Abstract
The discovery and development of antimicrobial therapies represents one of the most significant advancements in modern medicine. Although the primary therapeutic intent of antimicrobials is to eliminate their target pathogens, several antimicrobials have been shown to provide analgesia as a secondary benefit. Antimicrobials have demonstrated analgesic effects in conditions that involve dysbiosis or potential subclinical infection (e.g., chronic low back pain with Modic type 1 changes; chronic prostatitis/chronic pelvic pain; irritable bowel syndrome; inflammatory bowel disease; functional gastrointestinal disorders/dyspepsia; myalgic encephalomyelitis/chronic fatigue syndrome), and might even prevent the chronification of pain after acute infections that are associated with excessive systemic inflammation (e.g., post COVID-19 condition/long Covid, rheumatic fever). Clinical studies often assess the analgesic effects of antimicrobial therapies in an observational manner, without the ability to identify causative relationships, and significant gaps in the understanding remain regarding the analgesic potential of antimicrobials. Numerous interrelated patient-specific, antimicrobial-specific, and disease-specific factors altogether contribute to the perception and experience of pain, and each of these requires further study. Given worldwide concerns regarding antimicrobial resistance, antimicrobials must continue to be used judiciously and are unlikely to be repurposed as primary analgesic medications. However, when equipoise exists among several antimicrobial treatment options, the potential analgesic benefits of certain antimicrobial agents might be a valuable aspect to consider in clinical decision-making. This article (the second in a two-part series) aims to comprehensively review the evidence on the prevention and treatment of chronic pain using antimicrobial therapies and suggest a framework for future studies on this topic.
The multipurpose use of antimicrobial treatments to treat pain and other non-infectious conditions predates the advent of antibiotics and includes arsenic to treat pain in dentistry [1], mercury to treat myriad pain conditions [2], and bloodletting [3], which was considered a panacea for millennia in Western civilization for nearly all human ailments. Since the onset of widespread antibiotic use, physicians have also documented the commensurate alleviation of pain that accompanies the eradication or reduction in microbial burden.
As noted in the first part of this two-part series, antimicrobial therapies have also been shown to alleviate pain via off-target effects, independent of their germicidal properties and via direct targeted effects (e.g., reducing the microbial burden in spine pain and dyspepsia). Similar to other major medical advances, these effects are often discovered serendipitously. In the literature, there are numerous examples of antimicrobial agents exerting antinociceptive effects via indirect mechanisms. Signaling via the mammalian target of rapamycin, which controls mRNA translation and mediates opioid tolerance and hyperalgesia, is a potential target for improving opioid efficacy and analgesia [4]. In preclinical studies, the antifungal agent rapamycin has been shown to reduce inflammatory and neuropathic pain [5,6]. Other animal studies have found that tetracycline derivatives may attenuate neuropathic pain through the inhibition of EphB1 tyrosine kinase, which is a mediator of neuropathic pain and morphine tolerance, and that the antimicrobial peptide piscidin-1 may possess antinociceptive effects in neuropathic pain models [7,8]. Dapsone, which is approved by the United States Food and Drug Administration for leprosy and dermatitis herpetiformis, and is used off-label to treat malaria and Pneumocystis carinii (jirovecii), possesses anti-inflammatory properties and has shown efficacy in clinical studies for various inflammatory arthritides [9]. Since medical advances are often siloed, discoveries in the neuroscience and infectious disease literature may take decades before they are recognized by pain specialists and studied in acute and chronic pain patients.
The relationship between the immune system and the perception of pain is inextricable and complex. If the understanding of this relationship were metaphorically measured on a calendar, science would only just be waking up on New Year’s Day. The primary goal in the second part of this series is to highlight the evidence on the prevention and treatment of chronic pain using antimicrobial therapies, and provide the scaffolding for areas ripe for future investigation.
Several classes of antimicrobial medications (e.g., cephalosporins [10], rapalogues [11]) can confer analgesic effects pertinent to the treatment of chronic pain via the reduction of infectious burden [12,13], or via off-target effects that alleviate neuroinflammation [14,15]. Moreover, although data are sparse and mixed, there is evidence that early treatment of some infections associated with acute pain might prevent or reduce the incidence of subsequent chronic pain. Table 1 summarizes the systematic reviews, meta-analyses, and largest studies published that pertain to acute infection management/acute antimicrobial treatment and the prevention of subsequent chronic pain. The literature that is currently available is limited to postherpetic neuralgia (PHN), post COVID-19 condition/“long Covid,” and rheumatic fever.
PHN is defined as pain persisting more than 90 days after the onset or healing of the vesicular rash associated with varicella-zoster virus reactivation [16]. Numerous peripheral (e.g., ectopic discharges, peripheral sensitization) and central (e.g., glial cell activation, central sensitization) mechanisms are likely involved in the maintenance of PHN [17]. Although the virus is rarely eradicated despite antiviral treatment and primarily exists in a latent state in ganglion cells [18], an ongoing, uncontrolled infection is unlikely to be the cause of PHN symptoms in most individuals.
In a Cochrane systematic review and meta-analysis comprising 6 randomized controlled trials (RCTs) and 1,211 patients [19], acyclovir did not reduce the incidence of PHN at either 4 or 6 months after the onset of acute herpetic rash (based on 609 participants among three trials [20–22] and 476 participants among two trials [22,23], respectively) compared to a placebo. However, a meta-analysis of four trials [20,21,24,25] demonstrated a statistically significant benefit from acyclovir at one month after rash onset. There was insufficient data to complete a subgroup analysis assessing whether earlier administration of acyclovir (< 24 hours of rash onset) might influence outcomes. It is noteworthy that no additional RCTs were identified since the previous iteration of this review in 2009 [26], and although an update was intended for 2017 [19], it has yet to be completed or published.
Post COVID-19 condition, also known by various terms such as “long Covid,” “chronic Covid syndrome,” “long-haul covid,” or “post-acute sequelae of COVID-19” [27], is defined by the World Health Organization (WHO) as “the continuation or development of new symptoms 3 months after the initial SARS-COV-2 infection, with these symptoms lasting for at least 2 months with no other explanation [28].” Other diagnostic criteria are under development and differ in regard to minimum duration of symptoms (e.g., 4 weeks per the National Health Service of the United Kingdom [29] and the Centers for Disease Control and Prevention of the United States [30]). Among individuals with test-confirmed COVID-19 infections, the incidence of post-COVID-19 condition is estimated to range from 13.9%–14.7% (using a definition of ongoing symptoms > 2 months post-infection) [31] to as high as 45% (using a definition of ongoing symptoms at > 28 days post-infection) [27]. “New-onset” chronic pain (pain symptoms arising only subsequent to COVID-19 infection and persisting > 3 months) is one of the most common manifestations of post-COVID-19 condition, with an estimated incidence approaching 20% among COVID-19 survivors [32].
In one retrospective analysis of non-hospitalized patients via a United States Department of Veterans Affairs database [33], 9,217 individuals who were treated with oral nirmatrelvir within five days of a positive COVID-19 test were compared against 47,123 individuals who received no antimicrobial treatment within 30 days of diagnosis. The group that received nirmatrelvir demonstrated a reduced risk of post-acute sequelae of COVID-19 (hazard ratio [HR], 0.74; 95% confidence interval [CI], 0.69–0.81) that appeared to be independent of vaccination status or history of prior infection. This study was published on a preprint server and without peer-review, and these findings therefore require confirmation. One case series reported that among four patients diagnosed with COVID-19 who received nirmatrelvir, the three patients who had prior diagnoses of long Covid reported improvement, whereas the one patient who received nirmatrelvir within 24 hours of acute COVID-19 symptom onset experienced an overall worsening of symptoms [34]. As of March 2023, no additional studies or trials assessing the relationship between nirmatrelvir and post-COVID-19 condition have been published, and there is yet to be an established therapeutic regimen for post-COVID-19 condition [35].
Vaccination might help prevent post-COVID-19 condition among individuals who experience subsequent breakthrough infections, but uncertainties remain. In a recent prospective cohort study of 669 test-confirmed COVID-19 patients, non-vaccination status was associated with an adjusted odds ratio (OR) of 6.9 (95% CI, 4.2–11.3) for developing long Covid symptoms [36]. However, in a large retrospective cohort study of 9,479 vaccinated individuals matched to unvaccinated controls, receiving at least one COVID-19 vaccine dose was not associated with a decreased risk of long Covid, although the incidences of myalgia (HR, 0.78; 95% CI, 0.67–0.91) and pain (HR, 0.90; 95% CI, 0.81–0.99) decreased [37]. A recent, large systematic review by Byambasuren et al. [38] identified 16 observational studies assessing the effect of vaccination on the incidence of long Covid, altogether comprising over 614,000 patients. The authors did not identify any RCTs and could not perform a meta-analysis due to the heterogeneity of the data. Among the included studies, OR values ranged widely and at times approached or exceeded 1.0, and there was no clear pattern regarding whether additional vaccination doses confer greater protection (among individual included studies, the OR with one dose ranged from 0.22 to 1.03; 0.25–1.02 for two doses; 0.48–1.01 for any dose; in one study assessing three doses, the OR was 0.16 [95% CI, 0.03 to 0.85] [39]). Because the included studies varied in their diagnostic criteria (e.g., 28 days of symptoms versus longer durations) and data collection methods (e.g., self-reporting of symptoms versus provider assessment), and all studies were completed prior to the emergence of the omicron variant, the generalizability of these data is uncertain. Additionally, since a meta-analysis could not be performed, the investigators were not able to determine whether different vaccines (e.g., BNT 162b2, mRNA-1273, ChAdOx1 nCoV-1) might confer different rates of protection against post-COVID-19 condition. More studies are necessary to clarify whether one vaccine is superior to another in regard to prevention of post-COVID-19 conditions, and whether the timing of vaccination prior to breakthrough infection and the specific viral variant influences the likelihood of prevention.
