Abstract
Objective
Chiari II malformation (CM II) is still the main cause of severe morbidity and mortality in children with open neural tube defects (ONTDs). The goal of this study was to validate a CM II model in late-stage chick embryos with surgically induced ONTDs.
Methods
To make the chick embryo model of ONTD, their neural tubes were opened for a length of 5–6 somites at the thoracic level in Hamburger and Hamilton stage 18 chick embryos (n=150). They were reincubated in ovo. up to a total age of 17–21 days. A total of 19 embryos survived and were assigned to either the postoperative day (POD) 14–15 group (n=6) or the POD 17–18 group (n=13). Magnetic resonance imaging (MRI) and histopathologic findings of embryo heads with spinal ONTDs were compared with age-matched normal chick embryos.
Results
The chick embryos with ONTDs demonstrated definite and constant structural changes, such as downward displacement of the cerebellum to just above the foramen magnum and narrow and small cerebrospinal fluid spaces in the crowded small posterior fossa. These morphologic features were more prominent in the POD 17–18 group than in the POD 14–15 group.
Conclusion
This is the first description of CM II with spinal ONTD in a late-stage chick embryo model with MRI and histopathological analysis. The morphological changes of the posterior fossa in this study mimic those of CM II associated with spinal ONTD in humans. This model will facilitate investigation of the pathogenesis of CM II.
Chiari II malformation (CM II) is a representative anomaly associated with spinal open neural tube defects (ONTDs). Despite aggressive management of ONTD, CM II continues to cause various devastating neurological dysfunctions and leads to death in up to 38% of patients with spinal ONTDs [10]. The causal relationships between these entities, the sequence of their occurrences, and the result of fetal therapy for spinal ONTD are major topics of research. The use of proper animal models will provide clues to these questions.
In 1996, we reported Chiari-like malformation associated with surgically induced ONTD in chick embryos at midgestation (total age, 14 days) [12]. Other surgical experimental models of CM II associated with spinal ONTD were demonstrated in mice by Inagaki et al. [7], in rats by Weber Guimarães Barreto et al. [14] and in lambs by Fontecha et al. [3]. In 2000, Paek et al. [9] reported the first large animal model using sheep to demonstrate hindbrain herniation associated with myelomeningocele. These investigators showed that there is a direct causal relationship between CM II and spinal ONTD. Experimental and clinical research has demonstrated that CM II in spinal ONTD can be reversed by performing in utero repair of spinal ONTDs [1,9]. These findings suggested that CM II is caused, at least in part, by the continuous escape of cerebrospinal fluid (CSF) through the ONTD.
The aim of the present study was to validate a CM II model in late-stage chick embryos with surgically induced spinal ONTDs. We reappraised the embryopathophysiology of CM II associated with spinal ONTDs, focusing on magnetic resonance imaging (MRI) as well as histopathological examination. This model will serve as an important research tool to elucidate the pathogenesis of CM II. It will also be useful for the evaluation of various prenatal therapeutic modalities for CM II associated with spinal ONTD.
The Institutional Animal Care and Use Committee (IACUC) policy states that if embryos will be sacrificed prior to three days before hatching, the research is not subject to IACUC review. Since our study was based on unhatched chick embryos under 19 days, the approval for the study was not of any ethical consideration. Also, IACUC came to effect from 2021 (issued in February 5, 2016), and because our experiment was carried out 10 years ago, our research was not subject to the review.
Sim et al. [11] described a detailed method for the induction of ONTD in chick embryos. The procedures are summarized as follows. Fertile eggs were incubated under constant temperature (38–40°C) and humidity (60–70%). Embryos of Hamburger and Hamilton (HH) stage 18 were selected [6]. The posterior roof of the central canal of the neural tube was incised longitudinally for a length of 5–6 somites (approximately 1 mm) with a 30-gauge needle at the thoracic level. Following surgery, they were reincubated in ovo up to a total age of 17–18 and 20–21 days and assigned to two surgical groups, the postoperative day (POD) 14–15 group and the POD 17–18 group. First, six surviving embryos were harvested for the POD 14–15 group. For the remaining embryos, incubation was continued, and the harvesting procedures were then repeated for the POD 17–18 group. The control group consisted of age-matched normal chick embryos and was designated the incubation day (ID) 17–18 group (n=3) and ID 20–21 group (n=3). Because surgical manipulation was performed on HH stage 18 embryos 65–69 hours or approximately 3 days after incubation, these control groups correspond to the two postoperative groups. The length of the ONTD on the harvested embryos was measured with a ruler. The heads of the embryos, which had definite ONTDs, were fixed by immersion in 10% formalin solution.
