Abstract
Hepatocellular carcinoma (HCC) is a prevalent malignant tumor with high fatality. It has yet to be reported whether circ-SNX27 can affect the progression of HCC. This study attempted to analyze circ-SNX27’s precise role and underlying mechanisms in HCC. HCC cell lines and tumor specimens from HCC patients were analyzed using quantitative real-time PCR and Western blotting to quantify the expressions of circ-SNX27, miR-375, and ribophorin I (RPN1). Cell invasion and cell counting kit 8 experiments were conducted for the evaluation of HCC cell invasion and proliferation. Caspase-3 Activity Assay Kit was utilized to gauge the caspase-3 activity. Luciferase reporter and RNA immunoprecipitation assays were executed to ascertain the relationships among miR-375, circ-SNX27, and RPN1. To determine how circ-SNX27 knockdown affects the growth of HCC xenografts in vivo, tumor-bearing mouse models were constructed. Elevated expressions of circ-SNX27 and RPN1 as well as a reduced miR-375 expression were observed among HCC cells and HCC patient tumor specimens. Knocking-down circ-SNX27 in HCC cells abated their proliferative and invasive abilities but raised their caspase-3 activity. Moreover, the poor levels of circ-SNX27 inhibited HCC tumor growth among the mice. Circ-SNX27 enhanced RPN1 by competitively binding with miR-375. Silencing miR-375 in HCC cells promoted their malignant phenotypes. Nonetheless, the promotive effect of miR-375 silencing was reversible via the knockdown of circ-SNX27 or RPN1. This research demonstrated that circ-SNX27 accelerated the progression of HCC by modulating the miR-375/RPN1 axis. This is indicative of circ-SNX27’s potential as a target for the treatment of HCC.
Hepatocellular carcinoma (HCC), the most common malignant tumor in the liver, ranked sixth among the most prevalent cancers worldwide in 2020 [1]. The main clinical treatments for HCC are liver resection and liver transplantation. In addition, there are other treatment methods, namely interventional therapy, chemoradiotherapy, and immunotherapy [2]. Since its early symptoms are often not obvious, HCC is difficult to detect and diagnose early [3]. Most patients are diagnosed when they are already in the middle and late stages, thus losing opportunities for surgical resection. The recurrence rate of HCC after 5 years is as high as 70% [4]. The high recurrence rate and abilities to invade and metastasize lead to the extremely high mortality from HCC. Hence, it is crucial to investigate HCC’s molecular mechanism and search for its valuable diagnostic markers and therapeutic targets.
Circular RNAs (circRNAs) are single-stranded circular non-coding RNAs that exhibit tissue-specific expression patterns [5]. In the past few years, numerous research have confirmed that circRNAs are dysregulated in HCC and involved in HCC development [6,7]. For instance, circASAP1 is greatly expressed in HCC tumors with high metastatic potential. CircASAP1 accelerates the pulmonary metastasis of HCC through the miR-326/miR-532-5p/MAPK1/CSF-1 pathway [8]. CircMET acts as an oncogene in HCC by enhancing the immunosuppressive tumor microenvironment via the Snail/DPP4/CXCL10 axis [9]. CircTRIM33-12 regulates the miR-191/TET1 axis to repress the immune evasion abilities and malignant phenotypes of HCC cells [10]. Here, we found that a novel circRNA circ-SNX27 made from SNX27 gene was upregulated in HCC. The name of circ-SNX27 in circBase is hsa_circ_0014188. Nevertheless, circ-SNX27’s role in HCC has yet to be reported.
In the progression of HCC, microRNAs (miRNAs) serve as tumor suppressor genes or oncogenes to modulate the differentiation, proliferation, angiogenesis, invasion, and metastasis of tumor cells [11]. Lin et al. [12] have confirmed that miR-4454 elevates the vascularization of HCC cells by targeting Vps4A and Rab27A. MiR-125b-5p enhances sorafenib resistance in HCC cells by regulating ataxin-1-mediated epithelial-mesenchymal transition and stemness, which leads to poor prognosis among HCC patients [13]. Additionally, earlier reports have confirmed that miR-375 exerts an antitumor effect during HCC progression [14,15]. Still, it remains unclear whether circ-SNX27 can affect HCC progression by regulating miR-375.
Ribophorin I (RPN1) is an important endoplasmic reticulum oligosaccharyltransferase that assists the nascent protein to enter the rough endoplasmic reticulum and undergo glycosylation modification [16]. A previous study has found that RPN1 is upregulated in breast cancer. Moreover, its knockdown represses the malignant phenotypes of breast cancer cells [17]. RPN1 with high frequency mutations has been observed in gastric cancer tumors; it is possible that it may become a biomarker for gastric cancer [18]. According to an online database GEPIA (http://gepia.cancer-pku.cn/index.html), we found RPN1 with high expression in HCC, and its high expression predicted poor prognosis. Nevertheless, there currently no studies elucidating the mechanism of RPN1 in HCC. Moreover, TaregetScan predicted the targeting relationship between RPN1 and miR-375, but their targeting relationship has not been reported.
This study used bioinformatics online tools (CircInteractome and TargetScan) in predicting the potential binding sites of circ-SNX27, miR-375, and RPN1. We hypothesized that circ-SNX27 regulates the miR-375/RPN1 axis to promote the malignant phenotypes of HCC cells. This work suggests that circ-SNX27 may be a novel target for HCC treatment.
HCC patients (n = 36) who underwent partial hepatectomy were recruited in this study. None of the patients received any treatments before the operation. All patients were informed and signed a consent form. HCC tumoral tissue specimens were obtained from the patients. The adjacent normal tissues served as the control. Through pathological diagnoses, all tissue samples were confirmed to be HCC. All tissue samples were stowed and maintained at –80°C for further analysis. Ethics Committee of Wuhan Hospital of Traditional Chinese Medicine authorized all the protocols (approval number: 2021176). The protocols were all executed in accordance with the Declaration of Helsinki.
HCC cell lines (Hep 3B, Huh7, MHCC-97H, and SNU-182) and human normal liver cells (THLE-2) were cultivated in RPMI-1640 medium (Thermo Fisher Scientific) that contained 1% penicillin/streptomycin and 10% fetal bovine serum (FBS; Thermo Fisher Scientific). The cultures were incubated with 5% CO2 at 37°C. RPMI-1640 medium was replaced every two days. The cells were cultured up to the third passage for further use. All cell lines were acquired from the Cell Center of the Chinese Academy of Sciences.
Small interfering RNAs (siRNA) that specifically target circ-SNX27 (si-circ) and RPN1 (si-RPN1) were used to knockdown circ-SNX27 and RPN1, respectively. The miR-375 mimic (miR-375) was utilized to overexpress miR-375 while miR-375 inhibitor (inhibitor) was used to silence it. The scrambled siRNA (si-NC), miR-NC, and inhibitor-NC served as the controls. GenePharma synthesized all these oligonucleotides. Using 2 μl of Lipofectamine 2000 Transfection Reagent (Thermo Fisher Scientific), 1 μg of oligonucleotides were transfected into Huh7 and MHCC-97H cells (1 × 103/μl) and subsequently incubated for 48 h at 37°C. Quantitative real-time PCR (qRT-PCR) was conducted to assess the efficiency of the transfections.
PARIS Kit (Thermo Fisher Scientific) was utilized for the isolation of the total, nuclear, and cytoplasmic RNA from cells and tissues. In brief, cells were suspended in a cell fractionation buffer and maintained on ice for 10 min. Thereafter, the supernatant (cytoplasmic fraction) and pellet (nuclear fraction) were collected. In addition, cells and tissues were treated with cell disruption buffer, and the total lysate was collected. The total, nuclear, and cytoplasmic lysates were all treated with lysis/binding solution to collect total, nuclear, and cytoplasmic RNA. For RNase R assay, the total RNA was treated with 1 μl ribonuclease R (RNase R; BioVision) for 15 min.
The total, cytoplasmic, and nuclear RNA from cells and tissues were reverse transcribed into complementary DNA (cDNA) with the aid of the RevertAid First Strand cDNA Synthesis Kit (Thermo Fisher Scientific). The ABI 7500 FAST Real-Time PCR System (Applied Biosystems) and SYBR Green (Thermo Fisher Scientific) were used to carry out the PCR. U6 served as the internal control for miR-375 while circ-SNX27 and RPN1 mRNA were normalized against GAPDH. The 2-∆∆CT method was applied for the analysis of the data. Divergent primer spanning the junction of circRNA can be used to differentiate between circRNA and its linear gene [19]. Therefore, the divergent primer of circ-SNX27 was designed. Table 1 lists all the primers used.
In a 96-well plate, Huh7 and MHCC-97H cells at the logarithmic phase were maintained at 37°C with 5% CO2 for 24, 48, and 72 h. Subsequently, the cells were treated with 10 μl CCK-8 reagent (MedChem Express) for two more hours. Lastly, a microplate reader (Thermo Fisher Scientific) quantified the absorbance in each well at 450 nm.