Very limited data are available regarding whether the WHO-approved vaccines can prevent or treat post-COVID-19 condition if they are administered after infection has already occurred. In the systematic review by Byambasuren et al. [38], five studies reported data on vaccination administration subsequent to COVID-19 infection, and OR values for subsequent long Covid ranged from 0.38–0.91. However, in a small prospective open-label study in 42 patients diagnosed with long Covid (using a definition of persistent symptoms > 2 months after COVID-19 diagnosis), a single vaccination dose improved symptoms in 16.7% of patients but worsened symptoms in another 21.4% [40]. The patients who reported worsening symptoms had significantly higher levels of serum antibody titers compared to those who reported an improvement of symptoms or no change, suggesting that an excessive immune response might be the cause. It is important to note that the investigators did not report which vaccine or dosage they used, and therefore these findings require additional confirmation.
Rheumatic fever is the result of an autoimmune response to a pharyngeal infection with group A Streptococcus (GAS), most commonly Streptococcus pyogenes, likely mediated via molecular mimicry between streptococcal antigens and endogenous proteins [41]. Polyarthritis and carditis are the most common manifestations of acute rheumatic fever and are considered “major criteria” in the revised Jones criteria for diagnosis [42]. For primary prevention of rheumatic fever, consensus guidelines [43] recommend intramuscular benzathine penicillin G or a course of oral phenoxymethylpenicillin (penicillin V). In those who are at elevated risk of a severe adverse reaction (e.g., a previous history of anaphylaxis from penicillin), a macrolide antibiotic is recommended instead [41]. Unfortunately, despite the high in-vitro susceptibility of GAS to penicillin, GAS is not eradicated in all patients who are treated [43].
In a meta-analysis of 10 RCTs comprising 7,665 patients, antibiotics for the primary prevention of rheumatic fever only had a protective effect of approximately 70%, with a number needed-to-treat (NNT) of 53 [44]. When pooled analyses were restricted to data pertaining to penicillin antibiotics, the protective effect only increased to 80%. Of note, all included studies were completed between 1950–1961 among the young adult male population in the United States military, which might altogether suggest a prima facie lack of generalizability. However, contemporary estimates are similar in numeric value. The American Heart Association estimates that primary penicillin prophylaxis against rheumatic fever is 68% effective [45], and in a more recent meta-analysis of 6 RCTs in the school-age population, the estimated efficacy is approximately 60% [46]. A school-based RCT by Lennon et al. [47] (in which schools were the randomized units) comprising approximately 22,000 students demonstrated a non-significant reduction in rates of rheumatic fever despite implementation of onsite clinics for diagnosis and oral penicillin administration; however, in a subsequent cohort study involving 25,000 students by the same lead investigator in a similar patient population, the implementation of school-based sore throat clinics in addition to oral penicillin reduced the incidence of rheumatic fever by 58% after two years of implementation [48]. The investigators postulated that in their RCT [47], because school-zoning methods allowed for a child to attend a control-group school but a younger or older sibling in the same family to attend an intervention-group school, any protective effects from the intervention might have been diluted by household exposures to GAS [48]. No published studies or pooled analyses have specifically assessed the incidence of pain-related symptoms (e.g., polyarthralgia), but it stands to reason that the antibiotic prevention of rheumatic fever will also confer a protective effect, albeit of an uncertain magnitude.
Infections can cause pain through direct tissue damage and the induction of maladaptive immune responses (e.g., molecular mimicry, epitope spreading), which can lead to peripheral and central sensitization and propagate chronic pain [49]. There is evidence that some antimicrobial therapies provide analgesic effects by reducing known or subclinical infectious burden (e.g., chronic low back pain [50], chronic prostatitis/chronic male pelvic pain [51,52]), or by mitigating (through off-target effects) neuroinflammatory processes that facilitate nociception. Most clinical studies assessing the use of antimicrobials for treating chronic pain symptoms have focused on gastrointestinal conditions.
Table 2 provides a summary of published reviews and meta-analyses and their results, organized by pain condition (e.g., chronic low back pain, chronic prostatitis/pelvic pain syndrome, irritable bowel syndrome (IBS), inflammatory bowel disease (IBD), functional dyspepsia, myalgic encephalomyelitis/chronic fatigue syndrome [ME/CFS]).
IBS is diagnosed through the Rome IV criteria [53], which recognizes that the condition is a functional gastrointestinal disorder [54] resulting from a complex relationship involving microbial dysbiosis, altered immunologic responses, and central nervous dysregulation of gastrointestinal functions [53]. There is considerable evidence that microbial dysbiosis contributes to the pathophysiology of IBS by disrupting epithelial barriers in the gastrointestinal lumen, leading to overactivation of mucosal afferent nerves and immune responses, contributing to altered central nervous system signaling that might further impair bowel function and structural integrity [55].
Despite the likely role of dysbiosis in the etiology and maintenance of IBS, the volume of evidence for the effectiveness of antimicrobials is limited. One meta-analysis of 9 RCTs, comprising over 2,800 patients with IBS, assessed whether antibiotics are superior to a placebo [56]. Rifaximin was used in 7 studies, while neomycin and norfloxacin were each assessed in one study. When the data of four trials with low risk-of-bias were pooled, rifaximin was found to have a modest effect on improving IBS symptoms (risk ratio [RR] = 0.87, 95% CI, 0.82–0.93; NNT = 11, 95% CI, 8–21), and the individual trials of neomycin (RR = 0.73, 95% CI, 0.56–0.96; NNT = 5, 95% CI, 3–33) and norfloxacin (RR = 0.63, 95% CI, 0.49–0.80; NNT = 3, 95% CI, 2–5) were also positive. However, the authors were not able to determine which antibiotic doses or regimens might confer the greatest efficacy.
A recent large meta-analysis assessed the efficacy of pharmacologic therapies for patients with either diarrhea or mixed stool-pattern IBS, comprising 18 RCTs and over 9,800 patients [57]. Among the included studies, 12 RCTs assessed serotonin 5-hydroxytryptamine-3 receptor antagonists (alosetron or ramosetron), four assessed eluxadoline (a mixed μ-opioid receptor agonist, δ-opioid receptor antagonist, and κ-opioid receptor agonist), and only two assessed an antimicrobial (rifaximin). Although rifaximin conferred the lowest risk of adverse effects, including constipation, it did not demonstrate greater efficacy than a placebo for improvement in global IBS symptoms (RR = 0.91, 95% CI, 0.77–1.07) or abdominal pain (RR = 0.95, 95% CI, 0.89–1.01).
Additional studies are necessary to clarify the optimal role of antimicrobials in the management of IBS. Although efforts toward this continue, updated American College of Gastroenterology [58] and American Gastroenterological Association [59] guidelines now recommend the use of rifaximin for IBS with diarrhea on the premise that its potential benefits for the gut microbiome outweigh its few adverse effects.
IBD, which comprises ulcerative colitis and Crohn’s disease, is thought to result from a chronic, dysregulated immune response to intestinal microflora amidst incompletely understood genetic and environmental risk factors [60]. Most treatments for IBD are therefore immunomodulators such as corticosteroids, methotrexate, or azathioprine, or anti-inflammatory medications such as aminosalicylates and anti-tumor necrosis factor monoclonal antibodies [60].
There is limited evidence that exposure to antibiotics within the first year of life might increase the risk for IBD [61], and current guidelines do not recommend the routine use of antibiotics for the management of IBD due to uncertain benefit [62,63]. Nonetheless, antibiotics have been studied for their potential effects on IBD remission and symptom reduction. A recent Cochrane review of 13 RCTs comprising over 1,300 patients with Crohn’s disease assessed whether antibiotics could induce and maintain remission [64]. The authors identified a high heterogeneity of treatment regimens, ranging from those that include ciprofloxacin, rifaximin, metronidazole, cotrimoxazole, or clarithromycin, to those that include various combinations of these antibiotics in addition to corticosteroids, anti-inflammatory medications, or monoclonal antibodies. When data pertaining to all antibiotic therapies were pooled, patients who had received antibiotics had a significantly lower rate of remission failure compared to those who had received placebo, within 10 weeks (RR = 0.86, 95% CI, 0.76 to 0.98) as well as 14 weeks (RR = 0.77, 95% CI, 0.64 to 0.93). However, antibiotics did not appear to reduce rates of relapse within 1 year compared to a placebo (RR = 0.87, 95% CI, 0.52 to 1.47). In a small systematic review [65] of 15 studies in patients with IBD, one comparative-effectiveness study in 29 patients [66] found that a 10-day course of either metronidazole or ciprofloxacin reduced abdominal symptoms (e.g., bloating, pain) without a difference in efficacy between antibiotics.
Dyspepsia describes symptoms characteristic of upper gastrointestinal disorders, such as postprandial fullness, early satiety, and epigastric pain, whereas functional dyspepsia is the term used to describe these symptoms when they are chronic and occur without an established etiology [67]. Although the precise mechanisms have yet to be established, functional dyspepsia is likely the confluence of a disordered gut-brain axis, alterations in gastrointestinal sensorimotor function, gut dysbiosis, infection, gastrointestinal mucosal inflammation, and excessive immune activity [68].
