Abstract
Background
The common reference intervals (RIs) for thyroid hormones currently used in China are provided by equipment manufacturers. This study aimed to establish thyroid hormone RIs in the population of Lanzhou, a city in the subplateau region of northwest China, and compare them with previous reports and manufacturer-provided values.
Methods
In total, 3,123 individuals (1,680 men, 1,443 women) from Lanzhou, an iodine-adequate area of China, perceived as healthy were selected. The Abbott Architect analyzer was used to determine the serum concentration of thyroid hormones. The 95% RI was estimated using the 2.5th and 97.5th percentiles as the lower and upper reference limits, respectively.
Results
The serum levels of thyroid-stimulating hormone (TSH), total triiodothyronine (TT3), antithyroglobulin (ATG) antibody, and antithyroid peroxidase (ATPO) antibody levels were significantly correlated with sex (P<0.05). TSH, total thyroxine (TT4), and ATPO levels were significantly correlated with age (P<0.05). The serum levels of TSH, ATG, and ATPO in men were significantly lower than in women; in contrast, the serum TT3 level was significantly higher in men than in women (P<0.05). Serum TSH, TT3, TT4, and ATG levels differed across age groups (P<0.05), but no such variation was observed for ATG levels (P>0.05). The established RIs of TSH, ATG, and ATPO in this study differed between sexes (P<0.05). The thyroid hormone RIs established herein were inconsistent with the manufacturer-provided values.
Thyroid hormone levels, regulated by feedback mechanisms mediated by the hypothalamus–pituitary–thyroid axis, are crucial for the normal development and proper functioning of physiological systems [1-3]. A previous epidemiological survey in 31 cities in China showed that the overall prevalence of thyroid diseases was 50.96%, with higher prevalence rates among older than younger adults [4]. Since the prevalence of thyroid diseases is very high, understanding the mechanisms underlying the variations in thyroid-stimulating hormone (TSH) and thyroid hormone levels is crucial. Serum TSH, total triiodothyronine (TT3), total thyroxine (TT4), free triiodothyronine (FT3), and free thyroxine (FT4) levels are useful biomarkers in the clinical diagnosis of thyroid dysfunction, including subclinical thyroid disease [2,5,6]. An increase in the levels of positivity for thyroid autoantibodies, such as antithyroglobulin (ATG) antibody and antithyroid peroxidase (ATPO) antibody, may inform the diagnosis of autoimmune connective tissue disease [7]. According to the 2017 American Thyroid Association guidelines, establishing population-based and assay-specific reference intervals (RIs) [8] is required for accurately diagnosing thyroid dysfunction [9]. Notably, the RIs used presently in Lanzhou, China and elsewhere [10] are defined by testing equipment manufacturers and are derived from data from other countries. The RIs do not consider the influence of the environment or ethnicity on thyroid hormone levels, which may affect the clinical diagnosis. Previous studies have shown that thyroid hormone RIs differ among ethnic groups [11-13]. Lanzhou, located in Western China, has an average elevation of 1,500 meters above sea level and is a multi-ethnic city dominated by Han, Hui, Tibetan, Dongxiang, and other ethnic groups. Lanzhou residents’ actual iodine nutrition status is iodine-replete after the universal salt iodization (USI) program was implemented to correct iodine deficiencies in China about 20 years ago [14]. In this study, we hypothesized that the RIs established based on the local population in Lanzhou and Abbott chemiluminescence apparatus (Abbott, Santa Clara, CA, USA) would be inconsistent with the RIs supplied by the equipment manual due to the differences in source population characteristics. In our previous study, we established RIs specific to individuals from Lanzhou using an indirect method based on data from the laboratory information system and indicated deviations from the manual instructions provided [15]. The indirect method of establishing RIs depends on a large amount of data collected in a laboratory [16,17]. The data should exhibit a normal distribution or can be transformed into a normal distribution using mathematical methods [18]. However, the TSH-related data did not conform to a Gaussian distribution, even after converting them by the Box-Cox or caserank transformation methods. Accordingly, the primary aim of this study was to establish Abbott chemiluminescence apparatus-specific thyroid hormone RIs derived from the local population in Lanzhou. Establishing RIs by the direct method was proposed by the International Federation of Clinical Chemistry and Laboratory Medicine. The secondary objective of this study was to compare the RIs obtained in this study with those included in the manufacturer’s instructions.
