely through respiratory secretions (4). Enterovirus is infected through the digestive tract, proliferates primarily in the laryngopharynx or small intestine lymph nodes, and then moves to other tissues and organs, including the central nervous system. Although NPEV infection is often mild, it can cause a wide range of disorders due to various symptoms and severity, most of which occur in infants, children, and immunodeficiency patients. coxsackieviruses, echovirus, and numbered enterovirus are the main causes of aseptic meningitis, and cause neonatal sepsis-like disease, encephalitis, acute flaccid paralysis (AFP), non-specific febrile illness, hand-foot-and-mouth disease (HFMD), herpangina, pleurodynia, pericarditis and myocarditis (1, 5).
Among the numbered enterovirus, EV-A71 has been recognized as an important cause of severe central nervous system disease due to an increase in the number of infections over the past 20 years (3). EV-A71 is known as a common cause of infant HFMD and causes high mortality brainstem encephalitis, meningitis, and polio-like paralysis. EV-D68, another numbered enterovirus, was considered a rare cause of respiratory disease until the early 2000s, but was identified as the cause of severe respiratory disease in children associated with an increase in acute placid myelitis (AFM) in 2014. Since then, the incidence of AFM associated with EV-D68 respiratory disease has increased worldwide according to biennial seasonal patterns (6).
Group A coxsackievirus causes a wide range of myositis affecting striated mussels, and Group B coxsackievirus causes local myositis in striated mussels (7, 8). Coxsackievirus A16 belonging to group A is known to be one of the causes of HFMD. Group B coxsackievirus is associated with more serious diseases, including myocarditis, pericarditis, meningitis, pancreatitis, and spastic paralysis (9, 10). In particular, coxsackievirus B3 causes myocarditis, pericarditis, and sudden cardiac death in the heart, and continues to infect β cells in the pancreas and cause inflammation, acting as a factor in pancreatitis and type 1 diabetes (11, 12).
Rhinovirus is a virus that causes common cold and is mainly infected with upper respiratory cells (13, 14). It is associated with bronchiolitis in infants and exacerbates the patient’s condition with pneumonia, asthma, and COPD (15, 16, 17, 18, 19). In particular, it is reported that children who have suffered from rhinovirus infection are more likely to develop asthma (20). Rhinovirus initially replicates from airway epithelial cells without causing noticeable pathological effects and cell destruction, then damages epithelial cell barriers, causing anterior presence of pathogens and increasing complications of respiratory diseases (21, 22, 23).
As these threats to human health posed by Enterovirus increase, the importance of the countermeasures needed to target this broad group of viruses is emphasized. Except for the two recently released PV vaccines and EV-A71 vaccines in China, there are no antiviral drugs or treatments approved to treat or prevent Enterovirus infection (24).
STRUCTURE OF VIRUS
Enterovirus is a virus that does not have envelope of 27–30 nm size, has a form of tetrahedron, and has a single strand positive sense RNA genome of about 7.2–7.5 kb size. It consists of one open reading frame (ORF) and a non-coding region (NCR) that is not expressed as a protein at the 5’ and 3’ terminals, and ORF accounts for about 90% of all genes and is expressed as one polyprotein, consisting of about 2,185 amino acids 6272282. This polyprotein is divided into several different proteins by viral protease. One polyprotein is divided into structural protein parts P1 and non-structured protein parts P2 and P3, and the P1 part encrypts VP4, VP2, VP3, and VP1 in order, which are components of the virus capsid protein. VP1, VP2, and VP3 are located on the surface of the virus, and VP4 is located inside (Fig. 1). VP1 is a capsid protein composed of 297 amino acids and is the most external and major protein with a receptor binding site called a canyon. VP4 is a protein that holds viral RNA to the capsid (25, 26). The P2 part encrypts 2Apro which is a cysteine protein, 2B proteins which is an ion channel protein, and 2C which act as a NTPase, while the P3 part encrypts 3A which is a membrane binding protein, 3B which is a nucleic acid chaperone protein, known as VPg, 3Cpro which is a cysteine protease and 3D known as RNA dependent RNA polymerase (RD). VPg protein acts on the 3’ side as a primer and is involved in genome formation of the negative sense strand (27, 28). The 5’ NCR consists of 700 to 800bp and forms a well-preserved complex RNA secondary structure. This RNA secondary structure has two functions. The first provides the necessary starting point when positive sense RNA is synthesized by RdRp, and the second allows the synthesis of viral proteins by providing an internal ribosomal entry site (IRES) to which the host’s ribosome first binds in the cap-independent protein synthesis process (29). 3’ NCR consists of 100-150bp, and its secondary structure is known to be involved in the function of primers when negative sense RNA, the template of RNA genomes, is synthesized (Fig. 2) (30, 31, 32, 33, 34).