Caspase-3 Activity Assay Kit (Beyotime) was utilized to assess the caspase-3 activity by means of a spectrophotometric method. In brief, Huh7 and MHCC-97H cells were treated in a lysis buffer and maintained on ice for 15 min. The cells were subsequently incubated at 37°C for an hour with Ac-DEVD-pNA. A spectrophotometer (Thermo Fisher Scientific) was then used to detect the absorbance at 405 nm.
Transwell chambers (Corning Incorporated) were employed for the evaluation of cell invasion. Huh7 and MHCC-97H cells and serum-free RPMI-1640 were added into the upper compartments pre-coated with extracellular Matrix gel (Sigma-Aldrich). Meanwhile, the lower chambers were filled with RPMI-1640 supplemented with 10% FBS. After 12 h of incubation, the cells that successfully invaded the lower chambers were treated with 4% formaldehyde and 0.1% crystal violet (Beyotime) to fix and stain them, respectively. The invading cells were viewed and counted under a light microscope (Nikon).
Six- to eight-week-old male BALB/c nude mice (Shanghai SLAC Laboratory Animal Co., Ltd) were maintained in specific-pathogen-free conditions. Short hairpin RNAs (shRNA) that specifically target circ-SNX27 (sh-circ) and the scrambled shRNA (sh-NC) were synthesized by GenePharma. These shRNAs were packaged into lentiviral particles. Huh7 cells were infected with the lentiviruses (LV) carrying sh-circ to induce circ-SNX27 knockdown. Then, the nude mice were divided to sh-circ and sh-NC groups (five mice per group). The right armpits of the nude mice were subcutaneously injected with 2 × l04/μl of Huh7 cells infected with either LV-sh-circ-SNX27 or LV-sh-NC according to different groups. The tumor volume was recorded every week; tumor volume = (long diameter × short diameter2)/2. Five weeks after the inoculation, the tumors were extracted from the euthanized mice, and their weights were measured. The Ethics Committee of Wuhan Hospital of Traditional Chinese Medicine authorized all protocols (approval number: WHTCM2021039).
GenePharma synthesized the wild-type (WT) and mutant (MUT) luciferase reporter vectors pGL3-circ-SNX27 and pGL3-RPN1. Briefly, the WT fragments of circ-SNX27 and RPN1 with the binding site of miR-375 were amplified by PCR (circ-SNX27 forward primer: 5’-AAGCTTAGAGAAGAAGCCCCG-3’ and reverse primer: 5’-CTCGAGCCTCGTGCATATTCG-3’; RPN1 forward primer: 5’-AAGCTTGCACTGCTCTTCCCG-3’ and reverse primer: 5’-CTCGAGAAATGTTCACAGTCC-3’), and inserted into pGL3 luciferase vectors as WT-pGL3-circ-SNX27 and WT-pGL3-RPN1. To construct MUT vectors, the binding sites of circ-SNX27 and RPN1 for miR-1184 were mutated using QuikChange XL Site-Directed Mutagenesis Kit (Agilent Technologies), and also inserted into pGL3 luciferase vectors as MUT-pGL3-circ-SNX27 and MUT-pGL3-RPN1. Using 2 μl of the Lipofectamine 2000 Transfection Reagent, the Huh7 and MHCC-97H cells were transfected with a combination of a reporter vector (WT/MUT-pGL3-circ-SNX27 or WT/MUT-pGL3-RPN1) and either miR-375 mimic or miR-NC. Finally, the luciferase activity in the cells was detected by means of the dual-luciferase reporter gene assay system (Promega).
The interaction of circ-SNX27 with miR-375 was analyzed with the aid of the Magna RIP RNA Binding Protein Immunoprecipitation kit (EMD Millipore). Huh7 and MHCC-97H cells were treated with the RIPA Lysis Buffer (Beyotime). Magnetic beads were coated with antibodies against IgG (1:100 dilution; #ab172730; Abcam) or Argonaute 2 (Ago2; 1:50 dilution; #ab186733; Abcam) and then maintained for 6 h at 4°C. Thereafter, the cell lysate was maintained for an hour at 4°C with the magnetic beads-protein complex. At the end, qRT-PCR was conducted to analyze the RNA eluted from the magnetic beads-protein-RNA complex.
Cells and tissues were subjected to RIPA Lysis Buffer (Beyotime) treatment to extract their total proteins. The protein concentrations were then estimated with the aid of the BCA Protein Assay Kit (Beyotime). Afterward, the 25 μg of proteins per lane were electrophoresed through a 10% SDS-polyacrylamide gel. The separated proteins were moved onto nitrocellulose membranes and then treated with 10% skimmed milk solution for 2 h at room temperature. Next, the membranes were maintained at a temperature of 4°C overnight, together with GAPDH (1:2,500 dilution; #ab9485; Abcam) and rabbit anti-human RPN1 (1:2,000 dilution; #ab197888; Abcam) antibodies. On the following day, goat anti-rabbit IgG-HRP (1:5,000 dilution: #ab6721; Abcam) was added onto the membranes before they were incubated for 2 h at room temperature. GAPDH was the internal reference. The immunoreactive bands were imaged with an ECL chemiluminescence solution (Beyotime) and then analyzed in Image Lab Software.
Each assay was performed in triplicates. All values were analyzed in GraphPad Prism 8 (GraphPad Software Inc.) and indicated as the mean ± standard deviation. Student’s t-test and one-way ANOVA were applied for the analyses of the statistical differences between or among the groups. Spearman’s correlation ascertained the correlations between variables. There was a significant difference when the p < 0.05.
The differences in circ-SNX27 expression among the 36 pairs of HCC tumoral and adjacent normal tissues were compared via qRT-PCR. As shown in Fig. 1A, the tumor tissues from HCC patients manifested higher circ-SNX27 expressions than the matched adjacent tissues. Circ-SNX27 also had considerably higher expressions in the HCC cells (Hep 3B, Huh7, MHCC-97H, and SNU-182) than in the normal liver cells (THLE-2) (Fig. 1B). The MHCC-97H and Huh7 cells exhibited the highest expressions of circ-SNX27 among the cell lines used, hence they were used for further analyses. The levels of circ-SNX27 within the nuclei and cytoplasm of Huh7 and MHCC-97H cells were analyzed via qRT-PCR. The outcomes revealed that that circ-SNX27 was predominantly distributed within the cytoplasm (Fig. 1C). Additionally, RNase R was used to treat the total RNA to determine its influence on the stability of circ-SNX27. The results obtained from qRT-PCR uncovered that RNase R treatment resulted in a reduction in linear SNX27 expression but had no effect on that of circ-SNX27 in Huh7 and MHCC-97H cells (Fig. 1D). These findings suggest that the high levels of circ-SNX27 expression was closely linked with the progression of HCC.
The effect of circ-SNX27 silencing in MHCC-97H and Huh7 cells was investigated. QRT-PCR was conducted to analyze the transfection efficiency of si-circ-SNX27 (si-circ). The MHCC-97H and Huh7 cells manifested a remarkable downregulation of circ-SNX27 after the transfection of si-circ (Fig. 2A). CCK-8 assay was performed to evaluate the proliferation of HCC cells. As shown in Fig. 2B, the circ-SNX27 knockdown diminished the proliferative ability of MHCC-97H and Huh7 cells. The detection of caspase-3 activity revealed that circ-SNX27 deficiency significantly enhanced the caspase-3 activity among the MHCC-97H and Huh7 cells (Fig. 2C). Cell invasion assay was conducted to observe how the circ-SNX27 knockdown impacted the invasion ability of HCC cells. It was found that knocking-down circ-SNX27 significantly suppressed the invasion of MHCC-97H and Huh7 cells (Fig. 2D). Tumor-bearing mouse models were constructed to determine how the circ-SNX27 knockdown influences the growth of HCC tumors. The deficiency in circ-SNX27 notably decreased the weight and volume of the tumor xenografts (Fig. 2E). The abovementioned findings demonstrate that circ-SNX27 knockdown suppresses the development of HCC in vivo and in vitro.
The bioinformatics software CircInteractome predicted a potential miR-375 binding site on circ-SNX27 (Fig. 3A), which was confirmed by means of the luciferase reporter experiment. miR-375 mimic reduced the luciferase activity when the MHCC-97H and Huh7 cells were transfected with WT-circ-SNX27. However, miR-375 mimic could not change the luciferase activity in the MHCC-97H and Huh7 cells after the transfection of MUT-circ-SNX27 (Fig. 3B). The outcomes of the RIP assay revealed that the circ-SNX27 and miR-375 were enriched in anti-Ago2 group (Fig. 3C). These findings suggest that circ-SNX27 interacts with miR-375 in HCC. QRT-PCR results indicate the notable downregulation of miR-375 in the tumoral tissues from HCC patients (Fig. 3D). Spearman’s correlation ascertained the negative association of miR-375 expression with that of circ-SNX27 among the HCC patient specimens (Fig. 3E). Furthermore, the MHCC-97H and Huh7 cells manifested lower miR-375 expressions than the THLE2 cells (Fig. 3F). To investigate the role of circ-SNX27 in regulating miR-375 expression, qRT-PCR analysis was carried out after circ-SNX27 and miR-375 were silenced in MHCC-97H and Huh7 cells. The miR-375 expression in MHCC-97H and Huh7 cells was remarkably boosted by the circ-SNX27 knockdown but repressed by the miR-375 inhibitor. The miR-375 inhibition effected by the miR-375 inhibitor was counteracted by the deficiency in circ-SNX27 (Fig. 3G). Altogether, these findings suggest that circ-SNX27 directly targets miR-375 and negatively regulates its expression.