MRI was performed for three embryo heads of each group using a 1.5 Tesla MRI system (Philips Medical Power tract, 6000 Compact Plus ACS-NT, Amsterdam, the Netherland or Siemens Magneton Vision, Erlangen, Germany). T1-weighted images = 438 / 18 (repetition time [TR] / repetition echo times [TE]); slice thickness : 5 mm, and T2-weighted images = 2718 / 100 (TR / TE); slice thickness : 5 mm with flex small loop coils were obtained in the mid-sagittal (mid- and para-sagittal) plane of the posterior fossa. The representative images obtained were exported in JPEG starting from data DICOM stored on the picture archiving and communicating system [13]. The CSF signal in the infratentorial CSF space was evaluated on or near the midsagittal plane. The size and shape of the bony posterior fossa were evaluated. The size, shape, and location of the cerebellum, brain stem and the presence of hydrocephalic changes were investigated.
After fixation and decalcification of all embryo heads of each group, they were embedded in paraffin and serially sectioned at a thickness of 10 µm along the sagittal plane (suture). Hematoxylin and eosin staining was performed for histopathological examination. Under light microscopy, the size of the cisternal space in the posterior fossa was evaluated near the midsagittal plane. Similarly, the size and shape of both the bony posterior fossa and cerebellum were investigated as were the presence of hydrocephalic changes.
Of the 150 embryos that underwent surgical procedures, 131 died. The mortality rate in the POD 17–18 group was 85.1% (131/144 embryos). Overall, 19 embryos were examined, with isolated ONTD present in five embryos in the POD 14–15 group and 11 in the POD 17–18 group. Spontaneous closure of surgically induced ONTD was present in three embryos (one in the POD 14–15 group and two in the POD 17–18 group) and was excluded from this study (Table 1). There were no other abnormalities on gross examination. The mean length of the ONTD was 5.4 mm (range, 4.0–7.0) in the POD 14–15 group and 6.2 mm (range, 3.0–8.0) in the POD 17–18 group.
The morphology of the ONTD was quite similar to that of human ONTD, as described in our previous reports on microscopic evaluation of surgically induced ONTDs. Our model showed progressive eversion and hyperplasia, which are features seen in spontaneous ONTDs. Also, compared to rodent model that have long tails, secondary neurulation process is known to be more similar to human. In combination with the fact that surgical manipulation is easy and that it can be performed under direct vision, we thought that chick embryos would be a good model in evaluating the etiology of CM II.
The CSF signal was visible around the hindbrain and between the cerebellum and brainstem on the para-sagittal plane of the control group. The growth of the bony posterior fossa paralleled that of the supratentorial bony compartment in the control group and was independent of cerebellar growth. Significant cerebellar growth occurred in the ID 20–21 group compared to the ID 17–18 group. The configuration of the cerebellum gradually elongated rostrally and caudally (Fig. 1A and B).
MRI evaluation of the posterior fossa in embryos with ONTDs showed that the bony posterior fossa was smaller than that in the control group, with a rather flattened curvature. The CSF signal was also apparently absent or barely visible due to overcrowding in the surgical group. The cerebellum was located more caudally, but herniation into the vertebral column was not observed. The morphological changes were more prominent in the POD 17–18 group than in the POD 14–15 group. However, despite these features of CM II in the chick embryos with ONTDs, there was no evidence of hydrocephalus in this study (Fig. 1C and D).
Compared with the control groups, the embryos with ONTD had distinct morphological changes. Similar to the previously noted MRI findings, their skull was rather flattened, and the shape of the cerebellum was elongated rostrally and caudally. Additionally, the cerebellum was located at lower levels with a shorter distance between the posterocaudal end of the cerebellum and the upper margin of the posterior arch of C1. The spaces between the cerebellar cortex, anteriorly with the dorsal surface of the upper brain stem and posteriorly with the inner table of the skull, were narrower and smaller in the surgical group. The subarachnoid spaces around the cerebellar cortex were also narrower.
These morphologic changes were more prominent in the POD 17–18 group than in the POD 14–15 group. No evidence of hydrocephalus was present (Fig. 2).