Because chronic subclinical infections or microbiome alterations are putative factors in the development and maintenance of functional dyspepsia, antimicrobial therapies have been attractive targets for research. The most studied antimicrobial treatment for dyspepsia involves Helicobacter pylori eradication. A meta-analysis [69] of 25 studies comprising 5,555 patients with functional dyspepsia and known H. pylori demonstrated a statistically significant improvement in dyspepsia symptoms after antibiotic treatment (RR = 1.23; 95% CI, 1.12–1.36, P < 0.0001), but only after long-term (≥ 1 year) follow-up and not at earlier time points. There was no significant improvement in quality of life after H. pylori eradication, and side effects secondary to antibiotics were more common (RR = 2.02; 95% CI, 1.12–3.65, P = 0.02). However, histologic resolution of chronic gastritis was more likely with H. pylori eradication (RR = 7.13; 95% CI, 3.68–13.81, P < 0.00001), as well as the prevention of peptic ulcer disease (RR = 0.35; 95% CI, 0.18–0.68, P = 0.002), which might explain the delayed improvement in functional dyspepsia symptoms.
A meta-analysis [70] of 18 RCTs assessed the effects of H. pylori eradication on functional dyspepsia symptoms, with subgroup analyses performed according to local prevalence and geographical regions. The authors found that the eradication of H. pylori significantly improved dyspepsia symptoms (RR = 1.18; 95% CI, 1.07–1.30, P < 0.01) regardless of local prevalence, though in the subgroup analysis of RCTs conducted in Asia, there was no significant benefit (RR = 1.14; 95% CI, 0.99–1.33, P = 0.08). The authors postulated that differences in trial design (e.g., shorter duration of treatment regimens), smaller sample sizes, and conflicting results between the individual RCTs completed in Asia, perhaps due to significant heterogeneity, might have led to non-significant results in the subgroup analysis, which should be interpreted with caution [70].
A recent large meta-analysis [71] of 29 RCTs comprising 6,781 patients with functional dyspepsia and H. pylori infection assessed the efficacy of eradication therapy on symptoms. Eradication therapy was more likely than non-antibiotic therapies to result in symptom resolution (RR of non-cure = 0.91, 95% CI, 0.89 to 0.94; NNT = 14, 95% CI, 11 to 21) or symptomatic improvement (RR of no improvement = 0.84, 95% CI, 0.78 to 0.91; NNT = 9, 95% CI, 7 to 17). Subgroup analyses did not show a significant difference in benefit between different antibiotic regimens, but eradication therapies were associated with a higher risk of adverse events (RR = 2.19, 95% CI, 1.10 to 4.37; number needed to harm = 3, 95% CI, 1 to 40). Notably, in contrast to the findings of Kang et al. [70], the effect size of H. pylori eradication was greater in Asian studies (RR = 0.82, 95% CI, 0.73 to 0.91; NNT = 8, 95% CI, 5 to 15, P < 0.001) than in non-Asian studies (RR = 0.92, 95% CI, 0.89 to 0.95; NNT = 16, 95% CI, 12 to 25, P = 0.32), with a statistically significant between-group difference (P value for X2 = 0.04). The authors, overall, concluded that there is high-quality evidence supporting H. pylori eradication therapy in functional dyspepsia. They were unable to determine whether these benefits are directly due to the reduction of infectious burden, or via indirect effects on gastrointestinal dysbiosis, although the treatment effect size was larger in patients who had eradication of H. pylori (RR of non-cure = 0.74, 95% CI, 0.64 to 0.85; NNT = 6, 95% CI, 4 to 10).
Although antimicrobial therapies are recommended when patients with functional dyspepsia have known H. pylori infection [72], increasing rates of antibiotic resistance are a significant concern. Furthermore, the precise role of H. pylori in functional dyspepsia remains controversial [73], which has led some consensus guidelines to propose that a functional gastrointestinal diagnosis precludes a known or putative infection [74]. Because most of the existing literature pertaining to antimicrobial treatments for functional dyspepsia included only patients with H. pylori infection, it remains unknown whether antibiotics might also benefit patients with dyspepsia but no known infection. Additionally, there is a need for further clarification of the diagnostic definition of functional dyspepsia before the potential role of antibiotic therapies can be established.
ME, or CFS, is a multisystem disorder affecting the nervous, autonomic, immune, and endocrine systems [75,76]. Symptoms include cognitive dysfunction, sensorimotor deficits, exertional-induced fatigue, temperature dysregulation, orthostatic hypotonia and hypotension, sleep disturbance, and widespread pain [75]. Although the etiology of ME/CFS is unclear, a variety of pathogens such as Epstein–Barr virus, enterovirus, hepatitis C, cytomegalovirus, West Nile virus, Borrelia burgdorferi, human herpesvirus-6, parvovirus B-19, Chlamydia pneumoniae, and Coxiella burnetii have been implicated, and pathophysiologic similarities to “long Covid” described [77–80]. Gut dysbiosis is one proposed mechanism for the development of ME/CFS [81]. Because some antibiotics reduce bacterial diversity and facilitate dysbiosis while others improve microbiota composition [82], it is important to recognize that an antimicrobial regimen can be both a risk factor [81] or potential treatment [83] for ME/CFS depending on its overall impact on gut microbiota [82].
A recent systematic review [83] evaluating treatments for ME/CFS included nine placebo-controlled trials assessing pharmacologic therapies, of which four studies [84–87] utilized antimicrobial therapies. Two of these studies [84,85] were multicenter placebo-controlled trials assessing rintatolimod (Poly I:C12U), which is an intravenous immune modulator and antiviral drug. One study [84] in 92 patients demonstrated statistically significant improvements in Karnofsky Performance Scale Score (P < 0.03), exercise treadmill testing performance (P < 0.01), activities of daily living (P < 0.04), cognition (P = 0.05), and decreased symptom-relieving medication use (P < 0.05); the other study [85] evaluated 234 patients at baseline and at 40 weeks after treatment, demonstrating an improvement in exercise tolerance compared to baseline (21.3% increase, P = 0.047) and a reduced dependence on symptom-alleviating medications (P = 0.048). However, neither study reported measures directly pertaining to pain severity or magnitude of analgesia. In a small, placebo-controlled trial [86] evaluating valganciclovir in 30 patients with CFS and elevated human herpesvirus-6 and Epstein–Barr virus titers, improvements were seen in Multidimensional Fatigue Inventory-20 mental fatigue subscores (P = 0.039), Fatigue Severity Scale scores (P = 0.006), and cognition (P = 0.025); however, the study was underpowered, and no pain-specific outcomes were reported. In another small, placebo-controlled trial in 30 patients with CFS [87], intravenous immunoglobulin G was administered every 30 days for six months. Among the 28 patients who completed the trial, no significant benefit in symptoms or functional status was found.
Notably, in a 1988 placebo-controlled RCT assessing the efficacy of acyclovir in 27 patients with CFS [88], as well as in a 2012 unblinded retrospective chart review assessing the efficacy of valganciclovir in 61 patients with CFS [89], improvements in mood, cognition, or physical function were reported, but these were not correlated with titers of antibodies to suspected causative pathogens (e.g., Epstein–Barr virus) [88,89]. This raises the possibility that these improvements were due to placebo effect, or other factors independent of the antiviral medications [88]. In summary, while antimicrobials may have a role in the treatment of ME/CFS, the available evidence remains limited. There is a need for future studies that specifically assess pain and analgesia, and ideally correlate improvements to objective markers of pathogen reduction.
Since pain is a hallmark of infection and chronic pain is so prevalent, studies evaluating antimicrobial therapy may seek to determine whether the agents disproportionately reduce acute pain as the infection resolves, or serendipitously reduce non-infectious disease-related pain conditions (e.g., arthritis, spine pain). Given the risks associated with indiscriminate antimicrobial use, investigations on the use of antimicrobial drugs for chronic pain should seek to identify biomarkers or phenotypic characteristics that predict response to therapy, which could improve risk-benefit and cost-effectiveness ratios. As noted in the first article on analgesic mechanisms of antimicrobial therapies, indirect-acting drugs that target phages themselves or phage-derived peptides, virulence factors; antibiotic-drug conjugates; microbiome-modulating treatments, immunomodulators; drug potentiators and a variety of other unconventional targets [90] should be evaluated for possible antimicrobial effects, with research on microbiome-transforming treatments being particularly promising. Finally, given the mechanisms of action of many antimicrobial agents that include targeting the genome, immune mechanisms, and inflammatory cascade, studies evaluating the effect of antimicrobial agents on chronic pain should aim to determine whether these medications possess disease-modifying properties (e.g., by correlating pain relief with biomarkers and imaging). Fig. 1 illustrates interrelated patient-specific, antimicrobial-specific, and disease-specific factors that contribute to the perception of pain and require further study.
The primary therapeutic intent of antimicrobials is to eliminate their target pathogens before the host succumbs to infection and the infectious agents develop mechanisms of resistance. Several classes of antimicrobials have been observed to provide pain relief as a secondary benefit, and clinical studies have utilized them in a variety of painful disease states to assess their analgesic efficacy.
Although the available evidence is limited, antimicrobial agents have demonstrated analgesic effects in conditions that involve dysbiosis or potential subclinical infection (e.g., low back pain with Modic type 1 changes, functional gastrointestinal disorders), and might help prevent subsequent chronic pain from acute infections associated with excessive systemic inflammation (e.g., post COVID-19 condition/“long Covid,” rheumatic fever). Significant questions remain regarding whether antimicrobial dosage or timing of administration influences the potential magnitude of analgesia, as well as which patient-specific factors or co-morbidities might also be relevant.
Owing to concerns regarding antibiotic resistance, antimicrobials must be used judiciously and are unlikely to be repurposed as primary analgesic medications. However, when clinical equipoise exists among several treatment options, the potential analgesic benefit of certain antimicrobial agents might be valuable to consider.
Notes
REFERENCES
1. Hyson JM Jr. 2007; A history of arsenic in dentistry. J Calif Dent Assoc. 35:135–9. DOI: 10.1080/19424396.2007.12221210. PMID: 17494382.
2. Zhao M, Li Y, Wang Z. 2022; Mercury and mercury-containing preparations: history of use, clinical applications, pharmacology, toxicology, and pharmacokinetics in traditional Chinese medicine. Front Pharmacol. 13:807807. DOI: 10.3389/fphar.2022.807807. PMID: 35308204. PMCID: PMC8924441. PMID: b5d73bd0444543d3b1657c57ccffd9e5.