All procedures in this study were approved by the Institutional Review Board at Gansu Provincial Hospital (protocol: 2022-359). This study conformed to the standards set by the latest revision of the Declaration of Helsinki. A total of 3,123 individuals (without an established disease or any chronic treatment) who perceived themselves as healthy and resided in Lanzhou, an iodine-adequate area of China (n=3,123, 1,680 men and 1,443 women; median aged 48 years [range, 40 to 54]) were recruited from June to November 2019 at the Gansu Provincial Hospital Physical Examination Center. Written informed consent was obtained from all participants after informing them of the intended use of the samples. Among them, 2,014 participants (1,016 men) were excluded for at least one of the criteria recommended by the National Academy of Clinical Biochemistry: (1) abnormal serum levels of ATPO or ATG antibody; (2) a history of thyroid dysfunction (goiter, hyperthyroidism, thyroid inflammation, thyroid tumor); (3) a family history of thyroid disease; (4) abnormal thyroid ultrasonography results; (5) the presence of type 2 diabetes mellitus; (6) a systolic blood pressure >140 mm Hg or a diastolic pressure of >94 mm Hg; (7) pregnancy; (8) smoking before blood collection; and (9) hepatitis or other chronic diseases. All participants denied taking medications such as phenytoin or glucocorticoids that would affect the detection of thyroid hormones (Table 1).
Blood samples were collected during the morning hours (6:00 AM to 9:00 AM) following a more than 8-hour fast (fasting after 10:00 PM) from the cubital vein of the participants. The serum TSH, TT3, TT4, ATPO, and ATG levels were determined within 6 hours by the chemiluminescent microparticle immunoassay method using an Architect i2000 immunochemistry analyzer (Abbott). Two qualified and suitably trained researchers conducted serum sample testing according to the applicable standards and instructions. Two reference standards were tested, and quality control was performed before the test every day.
Data were analyzed using Excel 2010 (Microsoft Inc., Redmond, WA, USA), SPSS version 19.0 (IBM Inc., Armonk, NY, USA), and GraphPad Prism version 6.0 (GraphPad Software, La Jolla, CA, USA). The Kolmogorov-Smirnov test was used to evaluate the data distribution; normally distributed data were presented as mean±standard deviation. The Student’s t test or analysis of variance was used to compare groups (sex and age). Non-normally distributed data are presented as median (interquartile range [IQR]), and nonparametric analyses were used to compare these variables between groups. P values <0.05 were considered statistically significant. The 95% RI was estimated using the 2.5th percentile as the lower reference limit and the 97.5th percentile as the upper reference limit.
Associations between sex, age, and thyroid hormone levels are presented in Table 2. The serum levels of TSH, TT3, ATG, and ATPO, but not TT4, were significantly correlated with sex (P<0.05). The levels of TSH, TT4, and ATPO were correlated with age (P<0.05); in contrast, the levels of TT3 and ATG were not associated with age (P>0.05).
Fig. 1 shows the distributions of both sexes’ TSH, TT3, and FT4 levels. The serum levels of TSH (median, 1.74 mIU/L [IQR, 1.25 to 2.48]), ATG (median, 0.90 IU/L [IQR, 0.70 to 1.30]), and ATPO (median, 0.90 IU/mL [IQR, 0.30 to 2.31]) in men were significantly lower than those in women (TSH: median, 2.24 mIU/L [IQR, 1.60 to 3.00]; ATG: median, 1.03 IU/mL [IQR, 0.76 to 1.59]; ATPO: median, 1.25 IU/mL [IQR, 0.50 to 2.76]; P<0.05). However, the serum TT3 level was significantly higher in men (1.49±0.22 nmol/L) than in women (1.42±0.20 nmol/L; P<0.05). The serum TT4 level difference between the sexes was not significant (men: 97.28±14.26 pmol/L vs. women: 98.17±14.16 pmol/L, P>0.05).
Fig. 2 shows the distribution of TSH, TT4, and TT3 levels in the Lanzhou participants according to age. The serum TSH, TT3, TT4, and ATG levels differed among the groups (P<0.05), except for serum ATG (P>0.05).