The effect of circ-SNX27/miR-375 axis on HCC progression was explored in vitro. The outcomes of the CCK-8 assay revealed that miR-375 inhibition improved the proliferative ability of the MHCC-97H and Huh7 cells. However, that effect was counteracted by circ-SNX27 silencing (Fig. 4A). Low caspase-3 activity was detected among MHCC-97H and Huh7 cells after miR-375 knockdown. Circ-SNX27 silencing, on the other hand, increased the caspase-3 activity in HCC cells and offset the decrease brought about by the miR-375 inhibitor (Fig. 4B). Moreover, results of the cell invasion assay uncovered that the enhanced cell invasion induced by the miR-375 inhibitor was neutralized by circ-SNX27 silencing (Fig. 4C). In general, these suggest that circ-SNX27 knockdown inhibited HCC progression through miR-375 sponging.
The bioinformatics software TargetScan predicted the interaction of miR-375 with the 3’UTR of RPN1 mRNA (Fig. 5A). The results of the luciferase reporter experiment revealed that the combined transfection of WT-RPN1 and miR-375 mimic reduced the luciferase activity within MHCC-97H and Huh7 cells. On the other hand, no change in the relative activity of luciferase was observed upon the combined transfection of MUT-RPN1 and miR-375 mimic. These outcomes indicate that RPN1 is a miR-375 target (Fig. 5B). According to the data from GEPIA database, RPN1 expression was upregulated in HCC (Supplementary Fig. 1A), and its high expression predicted the poor prognosis (Supplementary Fig. 1B). Then, RPN1 expression was also assessed in HCC patient specimens via qRT-PCR. The results revealed an upregulation of RPN1 among the tumor specimens from HCC patients (Fig. 5C). Furthermore, Spearman’s correlation uncovered the negative correlation of RPN1 expression with miR-375 expression in HCC tumor specimens (Fig. 5D). In comparison with THLE2 cells, a remarkable RPN1 upregulation was observed among the MHCC-97H and Huh7 cells (Fig. 5E). The expression of RPN1 after the knockdown of RPN1 and miR-375 was analyzed via Western blotting. The MHCC-97H and Huh7 cells manifested a decreased RPN1 expression in the presence of si-RPN1. In contrary, an enhanced RPN1 expression was observed after the knockdown of miR-375 in MHCC-97H and Huh7 cells. RPN1 silencing offset the miR-375-inhibitor-enhanced expression of RPN1 (Fig. 5F). Taken together, these findings suggest that miR-375 interacted with RPN1 and repressed RPN1 expression.
Herein, the influence of the miR-375/RPN1 axis on the malignant phenotypes of HCC cells was explored. The results of the CCK-8 experiment indicated that the RPN1 knockdown resulted in reduced proliferation among MHCC-97H and Huh7 cells. In contrast, miR-375 deficiency enhanced the proliferation of the HCC cells. Nevertheless, RPN1 knockdown counteracted this promotive effect induced by the miR-375 inhibitor (Fig. 6A). The caspase-3 activity in MHCC-97H and Huh7 cells was enhanced by RPN1 deficiency and repressed by miR-375 silencing. Furthermore, the diminished caspase-3 activity effected by the miR-375 inhibitor was partially reversed by RPN1 knockdown (Fig. 6B). Cell invasion assay was performed assess cell invasion. It was observed that the low levels of RPN1 significantly abated the invasion ability of MHCC-97H and Huh7 cells. Meanwhile, inhibiting miR-375 stimulated the invasion of MHCC-97H and Huh7 cells, which was reversible by RPN1 silencing (Fig. 6C). In summary, the knockdown of miR-375 accelerated proliferation, apoptosis, and invasion of HCC cells by upregulating RPN1 expression.
HCC has a high incidence and fatality worldwide and is seriously endangering the health of humans. HCC patient tumor specimens and HCC cells manifested an upregulation of circ-SNX27 and RPN1 and a downregulation of miR-375. Circ-SNX27 enhanced RPN1 expression by sponging miR-375. Knocking-down circ-SNX27 repressed the invasion and proliferation of HCC cells and promoted their apoptosis through the miR-375/RPN1 axis. Moreover, the deficiency in circ-SNX27 repressed the growth of HCC tumor xenografts among the mice. All these findings demonstrate that circ-SNX27 advances the progression of HCC by regulating the miR-375/RPN1 axis.
CircRNAs are special endogenous non-coding RNAs with highly conserved structures and stable sequences [20]. CircRNAs can suppress or promote cancer during HCC progression by regulating tumor cell phenotypes [21]. For instance, a high circCRIM1 expression boosts cell proliferation and angiogenesis among HCC cells through the modulation of the miR-378a-3p/SKP2 axis. This can be used as a biomarker for the poor prognosis of HCC patients [22]. CircANTXR1 regulates the miR-532-5p/XRCC5 axis to accelerate the development of HCC in vivo and in vitro [23]. Meanwhile, low levels of circ_GLIS2 were observable in HCC cells and tumors. Circ_GLIS2 exerts an antitumor effect that represses cell growth and metastasis in HCC [24]. The role in HCC of the newly identified circRNA, circ-SNX27, has yet to be reported. This work has been the first to uncover the carcinogenic effect of circ-SNX27 in the progression of HCC. We found an upregulation of a novel circRNA, circ-SNX27, in the tumor specimens from HCC patients and in HCC cells. Knocking-down circ-SNX27 promoted apoptosis and repressed the proliferation and invasion of HCC cells. A deficiency in circ-SNX27 also inhibited the growth of HCC tumor xenografts in mice. Hence, these findings are indicative of circ-SNX27’s function as an oncogene in HCC.
An accumulation of research has confirmed that circRNAs can affect tumor progression by regulating miRNAs in HCC [25,26]. Considering that, we used bioinformatics tools and screened the downstream target gene of circ-SNX27 and miR-375. MiR-375 may suppress tumors during tumorigenesis. Chang et al. [27] have documented that miR-375 is poorly expressed in HCC cells and tumor tissues. Moreover, they reported that miR-375 reduces the ATG7-mediated autophagy of HCC cells while in hypoxic conditions. Circ_0072088 accelerates HCC development by repressing miR-375 expression and activating the JAK2/STAT3 signaling pathway [28]. Delivering a combination of miR-375 and doxorubicin by means of liposomes or lipid-coated calcium carbonate nanoparticles exerts a synergistic antitumor effect and reverses drug resistance in HCC [29,30]. Consistently, miR-375 exerted an anti-cancer effect in HCC. In our research, we also observed low levels of miR-375 in HCC cells and tumors. Furthermore, circ-SNX27 repressed miR-375 expression through sponging. The silencing of miR-375 then boosted the proliferation and invasion of HCC cells while also repressing their apoptosis. Nevertheless, these effects may be offset by knocking-down circ-SNX27. All these outcomes suggest that circ-SNX27 advanced HCC progression by sponging miR-375.
As a part of the oligosaccharyltransferase complex, RPN1 assists nascent proteins to enter the rough endoplasmic reticulum. Moreover, RPN1 participates in ribosome binding, transmembrane translocation, and further modification of nascent proteins [16]. A previous study has confirmed the presence of high RPN1 expression in breast cancer. RPN1 exerts an oncogenic function in breast cancer through the promotion of an endoplasmic-reticulum-stress-dependent apoptosis [17]. Milde-Langosch et al. [31] have revealed that the abnormal expression of the glycosylation gene RPN1 has prognostic significance in breast cancer. In addition, the rare structural rearrangement of the 3q21 loci around RPN1 has been observed in childhood acute myeloblastic leukemia [32]. Nonetheless, RPN1’s functional role in HCC remains unknown. Our work uncovered that circ-SNX27 elevated RPN1 levels through miR-375 sponging. Knocking-down RPN1 in HCC cells reversed the effects of miR-375 silencing, thus resulting in an enhanced apoptosis and reduced abilities to proliferate and invade. Our results demonstrate that circ-SNX27 accelerated HCC progression by regulating the miR-375/RPN1 axis.
Certainly, this article also has some shortcomings. The origin of Hep3B cell line is from pediatric HCC, whereas the origin of SNU-182, Huh7 and MHCC-97H cell lines is from adult HCC. In our study, circ-SNX29 expression showed higher expression in Huh7 and MHCC-97H, suggesting that the function of circ-SNX29 in pediatric HCC might be different from adult HCC. Therefore, we will collect more clinical samples from child and adults with HCC to further explore the effect of circ-SNX29. In vivo experiments confirmed that the circ-SNX27 deficiency inhibited the growth of HCC tumor xenografts. The function of the miR-375/RPN1 axis in regulating HCC progression shall be studied further in vivo. Additionally, tumorigenesis is a very complex process that involves many signaling pathways. This work only confirmed the mechanism of the circ-SNX27/miR-375/RPN1 axis in HCC. We will further explore other signaling pathways in the progression of HCC in the future.
In conclusion, this work confirms that circ-SNX27 regulates the miR-375/RPN1 axis to contribute to HCC progression. Herein, we highlighted the involvement of a novel regulatory network, circ-SNX27/miR-375/RPN1 axis, in the progression of HCC.
Supplementary data including one figure can be found with this article online at https://doi.org/10.4196/kjpp.2023.27.4.333.
REFERENCES
1. Sung H, Ferlay J, Siegel RL, Laversanne M, Soerjomataram I, Jemal A, Bray F. 2021; Global cancer statistics 2020: GLOBOCAN estimates of incidence and mortality worldwide for 36 cancers in 185 countries. CA Cancer J Clin. 71:209–249. DOI: 10.3322/caac.21660. PMID: 33538338.