3. Parapia LA. 2008; History of bloodletting by phlebotomy. Br J Haematol. 143:490–5. DOI: 10.1111/j.1365-2141.2008.07361.x. PMID: 18783398.


4. Sun L, Lutz BM, Tao YX. Preedy VR, editor. 2016. Chapter 48 - contribution of spinal cord mTORC1 to chronic opioid tolerance and hyperalgesia. Neuropathology of drug addictions and substance misuse. Academic Press;p. 482–9. DOI: 10.1016/B978-0-12-800634-4.00048-2.
5. Yeo JH, Kim SJ, Roh DH. 2021; Rapamycin reduces orofacial nociceptive responses and microglial p38 mitogen-activated protein kinase phosphorylation in trigeminal nucleus caudalis in mouse orofacial formalin model. Korean J Physiol Pharmacol. 25:365–74. DOI: 10.4196/kjpp.2021.25.4.365. PMID: 34187953. PMCID: PMC8255123.


6. Feng T, Yin Q, Weng ZL, Zhang JC, Wang KF, Yuan SY, et al. 2014; Rapamycin ameliorates neuropathic pain by activating autophagy and inhibiting interleukin-1β in the rat spinal cord. J Huazhong Univ Sci Technolog Med Sci. 34:830–7. DOI: 10.1007/s11596-014-1361-6. PMID: 25480578.


7. Ahmed MS, Wang P, Nguyen NUN, Nakada Y, Menendez-Montes I, Ismail M, et al. 2021; Identification of tetracycline combinations as EphB1 tyrosine kinase inhibitors for treatment of neuropathic pain. Proc Natl Acad Sci U S A. 118:e2016265118. DOI: 10.1073/pnas.2016265118. PMID: 33627480. PMCID: PMC7958374.


8. Chen WF, Huang SY, Liao CY, Sung CS, Chen JY, Wen ZH. 2015; The use of the antimicrobial peptide piscidin (PCD)-1 as a novel anti-nociceptive agent. Biomaterials. 53:1–11. DOI: 10.1016/j.biomaterials.2015.02.069. PMID: 25890701.


9. Chang DJ, Lamothe M, Stevens RM, Sigal LH. 1996; Dapsone in rheumatoid arthritis. Semin Arthritis Rheum. 25:390–403. DOI: 10.1016/S0049-0172(96)80004-7. PMID: 8792511.


10. Hajhashemi V, Hosseinzadeh H, Amin B. 2013; Antiallodynia and antihyperalgesia effects of ceftriaxone in treatment of chronic neuropathic pain in rats. Acta Neuropsychiatr. 25:27–32. DOI: 10.1111/j.1601-5215.2012.00656.x. PMID: 26953071.


11. Abdelaziz DM, Stone LS, Komarova SV. 2014; Osteolysis and pain due to experimental bone metastases are improved by treatment with rapamycin. Breast Cancer Res Treat. 143:227–37. DOI: 10.1007/s10549-013-2799-0. PMID: 24327332.


12. Albert HB, Sorensen JS, Christensen BS, Manniche C. 2013; Antibiotic treatment in patients with chronic low back pain and vertebral bone edema (Modic type 1 changes): a double-blind randomized clinical controlled trial of efficacy. Eur Spine J. 22:697–707. DOI: 10.1007/s00586-013-2675-y. PMID: 23404353. PMCID: PMC3631045.


13. Bråten LCH, Rolfsen MP, Espeland A, Wigemyr M, Aßmus J, Froholdt A, et al. AIM study group. 2019; Efficacy of antibiotic treatment in patients with chronic low back pain and Modic changes (the AIM study): double blind, randomised, placebo controlled, multicentre trial. BMJ. 367:l5654. DOI: 10.1136/bmj.l5654. PMID: 31619437. PMCID: PMC6812614.


14. Haight ES, Johnson EM, Carroll IR, Tawfik VL. 2020; Of mice, microglia, and (wo)men: a case series and mechanistic investigation of hydroxychloroquine for complex regional pain syndrome. Pain Rep. 5:e841. DOI: 10.1097/PR9.0000000000000841. PMID: 33490839. PMCID: PMC7808678. PMID: ed8454a3c3f045f58bf6a1e1ac2d8feb.


15. Zhou YQ, Liu DQ, Chen SP, Sun J, Wang XM, Tian YK, et al. 2018; Minocycline as a promising therapeutic strategy for chronic pain. Pharmacol Res. 134:305–10. DOI: 10.1016/j.phrs.2018.07.002. PMID: 30042091.


16. Cohen JI. 2013; Clinical practice: herpes zoster. N Engl J Med. 369:255–63. DOI: 10.1056/NEJMcp1302674. PMID: 23863052. PMCID: PMC4789101.
17. Devor M. 2018; Rethinking the causes of pain in herpes zoster and postherpetic neuralgia: the ectopic pacemaker hypothesis. Pain Rep. 3:e702. DOI: 10.1097/PR9.0000000000000702. PMID: 30706041. PMCID: PMC6344138. PMID: aff9ce7f303c46a4bc303e6f89988266.


18. Sauerbrei A. 2016; Diagnosis, antiviral therapy, and prophylaxis of varicella-zoster virus infections. Eur J Clin Microbiol Infect Dis. 35:723–34. DOI: 10.1007/s10096-016-2605-0. PMID: 26873382.


19. Chen N, Li Q, Yang J, Zhou M, Zhou D, He L. 2014; Antiviral treatment for preventing postherpetic neuralgia. Cochrane Database Syst Rev. 2:CD006866. DOI: 10.1002/14651858.CD006866.pub3.


20. Huff JC, Bean B, Balfour HH Jr, Laskin OL, Connor JD, Corey L, et al. 1988; Therapy of herpes zoster with oral acyclovir. Am J Med. 85:84–9. PMID: 3044099.
21. Harding SP, Porter SM. 1991; Oral acyclovir in herpes zoster ophthalmicus. Curr Eye Res. 10 Suppl:177–82. DOI: 10.3109/02713689109020376. PMID: 1864092.


22. Wood MJ, Johnson RW, McKendrick MW, Taylor J, Mandal BK, Crooks J. 1994; A randomized trial of acyclovir for 7 days or 21 days with and without prednisolone for treatment of acute herpes zoster. N Engl J Med. 330:896–900. DOI: 10.1056/NEJM199403313301304. PMID: 8114860.


23. Whitley RJ, Weiss H, Gnann JW Jr, Tyring S, Mertz GJ, Pappas PG, et al. 1996; Acyclovir with and without prednisone for the treatment of herpes zoster. A randomized, placebo-controlled trial. The National Institute of Allergy and Infectious Diseases Collaborative Antiviral Study Group. Ann Intern Med. 125:376–83. DOI: 10.7326/0003-4819-125-5-199609010-00004. PMID: 8702088.


24. Wood MJ, Ogan PH, McKendrick MW, Care CD, McGill JI, Webb EM. 1988; Efficacy of oral acyclovir treatment of acute herpes zoster. Am J Med. 85:79–83. PMID: 3044098.
25. Morton P, Thomson AN. 1989; Oral acyclovir in the treatment of herpes zoster in general practice. N Z Med J. 102:93–5. PMID: 2648213.
26. Li Q, Chen N, Yang J, Zhou M, Zhou D, Zhang Q, et al. 2009; Antiviral treatment for preventing postherpetic neuralgia. Cochrane Database Syst Rev. 2:CD006866. DOI: 10.1002/14651858.CD006866.pub2.


27. O'Mahoney LL, Routen A, Gillies C, Ekezie W, Welford A, Zhang A, et al. 2022; The prevalence and long-term health effects of Long Covid among hospitalised and non-hospitalised populations: a systematic review and meta-analysis. EClinicalMedicine. 55:101762. Erratum in: EClinicalMedicine 2023; 59: 101959. DOI: 10.1016/j.eclinm.2023.101959. PMID: 37096187. PMCID: PMC10115131.
28. World Health Organization. 2022. Post COVID-19 condition (Long COVID) [Internet]. World Health Organization;Geneva: https://www.who.int/europe/news-room/fact-sheets/item/post-covid-19-condition.
29. NHS inform. 2022. What is long COVID? [Internet]. NHS inform;https://www.nhsinform.scot/long-term-effects-of-covid-19-long-covid/about-long-covid/what-is-long-covid.
30. COVID.gov. 2023. COVID.gov/longcovid - Virus that causes COVID-19 can experience long-term effects from their infection [Internet]. U.S. Department of Health and Human Services;Washington, D.C.: https://www.covid.gov/longcovid.
31. Perlis RH, Santillana M, Ognyanova K, Safarpour A, Lunz Trujillo K, Simonson MD, et al. 2022; Prevalence and correlates of long COVID symptoms among US adults. JAMA Netw Open. 5:e2238804. DOI: 10.1001/jamanetworkopen.2022.38804. PMID: 36301542. PMCID: PMC9614581.