The overall and sex- and age-specific RIs for TSH, TT3, TT4, ATG, and ATPO levels estimated by the direct method are summarized in Table 3. The established RIs of TSH in men (95% confidence interval [CI], 0.67 to 4.62 mIU/L) were narrower than those in women (95% CI, 0.72 to 5.15 mIU/L), with different lower and upper limits between the sexes. Although the TT3 levels differed between the sexes, the corresponding lower limits were very close, while the upper limit in men was higher than in women (men: 95% CI, 1.07 to 1.91 nmol/L; women: 95% CI, 1.05 to 1.78 nmol/L). The TT4 level showed no significant difference between the sexes, and there was some overlap in the range of TT4 RIs between the sexes (men: 95% CI, 70.52 to 125.08 pmol/L; women: 95% CI, 70.26 to 126.00 pmol/L), which were relatively close. The upper limits of ATG (men: <2.90 IU/mL vs. women: <3.73 IU/mL) and ATPO (men: <4.60 IU/mL vs. women: <5.26 IU/mL) differed between the sexes. Although stratification for age in establishing the RIs was not recommended if the subgroups contained more than 2,000 values [19], we found that the examined RIs changed with age (Table 2), suggesting that age is an important parameter in the diagnosis of thyroid dysfunction.
Table 3 shows the differences between the thyroid-associated hormone RIs established in this study and those recommended by the manufacturer.
Table 4 shows a comparison of the TSH RIs in the present study and those of previous studies with various national and ethnic groups. The lower limit of TSH RIs estimated in different national or ethnic groups varied from 0.29 (American White) to 1.134 mIU/L (Indian), and the upper limit ranged from 3.24 (France) to 7.280 mIU/L (Indian).
The RIs of thyroid hormone levels in the healthy population in the residents of Lanzhou (in the subplateau region of China) in this study were inconsistent with those supplied in the manufacturer’s descriptions and the published literature. Each laboratory should establish separate reference ranges. Setting a sex-specific reference range of thyroid hormones is also necessary to diagnose thyroid diseases.
This study included 3,123 individuals perceived as physically fit. Among them, 2,014 participants (1,016 men) were excluded based on the criteria recommended by the National Academy of Clinical Biochemistry. The influence of thyroid nodules on thyroid dysfunction is a matter of debate [20]. A retrospective study reported an increased risk of thyroid nodule malignancy associated with the elevation of ATG, ATPO, and TSH [21]. However, other studies have suggested that approximately 71% to 79% of individuals with thyroid nodules have TSH levels in the normal range [22]. In this present study, the serum ATPO and ATG of each enrolled participant was detected, and the thyroid morphology was checked by palpation and ultrasound. Individuals with thyroid nodules were excluded to avoid confounding. Finally, only 1,109 participants (664 men) were included in the present study. This process revealed that it is challenging to recruit participants who meet the Clinical and Laboratory Standards Institute criteria.
The RIs of thyroid hormones help determine abnormal thyroid function [4,5,23]. The RIs currently used in China are derived from European and Northern American populations, which are not equivalent to the Chinese population [24]. It is necessary to establish values representative of the local population [10]. With its elevation of 1,500 m, Lanzhou is located in the subplateau zone. Previous studies have reported that altitude affects thyroid hormone levels [25,26]. We hypothesized that there would be a difference in the RIs of thyroid hormones of individuals from Lanzhou those supplied by the manufacturers. Our previous studies established this population’s normal reference range of thyroid-related hormones using an indirect method [15]. FT3 and FT4 were not included as indices in the present study, mainly because we found that the FT3 and FT4 levels were in line with a normal distribution. Therefore, we estimated the normal reference range of FT4 and FT3 in a previous study [15], and we did not include FT3 and FT4 as topics of research in the present study. Lanzhou, the capital of Gansu Province, has been iodine-replete since the national implementation of USI legislation in China in 1996. More recently, a Chinese nationally representative cross-sectional study including 78,470 participants showed that the median urinary iodine concentration (representing iodine intake) in the adult population was 177.89 μg/L (95% CI, 117.99 to 263.90) [27]. These values represent an adequate status of iodine intake in Lanzhou. However, despite applying transformation methods, TSH levels did not follow a Gaussian distribution; consequently, the indirect derivation of hormone levels was invalid. The establishment of RIs relies on three major factors: population selection, sampling and analysis methods, and statistical analysis [28,29]. The differences in the RIs of thyroid hormones between the values established in this study and those supplied by the manufacturer were as follows: TSH, 0.70 to 4.93 mIU/L in this study vs. 0.35 to 4.94 mIU/L from the manufacturer; TT3, 1.05 to 1.88 ng/L in this study vs. 0.98 to 2.33 ng/L from the manufacturer; TT4, 70.56 to 125.51 pmol/L in this study vs. 62.68 to 150.84 pmol/L from the