2. Eugen K. 2020; Current treatment options for hepatocellular carcinoma. Klin Onkol. 33(Supplementum 3):20–25. DOI: 10.14735/amko20203S20. PMID: 33213161.


3. Hartke J, Johnson M, Ghabril M. 2017; The diagnosis and treatment of hepatocellular carcinoma. Semin Diagn Pathol. 34:153–159. DOI: 10.1053/j.semdp.2016.12.011. PMID: 28108047.


4. Pascual S, Herrera I, Irurzun J. 2016; New advances in hepatocellular carcinoma. World J Hepatol. 8:421–438. DOI: 10.4254/wjh.v8.i9.421. PMID: 27028578. PMCID: PMC4807304.


5. Zhou WY, Cai ZR, Liu J, Wang DS, Ju HQ, Xu RH. 2020; Circular RNA: metabolism, functions and interactions with proteins. Mol Cancer. 19:172. DOI: 10.1186/s12943-020-01286-3. PMID: 33317550. PMCID: PMC7734744. PMID: d632b21718e94b679d98a2fde221e564.


6. Qiu L, Xu H, Ji M, Shang D, Lu Z, Wu Y, Tu Z, Liu H. 2019; Circular RNAs in hepatocellular carcinoma: biomarkers, functions and mechanisms. Life Sci. 231:116660. DOI: 10.1016/j.lfs.2019.116660. PMID: 31319086.


7. Qiu L, Wang T, Ge Q, Xu H, Wu Y, Tang Q, Chen K. 2019; Circular RNA signature in hepatocellular carcinoma. J Cancer. 10:3361–3372. DOI: 10.7150/jca.31243. PMID: 31293639. PMCID: PMC6603403.


8. Hu ZQ, Zhou SL, Li J, Zhou ZJ, Wang PC, Xin HY, Mao L, Luo CB, Yu SY, Huang XW, Cao Y, Fan J, Zhou J. 2020; Circular RNA sequencing identifies CircASAP1 as a key regulator in hepatocellular carcinoma metastasis. Hepatology. 72:906–922. DOI: 10.1002/hep.31068. PMID: 31838741.


9. Huang XY, Zhang PF, Wei CY, Peng R, Lu JC, Gao C, Cai JB, Yang X, Fan J, Ke AW, Zhou J, Shi GM. 2020; Circular RNA circMET drives immunosuppression and anti-PD1 therapy resistance in hepatocellular carcinoma via the miR-30-5p/snail/DPP4 axis. Mol Cancer. 19:92. DOI: 10.1186/s12943-020-01213-6. PMID: 32430013. PMCID: PMC7236145. PMID: c04084b82de94621b6820d97eb69f9a9.


10. Zhang PF, Wei CY, Huang XY, Peng R, Yang X, Lu JC, Zhang C, Gao C, Cai JB, Gao PT, Gao DM, Shi GM, Ke AW, Fan J. 2019; Circular RNA circTRIM33-12 acts as the sponge of MicroRNA-191 to suppress hepatocellular carcinoma progression. Mol Cancer. 18:105. DOI: 10.1186/s12943-019-1031-1. PMID: 31153371. PMCID: PMC6545035. PMID: 0941232519964eb28e6de3caea9e3619.


11. Oura K, Morishita A, Masaki T. 2020; Molecular and functional roles of MicroRNAs in the progression of hepatocellular carcinoma- a review. Int J Mol Sci. 21:8362. DOI: 10.3390/ijms21218362. PMID: 33171811. PMCID: PMC7664704. PMID: 6b8ff7c912b040bbb0dcb0b70fc64189.


12. Lin H, Zhang R, Wu W, Lei L. 2021; miR-4454 promotes hepatic carcinoma progression by targeting Vps4A and Rab27A. Oxid Med Cell Longev. 2021:9230435. DOI: 10.1155/2021/9230435. PMID: 34777698. PMCID: PMC8580624.


13. Hirao A, Sato Y, Tanaka H, Nishida K, Tomonari T, Hirata M, Bando M, Kida Y, Tanaka T, Kawaguchi T, Wada H, Taniguchi T, Okamoto K, Miyamoto H, Muguruma N, Tanahashi T, Takayama T. 2021; MiR-125b-5p is involved in sorafenib resistance through ataxin-1-mediated epithelial-mesenchymal transition in hepatocellular carcinoma. Cancers (Basel). 13:4917. DOI: 10.3390/cancers13194917. PMID: 34638401. PMCID: PMC8508441. PMID: de7840a3314e4a9ab1c3b4ebeda2b913.


14. Li D, Wang T, Sun FF, Feng JQ, Peng JJ, Li H, Wang C, Wang D, Liu Y, Bai YD, Shi ML, Zhang T. 2021; MicroRNA-375 represses tumor angiogenesis and reverses resistance to sorafenib in hepatocarcinoma. Cancer Gene Ther. 28:126–140. DOI: 10.1038/s41417-020-0191-x. PMID: 32616906. PMCID: PMC7886652.