Since CM II was first reported by Clelande in 1883 and described by Chiari in 1891, many theories have sought to explain its pathophysiology, and various therapeutic modalities, including fetal surgery, have been developed to improve the outcome of patients with CM II associated with spinal ONTD. Due to the complex nature of CM II, proper animal models are essential for understanding and developing treatment strategies for this disease entity.
Several surgical models of CM II have been developed in large mammals, such as sheep (term, 147–161 days). However, they require special facilities with high costs and long experimental periods because of their long gestation period. Among small mammals, the gestation periods are relatively shorter, and since their pregnancies are multiparous, they can be regarded as good candidates for study animals. However, they cannot tolerate the surgical manipulations and survive to late term. Although chick embryos are not mammals, from our experiences, they can be easily manipulated during the early incubation period under direct vision and can tolerate the operation well. They allow us to perform surgical procedures at the exact time, location, and size required. Previous reports have described the advantages and disadvantages of the surgical chick embryo model for the study of ONTD and CM II. The surgically induced ONTDs at the early phase allow the CSF to leak out throughout the incubation period, which also occurs in human cases. This will provide an opportunity to observe the morphologic changes that occur in the posterior fossa throughout the whole incubation period. Additionally, the growth pattern of the bony posterior fossa and cerebellum resembles that of human cases, providing us with further understanding.
In our study in 1996, we reported a Chiari-like malformation model associated with surgically induced ONTD in 14-day-old chick embryos. At that time, ONTDs were induced by making a longitudinal incision, 9–11 somite long, at an HH stage of 16–19. The histopathological results revealed that the majority of the embryos with ONTDs had smaller fourth ventricles and narrower subarachnoid spaces in the small bony posterior fossa than the non-ONTD control group. The cerebellum was elongated rostrally and caudally and displaced downward in severe cases. Hydrocephalus did not occur. The pilot phase of our study suggested a strong direct causal relationship between CM II and spinal ONTD. However, the morphologic changes of the posterior fossa were not always typical or consistent in the midgestational embryos. We predicted that analyzing later-stage embryos with surgically induced ONTD would demonstrate more typical morphological features of CM II.
Therefore, we tried to get the chick embryos to survive as long as possible, into a later stage of the incubation period, in the present study. The ONTDs were induced by making a shorter longitudinal incision, 5–6 somites long, to increase the survival rates of the embryos. The survival rates of the embryos slightly improved to approximately 15%. The location of the lesion was confined to the thoracic area because there were no differences between the cervicothoracic group and the lumbosacral group in a previous study [12]. The other techniques were almost the same as those used in the previous studies.
The morphologic findings with MRI complementary to the histologic examination showed representative features of the posterior fossa in CM II, readily distinguishing the surgical group from the control group. These findings also demonstrated that the posterior fossa was smaller in the surgical groups with a substantial decrease in the amount of CSF around the growing cerebellum compared to the control group. The caudal part of the cerebellum was displaced downward in the surgical groups, but it did not herniate into the upper cervical column. Compared to the previously used mid-gestational embryos, these surgical groups showed definite and constant morphologic changes in the posterior fossa characterized in CM II, similar to those of humans. The morphologic changes were more prominent in the POD 17–18 group than in the POD 14–15 group, reflecting the fact that the cerebellum commences its growth at later incubation periods and rapidly grows in the near term. From this, we can predict that more typical features of CM II would be observed at postnatal stages.
In our previous study, we evaluated the development of the normal posterior fossa in chick embryos with an incubation of 14–20 days using conventional MRI. The chick embryos showed a relatively late commencement of cerebellar growth, approximately from IDs 16–17, compared to the growth of the cerebral hemisphere and the bony posterior fossa. The cerebellum grew rapidly at approximately IDs 18–20.