manufacturer; ATG, <3.12 IU/mL in this study vs. <4.11 IU/mL from the manufacturer; and ATPO, <4.80 IU/mL in this study vs. <5.61 IU/mL from the manufacturer. The RIs obtained for TSH, TT3, and TT4 in this study were narrower than those provided by the manufacturer. In addition, we showed that the upper limits of the RIs of ATG and ATPO serum levels calculated in this study were lower than those supplied by the manufacturers. The use of the manufacturer-provided values may lead to misdiagnosis of thyroid dysfunction. These discrepancies may be due to the differences in the source population characteristics, including geographical setting and ethnic makeup. Thyroid hormone RIs differ among ethnic groups [12,30,31], and these values may not be generalizable from one group to another. Previous studies have described variations in TSH RIs between different countries and ethnic groups (Table 4). These studies were conducted in 11 countries in Asia, the Americas, Oceania, and Europe [32-42]. Large fluctuations in TSH RIs were found not only between different continents, but also within the same continent. In Asia, the upper and lower limits of the Indian TSH RIs were higher than those in South Korea, Japan, China (present study), and Turkey. India is a multi-ethnic country located in the South Asian subcontinent. Apart from ethnic differences, the prevalence of mild iodine deficiency disorder in India may be a probable reason for the significantly high TSH RIs [34]. The lower limit of the TSH RIs in seven European and American countries fluctuates between 0.3 (England) and 0.64 mIU/L (France), and the upper limit ranged from 3.24 (France) to 5.8 mIU/L (USA). We suggest that the TSH RIs might be related to ethnicity, geographical location, diet, and especially iodine intake, since even within a single country, differences can be found among ethnic and racial subgroups; for instance, in the United States, the TSH RIs differ among Caucasians, Mexicans, and African Americans [37].
This study followed strict eligibility criteria. Individuals with high blood pressure, diabetes, active smoking habits, and those with thyroid nodules detected by ultrasonography or a history of thyroid disease or other clinical disease were excluded; the final study sample included 1,109 individuals to ensure the study’s reliability.
Whether sex-specific or age-specific RIs for thyroid hormones should be established is debated [12,43]. In this study, TSH, ATPO, and ATG were higher in women than in men; these findings are consistent with some previous studies and inconsistent with others [10,12,44]. Xie et al. [10] recently reported that the levels of T3 and FT3 were lower in women than in men, while T4 and FT4 levels were relatively similar between the sexes. We derived sex-specific values for serum TSH, TT3, ATG, and ATPO levels. Sex probably affects TSH levels via estrogen-dependent pathways, genetic susceptibility, and environmental factors [44], and women are more vulnerable to autoimmune thyroid disorders than men. Consistent with previous studies, our study showed no significant differences in TT4 levels and RIs between the sexes. The levels of TSH, TT3, ATG, and ATPO differed among age groups; however, those of TT4 did not. The influence of age on thyroid hormone levels has not yet been clarified. Some studies showed minor effects of age on TSH reference ranges; however, in those studies, the median TSH level increased with age in participants aged >85 years [45,46]. Other studies reported that the values of TSH increased with age, which could be attributed to an age-related alteration in the TSH set point or reduced TSH bioactivity rather than occult thyroid disease. Because TSH levels gradually increase with age, some elderly people might be diagnosed with subclinical hypothyroidism. The prevalence of subclinical hypothyroidism may be significantly overestimated unless age-specific TSH RIs are used [46,47]. However, inconsistent evidence has been reported on the effects of age on TSH levels [4,11]. Agespecific RIs were established in this study to indicate that the influence of age on thyroid-related hormone levels should be considered (specifically, for patients aged >70 years) in the diagnosis of hypothyroidism.
To our knowledge, this study is the first to establish age- and sex-specific thyroid hormone RIs by a direct method in a sample of the healthy population residing in the Lanzhou region.
ACKNOWLEDGMENTS
This work was supported by the Talent Introduction Fund of Gansu Provincial People’s Hospital (22GSSYB-3).
We thank Professor Jian-Jun Wu for his professional assistance in statistics.
REFERENCES
1. Evron JM, Papaleontiou M. Decision making in subclinical thyroid disease. Med Clin North Am. 2021; 105:1033–45.


