Abstract
Background and Objectives
To examine whether ischemic retinal ganglion cells (RGCs) will be salvaged from cell death by human adipose-derived mesenchymal stem cells (ADSCs) in an organotypic retina model.
Methods and Results
Deprived of arterial oxygen supply, whole mice retinas were cultured as an ex vivo organotypic cultures on an insert membrane in a 24-well plate. The therapeutic potential of ADSCs was examined by co-culture with organotypic retinas. ADSCs were seeded on top of the RGCs allowing direct contact, or at the bottom of the well, sharing the same culture media and allowing a paracrine activity. The number of surviving RGCs was assessed using Brn3a staining and confocal microscopy. Cytokine secretion of ADSCs to medium was analyzed by cytokine array. When co-cultured with ADSCs, the number of surviving RGCs was similarly significantly higher in both treatment groups compared to controls. Analysis of ADSCs cytokines secretion profile, showed secretion of anti-apoptotic and pro-proliferative cytokines (threshold>1.4). Transplantation of ADSCs in a co-culture system with organotypic ischemic retinas resulted in RGCs recovery. Since there was no advantage to direct contact of ADSCs with RGCs, the beneficial effect seen may be related to paracrine activity of ADSCs.
Ischemia and hypoxia are the basis for the pathogenesis of the two main diseases that lead to irreversible vision loss: central retinal artery occlusion and ischemic optic neuropathy. Hypoxia can lead to neuronal and epithelial cell death or glial cell dysfunction in the retina (1).
Retinal ganglion cells (RGCs) are part of the inner retina and initiate the visual stimuli through axonal extensions to the optic nerve and to the brain (2). RGCs are sensitive to hypoxic damage known to be irreversible within a few hours (3). To date, there is no treatment to reverse the hypoxic damages to the RGCs from diseases such as ischemic optic neuropathy, or central artery occlusion.
Mesenchymal stem cells (MSC) therapeutic potential is broadly studied in neurodegenerative diseases research. In preclinical models of optic nerve neurodegeneration, transplantation of MSCs appears to attenuate neuronal death. The neuroprotective ability of MSCs has been already noted with bone marrow MSCs transplantation into the posterior vitreous cavity following ischemia (4).
MSCs are known with antioxidant and neurotrophic characteristics that support neuronal survival when transplanted to ectopic degenerative sites of CNS. In addition, MSCs have immunomodulatory properties and strong paracrine effects as they can secrete growth factors, neurotrophic factors, and cytokines as a response to external stimuli (5). Subcutaneous adipose tissue is a significant source for harvest of MSCs, since the harvest of fat can be conducted in a minimally invasive procedure, and yields abundant highly potent ADSCs (6).
Moreover, a recent study from our group showed a neuroprotective effect of ADSCs, with salvage of photoreceptors, when transplanted into subretinal space in a mice model of retinal degeneration. Furthermore, ADSCs’ conditioned medium (CM) exhibited a protective effect on retinal pigment epithelium (RPE) cells after H2O2 exposure (7).
Organotypic retina culture technique enables to examine retina’s function and cell-to-cell interaction ex vivo while maintaining the whole retina intact (8).
In this study we explored the therapeutic effect of ADSCs on ischemic RGCs, focusing on cell rescue from cell death, using a co-culture system of ADSCs with organotypic retinal explant. We examined the effect of ADSCs while seeded directly on RGCs, or their paracrine effect when sharing the same culture media with whole retina explant. Moreover, we studied the cytokines secretion profile of ADSCs by cytokine array.
All clinical procedures involving patients were in accordance with the declaration of Helsinki and approved by the TLVMC Institutional Review Board (IRB) (Helsinki Committee, approval number 920120440); Informed consent was obtained from all patients. The study protocol was adhered to the ARVO Statement for the Use of Animals in Ophthalmic and Vision Research.
Organotypic retinal explants were prepared as previously described (9). Briefly, adult male wild type C57Bl/6 mice were euthanized, and retinas were dissected from enucleated eyes. The retinas were transferred onto a cell culture insert with the RGC facing upwards. The insert was placed into 24-wells plate with a medium containing Neurobasal-A (Thermo-Fisher Scientific), B-27 (Invitrogen), N2 (Thermo-Fisher Scientific) and penicillin-streptomycin solution (Biological Industries) (Fig. 1; schematic diagram).
Adipose tissue was harvested from subcutaneous abdominal human fat from 2 patients undergoing abdominoplasty, with mean age of 70.46±10.69. No severe medical history was reported.