32. Soares FHC, Kubota GT, Fernandes AM, Hojo B, Couras C, Costa BV, et al. "Pain in the Pandemic Initiative Collaborators". 2021; Prevalence and characteristics of new-onset pain in COVID-19 survivours, a controlled study. Eur J Pain. 25:1342–54. DOI: 10.1002/ejp.1755. PMID: 33619793. PMCID: PMC8013219.


33. Xie Y, Choi T, Al-Aly Z. 2022; Nirmatrelvir and the risk of post-acute sequelae of COVID-19. medRxiv [Preprint]. Available at: https://www.medrxiv.org/content/10.1101/2022.11.03.22281783v1.abstract. cited 2023 Feb 11. DOI: 10.1101/2022.11.03.22281783.


34. Peluso MJ, Anglin K, Durstenfeld MS, Martin JN, Kelly JD, Hsue PY, et al. 2022; Effect of oral nirmatrelvir on long COVID symptoms: 4 cases and rationale for systematic studies. Pathog Immun. 7:95–103. DOI: 10.20411/pai.v7i1.518. PMID: 35800257. PMCID: PMC9254867. PMID: 7168969bc6034d9cb6df09a3d96f3fc5.
35. Davis HE, McCorkell L, Vogel JM, Topol EJ. 2023; Long COVID: major findings, mechanisms and recommendations. Nat Rev Microbiol. 21:133–46. Erratum in: Nat Rev Microbiol 2023; 21: 408. DOI: 10.1038/s41579-022-00846-2. PMID: 36639608. PMCID: PMC9839201.


36. Abu Hamdh B, Nazzal Z. 2023; A prospective cohort study assessing the relationship between long-COVID symptom incidence in COVID-19 patients and COVID-19 vaccination. Sci Rep. 13:4896. DOI: 10.1038/s41598-023-30583-2. PMID: 36966161. PMCID: PMC10039348. PMID: 6cda5ddc6be044819cfd25c682fb9f77.


37. Taquet M, Dercon Q, Harrison PJ. 2022; Six-month sequelae of post-vaccination SARS-CoV-2 infection: a retrospective cohort study of 10,024 breakthrough infections. Brain Behav Immun. 103:154–62. DOI: 10.1016/j.bbi.2022.04.013. PMID: 35447302. PMCID: PMC9013695.


38. Byambasuren O, Stehlik P, Clark J, Alcorn K, Glasziou P. 2023; Effect of covid-19 vaccination on long covid: systematic review. BMJ Med. 2:e000385. DOI: 10.1136/bmjmed-2022-000385. PMID: 36936268. PMCID: PMC9978692.


39. Azzolini E, Levi R, Sarti R, Pozzi C, Mollura M, Mantovani A, et al. 2022; Association between BNT162b2 vaccination and long COVID after infections not requiring hospitalization in health care workers. JAMA. 328:676–8. DOI: 10.1001/jama.2022.11691. PMID: 35796131. PMCID: PMC9250078.


40. Tsuchida T, Hirose M, Inoue Y, Kunishima H, Otsubo T, Matsuda T. 2022; Relationship between changes in symptoms and antibody titers after a single vaccination in patients with Long COVID. J Med Virol. 94:3416–20. DOI: 10.1002/jmv.27689. PMID: 35238053. PMCID: PMC9088489.


41. Karthikeyan G, Guilherme L. 2018; Acute rheumatic fever. Lancet. 392:161–74. Erratum in: Lancet 2018; 392: 820. DOI: 10.1016/S0140-6736(18)30999-1. PMID: 30025809.


42. Gewitz MH, Baltimore RS, Tani LY, Sable CA, Shulman ST, et al. Carapetis J; American Heart Association Committee on Rheumatic Fever. Endocarditis. and Kawasaki Disease of the Council on Cardiovascular Disease in the Young. 2015; Revision of the Jones Criteria for the diagnosis of acute rheumatic fever in the era of Doppler echocardiography: a scientific statement from the American Heart Association. Circulation. 131:1806–18. Erratum in: Circulation 2020; 142: e65. DOI: 10.1161/CIR.0000000000000205. PMID: 25908771.


43. Gerber MA, Baltimore RS, Eaton CB, Gewitz M, Rowley AH, Shulman ST, et al. 2009; Prevention of rheumatic fever and diagnosis and treatment of acute Streptococcal pharyngitis: a scientific statement from the American Heart Association Rheumatic Fever, Endocarditis, and Kawasaki Disease Committee of the Council on Cardiovascular Disease in the Young, the Interdisciplinary Council on Functional Genomics and Translational Biology, and the Interdisciplinary Council on Quality of Care and Outcomes Research: endorsed by the American Academy of Pediatrics. Circulation. 119:1541–51. DOI: 10.1161/CIRCULATIONAHA.109.191959. PMID: 19246689.


44. Robertson KA, Volmink JA, Mayosi BM. 2005; Antibiotics for the primary prevention of acute rheumatic fever: a meta-analysis. BMC Cardiovasc Disord. 5:11. DOI: 10.1186/1471-2261-5-11. PMID: 15927077. PMCID: PMC1164408. PMID: cbce5f75b5e84f1dba5b138fd3f5e1ca.


45. Kumar RK, Antunes MJ, Beaton A, Mirabel M, Nkomo VT, et al. Okello E; American Heart Association Council on Lifelong Congenital Heart Disease and Heart Health in the Young; Council on Cardiovascular and Stroke Nursing; and Council on Clinical Cardiology. 2020; Contemporary diagnosis and management of rheumatic heart disease: implications for closing the gap: a scientific statement from the American Heart Association. Circulation. 142:e337–57. Erratum in: Circulation 2021; 143: e1025-6. DOI: 10.1161/CIR.0000000000000921. PMCID: PMC7578108.


46. Lennon D, Kerdemelidis M, Arroll B. 2009; Meta-analysis of trials of streptococcal throat treatment programs to prevent rheumatic fever. Pediatr Infect Dis J. 28:e259–64. DOI: 10.1097/INF.0b013e3181a8e12a. PMID: 19561421.


47. Lennon D, Stewart J, Farrell E, Palmer A, Mason H. 2009; School-based prevention of acute rheumatic fever: a group randomized trial in New Zealand. Pediatr Infect Dis J. 28:787–94. DOI: 10.1097/INF.0b013e3181a282be. PMID: 19710585.
48. Lennon D, Anderson P, Kerdemilidis M, Farrell E, Crengle Mahi S, Percival T, et al. 2017; First presentation acute rheumatic fever is preventable in a community setting: a school-based intervention. Pediatr Infect Dis J. 36:1113–8. DOI: 10.1097/INF.0000000000001581. PMID: 28230706.
49. Cohen SP, Wang EJ, Doshi TL, Vase L, Cawcutt KA, Tontisirin N. 2022; Chronic pain and infection: mechanisms, causes, conditions, treatments, and controversies. BMJ Med. 1:e000108. DOI: 10.1136/bmjmed-2021-000108. PMID: 36936554. PMCID: PMC10012866.


50. Gilligan CJ, Cohen SP, Fischetti VA, Hirsch JA, Czaplewski LG. 2021; Chronic low back pain, bacterial infection and treatment with antibiotics. Spine J. 21:903–14. DOI: 10.1016/j.spinee.2021.02.013. PMID: 33610802.


51. Anothaisintawee T, Attia J, Nickel JC, Thammakraisorn S, Numthavaj P, McEvoy M, et al. 2011; Management of chronic prostatitis/chronic pelvic pain syndrome: a systematic review and network meta-analysis. JAMA. 305:78–86. DOI: 10.1001/jama.2010.1913. PMID: 21205969.


52. Franco JV, Turk T, Jung JH, Xiao YT, Iakhno S, Tirapegui FI, et al. 2019; Pharmacological interventions for treating chronic prostatitis/chronic pelvic pain syndrome. Cochrane Database Syst Rev. 10:CD012552. DOI: 10.1002/14651858.CD012552.pub2. PMID: 31587256.


53. Drossman DA, Hasler WL. 2016; Rome IV-functional GI disorders: disorders of gut-brain interaction. Gastroenterology. 150:1257–61. DOI: 10.1053/j.gastro.2016.03.035. PMID: 27147121.


54. Palsson OS, Whitehead WE, van Tilburg MA, Chang L, Chey W, Crowell MD, et al. 2016; Rome IV diagnostic questionnaires and tables for investigators and clinicians. Gastroenterology. S0016-5085(16)00180-3. DOI: 10.1053/j.gastro.2016.02.014. PMID: 27144634.
55. Barbara G, Feinle-Bisset C, Ghoshal UC, Quigley EM, Santos J, Vanner S, et al. 2016; The intestinal microenvironment and functional gastrointestinal disorders. Gastroenterology. S0016-5085(16)00219-5. DOI: 10.1053/j.gastro.2016.02.028. PMID: 27144620.


56. Ford AC, Harris LA, Lacy BE, Quigley EMM, Moayyedi P. 2018; Systematic review with meta-analysis: the efficacy of prebiotics, probiotics, synbiotics and antibiotics in irritable bowel syndrome. Aliment Pharmacol Ther. 48:1044–60. DOI: 10.1111/apt.15001. PMID: 30294792.


57. Black CJ, Burr NE, Camilleri M, Earnest DL, Quigley EM, Moayyedi P, et al. 2020; Efficacy of pharmacological therapies in patients with IBS with diarrhoea or mixed stool pattern: systematic review and network meta-analysis. Gut. 69:74–82. DOI: 10.1136/gutjnl-2018-318160. PMID: 30996042.