Fig. 1
Structure of the HEVs virion.
VP1, VP2 and VP3 are showing the psedoequivalent packing arrangement in schematic of the HEVs capsid. VP4 is located inside of the HEVs capsid. The icosahedral asymmetric subunit is not same with the biologic promoter.
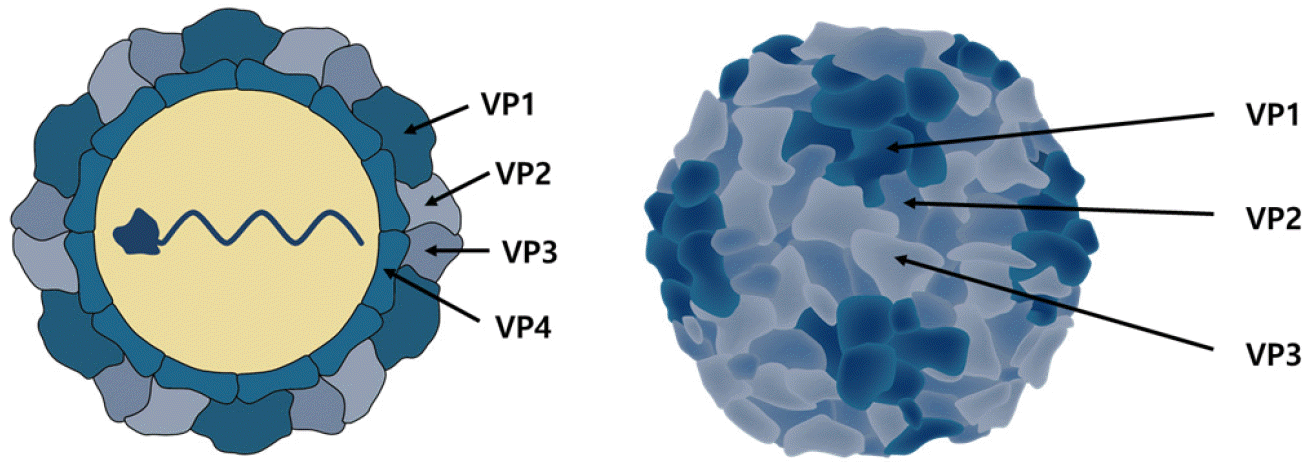
Fig. 2
Schematic of the HEVs genome, the polyprotein products and their major functions.
The human enteroviruses genome is approximately 7.4 kb single-stranded polyadenylated virus RNA genome. P1 region of the genome encodes all the structural proteins. Viral proteases cleaves the P1 region into VP1, VP2, VP3 and VP4. 2A~2C and 3A~3D of seven non-structural proteins encoded by the P2 and P3 regions.
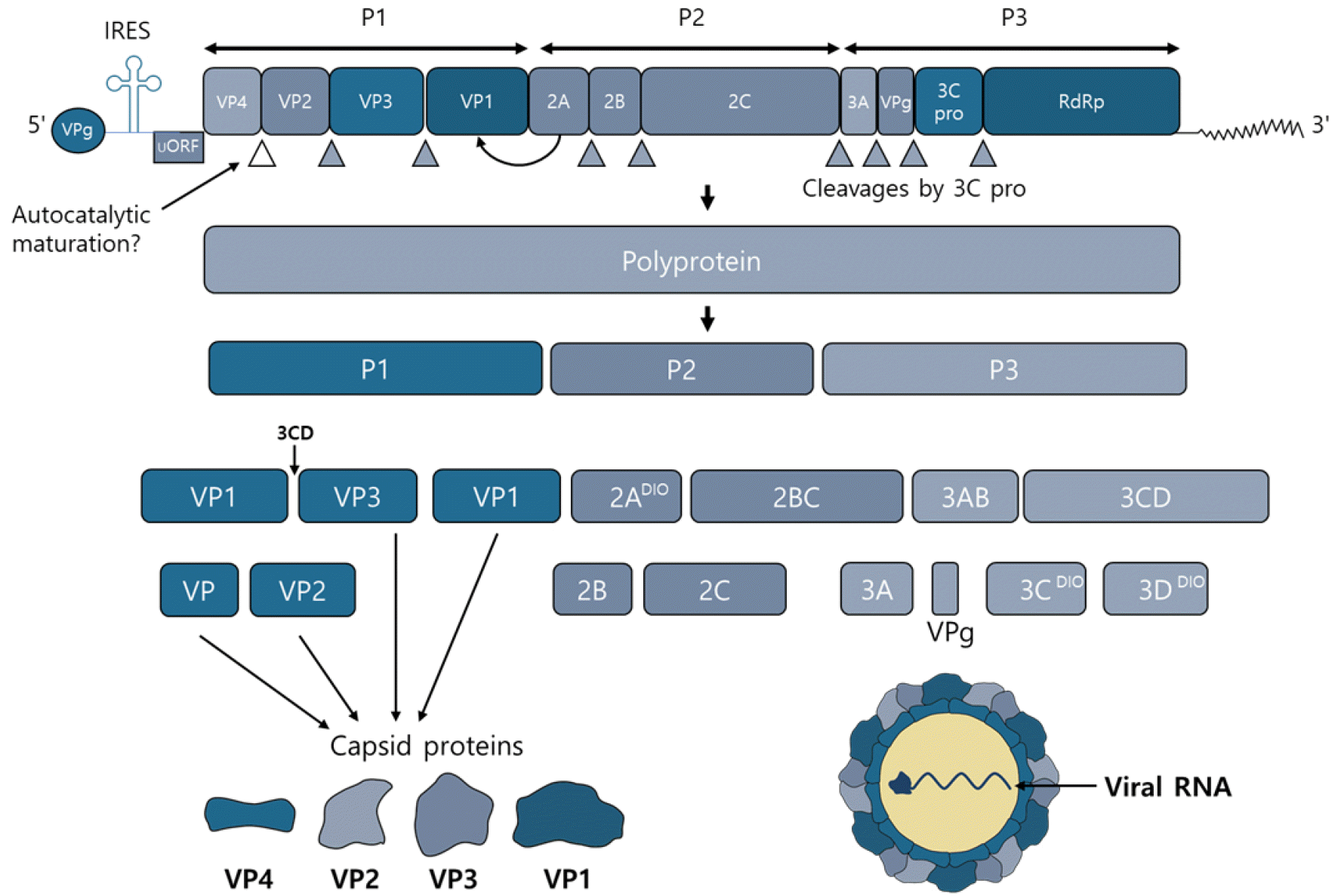
VIRUS LIFE CYCLE
The life cycle of all enterovirus begins with the binding of the virus’ VP1 protein to one or more cell surface receptors, resulting in receptor-mediated endocytosis. Enterovirus can use different endocytic routes depending on serum type and cell type. More than 90% of the viruses in Picornaviridae come through immunoglobulin superfamily (IgSF) acceptors (35). These IgSF include Coxsackievirus and adenovirus acceptor (CAR) of Coxsackievirus and Intercellular Adhesion Molecule 1 (ICAM1) of Human Rhinovirus. Group B coxsackievirus and echovirus are known to interact with attachment receptors such as decay-accelerating factor (DAF) as well as IgSF receptors (36, 37). In the case of EV71, two other receptors were identified: scavenger receptor class B member 2 (SCARB2) and