2. Ortiga-Carvalho TM, Chiamolera MI, Pazos-Moura CC, Wondisford FE. Hypothalamus-pituitary-thyroid axis. Compr Physiol. 2016; 6:1387–428.
3. Babic Leko M, Gunjaca I, Pleic N, Zemunik T. Environmental factors affecting thyroid-stimulating hormone and thyroid hormone levels. Int J Mol Sci. 2021; 22:6521.


4. Liu Y, Shan Z; Endocrine Metabolic Diseases Group of the Chinese Geriatrics Society; Thyroid Group of the Chinese Society of Endocrinology, Chinese Medical Association. Expert consensus on diagnosis and treatment for elderly with thyroid diseases in China (2021). Aging Med (Milton). 2021; 4:70–92.


5. Biondi B, Cappola AR, Cooper DS. Subclinical hypothyroidism: a review. JAMA. 2019; 322:153–60.
6. Esfandiari NH, Papaleontiou M. Biochemical testing in thyroid disorders. Endocrinol Metab Clin North Am. 2017; 46:631–48.


7. Wang Q, Shangguan J, Zhang Y, Pan Y, Yuan Y, Que W. The prevalence of thyroid autoantibodies in autoimmune connective tissue diseases: a systematic review and meta-analysis. Expert Rev Clin Immunol. 2020; 16:923–30.


8. Han L, Zheng W, Zhai Y, Xie X, Zhang J, Zhang S, et al. Reference intervals of trimester-specific thyroid stimulating hormone and free thyroxine in Chinese women established by experimental and statistical methods. J Clin Lab Anal. 2018; 32:e22344.


9. Xiong J, Liu S, Hu K, Xiong Y, Wang P, Xiong L. Study of reference intervals for free triiodothyronine, free thyroxine, and thyroid-stimulating hormone in an elderly Chinese Han population. PLoS One. 2020; 15:e0239579.
10. Xie T, Su M, Feng J, Pan X, Wang C, Tang T. The reference intervals for thyroid hormones: a four year investigation in Chinese population. Front Endocrinol (Lausanne). 2023; 13:1046381.


11. Jonklaas J, Razvi S. Reference intervals in the diagnosis of thyroid dysfunction: treating patients not numbers. Lancet Diabetes Endocrinol. 2019; 7:473–83.


12. Li ZZ, Yu BZ, Wang JL, Yang Q, Ming J, Tang YR. Reference intervals for thyroid-stimulating hormone and thyroid hormones using the access TSH 3rd IS method in China. J Clin Lab Anal. 2020; 34:e23197.


13. Wang X, Li Y, Zhai X, Wang H, Zhang F, Gao X, et al. Reference intervals for serum thyroid-stimulating hormone based on a recent nationwide cross-sectional study and meta-analysis. Front Endocrinol (Lausanne). 2021; 12:660277.


14. Liu T, Li Y, Teng D, Shi X, Yan L, Yang J, et al. The characteristics of iodine nutrition status in china after 20 years of universal salt iodization: an epidemiology study covering 31 provinces. Thyroid. 2021; 31:1858–67.


15. Lu Y, Yue JY, Li DH, Zhou SH. To establish reference interval for serum thyroid hormone in adults of subaltitude determined by indirect method. Int J Lab Med. 2018; 39:2832–41.
16. Jones GR, Haeckel R, Loh TP, Sikaris K, Streichert T, Katayev A, et al. Indirect methods for reference interval determination: review and recommendations. Clin Chem Lab Med. 2018; 57:20–9.
17. Zierk J, Baum H, Bertram A, Boeker M, Buchwald A, Cario H, et al. High-resolution pediatric reference intervals for 15 biochemical analytes described using fractional polynomials. Clin Chem Lab Med. 2021; 59:1267–78.


18. Wang Y, Zhang YX, Zhou YL, Xia J. Establishment of reference intervals for serum thyroid-stimulating hormone, free and total thyroxine, and free and total triiodothyronine for the Beckman Coulter DxI-800 analyzers by indirect method using data obtained from Chinese population in Zhejiang Province, China. J Clin Lab Anal. 2017; 31:e22069.
19. Ma C, Wang X, Xia L, Cheng X, Qiu L. Effect of sample size and the traditional parametric, nonparametric, and robust methods on the establishment of reference intervals: Evidence from real world data. Clin Biochem. 2021; 92:67–70.


20. Oron T, Lazar L, Feldhamer I, Manor M, Koren-Morag N, Tenenbaum A, et al. Pediatric reference values of TSH should be personalized according to BMI and ethnicity. Eur J Endocrinol. 2020; 183:419–26.


21. Adhami M, Michail P, Rao A, Bhatt CR, Grodski S, Serpell JW, et al. Anti-thyroid antibodies and TSH as potential markers of thyroid carcinoma and aggressive behavior in patients with indeterminate fine-needle aspiration cytology. World J Surg. 2020; 44:363–70.


22. Goundan PN, Lee SL. Lack of a threshold size for autonomously functioning thyroid nodules that excludes hyperthyroidism. Endocr Pract. 2019; 25:1263–7.


23. Sun Q, Avallone L, Stolze B, Araque KA, Ozarda Y, Jonklaas J, et al. Demonstration of reciprocal diurnal variation in human serum T3 and rT3 concentration demonstrated by mass spectrometric analysis and establishment of thyroid hormone reference intervals. Ther Adv Endocrinol Metab. 2020; 11:2042018820922688.