The isolation and culture of ADSCs was performed as previously described (9). Briefly, 60∼120 ml of lipoaspi-rates were enzymatically digested using 0.75% collagenase type I (C1639, Sigma-Aldrich, Germany), and centrifuged at 400-g for 15 minutes. 1×106 cells were plated with ADSC medium and incubated at 37℃, 8% CO2 upon reaching 90% confluency. Experiments were performed at passages 3∼4. Cells exhibited classical mesenchymal stem cell markers (positive to CD90, 105, 29, negative to CD34) (CD90: 100±1.98, CD73: 97±5.2, and CD105: 97.8±1.7% of population) as shown by immunostaining and Fluo-rescence-activated cell sorting (FACS) analysis (7).
Each retina explant was co-cultured with 20,000 ADSCs. To evaluate whether therapeutic effect achieved by direct contact or by a paracrine effect, the ADSCs were seeded on top of the retinas, directly on the RGCs layer (n=4) allowing direct contact, or on the tissue culture plate, below the insert with the retinas, sharing the same medium (n=3) (Fig. 1).
To examine the hypoxic damage to RGCs, organotypic retinas were analyzed at different time points (T0, T24, T48, T72 hours). Surviving RGCs was evaluated and quantified using immunohistochemistry staining of Brn3a, a known marker of RGCs viability (10).
Retinas were fixed using 4% paraformaldehyde (Sigma-Aldrich), before incubating retinas with anti-Brn3a antibody (Santa Cruz Biotech) at 4℃ for overnight, tissues were incubated with blocking solution (1% bovine serum albumin (Sigma-Aldrich), 5% normal goat serum (Thermo-Fisher Scientific). The Next day, tissues were incubated with secondary antibody (AF488 goat anti-mouse, Invitrogen, USA). The retinas were flat mounted and evaluated by LSM-700 confocal microscope (Zeiss).
The paracrine activity of ADSCs was studied by cytokines array on collected culture medium. ADSCs medium was replaced with serum-free medium and incubated for 48 h. the medium was then collected and centrifuged at 4,500 rpm for 5 min. supernatants were collected for analysis. Levels of cytokines and growth factors were measured using Ray-BioⓇ Human Cytokine Antibody Array G10 (Thermo-Fisher Scientific).
Studied groups of ADSCs seeded directly on retinas (n=4), ADSCs seeded on the plate below the retinas (n=3) were compared to controls comprising non treated retinas at T0 (n=4) and T48 (n=2). retinas at T0 (n=4) and at T48 (n=2), cultured and treated with CM with no ADSCs.
The average number of Brn3a positive cells, from 4 different areas, was taken for each examined retina.
Kruskal-Wallis test was conducted to examine differences in number of Brn3a positive cells between studied groups. A significant difference (p<0.001) was detected; then, we conducted post-hoc analysis tests to compare the groups placed above and below at T48 (p=0.001 for both).
To examine the possibility of a difference between T0 and the treatment groups, we also performed a Kruskal-Wallis test which compared the medians of the groups.
The organotypic retina is deprived of arterial blood supply, hence may use as a model for examining hypoxic effect on RGCs at different time points. We evaluated the surviving RGCs in hypoxic retinas at T0, T24, T48, and T72 hours post dissection, using Brn3a staining. We observed that the number of viable RGCs decreased in a time dependent manner, as shown in Fig. 2E (T0=160± 31, T24=72±5.8, T48=32±5.7, T72=0).
ADSCs seeded above or below the ischemic retinas at T48, lead to a significant increase in Brn3a positive cells compared to controls. No significant difference was found between the treatment groups suggesting that direct contact of ADSCs with RGCs did not contribute to the positive effect, but rather ADSCs paracrine activity. Interestingly, the number of viable RGCs at T48 was slightly higher in both treatment groups when compared to number of viable RGCs in controls at T0 (Fig. 3).
To further characterize the paracrine activity of ADSCs, we analyzed the cytokine secretion profile of ADSCs using Ray-bio–Antibody Array on ADSCs CM. We found that the most abundant cytokines secreted in all samples were Osteoprotegerin, TIMP-1 and TIMP-2 (Fig. 4) known for their anti-apoptotic and pro-proliferative traits (11-13). These data may explain the increase of Brn3a positive cells after ADSCs treatment compared to controls at 48 h as well as compared to control at T=0.
In this study, we examined the protective effect of ADSCs on RGCs survival in ischemic retinas in a co culture system with organotypic retinas. We demonstrated a time-dependent effect of hypoxia on RGCs viability. Next, we showed that treatment with ADSCs either by direct contact with RGCs or by paracrine activity resulted in salvage of RGCs from cell death. We also analyzed the cytokines secretion profile of CM of ADSCs and found it to be rich with anti-apoptotic and pro-proliferative cytokines.