P-selection glycoprotein ligand 1 (PSGL1) (38, 39). Most HRVs use low density lipoprotein receptor (LDLR) and intercellular adhesion molecule-1 (ICAM-1) that bind to the outside of the canyon as receptors, and in the case of HRV-C, cadherin-related family member 3 (CDHR3) is known to be used as receptors (40, 41, 42).
Changes in the binding and pH of receptors in the endosomal system cause viral uncoating, which is the release of viral genomes into the cytoplasm from the viral capsid through the pores of the endosomal membrane. After Genome was transferred to the cytoplasm, the viral RNA was converted into one large polyprotein, followed by proteolysis by viral proteases which is 2Apro, 3Cpro, and 3CDpro and converted to 10 proteins (capsid proteins VP0, VP1, VP3 and some replication proteins 2A, 2B, 2C, 3A, 3B, 3C, and3D) and some functional cut intermediate product. Enterovirus’s genome replication occurs in a viral membrane structure called replication organelles (ROs) (43). Negative-strand genome is synthesized by RNA-dependent RNA polymerase, which is used as a template in positive-strand viral RNA genome synthesis. The newly synthesized viral RNA serves as a template for further translation and replication or is encapsulated with new virions. Enterovirus particles are assembled with the structural capsid proteins VP0, VP1, and VP3 into protomers and pentamers to form a provirion, and then assembled with the synthesized viral RNA to form a mature virion (Fig. 3) (44).
Fig. 3
Schematic of the HEVs lifecycle.