58. Lacy BE, Pimentel M, Brenner DM, Chey WD, Keefer LA, Long MD, et al. 2021; ACG clinical guideline: management of irritable bowel syndrome. Am J Gastroenterol. 116:17–44. DOI: 10.14309/ajg.0000000000001036. PMID: 33315591.


59. Lembo A, Sultan S, Chang L, Heidelbaugh JJ, Smalley W, Verne GN. 2022; AGA clinical practice guideline on the pharmacological management of irritable bowel syndrome with diarrhea. Gastroenterology. 163:137–51. DOI: 10.1053/j.gastro.2022.04.017. PMID: 35738725.


60. Rosen MJ, Dhawan A, Saeed SA. 2015; Inflammatory bowel disease in children and adolescents. JAMA Pediatr. 169:1053–60. DOI: 10.1001/jamapediatrics.2015.1982. PMID: 26414706. PMCID: PMC4702263.


61. Shaw SY, Blanchard JF, Bernstein CN. 2010; Association between the use of antibiotics in the first year of life and pediatric inflammatory bowel disease. Am J Gastroenterol. 105:2687–92. DOI: 10.1038/ajg.2010.398. PMID: 20940708.


62. Gomollón F, Dignass A, Annese V, Tilg H, Van Assche G, et al. Lindsay JO; ECCO. 3rd European evidence-based consensus on the diagnosis and management of Crohn'. s disease 2016:. Part. DOI: 10.1093/ecco-jcc/jjw168. PMID: 27660341.
63. Singh S, Allegretti JR, Siddique SM, Terdiman JP. 2020; AGA technical review on the management of moderate to severe ulcerative colitis. Gastroenterology. 158:1465–96.e17. DOI: 10.1053/j.gastro.2020.01.007. PMID: 31945351. PMCID: PMC7117094.


64. Townsend CM, Parker CE, MacDonald JK, Nguyen TM, Jairath V, Feagan BG, et al. 2019; Antibiotics for induction and maintenance of remission in Crohn's disease. Cochrane Database Syst Rev. 2:CD012730. DOI: 10.1002/14651858.CD012730.pub2. PMID: 30731030. PMCID: PMC6366891.


65. Norton C, Czuber-Dochan W, Artom M, Sweeney L, Hart A. 2017; Systematic review: interventions for abdominal pain management in inflammatory bowel disease. Aliment Pharmacol Ther. 46:115–25. DOI: 10.1111/apt.14108. PMID: 28470846.


66. Castiglione F, Rispo A, Di Girolamo E, Cozzolino A, Manguso F, Grassia R, et al. 2003; Antibiotic treatment of small bowel bacterial overgrowth in patients with Crohn's disease. Aliment Pharmacol Ther. 18:1107–12. DOI: 10.1046/j.1365-2036.2003.01800.x. PMID: 14653830.


67. Oustamanolakis P, Tack J. 2012; Dyspepsia: organic versus functional. J Clin Gastroenterol. 46:175–90. DOI: 10.1097/MCG.0b013e318241b335. PMID: 22327302.
68. Ford AC, Mahadeva S, Carbone MF, Lacy BE, Talley NJ. 2020; Functional dyspepsia. Lancet. 396:1689–702. DOI: 10.1016/S0140-6736(20)30469-4. PMID: 33049222.


69. Du LJ, Chen BR, Kim JJ, Kim S, Shen JH, Dai N. 2016; Helicobacter pylori eradication therapy for functional dyspepsia: systematic review and meta-analysis. World J Gastroenterol. 22:3486–95. DOI: 10.3748/wjg.v22.i12.3486. PMID: 27022230. PMCID: PMC4806206.
70. Kang SJ, Park B, Shin CM. 2019; Helicobacter pylori eradication therapy for functional dyspepsia: a meta-analysis by region and H. pylori prevalence. J Clin Med. 8:1324. DOI: 10.3390/jcm8091324. PMID: 31466299. PMCID: PMC6780123. PMID: 72226e181a0c4134944ac766010870fe.


71. Ford AC, Tsipotis E, Yuan Y, Leontiadis GI, Moayyedi P. 2022; Efficacy of Helicobacter pylori eradication therapy for functional dyspepsia: updated systematic review and meta-analysis. Gut. gutjnl-2021-326583. DOI: 10.1136/gutjnl-2021-326583. PMID: 35022266.
72. Malfertheiner P, Megraud F, O'Morain CA, Gisbert JP, Kuipers EJ, Axon AT, et al. European Helicobacter and Microbiota Study Group and Consensus panel. 2017; Management of Helicobacter pylori infection-the Maastricht V/Florence Consensus Report. Gut. 66:6–30. DOI: 10.1136/gutjnl-2016-312288. PMID: 27707777.


73. Suzuki H, Nishizawa T, Hibi T. 2011; Can Helicobacter pylori-associated dyspepsia be categorized as functional dyspepsia? J Gastroenterol Hepatol. 26 Suppl 3:42–5. DOI: 10.1111/j.1440-1746.2011.06629.x. PMID: 21443708.


74. Sugano K, Tack J, Kuipers EJ, Graham DY, El-Omar EM, Miura S, et al. faculty members of Kyoto Global Consensus Conference. 2015; Kyoto global consensus report on Helicobacter pylori gastritis. Gut. 64:1353–67. DOI: 10.1136/gutjnl-2015-309252. PMID: 26187502. PMCID: PMC4552923.


75. Carruthers BM, van de Sande MI, De Meirleir KL, Klimas NG, Broderick G, Mitchell T, et al. 2011; Myalgic encephalomyelitis: International Consensus Criteria. J Intern Med. 270:327–38. Erratum in: J Intern Med 2017; 282: 353. DOI: 10.1111/joim.12658. PMID: 28929634. PMCID: PMC6885980.


76. Bateman L, Bested AC, Bonilla HF, Chheda BV, Chu L, Curtin JM, et al. 2021; Myalgic encephalomyelitis/chronic fatigue syndrome: essentials of diagnosis and management. Mayo Clin Proc. 96:2861–78. DOI: 10.1016/j.mayocp.2021.07.004. PMID: 34454716.


77. Rasa S, Nora-Krukle Z, Henning N, Eliassen E, Shikova E, Harrer T, et al. European Network on ME/CFS (EUROMENE). 2018; Chronic viral infections in myalgic encephalomyelitis/chronic fatigue syndrome (ME/CFS). J Transl Med. 16:268. DOI: 10.1186/s12967-018-1644-y. PMID: 30285773. PMCID: PMC6167797. PMID: 0101f485cef745a7a69cfca3a3b3013e.


78. Wong TL, Weitzer DJ. 2021; Long COVID and myalgic encephalomyelitis/chronic fatigue syndrome (ME/CFS)-A systemic review and comparison of clinical presentation and symptomatology. Medicina (Kaunas). 57:418. DOI: 10.3390/medicina57050418. PMID: 33925784. PMCID: PMC8145228. PMID: 82ebe8ecc2d24e7792819b2a712e679c.


79. Komaroff AL, Lipkin WI. 2021; Insights from myalgic encephalomyelitis/chronic fatigue syndrome may help unravel the pathogenesis of postacute COVID-19 syndrome. Trends Mol Med. 27:895–906. DOI: 10.1016/j.molmed.2021.06.002. PMID: 34175230. PMCID: PMC8180841.


80. Komaroff AL, Bateman L. 2021; Will COVID-19 lead to myalgic encephalomyelitis/chronic fatigue syndrome? Front Med (Lausanne). 7:606824. DOI: 10.3389/fmed.2020.606824. PMID: 33537329. PMCID: PMC7848220. PMID: eb40d4b0e1e942b9b4c07c1cbe42bf32.


81. König RS, Albrich WC, Kahlert CR, Bahr LS, Löber U, Vernazza P, et al. 2022; The gut microbiome in myalgic encephalomyelitis (ME)/chronic fatigue syndrome (CFS). Front Immunol. 12:628741. Erratum in: Front Immunol 2022; 13: 878196. DOI: 10.3389/fimmu.2021.628741. PMID: 35046929. PMCID: PMC8761622. PMID: ddf59507dd584643ae1adee542c0aa80.


82. Ianiro G, Tilg H, Gasbarrini A. 2016; Antibiotics as deep modulators of gut microbiota: between good and evil. Gut. 65:1906–15. DOI: 10.1136/gutjnl-2016-312297. PMID: 27531828.


83. Smith ME, Haney E, McDonagh M, Pappas M, Daeges M, Wasson N, et al. 2015; Treatment of myalgic encephalomyelitis/chronic fatigue syndrome: a systematic review for a national institutes of health pathways to prevention workshop. Ann Intern Med. 162:841–50. DOI: 10.7326/M15-0114. PMID: 26075755.


84. Strayer DR, Carter WA, Brodsky I, Cheney P, Peterson D, Salvato P, et al. 1994; A controlled clinical trial with a specifically configured RNA drug, poly(I).poly(C12U), in chronic fatigue syndrome. Clin Infect Dis. 18 Suppl 1:S88–95. DOI: 10.1093/clinids/18.Supplement_1.S88. PMID: 8148460.
85. Strayer DR, Carter WA, Stouch BC, Stevens SR, Bateman L, Cimoch PJ, Mitchell WM, et al. Chronic Fatigue Syndrome AMP-516 Study Group. 2012; A double-blind, placebo-controlled, randomized, clinical trial of the TLR-3 agonist rintatolimod in severe cases of chronic fatigue syndrome. PLoS One. 7:e31334. DOI: 10.1371/journal.pone.0031334. PMID: 22431963. PMCID: PMC3303772. PMID: f22a0d4d54c84b618175fd9f35ada78a.