We have found escalating damage to RGCs with time, evident by fewer viable Brn3a positive cells with increasing time from tissue dissection, with extensive death rate at 48 h and almost absolute cell death at 72 h. These data are consistent with the irreversible damage to vision in retinal and optic nerve ischemic diseases that are observed in humans (14). As most patients will need medical help at around 48 h from onset of symptoms (15), our model of treatment at 48 h is in line with clinical setting demands. Moreover, treatment with ADSCs resulted in rescue of ischemic RGCs even after 48 h. Considering these data, ADSCs may serve as a potential cell therapy module in the treatment of dying RGCs in glaucoma.
ADSCs can serve as an origin of antioxidants, free radical scavengers and heat shock proteins at the site of tissue insult (16). We found that direct contact and paracrine activity resulted in salvage of RGC with no significant preference for either method, implying to a paracrine activity that led to salvage of RGCs rather than direct contact of ADSCs. These data are compatible with our analysis in this study of ADSCs CM that was shown to be enriched with anti-apoptotic and pro-proliferative cytokines.
It was previously shown that ADSCs secrete neurotrophic factors such BDNF and HGF (7). In this study, we found that the most abundant proteins secreted in all samples were Osteoprotegerin (OPG) and TIMP-1/2.
It has been reported that OPG binding to TNF-related apoptosis-inducing ligand (TRAIL) inhibits TRAIL/TRAIL-Receptors interaction, as revealed by the inhibition of TRAIL-induced apoptosis. OPG also known as a receptor activator of nuclear factor-kappaB ligand (RANKL)/RANK. Enhanced RANKL/RANK signaling showed neuroprotective effects with reduced expression in inflamma-tory cytokines and may potentially decrease inflammation through a Toll-like receptor signaling pathway in microglia (17).
TIMP-1and TIMP-2 are highly secreted by ADSCs and known for its anti-apoptotic properties in ischemic brain injury and retinal degeneration (11). Thus the secretion of OPG and TIMP by ADSCs may suggest a potential mechanism of action in salvage of ischemic RGCs when co cultured with ADSCs. A future study should examine whether or not blockage of this secretion will atlter the therapeutic effect seen here. Another exciting aim to explore in future study is the secretion profile of extracellular vesicles derived from ADSC and its effect in a co culture with organotypic retinas.
We observed that after treatment with ADSCs, the number of viable RGCs was higher at T48 than at T0. This implies that treatment with ADSCs may have contributed to RGCs proliferation, a fact that may be explained by the secretion of pro-proliferative cytokines we have found in ADSC’s CM. This finding could possibly explained by Müller cells conversion to RGCs converted, as a response to ischemic insult, and also expressed Brn3a. Wei et al. (18) previously showed that retinal neuronal identity switching, triggered migration of amacrine interneurons from the inner nuclear layer to the RGCs layer which may imply that neurons exhibit surprisingly unexpected plasticity, which could result in regenerative manifestations.
To the best of our knowledge, this is the first study to examine the effect of MSCs harvested from subcutaneous adipose tissue on ischemic organotypic retinas. This population of stem cells is attractive for future development of autologous cell therapy due to their accessibility and potency (6). Moreover, since adipose tissue has a relatively low oxygen supply, MSCs isolated from adipose tissue are more resilient to ischemic conditions and thus, may be more suitable as a therapeutic tool in ischemic settings (19).
In conclusion, this study showed that transplantation of ADSCs significantly improved recovery of RGCs in ischemic retinas, probably via paracrine pathways, and suggest the potential of using these cells in future studies of regenerative therapy for ischemic retinas and ischemic RGCs.
We acknowledge several limitations to our study. First, the insufficient size of the cohort, and we did not include a study group in which only CM from ADSCs was used to treat ischemic retinas. These points are beyond the scope of this study and may be a subject for future research.
References
1. Olivares-González L, Martínez-Fernández de la Cámara C, Hervás D, Marín MP, Lahoz A, Millán JM, Rodrigo R. 2016; cGMP-phosphodiesterase inhibition prevents hypoxia-induced cell death activation in porcine retinal explants. PLoS One. 11:e0166717. DOI: 10.1371/journal.pone.0166717. PMID: 27861632. PMCID: PMC5115799. PMID: cf42187bb8aa4a45ae87ea861036b353.