A series of structural changes occur in the virus capsid and formed pores in the cell membrane when an enterovirus binds with the specific receptor on the cell surface. The virion RNA is released into the host cell cytoplasm through the pores in the cell membrane. The parent virus RNA acts as a messenger RNA and is translated into a large polypeptide that is immediately cleaved into 11 mature structural and non-structural proteins by the proteases. Virus genome replicated in a place of vesicle membrane structure by the RNA-dependent RNA polymerases. Viral protease 2A shut down the machinery of the host cellular protein synthesis, while viral protein synthesis remains unaffected. The packaging of a progeny viral RNA into a virus capsid in the cytoplasm of the infected cells is the way of forming infectious virus particle. And these matured infectious virus particles are released when the infected cell is lysated.
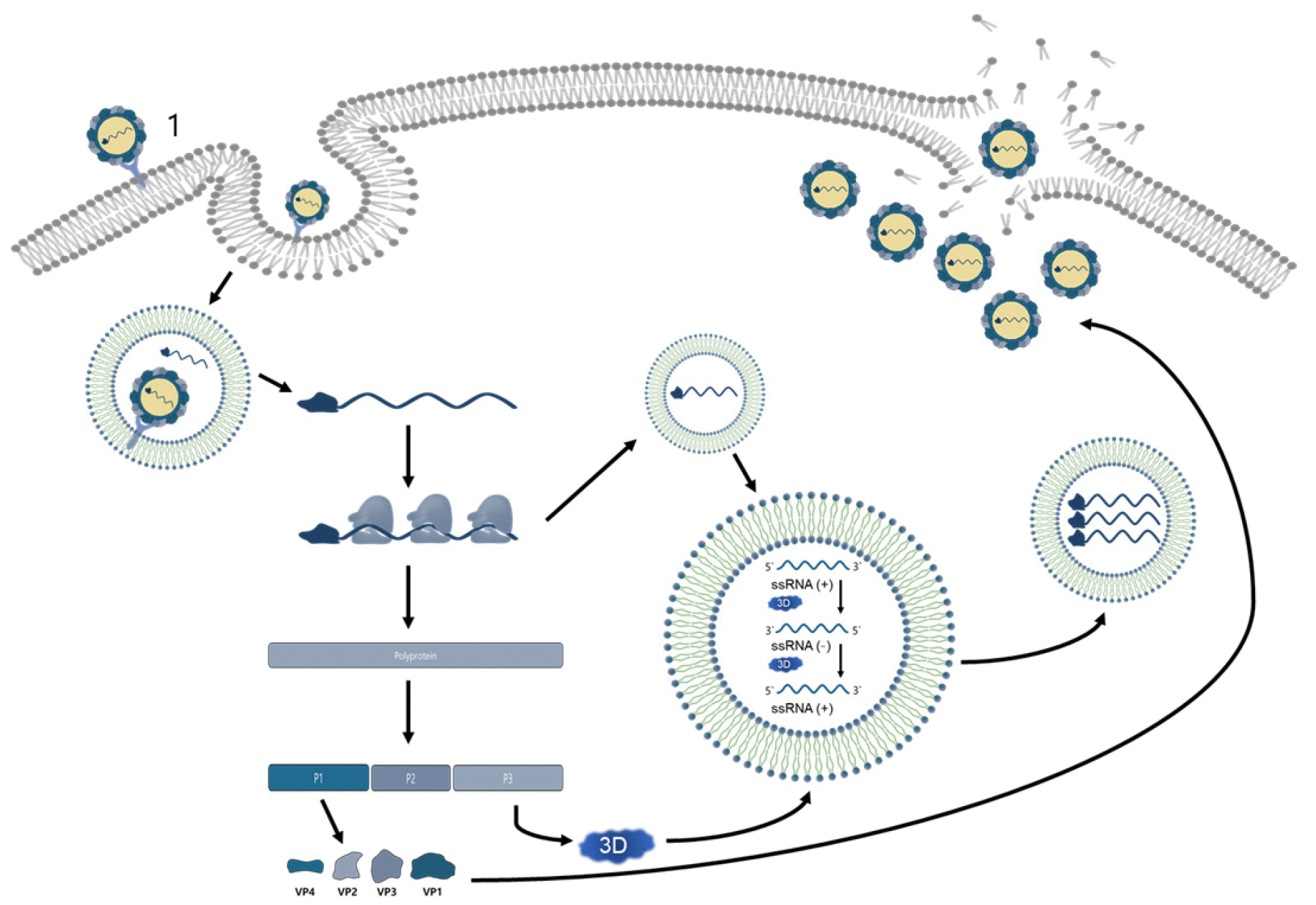
FLAVONOIDS AGAINST VIRUSES
Flavonoid-based compounds are naturally occurring polyphenolic phytochemicals widely found in plants and play various biological functions. Flavonoids have many subcategories, such as flavones, flavanols, flavanonols, flavanones, isoflavones, and anthocyanidins, and researchers have more than 6,000 flavonoids according to the basic structure consisting of c6-c3-c6 (45, 46, 47, 48). Among the pharmacological properties of flavonoids, antioxidant effects are closely associated with numerous diseases such as cancer, Alzheimer’s, and coronary artery disease, and also show pharmacological activity such as anti-tumor, cardiovascular disease prevention (49), antifungal, anti-allergic, estrogen, anti-inflammatory (50), and antiviral activity (45).