86. Montoya JG, Kogelnik AM, Bhangoo M, Lunn MR, Flamand L, Merrihew LE, et al. 2013; Randomized clinical trial to evaluate the efficacy and safety of valganciclovir in a subset of patients with chronic fatigue syndrome. J Med Virol. 85:2101–9. DOI: 10.1002/jmv.23713. PMID: 23959519.


87. Peterson PK, Shepard J, Macres M, Schenck C, Crosson J, Rechtman D, et al. 1990; A controlled trial of intravenous immunoglobulin G in chronic fatigue syndrome. Am J Med. 89:554–60. DOI: 10.1016/0002-9343(90)90172-A. PMID: 2239975.


88. Straus SE, Dale JK, Tobi M, Lawley T, Preble O, Blaese RM, et al. 1988; Acyclovir treatment of the chronic fatigue syndrome. Lack of efficacy in a placebo-controlled trial. N Engl J Med. 319:1692–8. DOI: 10.1056/NEJM198812293192602. PMID: 2849717.


89. Watt T, Oberfoell S, Balise R, Lunn MR, Kar AK, Merrihew L, et al. 2012; Response to valganciclovir in chronic fatigue syndrome patients with human herpesvirus 6 and Epstein-Barr virus IgG antibody titers. J Med Virol. 84:1967–74. DOI: 10.1002/jmv.23411. PMID: 23080504.


90. Theuretzbacher U, Outterson K, Engel A, Karlén A. 2020; The global preclinical antibacterial pipeline. Nat Rev Microbiol. 18:275–85. DOI: 10.1038/s41579-019-0288-0. PMID: 31745331. PMCID: PMC7223541.


