Abstract
Purpose
The study was to investigate exercise capacity (peak oxygen uptake [peak VO2]) and pulmonary capacitance (GXcap), which is an estimate of pulmonary vascular capacitance, in patients with nonalcoholic steatohepatitis (NASH).
Methods
This study utilized a database of patients with NASH (n=26 [17 male and 9 female], aged 58.9±4.3 years) and healthy individuals (n=23 [12 male and 11 female, aged 58.6±7.9 years) who underwent a maximal exercise test on a recumbent cycle ergometer (Corival; Lode) in our laboratory. During cardiopulmonary exercise tests, breathing patterns and respiratory gas exchange including breathing efficiency (VE/VCO2) and end-tidal CO2 (PETCO2) were measured. In addition, peak VO2 was obtained via averaging the last 30 seconds at peak level and GXcap was obtained by calculation as follows: GXcap=oxygen pulse (O2 pulse)×PETCO2.
Results
The NASH group demonstrated reduced peak VO2 relative to the healthy group (17.5±8.4 mL/kg/min vs. 34±10.2 mL/kg/min, respectively; p<0.05). In addition, there was a higher VE/VCO2 relationship in the NASH group relative to the healthy group (34.9±5.5 vs. 32.2±4.0, respectively; p<0.05) and lower PETCO2 in the NASH group compared to the healthy group (32.8±4.0 mm Hg vs. 35.3±3.8 mm Hg, respectively; p<0.05). Furthermore, the NASH group showed lower GXcap than the healthy group (456±150 vs. 551±202, respectively; p<0.05).
Nonalcoholic steatohepatitis (NASH) is a severe form of nonalcoholic fatty liver disease (NAFLD), and it is characterized by hepatic steatosis associated with injury to the liver cell and inflammation. The prevalence of NASH has significantly increased in the last decade. There’s an estimate of about 24% of United States adults have NAFLD and about 1.5% to 6.5% have NASH1. Given this prevalence and health effects, NAFLD/NASH is a significant issue for the public’s health. NAFLD/NASH is closely linked to metabolic complications, diabetes, and dyslipidemia furthermore it appears to be associated with disease comorbidities including pulmonary dysfunction, cardiovascular disease, and kidney failure2. With respect to pulmonary function, several epidemiologic studies and longitudinal cohort studies revealed impaired pulmonary function in NAFLD/NASH3,4. Furthermore, patients with a higher degree of NASH are at an increased risk of decreased lung function5.
This impaired pulmonary function in this type of liver disease seems linked to increased metabolic complications and the inflammatory process. In our recent in vivo study, more severe inflammation and mitochondrial dysfunction in pulmonary parenchyma tissues were found in NAFLD model relative to the control6. In addition, increased pulmonary inflammation appeared to be highly associated with reduced exercise capacity and pulmonary vascular dysfunction7. Therefore, it can be hypothesized that patients with NASH may have lower exercise capacity and pulmonary capacitance compared to similar age-matched healthy adults; however, it has not been investigated extensively. Accordingly, the present study retrospectively investigated exercise capacity (peak oxygen uptake [peak VO2]), pulmonary capacitance (GXcap), and dynamic respiratory gas exchange and breathing pattern during exercise in patients with NASH.
To achieve the purpose of the present preliminary study, a database of patients with NASH, who were clinically diagnosed, and age-matched healthy individuals who underwent cardiopul-monary exercise tests (CPET) in our laboratory was utilized. The exercise modality was a recumbent cycle ergometer (Corival; Lode) and the exercise protocol included 2 minutes of resting phase and an incremental exercise phase. The wattage was increased by 25 W every 2 minutes until volitional fatigue. During CPET, VO2, breathing patterns and respiratory gas exchange including breathing efficiency (VE/VCO2) and end-tidal CO2 (PETCO2) were measured, and heart rate (HR) was monitored via electro-cardiograph. To determine peak exercise capacity, peak VO2 was obtained by averaging the last 30 seconds at peak level. In addition, oxygen pulse (O2 pulse), which represents stroke volume8, was calculated using the formula: O2 pulse=(absolute VO2×1,000)/HR; and GXcap was calculated using the formula: GXcap=O2 pulse× PETCO2. This gas exchange-based estimate of GXcap was pre-viously validated by our laboratory9,10 and confirmed to be well correlated to invasive measures of mean pulmonary arterial pressure and pulmonary vascular resistance9.
All values are illustrated as the mean±standard deviation. To observe the difference between the NASH group and the healthy group, an independent t-test or nonparametric t-test was conducted. Statistical analyses were performed using the IBM SPSS version 28.0 (IBM Corp.). The statistical significance level was set at p<0.05.
The present study was reviewed and approved by the Institutional Review Board of Mayo Clinic (No. 20-007471). This study is a retrospective study and does not require informed consent from the subjects.
There was no significant difference in age between the NASH group and the healthy group (p>0.05); however, the NASH group demonstrated a higher body mass index (BMI) relative to the healthy group (p<0.05) (Table 1). The NASH group demonstrated reduced peak VO2 relative to the healthy group (p<0.05; Fig. 1A). With respect to the key gas exchange, the NASH group demonstrated a higher VE/VCO2 (Table 1) and a lower PETCO2 (Fig. 1B) compared to the healthy group (p<0.05). In addition, although there was no significant difference in O2 pulse between groups (p>0.05; Fig. 1C), the NASH group showed lower GXcap than the healthy group (p<0.05; Fig. 1D).
In the present study, the NASH group demonstrated higher body weight and BMI compared to the healthy group. They showed reduced peak oxygen uptake, breathing efficiency, and gas exc-hange relative to the healthy group. In addition, they had reduced pulmonary capacitance compared to the Healthy group. Given that there were no significant differences in O2 pulse and HR at a given VO2 between groups, reduced pulmonary capacitance in the NASH group was more likely derived from reduced PETCO2, which is closely pulmonary arterial pressure and pulmonary vascular resistance11. These results suggest that patients with NASH have lower exercise capacity than age-matched healthy adults and moreover impaired dynamic respiratory gas exchange and pulmonary capacitance during exercise contribute largely to redu-ced exercise capacity. There may be several factors that can elicit impairments in respiratory gas exchange and pulmonary capa-citance in this type of liver disease; however, it can be speculated that increased pulmonary inflammation6 and altered pulmonary vascular tone12 have pivotal roles.
Except for spirometry-measured pulmonary function, there is still a lack of clinical insight into more in-depth pulmonary patho-physiology in NASH. In clinical practice, more specific pulmonary pathophysiology needs to be evaluated via multi-dimensional assessments. Although the present study reports preliminary data, the results may provide future directions to improve the therapeutic approach and help guide decision-making. However, this requires further studies with larger sample sizes to investigate clinical insights.
Acknowledgments
The present study was supported by Preventive Medicine Funding Program, Department of Cardiovascular Disease, Mayo Clinic.
REFERENCES
1. Gutiérrez-Cuevas J, Lucano-Landeros S, López-Cifuentes D, Santos A, Armendariz-Borunda J. 2022; Epidemiologic, genetic, pathogenic, metabolic, epigenetic aspects involved in NASH-HCC: current therapeutic strategies. Cancers (Basel). 15:23. DOI: 10.3390/cancers15010023.