Conventional antiviral drugs have the disadvantage of low antiviral activity and varying toxicity to patients, but phytochemicals such as flavonoids can be used as direct medicines because they exhibit a wide range of biological activities (45, 46).
Viruses are classified with DNA or RNA genome and capsid, outer envelope, etc. These viruses rely on the metabolism of the host and surrounding environment to reproduce and survive. They spread the virus using the host’s cellular machine (51). Flavonoids have been studied for a wide range of viruses. Flavonoids can act on various stages, such as the virus’ attachment and entry into cells, virus genome replication, Protein Translation, and poly-protein processing, preventing the released virus from infecting other cells. In addition, indirect control through interaction with the immune system can reduce the load of the virus (45, 46, 52). In this review, the antiviral activity of flavonoid against viruses in the genus of enterovirus will be mainly discussed.
FLAVONOIDS THAT TARGET COXSACKIEVIRUS
Baicalin
Baicalin is a flavonoid mainly contained in scutellaria radix. Baicalin was confirmed to inhibit the formation of autophagy and lipid content increased by infection of CVB3 in Hela cell. To confirm that Baicalin inhibits viral replication and reduces autophagosome formation through cell lipid reduction, exogenous palmitate was added to cell culture to promote lipid synthesis within cells. To confirm that Baicalin inhibits viral replication and reduces autophagosome formation through cell lipid reduction, exogenous palmitate was added to cell culture to promote lipid synthesis within cells. As a result, there was no change in the expression of LC3-II and CVB3/VP1 in both the Hela cell that infected CVB3 and the Hela cell that did not infect CVB3. This shows that lipids play an important role in replication of CVB3, and Baicalin inhibits replication of CVB3 by inhibiting autophagosome formation and cell lipid synthesis (53).
Chysin
Chrysin is natural flavonoids present in plants and contains a large amount of honey and propolis. Chrysin was tested for antiviral activity against coxsackievirus B3. Song et al. confirmed that 10μM concentrations of Chrysin and Chrysin derivatives showed antiviral activity against coxsackievirus B3 in vitro. In addition, chrysin reduced cxcl1 in serum and alleviated pancreatic inflammation in mice infected with CVB3 (54).
Luteolin
Luteolin is a flavonoid present in Resedaluteola. Luteolin inhibits CVB3 dose-dependently and has been shown to reduce CVB3 RNA and protein synthesis. CVB3 inhibition of Luteolin can be a potential inhibitor or supplement to CVB3 infection by inhibits phosphorylation of p38 MAPK and JNK MAPK and suppresses NF-κB nuclear translocation, thereby attenuating the expression of inflammatory cytokines in CVB3-infected cells, thereby suppressing inflammation against CVB3 infection (55).
In addition, Luteolin inhibited cytotoxicity by CVA16 by 50% at a concentration of 10μM. To investigate the dynamics of viral RNA replication for Luteolin, RD cells were inoculated with CVA16 and then tested in a group treated with Luteolin 50μM per hour and not treated. When Luteolin was treated up to 3 hours after infection, viral RNA similarly increased in cells treated with Luteolin and cells not treated. However, 4 hours after infection, viral RNA increased dramatically in cells that were not treated with luteolin but remained constant in cells treated with luteolin. Consequently, Luteolin was thought that CVA16 would affect RNA-dependent RNA polymerase, which is important for viral replication, without affecting binding and entry into cells (56).
Eupafolin
Eupafolin is a flavonoid isolated from the stem of Kalanchoe gracilis. Eupafolin inhibited cytotoxicity by CVA16 in RD cells by about 30%. Phosphorylation of ERK1/2, c-Jun and STAT3 was increased after 9 hours of CVA16 infection in RD cell. However, Eupafolin inhibited the activation of ERK1/2, c-Jun, and STAT3 by CVA16 infection and suppressed upregulation of IL-6 and RANTES gene expression specifically. Therefore, Eupafolin is a potential therapeutic candidate for CVA16 infection through strong antiviral and anti-inflammatory activity (57).