15. Wang C, Luo J, Chen Z, Ye M, Hong Y, Liu J, Nie J, Zhao Q, Chang Y. 2021; MiR-375 impairs the invasive capabilities of hepatoma cells by targeting HIF1α under hypoxia. Dig Dis Sci. 66:493–502. DOI: 10.1007/s10620-020-06202-9. PMID: 32215815.


16. Takeda K, Qin SY, Matsumoto N, Yamamoto K. 2014; Association of malectin with ribophorin I is crucial for attenuation of misfolded glycoprotein secretion. Biochem Biophys Res Commun. 454:436–440. DOI: 10.1016/j.bbrc.2014.10.102. PMID: 25451265.


17. Ding J, Xu J, Deng Q, Ma W, Zhang R, He X, Liu S, Zhang L. 2021; Knockdown of Oligosaccharyltransferase subunit ribophorin 1 induces endoplasmic-reticulum-stress-dependent cell apoptosis in breast cancer. Front Oncol. 11:722624. DOI: 10.3389/fonc.2021.722624. PMID: 34778038. PMCID: PMC8578895. PMID: 6da03cac95164c21ab93aa445f99c353.


18. Liu D, Li L, Wang L, Wang C, Hu X, Jiang Q, Wang X, Xue G, Liu Y, Xue D. 2021; Recognition of DNA methylation molecular features for diagnosis and prognosis in gastric cancer. Front Genet. 12:758926. DOI: 10.3389/fgene.2021.758926. PMID: 34745226. PMCID: PMC8566671. PMID: 9fa9baea30a043f688655922bef75ce1.


19. Dudekula DB, Panda AC, Grammatikakis I, De S, Abdelmohsen K, Gorospe M. 2016; CircInteractome: a web tool for exploring circular RNAs and their interacting proteins and microRNAs. RNA Biol. 13:34–42. DOI: 10.1080/15476286.2015.1128065. PMID: 26669964. PMCID: PMC4829301.


20. Yu T, Wang Y, Fan Y, Fang N, Wang T, Xu T, Shu Y. 2019; CircRNAs in cancer metabolism: a review. J Hematol Oncol. 12:90. DOI: 10.1186/s13045-019-0776-8. PMID: 31484561. PMCID: PMC6727394. PMID: a8a94aef9e8d402fbf07ab6c1955359b.


21. Xiong DD, Dang YW, Lin P, Wen DY, He RQ, Luo DZ, Feng ZB, Chen G. 2018; A circRNA-miRNA-mRNA network identification for exploring underlying pathogenesis and therapy strategy of hepatocellular carcinoma. J Transl Med. 16:220. DOI: 10.1186/s12967-018-1593-5. PMID: 30092792. PMCID: PMC6085698. PMID: dc69d800dd6349869c0b534291b49bb1.


22. Ji Y, Yang S, Yan X, Zhu L, Yang W, Yang X, Yu F, Shi L, Zhu X, Lu Y, Zhang C, Lu H, Zhang F. 2021; CircCRIM1 promotes hepatocellular carcinoma proliferation and angiogenesis by sponging miR-378a-3p and regulating SKP2 expression. Front Cell Dev Biol. 9:796686. DOI: 10.3389/fcell.2021.796686. PMID: 34869393. PMCID: PMC8634842. PMID: fecaed6fef494100bbadfdad8baca916.


23. Huang C, Yu W, Wang Q, Huang T, Ding Y. 2021; CircANTXR1 contributes to the malignant progression of hepatocellular carcinoma by promoting proliferation and metastasis. J Hepatocell Carcinoma. 8:1339–1353. DOI: 10.2147/JHC.S317256. PMID: 34786378. PMCID: PMC8590609.


24. Xing W, Zhou PC, Zhang HY, Chen LM, Zhou YM, Cui XF, Liu ZG. 2022; Circular RNA circ_GLIS2 suppresses hepatocellular carcinoma growth and metastasis. Liver Int. 42:682–695. DOI: 10.1111/liv.15097. PMID: 34743403.


25. Zhang L, Zhang J, Li P, Li T, Zhou Z, Wu H. 2022; Exosomal hsa_circ_0004658 derived from RBPJ overexpressed-macrophages inhibits hepatocellular carcinoma progression via miR-499b-5p/JAM3. Cell Death Dis. 13:32. DOI: 10.1038/s41419-021-04345-9. PMID: 35013102. PMCID: PMC8748962. PMID: 502fa7fb80b04c35ac3ca8aec42a0800.


26. Li D, Zhang J, Yang J, Wang J, Zhang R, Li J, Zhang R. 2021; CircMTO1 suppresses hepatocellular carcinoma progression via the miR-541-5p/ZIC1 axis by regulating Wnt/β-catenin signaling pathway and epithelial-to-mesenchymal transition. Cell Death Dis. 13:12. DOI: 10.1038/s41419-021-04464-3. PMID: 34930906. PMCID: PMC8688446. PMID: d4fa7a43ad5d47aa89dbbfd957771eab.


27. Chang Y, Yan W, He X, Zhang L, Li C, Huang H, Nace G, Geller DA, Lin J, Tsung A. 2012; miR-375 inhibits autophagy and reduces viability of hepatocellular carcinoma cells under hypoxic conditions. Gastroenterology. 143:177–187.e8. DOI: 10.1053/j.gastro.2012.04.009. PMID: 22504094.


28. Li L, Xiao C, He K, Xiang G. 2021; Circ_0072088 promotes progression of hepatocellular carcinoma by activating JAK2/STAT3 signaling pathway via miR-375. IUBMB Life. 73:1153–1165. DOI: 10.1002/iub.2520. PMID: 34148288.


29. Fan YP, Liao JZ, Lu YQ, Tian DA, Ye F, Zhao PX, Xiang GY, Tang WX, He XX. 2017; MiR-375 and doxorubicin co-delivered by liposomes for combination therapy of hepatocellular carcinoma. Mol Ther Nucleic Acids. 7:181–189. DOI: 10.1016/j.omtn.2017.03.010. PMID: 28624193. PMCID: PMC5415965. PMID: a5e56e9582a44791a292feb19a4e6314.


30. Zhao P, Wu S, Cheng Y, You J, Chen Y, Li M, He C, Zhang X, Yang T, Lu Y, Lee RJ, He X, Xiang G. 2017; MiR-375 delivered by lipid-coated doxorubicin-calcium carbonate nanoparticles overcomes chemoresistance in hepatocellular carcinoma. Nanomedicine. 13:2507–2516. DOI: 10.1016/j.nano.2017.05.010. PMID: 28577837.


31. Milde-Langosch K, Karn T, Schmidt M, zu Eulenburg C, Oliveira-Ferrer L, Wirtz RM, Schumacher U, Witzel I, Schütze D, Müller V. 2014; Prognostic relevance of glycosylation-associated genes in breast cancer. Breast Cancer Res Treat. 145:295–305. DOI: 10.1007/s10549-014-2949-z. PMID: 24737166.


32. Haltrich I, Kost-Alimova M, Kovács G, Klein G, Fekete G, Imreh S. 2006; Multipoint interphase FISH analysis of chromosome 3 abnormalities in 28 childhood AML patients. Eur J Haematol. 76:124–133. DOI: 10.1111/j.1600-0609.2005.00576.x. PMID: 16405433.