24. Zou Y, Wang D, Cheng X, Ma C, Lin S, Hu Y, et al. Reference intervals for thyroid-associated hormones and the prevalence of thyroid diseases in the Chinese population. Ann Lab Med. 2021; 41:77–85.


25. von Wolff M, Nakas CT, Tobler M, Merz TM, Hilty MP, Veldhuis JD, et al. Adrenal, thyroid and gonadal axes are affected at high altitude. Endocr Connect. 2018; 7:1081–9.


26. Nepal O, Pokhrel BR, Khanal K, Gyawali P, Malik SL, Koju R, et al. Thyroid hormone levels in highlanders- a comparison between residents of two altitudes in Nepal. Kathmandu Univ Med J (KUMJ). 2013; 11:18–21.


27. Li Y, Teng D, Ba J, Chen B, Du J, He L, et al. Efficacy and safety of long-term universal salt iodization on thyroid disorders: epidemiological evidence from 31 provinces of Mainland China. Thyroid. 2020; 30:568–79.


28. Barth JH, Luvai A, Jassam N, Mbagaya W, Kilpatrick ES, Narayanan D, et al. Comparison of method-related reference intervals for thyroid hormones: studies from a prospective reference population and a literature review. Ann Clin Biochem. 2018; 55:107–12.


29. Xing D, Liu D, Li R, Zhou Q, Xu J. Factors influencing the reference interval of thyroid-stimulating hormone in healthy adults: a systematic review and meta-analysis. Clin Endocrinol (Oxf). 2021; 95:378–89.


30. Ho CK, Tan ET, Ng MJ, Yeo GS, Chern B, Tee NW, et al. Gestational age-specific reference intervals for serum thyroid hormone levels in a multi-ethnic population. Clin Chem Lab Med. 2017; 55:1777–88.


31. Lee S, Ong CM, Zhang Y, Wu AH. Narrowed reference intervals for complete blood count in a multiethnic population. Clin Chem Lab Med. 2019; 57:1382–7.


32. Kwon H, Kim WG, Jeon MJ, Han M, Kim M, Park S, et al. Age-specific reference interval of serum TSH levels is high in adolescence in an iodine excess area: Korea national health and nutrition examination survey data. Endocrine. 2017; 57:445–54.


33. Yoshihara A, Noh JY, Ohye H, Sato S, Sekiya K, Kosuga Y, et al. Reference limits for serum thyrotropin in a Japanese population. Endocr J. 2011; 58:585–8.
34. Sarkar R. Non-transference of biological reference interval of TSH by electrochemiluminescence immunoassay: an Indian population perspective. Clin Chim Acta. 2013; 423:130–4.


35. Yildiz Z, Dagdelen LK. Reference intervals for thyroid disorders calculated by indirect method and comparison with reference change values. Biochem Med (Zagreb). 2023; 33:010704.


36. Hickman PE, Koerbin G, Simpson A, Potter JM, Hughes DG, Abhayaratna WP, et al. Using a thyroid disease-free population to define the reference interval for TSH and free T4 on the Abbott Architect analyser. Clin Endocrinol (Oxf). 2017; 86:108–12.


37. Hollowell JG, Staehling NW, Flanders WD, Hannon WH, Gunter EW, Spencer CA, et al. Serum TSH, T(4), and thyroid antibodies in the United States population (1988 to 1994): National Health and Nutrition Examination Survey (NHANES III). J Clin Endocrinol Metab. 2002; 87:489–99.
38. Gurgel MH, Ponte CM, Fontes R, Rocha IV, Batista LA, Sousa TC, et al. Establishment of reference interval for thyroid-stimulating hormone using electrochemiluminescence assay in a healthy adult population from Fortaleza, Brazil. Arch Endocrinol Metab. 2020; 64:362–8.
39. Larisch R, Giacobino A, Eckl W, Wahl HG, Midgley JE, Hoermann R. Reference range for thyrotropin: post hoc assessment. Nuklearmedizin. 2015; 54:112–7.
40. Reix N, Massart C, d’Herbomez M, Gasser F, Heurtault B, Agin A. Thyroid-stimulating hormone and free thyroxine on the ADVIA Centaur immunoassay system: a multicenter assessment of analytical performance. Clin Biochem. 2013; 46:1305–8.


41. Javaid U, Kennedy D, Addison C, Tsatlidis V, Razvi S. Frequency, determinants and costs of thyroid function testing in a laboratory serving a large population. Eur J Endocrinol. 2022; 186:553–60.