Amentoflavone
Amentoflavone is a biflavonoid component of many plants, including Ginkgobilova, Chamaecyparis obtusa, and Hypericum perforatum. In the event of CVB3 infection, several intracellular pathways for viral transcription, RNA replication, and progeny release are changed, of which the expression of fatty acid synthase (FAS) is increased. One activity of Amentoflavone is based on a direct inhibitory effect on FAS enzyme activity. In order to study antiviral activity through inhibition of FAS enzyme activity for Amentoflavone in CVB3-infected white blood cells, human Raji cells were infected with CVB3, and the effect of FAS-induced expression and FAS inhibitor Amentoflavone on CVB3 replication was analyzed. Treatment of 200μM of Amentoflavone after CVB3 infection in human Raji cells showed about 99% antiviral activity after 8 hours. p38 MAPK is a kinase activated by various stimuli, including viral infection, and regulates various fundamental cell functions, which plays a pivotal role in signaling pathways involved in the regulation of various pathophysiological processes, as it can phosphorylate various transcriptional factors. Since the activation of the p38 MAPK pathway in case of Enterovirus 71 infection was previously demonstrated (58, 59), it was expected that the p38 MAPK pathway would also be activated in case of CVB3 infection. FAS is one of the enzymes regulated by p38 MAPK dependence mechanisms, and activation of p38 MAPK in the early stages of CVB3 replication will trigger immediate expression of FAS in Raji cells. Reduction of p38 MAPK activity in treatment with SB239063, a p38 MAPK inhibitor, in Raji cells actually reduced FAS expression and viral replication. Experiments were conducted using tetrahydrolipstatin orlistat, another FAS inhibitor, to confirm that the enzyme activity of FAS could be involved in CVB3 replication. It is known that tetrahydrolipstatinorlistat inhibits the thioesterase domain of FAS. Treatment of Raji cells infected with CVB3 with Orlistat confirmed that the intracellular viral concentration was reduced and that FAS activity was actually important for CVB3 replication. These results may be helpful in the development of a new treatment approach for common picornavirus-induced diseases as well as CVB3 infections (60).
Dihydroquercetin
Dihydro quercetin (DHQ), a flavonoid extracted from deciduous pine, reduced dose-dependent viral titer in pancreatic tissues of mice infected with CVB3, and restored antioxidant activity of damaged pancreatic tissue. As the first mechanism, DHQ can have direct antiviral activity targeting virus proteins that have not yet been identified. The activity was highest when the compound was added at the initial stage of the viral life cycle (p.i. 0-2 hours), suggesting that DHQ targets the initial stage of viral replication and limiting the reproduction and spread of viruses in target institutions. As the second mechanism, DHQ may control the ROS-mediated signal or metabolic response required for virus replication. As the third mechanism, in pancreatic tissue, DHQ can reduce tissue damage and alleviate the progression of pancreatitis in mice by reducing the level of ROS production by inflammatory cells and processes. This is supported by the results showing that CVB4 infection reduces the antioxidant activity of pancreatic tissue due to the high level of free radicals produced in inflammatory tissue (61).
FLAVONOIDS THAT TARGET ENTEROVIRUS A71
Flavonoid separated from Scutellaria Baicalensis Georgia.
norwogonin, oroxylin A, and mosloflavone isolated from Scutellaria baicalensis Georgia are flavons reported to exhibit antiviral activity in EV-A71. norwogonin, oroxylin A, and mosloflavone all showed viral inhibitory activity of over 60% at a concentration of 50μg/mL. All of these compounds showed antiviral activity within 4 hours of infection and reduced the expression of VP2 protein in EV-A71 (62).
Flavonoids separated from Lagerapterodonta leaves.
Chrysoplenetin and penduletin are o-methylated flavonols separated from the leaves of Lagerapter odonta, indicating antiviral activity against EV-A71. Chrysoplenetin and penduletin both inhibited CPE by 50% at a concentration of 0.17μM in Vero cell, in RD cell, chrysoplenetin showed IC50 values at a concentration of 0.20μM and penduletin at a concentration of 0.37μM. Chrysoplenetin and penduletin were confirmed through time-of-addition experiments to inhibit the stage in which the virus attaches to cells and the stage in which the virus replicates, indicating antiviral activity (63).
Formononetin
Formonetin is a isoflavone with many pharmacological characteristics such as antioxidative, anti-inflammatory, antique activities, acceleration of sound repair, natural protective, and cardioprotective effects. IC50 for EV-A71 in Formononetin was identified as 3.45μmol/L in Vero cell and 3.98μmol/L in SK-N-SH cell. Formononetin reduced VP1 RNA expression and protein expression of EV-A71 with dose dependence. Inflammatory pathways such as ERK, p38, JNK, and MAPK are generally upregulated in EV-A71 infections and help virus reproduction. Formononetin inhibits phosphorylation of ERK, p38 and JNK elevated due to EV-A71.