Fig. 1
Circ-SNX27 upregulation was observed in hepatocellular carcinoma (HCC) cell lines and tumors.
(A) Circ-SNX27 expressions in the normal or tumor samples from HCC patient specimens were quantified via qRT-PCR. (B) Circ-SNX27 expressions in normal liver cells (THLE-2) and HCC cells (Hep 3B, SNU-182, Huh7 and MHCC-97H) were determined by means of qRT-PCR. **p < 0.001 vs. THLE2 group. (C) qRT-PCR analysis of circ-SNX27 expression within the nuclei and cytoplasm of MHCC-97H and Huh7 cells. (D) The circ-SNX27 and linear SNX27 expressions in the MHCC-97H and Huh7 cells after RNase R treatment were measured via qRT-PCR. **p < 0.001 vs. control group. Values are presented as mean ± SD.
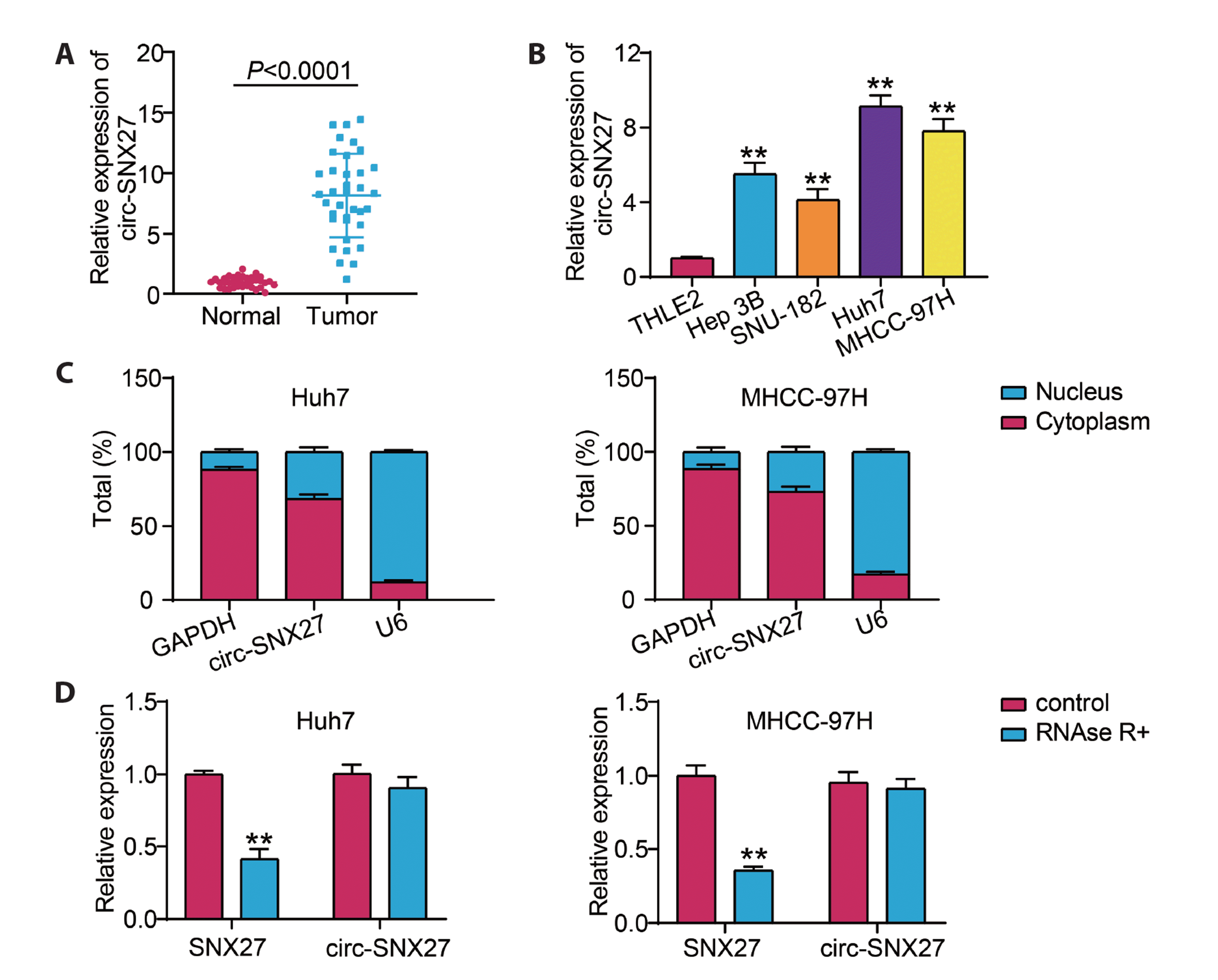
Fig. 2
Knocking-down circ-SNX27 inhibited the progression of hepatocellular carcinoma (HCC) in vivo and in vitro.
MHCC-97H and Huh7 cells were transfected with either si-NC or si-circ-SNX27 (si-circ). (A) The circ-SNX27 expression in the transfected HCC cells was analyzed through qRT-PCR. **p < 0.001 vs. si-NC group. (B) CCK-8 experiment was executed to assess the proliferative ability of these transfected HCC cells. *p < 0.05 vs. sin-NC group. **p < 0.001 vs. si-NC group. (C) Caspase-3 Activity Assay Kit was utilized to detect caspase-3 activity and assess apoptosis among the transfected HCC cells. **p < 0.001 vs. si-NC group. (D) Cell invasion assay was performed to assess the invasion ability of the transfected HCC cells. **p < 0.001 vs. si-NC group by Transwell invasion assays. ×200. (E) Tumor photos, weight, and volume of tumors in mice following the injection of LV-sh-circ-SNX27-infected Huh7 cells or LV-sh-NC-infected Huh7. **p < 0.001 vs. sh-NC group. Values are presented as mean ± SD.
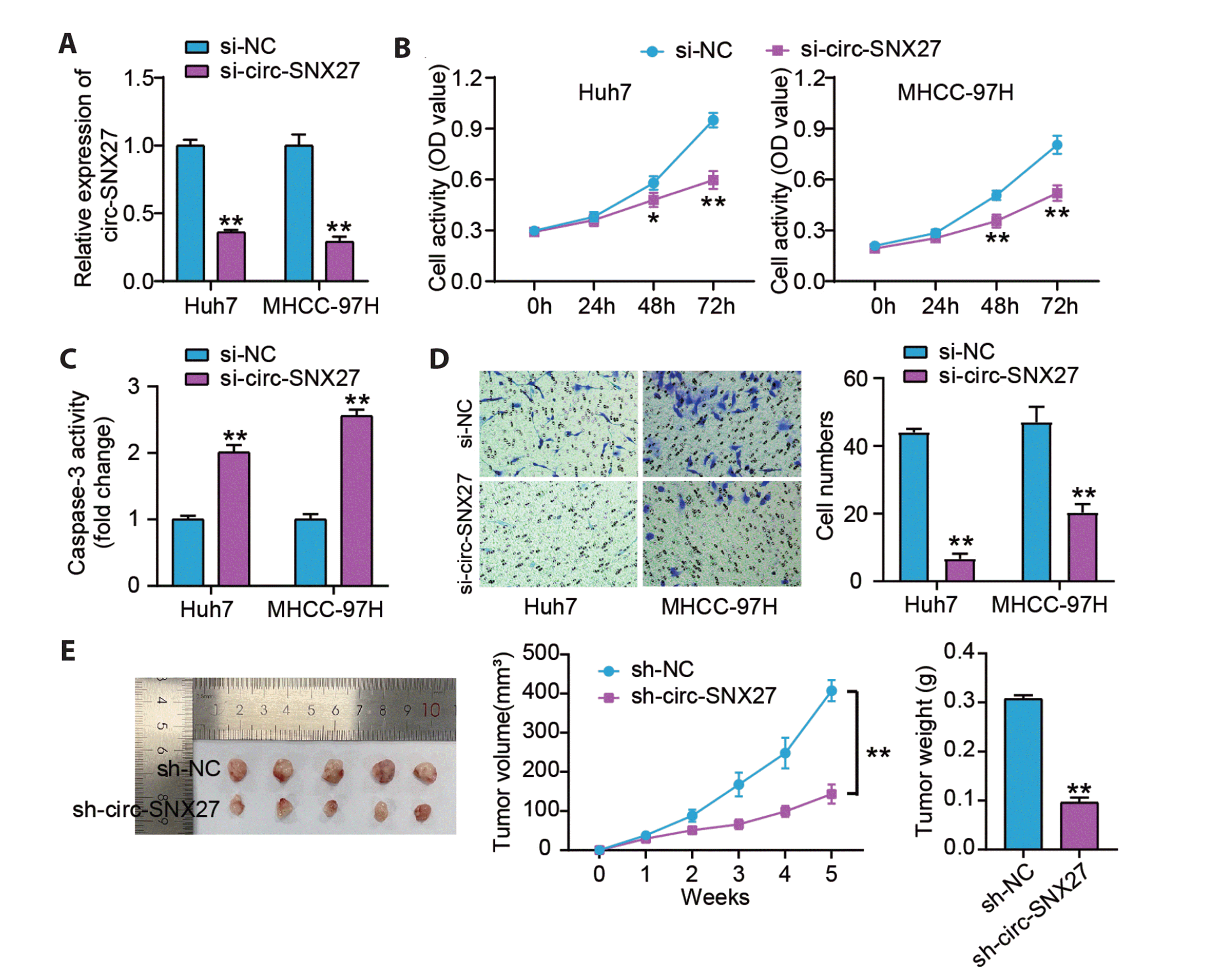
Fig. 3
Circ-SNX27 directly targeted miR-375.