2. Rosato V, Masarone M, Dallio M, Federico A, Aglitti A, Persico M. 2019; NAFLD and extra-hepatic comorbidities: current evidence on a multi-organ metabolic syndrome. Int J Environ Res Public Health. 16:3415. DOI: 10.3390/ijerph16183415. PMID: 31540048. PMCID: PMC6765902.


3. Song JU, Jang Y, Lim SY, et al. 2019; Decreased lung function is associated with risk of developing non-alcoholic fatty liver disease: a longitudinal cohort study. PLoS One. 14:e0208736. DOI: 10.1371/journal.pone.0208736. PMID: 30673698. PMCID: PMC6343945.
4. Mantovani A, Lonardo A, Vinco G, et al. 2019; Association between non-alcoholic fatty liver disease and decreased lung function in adults: a systematic review and meta-analysis. Diabetes Metab. 45:536–44. DOI: 10.1016/j.diabet.2019.04.008. PMID: 31067493.


5. Botello-Manilla AE, López-Sánchez GN, Chávez-Tapia NC, Uribe M, Nuño-Lámbarri N. 2021; Hepatic steatosis and respiratory diseases: a new panorama. Ann Hepatol. 24:100320. DOI: 10.1016/j.aohep.2021.100320. PMID: 33549735.


6. Cho J, Johnson BD, Watt KD, Niven AS, Yeo D, Kim CH. 2022; Exercise training attenuates pulmonary inflammation and mitochondrial dysfunction in a mouse model of high-fat high-carbohydrate-induced NAFLD. BMC Med. 20:429. DOI: 10.1186/s12916-022-02629-1. PMID: 36348343. PMCID: PMC9644617.
7. McNeill JN, Lau ES, Zern EK, et al. 2021; Association of obesity-related inflammatory pathways with lung function and exercise capacity. Respir Med. 183:106434. DOI: 10.1016/j.rmed.2021.106434. PMID: 33964816. PMCID: PMC8144063.


8. Bhambhani Y, Norris S, Bell G. 1994; Prediction of stroke volume from oxygen pulse measurements in untrained and trained men. Can J Appl Physiol. 19:49–59. DOI: 10.1139/h94-003. PMID: 8186762.


9. Taylor BJ, Olson TP, Chul-Ho Kim, Maccarter D, Johnson BD. 2013; Use of noninvasive gas exchange to track pulmonary vascular responses to exercise in heart failure. Clin Med Insights Circ Respir Pulm Med. 7:53–60. DOI: 10.4137/CCRPM.S12178. PMID: 24093002. PMCID: PMC3785385.


10. Kim CH, Hansen JE, MacCarter DJ, Johnson BD. 2017; Algorithm for predicting disease likelihood from a submaximal exercise test. Clin Med Insights Circ Respir Pulm Med. 11:1179548417719248. DOI: 10.1177/1179548417719248. PMID: 28757799. PMCID: PMC5513526.
11. Taylor BJ, Smetana MR, Frantz RP, Johnson BD. 2015; Submaximal exercise pulmonary gas exchange in left heart disease patients with different forms of pulmonary hypertension. J Card Fail. 21:647–55. DOI: 10.1016/j.cardfail.2015.04.003. PMID: 25887446. PMCID: PMC4522389.


12. Türker F, Sahın T, Oral A, et al. 2022; Evaluation of predisposing metabolic risk factors for portopulmonary hypertension in patients with NASH cirrhosis. Int J Gen Med. 15:859–65. DOI: 10.2147/IJGM.S339474.


Fig. 1
The difference in exercise capacity and gas exchange between groups. Nonalcoholic steatohepatitis group (NASH group) and age-matched healthy group (healthy group). (A) Peak oxygen uptake (peak VO2), (B) end-tidal carbon dioxide (PETCO2), (C) oxygen pulse (O2 pulse), and (D) pulmonary capacitance (GXcap). NS: not significant. *p<0.05, Healthy group versus NASH group, **p<0.001, healthy group vs. NASH group.
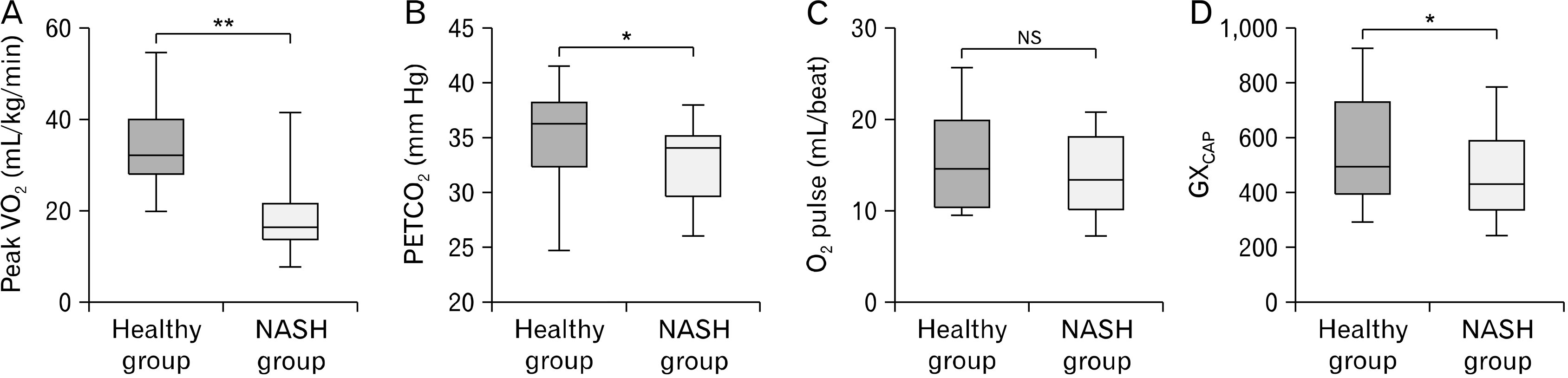
Table 1
Subject characteristic