And EV71-induced COX-2 expression and PGE2 release are suppressed through MAPK pathway (64).
Fisetin
Fisetin is flavonol and has been reported to inhibit CPE caused by the virus by 50% at a concentration of 84.5μM for EV-A71. In the integrator screening asset using recombinant EV-A71 3C protease (3Cpro), fisetin inhibited 3C protease with dose dependence. In Cell-based fluorescence resonance energy transfer (FRET) assay using EV-A71 3C problem motif probe, fisetin was also found to inhibit replication of EV-A71 in cells (65).
Apigenin
Apigenin is a flavonoid known to interact with heterogeneous nuclear ribonucleoprotein A2 (hnRNPA2), a factor associated with splicing regulation, mRNA stability, and mRNA transport. Apigenin binds to a domain rich in C-terminal glycine of hnRNPA2 to prevent hnRNPA2 from forming homogeneous dimers, thus disturbing alternative splicing of several human hnRNPA2 targets. Apigenin inhibited CPE by EV-A71 by 50% at concentrations of 10.3μM in in-vitro and selectively blocked EV71 infection by interfering with viral RNA binding to hnRNPA1 and A2 proteins (66, 67). Apigenin also inhibited 2Apro or 3Cpro, two viral proteases that cut the virus’ polyprotein and by interacting with IRES which starting cap-independent translation to 5′ UTR of EV71, the translation of EV71 was suppressed. In addition, p38 MAP kinase and JNK are phosphorylated upon EV71 infection, and apigenin has been reported to be able to inhibit replication of EV71 by controlling the phosphorylation of JNK (68).
Kaempferol
EV71 has an internal ribosome entry site (IRES) domain for virus translation and replication in 5′-untranslated region (UTR). Among the cell factors, endogenous Far upstream element binding protein 1 (FUBP1), Far upstream element binding protein 3 (FUBP3), Heterogeneous nuclear ribonucleoprotein D0 (HNRPD), Heterogeneous nuclear ribonucleoprotein H (HNRH1), Heterogeneous nuclear ribonucleoprotein F (HNRPF) et al are transaction factors related to EV71 IRES and are involved in the activity of IRES in EV71. Accordingly, kaempferol changes the composition of IRES-related transaction factors and affects IRES function and EV71 virus replication, indicating antiviral activity. To evaluate the antiviral effect of kaempferol on EV71 replication, 35μM kaempferol was treated on RD cells and the virus yield was analyzed at 0, 6, 12, 24 and 48 hours after EV71 infection. Infectious EV71 released from cells treated with kaempferol was at least 1 log unit lower than cells treated with kaempferol regardless of collection time. We also treated kaempferol and confirmed that the virus yield decreased by about 6 logs after 24 hours of EV71 infection (69).
FLAVONOIDS THAT TARGET HUMAN RHINOVIRUS
Quercetin
Quercetin is a plant flavonoid present in many plants, including broccoli, apples, strawberries, onions and tea. Quercetin reduced RV by up to 75-85% at various concentrations in BEAS-2B cells. RV requires phosphoinositide 3-kinase (PI3K) activation used for cell growth and cell proliferation to enter and infect host cells. Quercetin is known to inhibit PI3K. RV was infected with cultured BEAS-2B cells to determine if Quercetin could stop endocytosis. It was confirmed that endocytosis by the virus was suppressed at a quercetin concentration of 10μM. In addition, to confirm the endocytosis-independent effect of quercetin, RV was infected in BEAS-2B cells and incubated for 6 hours to allow enough time for the virus to be endocytosis. Addition of quercetin 6 hours after RV infection reduce viral load, interleukin-8, and IFN responses in BEAS-2B cells. It was found that this was associated with a decrease in level and RV capsid protein of negative and positive strand viral RNA, inhibition of eIF4GI cutting induced by RV, and increase phosphorylation of eIF2α. Increased phosphorylation of eIF2α limits mRNA translation and inhibits viral replication in infected cells. Quercetin treatment confirmed that these phenomena were increased and also confirmed that the inhibition of cutting of eIF4GI could reduce viral genome translation. And through the reduction of RV capsid protein (VP2, VP2+VP4), it was verified that the change in translation initiation induced by quercetin was consistent with the concept that viral genome translation could be reduce (70).
Quercetin treatment in RV-infected mice reduced viral replication and expression of chemokine and cytokine. In addition, quercetin treatment weakened RV-induced airway cholinergic hyperresponsiveness. These results confirmed that Quercetin inhibited endocytosis and replication of RV in airway epitaxial cells, reduced expression of pro-inflammatory cytokines, and improved lung function of RV-infected mice (70).