42. Clerico A, Trenti T, Aloe R, Dittadi R, Rizzardi S, Migliardi M, et al. A multicenter study for the evaluation of the reference interval for TSH in Italy (ELAS TSH Italian Study). Clin Chem Lab Med. 2018; 57:259–67.


43. Bohn MK, Higgins V, Asgari S, Leung F, Hoffman B, Macri J, et al. Paediatric reference intervals for 17 Roche cobas 8000 e602 immunoassays in the CALIPER cohort of healthy children and adolescents. Clin Chem Lab Med. 2019; 57:1968–79.


44. Musa IR, Ali NI, Elseed SA, Osman OE, Adam I. Reference intervals of thyroid hormones in Khartoum, Sudan. BMC Res Notes. 2018; 11:729.


45. Kahapola-Arachchige KM, Hadlow N, Wardrop R, Lim EM, Walsh JP. Age-specific TSH reference ranges have minimal impact on the diagnosis of thyroid dysfunction. Clin Endocrinol (Oxf). 2012; 77:773–9.


Fig. 1.
Serum levels of (A) thyroid-stimulating hormone (TSH), (B) total triiodothyronine (TT3), (C) total thyroxine (TT4), (D) antithyroglobulin (ATG) antibody, and (E) antithyroid peroxidase (ATPO) antibody according to sex.
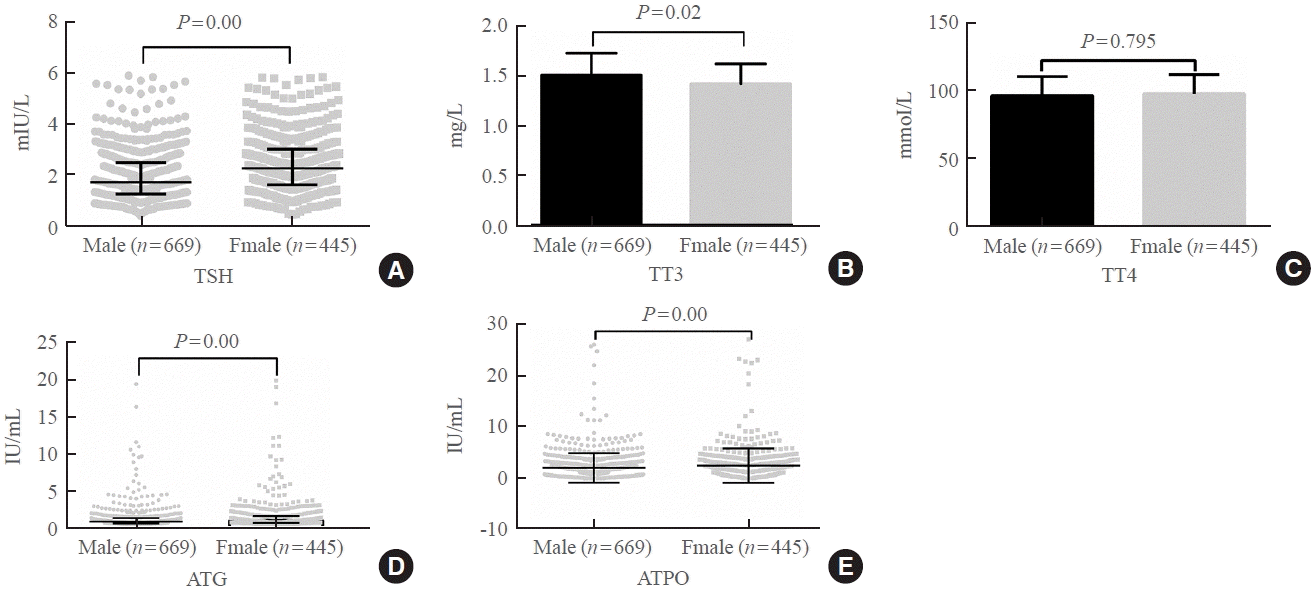
Fig. 2.
Serum levels of (A) thyroid-stimulating hormone (TSH), (B) total triiodothyronine (TT3), (C) total thyroxine (TT4), (D) antithyroglobulin (ATG) antibody, and (E) antithyroid peroxidase (ATPO) antibody in Lanzhou residents according to age.
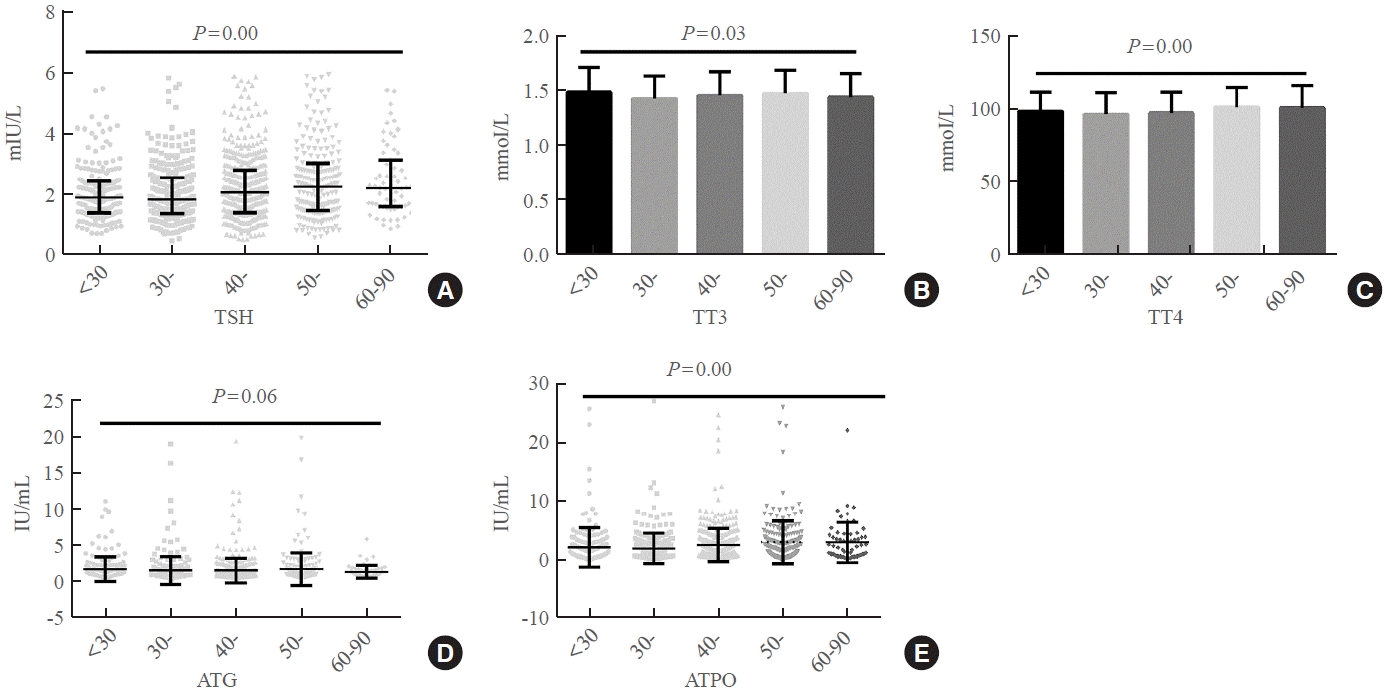
Table 1.
Baseline Characteristics of Participants
Table 2.
Correlations of Sex and Age with Serum Levels of Thyroid Hormones