(A) The circ-SNX27 and miR-375 binding site was predicted by CircInteractome. (B) Luciferase reporter experiment was executed to examine the interaction of circ-SNX27 with miR-375. **p < 0.001 vs. miR-NC group. (C) RIP assay was performed to assess circ-SNX27’s interaction with miR-375. **p < 0.001 vs. Anti-IgG group. (D) The miR-375 expressions in normal and tumoral hepatocellular carcinoma (HCC) tissues were quantified via qRT-PCR. (E) Spearman’s correlation analyzed the association of circ-SNX27 with miR-375. (F) MiR-375 expressions in normal cells (THLE2) and HCC cells (MHCC-97H and Huh7) were determined by means of qRT-PCR. **p < 0.001 vs. THLE2 group. (G) The relative miR-375 expression in MHCC-97H and Huh7 cells following the transfection of si-NC, inhibitor-NC, si-circ-SNX27 (si-circ), miR-375 inhibitor (inhibitor), or si-circ + inhibitor was analyzed by means of qRT-PCR. **p < 0.001 vs. si-NC group; &&p < 0.001 vs. inhibitor-NC group; ##p < 0.001 vs. si-circ + inhibitor group. Values are presented as mean ± SD. WT, wild-type; MUT, mutant; Ago2, Argonaute 2.
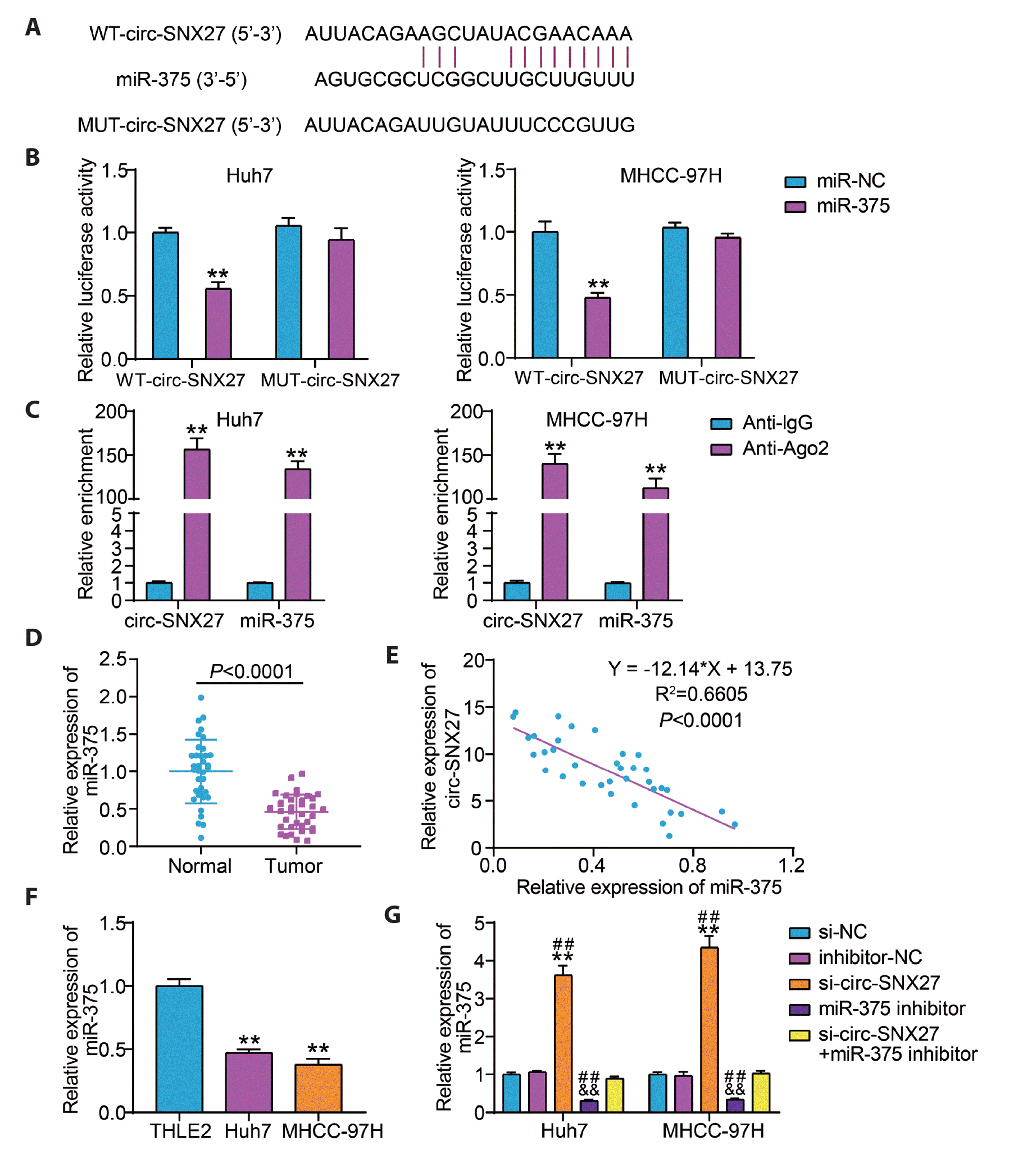
Fig. 4
Circ-SNX27 knockdown promoted apoptosis and repressed the proliferation and invasion of hepatocellular carcinoma (HCC) cells by regulating the expression of miR-375.
MHCC-97H and Huh7 cells were transfected with si-NC, inhibitor-NC, si-circ-SNX27 (si-circ), miR-375 inhibitor (inhibitor), or si-circ + inhibitor. (A) CCK-8 assay was conducted to evaluate the proliferation of the transfected HCC cells. (B) The caspase-3 activity in the transfected HCC cells was examined by means of the caspase-3 activity assay. (C) Cell invasion assay was executed to assess the invasion ability of the transfected HCC cells by transwell invasion assays. ×200. Values are presented as mean ± SD. *p < 0.05 and **p < 0.001 vs. si-NC group; &&p < 0.001 vs. inhibitor-NC group; #p < 0.05 and ##p < 0.001 vs. si-circ + inhibitor group.