Sakuranetin
Sakuranetin is a flavonoid belonging to the methoxylated flavanone group, and is widely distributed in Polyomnia fructicosa. Choi et al. reported that sacuranetin is effective against Human rhinoviruses 3 (HRV3) proliferating in human epithelioid carcinoma cervix (HeLa) cell purchased by ATCC (American Type Culture Collection, Manassas, VA, USA). Human rhinovirus 3 is associated with causing colds and exacerbating chronic inflammatory respiratory diseases. In this study, Sakuranetin showed excellent antiviral activity of about 67% at 100ug/mL and about 41% at 10ug/mL for HRV3 (71).
Quercetin 7-glucoside
Quercetin 7-glucoside (Q7G), also known as quercimeritrin, is a member of the class of the components known as flavonoid-7-o-glycosides. After infecting Human rhinovirus 2 (HRV2) in human epithelioid carcinoma cervix (HeLa) cell, addition Q7G showed activity to suppress HRV2 by 50% at a concentration of 4.58μg/ml. And then, after Q7G reacted with HRV2 at 4°C for 1 hour, HRV2 infected HeLa cell and was washed after 1 hour incubation. As a result of confirming the antiviral activity of Q7G 48 hours later, no antiviral activity of Q7G was found. This confirmed that Q7G does not interact with HRV2 particles to exhibit antiviral activity. Next, as a result of the time of addition experiment using Q7G, more than 95% of antiviral activity was shown when Q7G was treated within 3 hours of virus infection, but antiviral activity decreased by more than 40% when Q7G was treated after 4 hours of infection. As a result of this, Q7G predicted that the virus, not an interaction with the viral particles at the initial stage of viral infection, would inhibit the translation or replication stage of the virus after infection with cells (72).
6-chloro-3-methoxy flavone-4′-carboxylic acid and 6-chloro-3-methoxy-4′-oxazolinyl flavone and 6-chloro-4′-oxazolinyl flavonoids.
Desideri et al. infected HeLa cell with human rhinovirus 1B and tested antiviral activity in HRV-1B with various flavonoids. Among them, 6-chloro-3-methoxy flavone-4′-carboxylic acid 6-chloro-3-methoxy-4′-oxazolinyl flavone and 6-chloro-4′-oxazolinyl flavonoids showed strong antiviral activity against HRV-1B. 6-chloro-3-methoxy flavone-4′-carboxylic acid, 6-chloro-3-methoxy-4′-oxazolinyl flavone and 6-chloro-4′-oxazolinyl flavonoids at concentrations of 3.82μM, 5.161μM and 4.461μM, inhibited cell toxicity to HRV-1B by 50% (73).
Orobol 7-O-d-glucoside
Orobol 7-O-d-glucoside (O7G) is a flavonoid separated from Lagerstromia speciosa, and antiviral activity against a wide range of for HRVs, including type A human rhinovirus (HRV1B, HRV2, HRV15 and HRV40)/type B human rhinovirus (HRV3, HRV6 and HRV14)/pleconaril-resistant human virus (HRV5), etc, was evaluated in human epithelioid carcinoma cervix (HeLa) cell. The IC50 concentrations for HRV1B, HRV2, HRV15, HRV40, HRV3, HRV5, HRV6 and HRV14 of O7G are 5.93μg/ml, 4.67μg/ml, 8.80μg/ml, 5.07μg/ml, 0.58μg/ml, 8.18μg/ml, 8.18μg/ml, respectively. These results showed that O7G provides opportunities for the development of broad antiviral drugs for HRVs with more than 100 serotypes (74).
CONCLUSIONS
Flavonoids aimed at various stages of viral infection are becoming more focused topics in exploring their potential as antiviral drugs in the present era. In addition to the classical antioxidant properties, some flavonoids showed to inhibit viruses at the molecular level both in vitro and in vivo. Flavonoid described in this review showed strong antiviral activity at the molecular level both in vitro and in vivo against several viruses in the enterovirus genus. There are currently no approved antiviral drugs for Enteroviruses. This is due to several reasons. First, Enteroviruses are genetically diverse and can mutate rapidly, making it difficult to develop drugs that are effective against all strains. Second, Enteroviruses replicate inside host cells, making it challenging to develop drugs that specifically target the virus without harming the host cells. Third, there has been relatively little investment in the development of antiviral drugs for Enteroviruses compared to other viruses that cause more high-profile diseases (75, 76). Despite these challenges, certain flavonoids have shown potential as candidate substances for development as antiviral drugs for Enteroviruses, considering their in vivo efficacy against the virus. However, the use of flavonoids as antiviral agents requires more pharmacological properties and clinical studies. Further research on flavonoids can provide more insight into the antiviral effects of these phytochemicals.