Our results are compatible with the MRI studies of the human fetus [16]. In the normal development of the human fetal brain, the volume of the bony posterior fossa parallels that of the supratentorial compartments and precedes the growth of the cerebellum. The most important factor for the increase in skull size is the pressure exerted by the underlying growing brain. The growth of the cerebral hemisphere and supratentorial bony component is closely matched in the fetus, supporting the causal link between the two. However, the rate of growth of the bony posterior fossa is not correlated with that of the cerebellum but with that of the supratentorial bony component. The growth of the posterior cranial fossa in the second trimester is more rapid than that in the first trimester, with the fastest growth rates during the 4th and 5th months of gestation [5].
The cerebellum has a dual mesencephalic-metencephalic origin. Thus, the development of the cerebellum is closely related to the brainstem. The infratentorial CSF space surrounding the hindbrain is large at gestational weeks 31–32 and remains visible on fetal MRI in later gestational stages. Significant growth of the cerebellum is observed during the last weeks (33–37 weeks of gestation), which is disproportionate to that of the bony posterior fossa [4].
The development of CM II with spinal ONTD and its impact on the developing nervous system begins in the embryos and continues throughout the whole gestational period. The lack of a ventricular CSF driving force due to leakage through the ONTD results in a smaller bony posterior fossa, which is characteristic of CM II. With the later rapid growth of the hindbrain, the cerebellum is forced both cephalad and caudal along with the brain stem. These progressive changes lead to an overcrowded posterior fossa and hindbrain herniation at term or in the postnatal stages.
As seen in human fetuses with CM II during the late first and early second trimesters, cerebellar herniation below the foramen magnum and hydrocephalus are absent in chick embryos due to the late commencement of cerebellar growth. This supports the theory that further growth of the hindbrain is required for the compromise of CSF spaces, resulting in hydrocephalus, which can then cause additional displacement of the hindbrain [15]. Our current study revealed the narrowing of subarachnoid spaces and crowding of posterior fossa but no hydrocephalus. Similarly, rat models suggested by Briner and Lieske [2], showed that none of fetal rats with spinal ONTD and hindbrain herniation showed hydrocephalus. According to these results, it can be hypothesized that in both rodents and avian, hydrocephalic changes could occur in later postnatal stages of development like human. Based on Unified theory, proposed by McLone and his colleagues [8], it would be interesting to speculate about the occurrence of hydrocephalus because surgically induced ONTDs at the early stage allow the CSF to leak out throughout the incubation period. This will provide an opportunity to observe the morphologic changes of posterior fossa during the incubation period and will provide further understandings of human cases due its resemblance.
To the best of our knowledge, this is the first MRI study of CM II associated with surgically induced ONTD in late-stage chick embryos. Technically, exact visualization of the histopathologic features of the fetal posterior fossa is limited due to artifacts from the skull base during decalcification and paraffin sectioning. Complementary to histopathological examinations, MRI can be used as a noninvasive imaging technique in the evaluation of chick embryos. In this study, postmortem MRI, although insufficient, provided visualization of the posterior fossa components, such as the cerebellum, brain stem and CSF spaces, without significant artifacts. Although there are limitations of MRI images, such as embryo motion artifacts and partial volume averaging with fluids such as amniotic fluid, yolk and albumen, they can provide additional information to complement histological examination. The techniques for overcoming these limitations are beyond the scope of this study.
In conclusion, we validated a simple and reliable CM II model in late-stage chick embryos with appropriate reproducibility and little variability. Characterization of the posterior fossa by MRI and histological examination in chick embryos with surgical ONTD demonstrated progressive pathologic changes seen in CM II with morphologic similarity to those of humans. We think that this model can serve as an important tool in understanding the pathogenesis of CM II and can be applied in future experimental studies investigating various fetal therapeutic strategies.
Notes
ACKNOWLEDGMENTS
This work was supported by the 2022 education, research and student guidance grant funded by Jeju National University.
References
1. Bouchard S, Davey MG, Rintoul NE, Walsh DS, Rorke LB, Adzick NS. Correction of hindbrain herniation and anatomy of the vermis after in utero repair of myelomeningocele in sheep. J Pediatr Surg. 38:451–458. discussion 451-458. 2003.