Fig. 5
Ribophorin I (RPN1) was a miR-375 target gene.
(A) The miR-375 and RPN1 binding site was predicted by TargetScan. (B) Luciferase reporter assay was conducted to assess miR-375’s interaction with RPN1. **p < 0.001 vs. miR-NC group. (C) RPN1 expression in normal and tumoral hepatocellular carcinoma (HCC) tissues was quantified by means of qRT-PCR. (D) Spearman’s correlation analyzed the association of miR-375 with RPN1. (E) The RPN1 expressions among normal cells (THLE2) and HCC cells (MHCC-97H and Huh7) were determined via qRT-PCR. **p < 0.001 vs. THLE2 group. (F) The relative protein expressions of RPN1 expression in the MHCC-97H and Huh7 cells transfected with si-NC, inhibitor-NC, si-RPN1, miR-375 inhibitor (inhibitor), or si-RPN1 + inhibitor were quantified via Western blotting. *p < 0.05 and **p < 0.001 vs. si-NC group; &p < 0.01 and &&p < 0.001 vs. inhibitor-NC group; ##p < 0.001 vs. si-RNP1 + inhibitor group. Values are presented as mean ± SD. WT, wild-type; MUT, mutant.
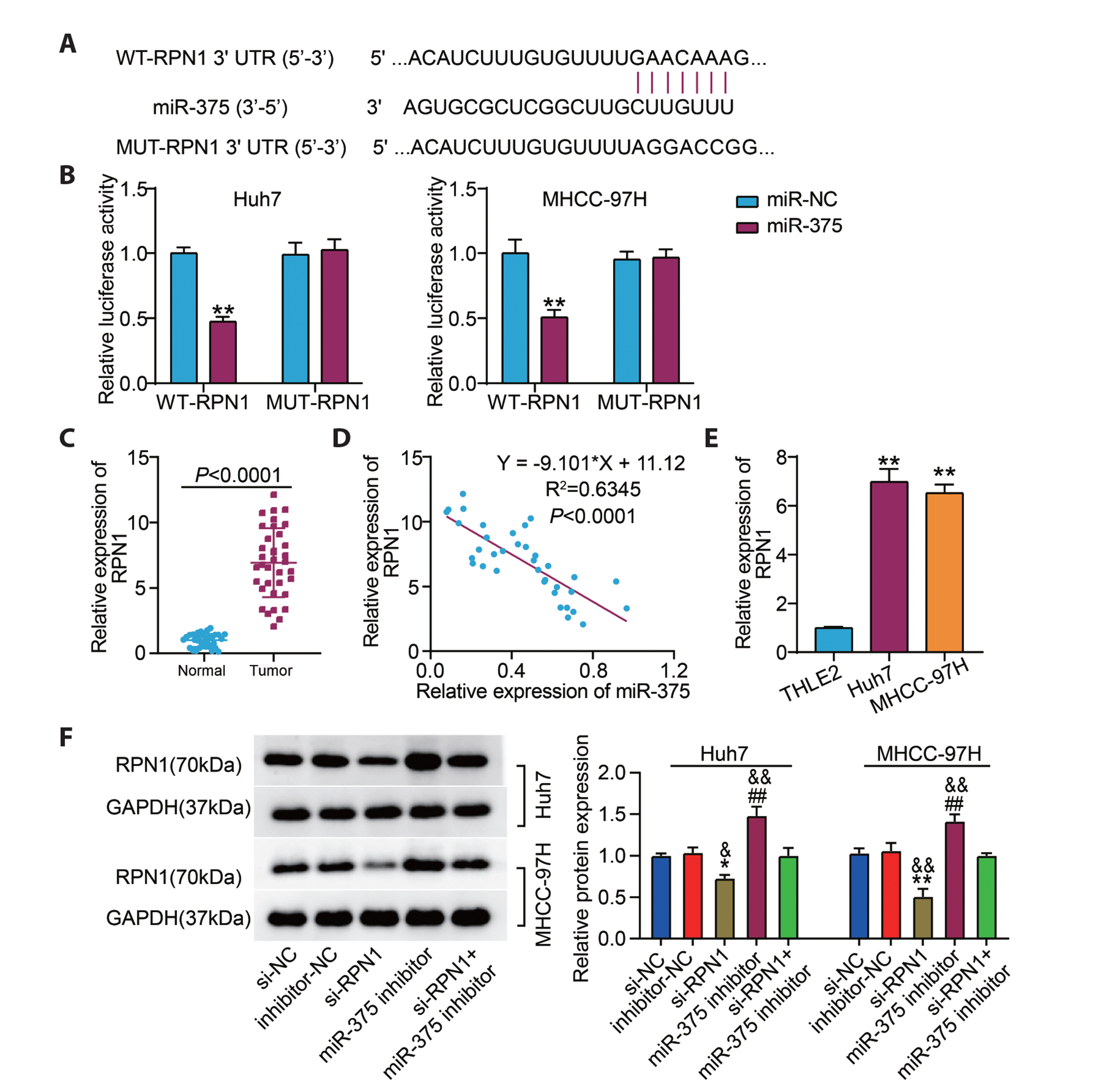
Fig. 6
MiR-375 knockdown inhibited apoptosis and stimulated the proliferation and invasion of hepatocellular carcinoma (HCC) cells by modulating ribophorin I (RPN1) expression.
MHCC-97H and Huh7 cells were transfected with si-NC, inhibitor-NC, si-RPN1, miR-375 inhibitor (inhibitor), or si-RPN1 + inhibitor. (A) CCK-8 assay was used to evaluate the proliferation of the transfected HCC cells. (B) The caspase-3 activity in the transfected HCC cells was examined by means of the caspase-3 activity assay. (C) Cell invasion assay was executed to assess the invasion ability of the transfected HCC cells by transwell migration assay. ×200. Values are presented as mean ± SD. *p < 0.05 and **p < 0.001 vs. si-NC group; &&p < 0.001 vs. inhibitor-NC group; #p < 0.05 and ##p < 0.001 vs. si-RPN1 + inhibitor group.
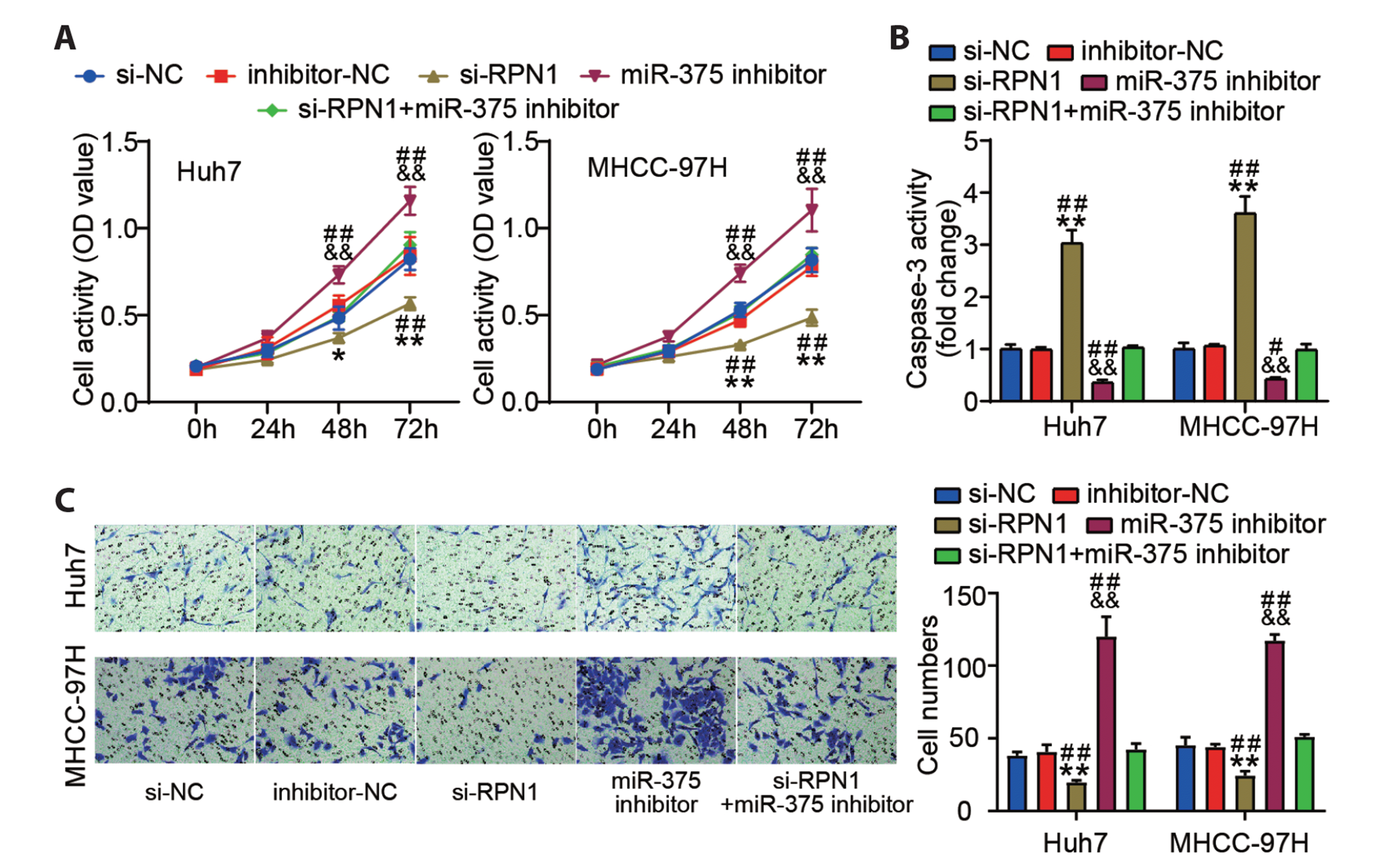
Table 1
Primer sequences used for qRT-PCR