Fig. 1
Venn diagram illustrating the overlap of patient-specific, antimicrobial-specific, and disease-specific contributors to pain from infection.
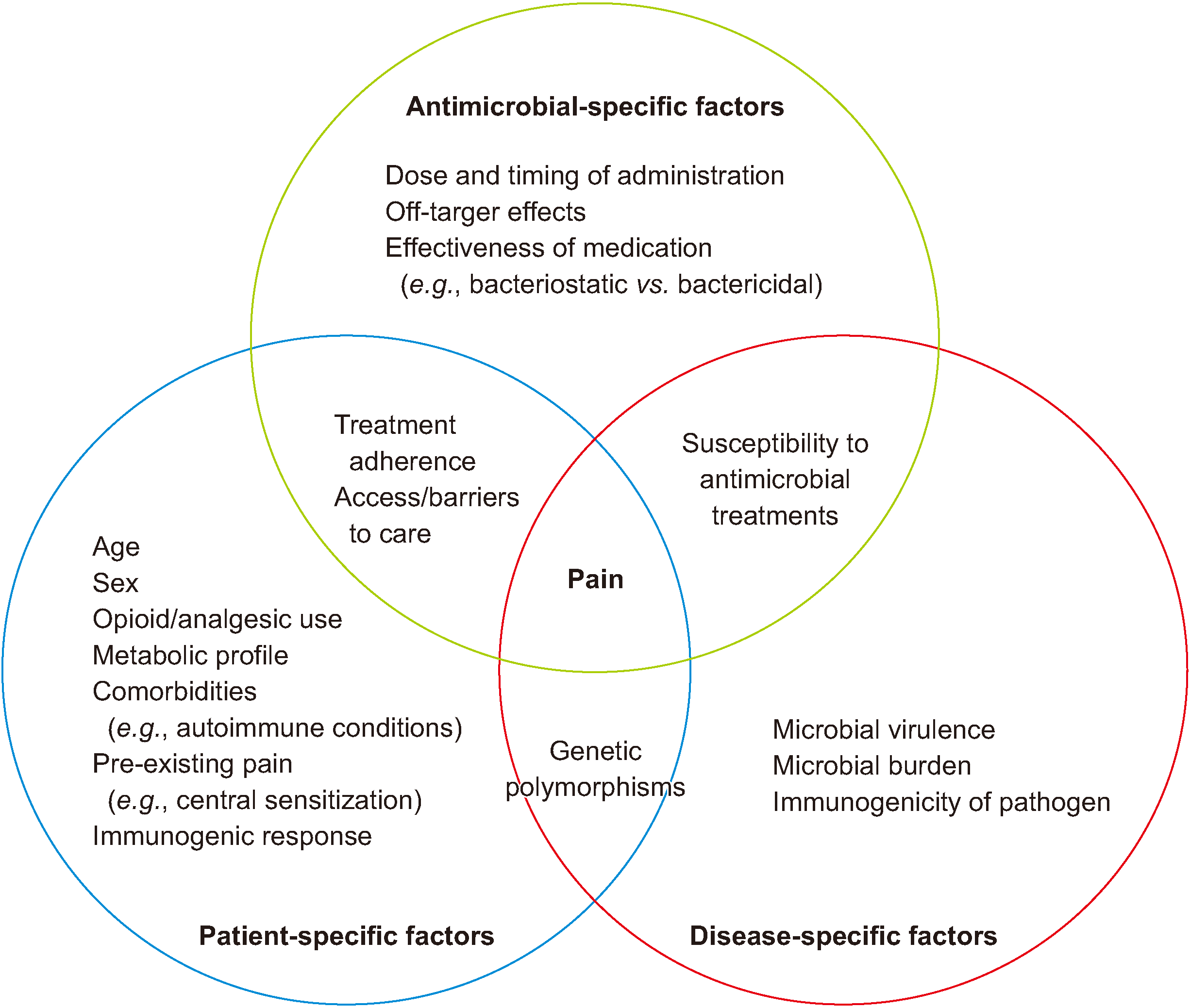
Table 1
Summary of studies, systematic reviews, and meta-analyses examining the effect of acute antimicrobial treatment on preventing chronic pain symptoms
Study, Year | Study type | Conditions & populations | Results | Comments |
---|---|---|---|---|
Chen et al., 2014 [19] | Systematic review and meta-analysis | Herpes zoster, prevention of PHN; 6 included studies (n = 1,211) | Meta-analysis of 3 RCTs (n = 609) found no difference between acyclovir and control groups for incidence of PHN 4 months after acute herpetic rash (RR, 0.75; 95% CI, 0.51 to 1.11) or at 6 months based on 2 trials. In 4 trials (n = 692), there was some evidence for a reduction in pain 4 weeks after onset of rash. | One study (n = 419) for famciclovir found no evidence for 500 mg or 750 mg for reducing incidence of PHN compared to placebo. |
Xie et al., 2022 [33] | Retrospective study in patients with at least 2 risk factors for “long Covid” and were not hospitalized after positive test | Prevention of long Covid in veterans; included 9,217 patients who received nirmatrelvir within 5 days of positive COVID test and 47,123 who did not. | Receiving treatment resulted in a reduced risk (HR, 0.74; 95% CI, 0.69, 0.81) of post-acute sequelae of COVID, including myalgia. | Published on a preprint server, without peer review. Among 12 variables examined, including muscle pain, the only 2 which did not decrease in incidence were cough and new-onset diabetes. |
Byambasuren et al., 2023 [38] | Systematic review | Vaccination and prevention of long Covid; 16 observational studies included, comprising 614,392 patients. No RCTs identified. No meta-analysis due to heterogeneity of data. | OR values ranged widely and exceeded 1.0 (0.22 to 1.03 for one dose; 0.25–1.02 for two doses; 0.48–1.01 for any dose); CIs not available due to lack of meta-analysis. No clear pattern regarding whether additional vaccination doses confer greater protection. Five studies reported data on vaccine administration subsequent to infection, and OR values ranged from 0.38–0.91. | Unknown whether different vaccines (e.g., BNT 162b2, mRNA-1273, ChAdOx1 nCoV-1) confer different magnitudes of long Covid prevention. |
Robertson et al., 2005 [44] | Meta-analysis of randomized or quasi-randomized studies comparing antibiotics to no antibiotics. | Prevention of rheumatic fever in patients treated for sore throat, with or without documentation of GAS infection. | In 10 trials (n = 7,665), antibiotics had a protective effect of 70% (RR, 0.32; 95% CI, 0.21–0.48). The absolute risk reduction was 1.67% with an NNT of 53. In the 9 studies evaluating penicillin, the protective effect was 80%. | Although pain is a prominent symptom of rheumatic fever, it was not evaluated separately. All studies were performed before 1962, with 8 of 10 including only adult males on military bases. Only 3 studies utilized a placebo. 9 studies evaluated penicillin, and 2 evaluated other antibiotic regimens (1 study evaluated 3 antibiotics versus placebo). |
Lennon et al., 2009 [46] | Meta-analysis of RCTs, or trials of “before/after design,” that utilized penicillin for primary prophylaxis for rheumatic fever. | Prevention of rheumatic fever in school-aged children treated with penicillin via school- and/or community-based programs | Meta-analysis of 6 RCTs (n = 1,087,874; authors note that for one of the included studies, they adjusted the sample size “to allow for the unit of randomization being school [sic]” without further details given). Penicillin had a protective effect of approximately 60% (RR, 0.41; 95% CI, 0.23–0.70). | Although pain is a prominent symptom of rheumatic fever, it was not evaluated separately. Authors did not report an “unadjusted” total n value. Follow-up periods in some included studies were not clearly defined. |
Lennon et al., 2009 [47] | RCT of schools in Auckland, New Zealand; entire schools, rather than individual students, were randomized. | Prevention of rheumatic fever in school-aged children (n = approximately 22,000; 86,874 person-years). Schools (n = 53) randomized to intervention (n = 27) received an onsite clinic that could diagnose and treat GAS pharyngitis. Children in schools randomized to control (n = 26) continued usual care via their primary care providers. | Using 1956 (probable) and 1965 (definite) Jones Criteria (“Analysis A”), risk reduction was 21% (incidence RR, 0.79; 95% CI, 0.41–1.52, P = 0.47). Using 1992 Jones Criteria and echocardiography (“Analysis B”), risk reduction was 28% (incidence RR, 0.72; 95% CI, 0.40–1.30, P = 0.27). In both analyses, the difference between the intervention and control group was non-significant. | Because school zoning allowed for a child to attend a control-group school and a younger or older sibling in the same family to attend an intervention-group school, any protective effects from the intervention might have been negated by household exposures to GAS. Pain-related symptoms were not evaluated separately. |
Lennon et al., 2017 [48] | Prospective cohort study of school-aged children in Auckland, New Zealand. | Prevention of rheumatic fever in school-aged children (n = approximately 25,000) via the implementation of onsite sore throat clinics in schools (n = 61). | After two years of clinic availability, rates of rheumatic fever among 5–13 year-olds decreased by 58% (88 [95% CI, 79–111] per 100,000 pre-intervention to 37 [95% CI, 15–83] per 100,000). Pharyngeal GAS prevalence decreased from 22.4% (16.5–30.5) pre-intervention to 11.9% (8.6–16.5) and 11.4% (8.2–15.7) 1 or 2 years later (P = 0.005). | Onsite school clinics were established within geographic regions, reducing the likelihood of household contamination and spread if one sibling were to have access to a clinic while another did not. Clinics likely increased timely access to penicillin. Pain-related symptoms were not evaluated separately. |
Table 2
Reviews of antibiotic therapy for chronic pain conditions
Study, Year | Study type | Conditions & populations | Results | Comments |
---|---|---|---|---|
Gilligan et al., 2021 [50] | Narrative review | 8 studies, 3 of which were placebo-controlled (controlled trials pertaining to chronic back pain and vertebral endplate signal changes). | 2 of 4 observational studies and 2 of 3 controlled trials were positive. Herniated and degenerated discs might contain bacteria at concentrations below detection thresholds. Nearly 50% of degenerated discs were infected (most commonly with Cutibacterium acnes). | 2 positive controlled studies assessed patients with Modic type 1 changes and used amoxicillin-clavulanic acid. The negative controlled study enrolled patients with Modic type 1 or type 2 changes and used amoxicillin (without clavulanic acid). All studies entailed a 100-day antibiotic course. Both positive controlled studies showed a statistically significant reduction in disability and pain, but more studies are necessary to determine which subpopulations would benefit most from antibiotics. |
Anothaisintawee et al., 2011 [51] | Systematic review and meta-analysis | 3 studies, 226 patients with chronic prostatitis/pelvic pain syndrome | Reduction in pain compared to placebo: −4.4 (95% CI, −7.0 to −1.9) for antibiotics, −5.7 (95% CI, −7.8 to −3.6) for combination of α-blockers + antibiotics. | Based on a single study [90], the combination of antibiotics + α-blockers was more effective than other treatments. Duration of treatment was 6–12 weeks. Antibiotics included ciprofloxacin, levofloxacin, and tetracycline. |
Franco et al., 2019 [52] | Systematic review and meta-analysis | 99 studies, 9,119 men with chronic prostatitis/pelvic pain syndrome; of these, 6 studies in 693 participants compared antibiotics to placebo | Antibiotics (fluoroquinolones) may reduce symptoms compared to placebo. Mean difference in National Institute of Health - Chronic Prostatitis Symptom Index score: –2.43 (95% CI, –4.72 to –0.15) from pooled data of 5 studies, 372 participants; low quality of evidence. Antibiotics do not significantly improve sexual dysfunction or quality of life, but are not associated with adverse events (moderate quality of evidence). | Because all included studies utilized fluoroquinolones (levofloxacin or ciprofloxacin), these results cannot be generalized to other antimicrobial classes. |
Ford et al., 2018 [56] | Systematic review and meta-analysis | 9 studies, 2,845 patients with irritable bowel syndrome | All studies favored antibiotics compared to placebo. | 7 studies evaluated rifaximin, with 2 evaluating ciprofloxacin and neomycin, both of which were positive. When 4 rifaximin studies with low risk-of-bias were evaluated separately, results favored antibiotic treatment (RR = 0.87, 95% CI, 0.82–0.93). |
Black et al., 2020 [57] | Systematic review and meta-analysis | 18 studies, 9,844 patients with irritable bowel syndrome | 5-hydroxytryptamine-3 receptor antagonists (e.g., alosetron, ramosetron) were the most effective medications, compared to eluxadoline (a mixed μ-opioid receptor agonist, δ-opioid receptor antagonist, and κ-opioid receptor agonist), rifaximin, and placebo. | Rifaximin conferred the lowest risk of adverse effects, including constipation, but did not demonstrate greater efficacy than placebo for improvement in global IBS symptoms (RR, 0.91, 95% CI, 0.77–1.07) or abdominal pain (RR, 0.95, 95% CI, 0.89–1.01). |
Townsend et al., 2019 [64] | Systematic review and meta-analysis | 13 studies, 1,303 patients with Crohn’s disease | Patients who received antibiotics had a lower rate of failure to achieve remission compared to those who received placebo, within 6–10 weeks (RR, 0.86, 95% CI, 0.76 to 0.98; 7 studies, high certainty evidence) and 10–14 weeks (RR, 0.77, 95% CI, 0.64 to 0.93; 5 studies, moderate certainty evidence). | Antibiotics did not appear to reduce rates of relapse within 52 weeks compared to placebo (RR, 0.87, 95% CI, 0.52 to 1.47; 2 studies, low certainty evidence). |
Norton et al., 2017 [65] | Systematic review | 15 studies, with only 1 randomized comparative- effectiveness study assessing antibiotics for inflammatory bowel disease (n = 29) [65] | In the randomized comparative-effectiveness study [65], either metronidazole or ciprofloxacin reduced abdominal symptoms (84% response rate for bloating, 47% for abdominal pain), without difference in efficacy between antibiotics. | Small bowel bacterial overgrowth was present in 29 of 145 patients with Crohn’s disease, with baseline abdominal pain present in 48%. There was no difference in outcomes between a 10-day course of either metronidazole or ciprofloxacin. Glucose breath tests normalized in most patients (27 of 29) after antibiotic treatment. |
Du et al., 2016 [69] | Systematic review and meta-analysis | 25 studies, 5,555 patients with functional dyspepsia and Helicobacter pylori | Pooled RR was 1.23 (95% CI, 1.12–1.36, P < 0.0001) for symptom improvement after H. pylori eradication therapy. Symptoms improved at follow-up ≥ 1 year (RR = 1.24; 95% CI, 1.12–1.37, P < 0.0001) but not < 1 year (RR = 1.26; 95% CI, 0.83–1.92, P = 0.27). Seven studies showed no benefit on quality of life (SMD –0.01, 95% CI, –0.11 to 0.08, P = 0.80). | There was no short-term (< 1 year) symptomatic improvement, but there was significant long-term (≥ 1 year) improvement. This might be attributable to a greater likelihood of antibiotic-treated patients achieving histologic resolution of chronic gastritis (RR = 7.13; 95% CI, 3.68–13.81, P < 0.00001) and not developing peptic ulcer disease (RR = 0.35; 95% CI, 0.18–0.68, P = 0.002). |
Kang et al., 2019 [70] | Systematic review and meta-analysis | 18 studies, 4,774 patients with functional dyspepsia and H. pylori | Eradication of H. pylori significantly improved dyspepsia symptoms (RR, 1.18; 95% CI, 1.07–1.30, P < 0.01) regardless of local prevalence. | In the subgroup analysis of RCTs conducted in Asia, there was no significant benefit from H. pylori eradication therapy (RR, 1.14; 95% CI, 0.99–1.33, P = 0.08). Due to differences in trial design (e.g., shorter duration of treatment regimens), smaller sample sizes, and conflicting results between the individual RCTs completed in Asia, this result should be interpreted with caution. |
Ford et al., 2022 [71] | Systematic review and meta-analysis | 29 studies, 6,781 patients with functional dyspepsia and H. pylori | Eradication therapy was more likely than non-antibiotic therapies to result in symptom resolution (RR of non-cure = 0.91, 95% CI, 0.89 to 0.94; NNT = 14, 95% CI, 11 to 21) or symptomatic improvement (RR of no improvement = 0.84, 95% CI, 0.78 to 0.91; NNT =,9, 95% CI, 7 to 17). Subgroup analyses did not show a significant difference between different antibiotic regimens. | Authors concluded there is high-quality evidence supporting H. pylori eradication therapy in functional dyspepsia, but were unable to determine whether these benefits are directly due to the reduction of infectious burden or via indirect effects on gastrointestinal dysbiosis. The treatment effect size was larger in patients who had eradication of H. pylori (RR of non-cure = 0.74, 95% CI, 0.64 to 0.85; NNT = 6, 95% CI, 4 to 10). |
Smith et al., 2015 [83] | Systematic review | Myalgic encephalomyelitis/ chronic fatigue syndrome | Four studies [83–86] assessed antimicrobial therapies. Two [83,84] assessed rintatolimod, one [85] assessed valganciclovir, and one [86] assessed intravenous immunoglobulin G. Improvements in physical function and cognition were reported, but two studies were small [85,86], and one study [86] showed no benefit. | Although nearly all patients reported pain symptoms (e.g., myalgia, headaches, arthralgia), changes in pain were not separately assessed in the included studies. Changes in inflammatory cytokines and immune cell counts were observed. |