2. Briner W, Lieske R. Arnold-Chiari-like malformation associated with a valproate model of spina bifida in the rat. Teratology. 52:306–311. 1995.


3. Fontecha CG, Peiro JL, Sevilla JJ, Aguirre M, Soldado F, Fresno L, et al. Fetoscopic coverage of experimental myelomeningocele in sheep using a patch with surgical sealant. Eur J Obstet Gynecol Reprod Biol. 156:171–176. 2011.


5. Griffiths PD, Wilkinson ID, Variend S, Jones A, Paley MN, Whitby E. Differential growth rates of the cerebellum and posterior fossa assessed by post mortem magnetic resonance imaging of the fetus: implications for the pathogenesis of the chiari 2 deformity. Acta Radiol. 45:236–242. 2004.


6. Hamburger V, Hamilton HL. A series of normal stages in the development of the chick embryo. J Morphol. 88:49–92. 1951.


7. Inagaki T, Schoenwolf GC, Walker ML. Experimental model: change in the posterior fossa with surgically induced spina bifida aperta in mouse. Pediatr Neurosurg. 26:185–189. 1997.


8. McLone DG, Knepper PA. The cause of Chiari II malformation: a unified theory. Pediatr Neurosci. 15:1–12. 1989.


9. Paek BW, Farmer DL, Wilkinson CC, Albanese CT, Peacock W, Harrison MR, et al. Hindbrain herniation develops in surgically created myelomeningocele but is absent after repair in fetal lambs. Am J Obstet Gynecol. 183:1119–1123. 2000.


10. Park TS, Hoffman HJ, Hendrick EB, Humphreys RP. Experience with surgical decompression of the Arnold-Chiari malformation in young infants with myelomeningocele. Neurosurgery. 13:147–152. 1983.


11. Sim KB, Cho BK, Chi JG, Wang KC. Morphological study of surgically induced open neural tube defect in old (14 and 21 days) chick embryos. Neurosci Lett. 192:61–64. 1995.


12. Sim KB, Hong SK, Cho BK, Choi DY, Wang KC. Experimentally induced Chiari-like malformation with myeloschisis in chick embryos. J Korean Med Sci. 11:509–516. 1996.


13. Sim KB, Lee CS, Shin TK. A study for normal development of the posterior cranial fossa in the chick embryos (gestation 14-20 days) with MR images and histopathology. Environmental Mutagens and Carcinogens. 25:25–31. 2005.
14. Weber Guimarães Barreto M, Ferro MM, Guimarães Bittencourt D, Violin Pereira LA, Barini R, Sbragia L. Arnold-Chiari in a fetal rat model of dysraphism. Fetal Diagn Ther. 20:437–441. 2005.