Table 3.
Reference Intervals for Thyroid-Associated Hormones Established in This Study and Those Recommended by the Manufacturer
Table 4.
Comparison of TSH Reference Intervals in the Present Study and Those of Previous Studies with Various National and Ethnic Groups
Nationality | TSH, mIU/L | Equipment | Method | Reference |
---|---|---|---|---|
China (present study) | 0.70–4.93 | Chemiluminescence immunometric assay | Direct method | |
Korea | 0.62–6.84 | Electro-chemiluminescence immunoassays | Direct method | [32] |
Japan | 0.44–4.93 | Electro-chemiluminescence immunoassays | Direct method | [33] |
India | 1.134–7.280 | Electro-chemiluminescence immunoassays | Direct method | [34] |
Turkey | 0.41–4.37 | Electro-chemiluminescence immunoassays | Indirect method | [35] |
Australia | 0.43–3.28 | Abbott Architect ci16200 | Direct method | [36] |
USA | 0.33–5.8 (total) | Chemiluminescence immunometric assay | Direct method | [37] |
0.29–6.47 (White) | ||||
0.37–3.9 (Black) | ||||
0.38–5.72 (Mexican Americans) | ||||
Brazil | 0.56–4.45 | Electro-chemiluminescence immunoassays | Direct method | [38] |
German | 0.57–3.32 | Chemiluminescence immunometric assay | Direct method | [39] |
France | 0.64–3.24 | Chemiluminescence immunometric assay/Electro-chemiluminescence immunoassays | Direct method | [40] |
England | 0.3–4.5 | Electro-chemiluminescence immunoassays | Direct method | [41] |
Italy | 0.362–5.280 | Enzymatic chemiluminescence immunoassays | Direct method | [42] |