Fig. 1.
Mid-sagittal magnetic resonance imaging images of the head of chick embryos. Control group : (A) incubation day (ID) 17–18 and (B) ID 20–21. Surgical group : (C) postoperative day (POD) 14–15 and (D) POD 17–18. T1 weighted image (WI) (A) and T2 WI (B) : sagittal images show the cerebrospinal fluid (CSF) signal (marked with yellow arrows) surrounding the hindbrain and between the cerebellum and brainstem. Significant cerebellar growth occurred in the ID 20–21 group compared to the ID 17–18 group. Embryos in the ID 17–18 group showed physiologic levels of CSF in the posterior fossa and a normal configuration of the cerebellum. The configuration of the cerebellum also becomes elongated rostrally and caudally to some extent. T1 WI (C) and T1 WI (D) : mid-sagittal images demonstrate that the CSF signal surrounding the hindbrain and between the cerebellum and brainstem is absent or barely visible due to overcrowding in the smaller bony posterior fossa. The cerebellum is more elongated rostrally and caudally and is located more caudally. The morphologic changes are more prominent in the POD 17–18 group than in the POD 14–15 group. There is no evidence of hydrocephalus.
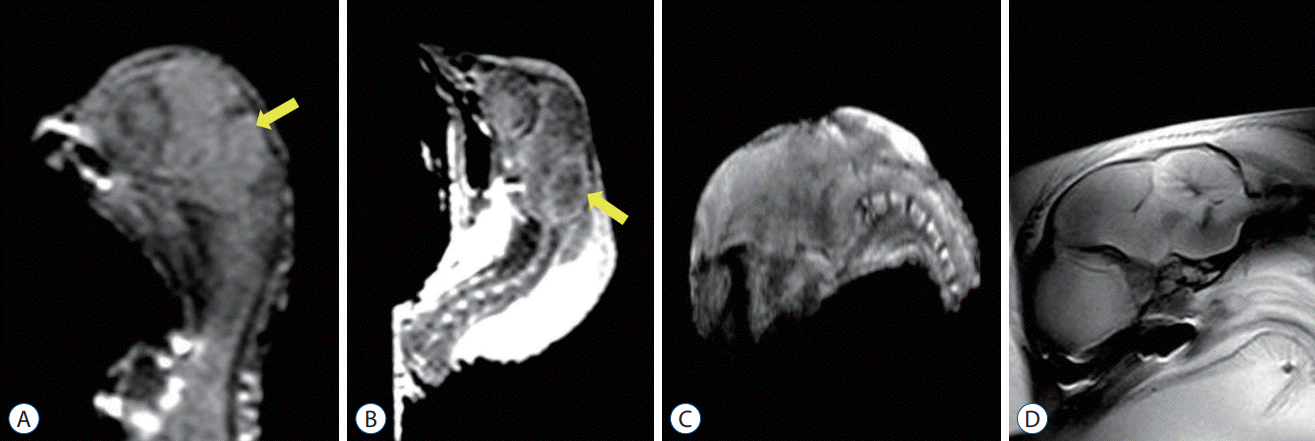
Fig. 2.
Light microscopic findings of a median section of the posterior fossa of a chick embryo. Control group : (A) incubation day (ID) 17–18 and (B) ID 20–21 (Hematoxylin and Eosin [H&E] stain, ×12.5). Surgical group : (C) postoperative day (POD) 14–15 group and (D) POD 17–18 group (H&E stain, ×12.5). The subarachnoid spaces between the cerebellar cortex and the inner surface of the skull and between the cerebellum and the brainstem (marked with red arrow and yellow arrow, respectively) are narrower in the surgical group than in the control group. The cerebellum is elongated rostrally and caudally and located downward in the overcrowded smaller posterior fossa. There is no evidence of hindbrain herniation or hydrocephalus. The cerebellum increases in size, and a folia pattern appears. There is significant development of the cerebellar hemispheres, along with an increase in volume manifested by the more typical mushroom-shaped configuration in both the surgical and control groups with increased incubation time. Of note, the cerebellum did not completely herniate into the cervical spinal canal.