2. Mead B, Tomarev S. 2016; Evaluating retinal ganglion cell loss and dysfunction. Exp Eye Res. 151:96–106. DOI: 10.1016/j.exer.2016.08.006. PMID: 27523467. PMCID: PMC5045805.


3. Klemm P, Hurst J, Dias Blak M, Herrmann T, Melchinger M, Bartz-Schmidt KU, Zeck G, Schultheiss M, Spitzer MS, Schnichels S. 2019; Hypothermia protects retinal ganglion cells against hypoxia-induced cell death in a retina organ culture model. Clin Exp Ophthalmol. 47:1043–1054. DOI: 10.1111/ceo.13565. PMID: 31152487.


4. Li N, Li XR, Yuan JQ. 2009; Effects of bone-marrow mesenchymal stem cells transplanted into vitreous cavity of rat injured by ischemia/reperfusion. Graefes Arch Clin Exp Ophthalmol. 247:503–514. DOI: 10.1007/s00417-008-1009-y. PMID: 19084985.


5. Baraniak PR, McDevitt TC. 2010; Stem cell paracrine actions and tissue regeneration. Regen Med. 5:121–143. DOI: 10.2217/rme.09.74. PMID: 20017699. PMCID: PMC2833273.


6. Barzelay A, Levy R, Kohn E, Sella M, Shani N, Meilik B, Entin-Meer M, Gur E, Loewenstein A, Barak A. 2015; Power-assisted liposuction versus tissue resection for the isolation of adipose tissue-derived mesenchymal stem cells: phenotype, senescence, and multipotency at advanced passages. Aesthet Surg J. 35:NP230–NP240. DOI: 10.1093/asj/sjv055. PMID: 26319084.


7. Barzelay A, Weisthal Algor S, Niztan A, Katz S, Benhamou M, Nakdimon I, Azmon N, Gozlan S, Mezad-Koursh D, Neudorfer M, Goldstein M, Meilik B, Loewenstein A, Barak A. 2018; Adipose-derived mesenchymal stem cells migrate and rescue RPE in the setting of oxidative stress. Stem Cells Int. 2018:9682856. DOI: 10.1155/2018/9682856. PMID: 30651740. PMCID: PMC6311721. PMID: 5e93dd85401b4290929c3bb39a1a050d.


8. Müller B. 2019; Organotypic culture of adult mouse retina. Methods Mol Biol. 1940:181–191. DOI: 10.1007/978-1-4939-9086-3_13. PMID: 30788826.


9. Wang F. 2006; Culture of animal cells: a manual of basic technique, fifth edition. In Vitro Cell Dev Biol Anim. 42:169. DOI: 10.1290/BR090501.1.


10. Nadal-Nicolás FM, Jiménez-López M, Sobrado-Calvo P, Nieto-López L, Cánovas-Martínez I, Salinas-Navarro M, Vidal-Sanz M, Agudo M. 2009; Brn3a as a marker of retinal ganglion cells: qualitative and quantitative time course studies in naive and optic nerve-injured retinas. Invest Ophthalmol Vis Sci. 50:3860–3868. DOI: 10.1167/iovs.08-3267. PMID: 19264888.


11. Boudreau N, Sympson CJ, Werb Z, Bissell MJ. 1995; Suppression of ICE and apoptosis in mammary epithelial cells by extracellular matrix. Science. 267:891–893. DOI: 10.1126/science.7531366. PMID: 7531366. PMCID: PMC3004777.


12. Tejima E, Guo S, Murata Y, Arai K, Lok J, van Leyen K, Rosell A, Wang X, Lo EH. 2009; Neuroprotective effects of overexpressing tissue inhibitor of metalloproteinase TIMP-1. J Neurotrauma. 26:1935–1941. DOI: 10.1089/neu.2009.0959. PMID: 19469687. PMCID: PMC2822804.


13. Zhang P, Li J, Qi Y, Tang X, Duan J, Liu L, Wu Z, Liang J, Li J, Wang X, Zeng G, Liu H. 2016; Tissue inhibitor of matrix metalloproteinases-1 knockdown suppresses the prolifera-tion of human adipose-derived stem cells. Stem Cells Int. 2016:4761507. DOI: 10.1155/2016/4761507. PMID: 27239203. PMCID: PMC4863124. PMID: 69e3683105534e469b7513d5b969e040.


14. Brown SM, Lark KK. 2019; Re: Biousse et al.: Management of acute retinal ischemia: follow the guidelines! (Ophthal-mology. 2018;125:1597-1607). Ophthalmology. 126:e36–e37. DOI: 10.1016/j.ophtha.2018.11.032. PMID: 31005195.
15. Chen CS, Lee AW. 2008; Management of acute central retinal artery occlusion. Nat Clin Pract Neurol. 4:376–383. DOI: 10.1038/ncpneuro0811. PMID: 18542123.


16. Gimble JM, Katz AJ, Bunnell BA. 2007; Adipose-derived stem cells for regenerative medicine. Circ Res. 100:1249–1260. DOI: 10.1161/01.RES.0000265074.83288.09. PMID: 17495232. PMCID: PMC5679280.


17. Shimamura M, Nakagami H, Osako MK, Kurinami H, Koriyama H, Zhengda P, Tomioka H, Tenma A, Wakayama K, Morishita R. 2014; OPG/RANKL/RANK axis is a critical inflammatory signaling system in ischemic brain in mice. Proc Natl Acad Sci U S A. 111:8191–8196. DOI: 10.1073/pnas.1400544111. PMID: 24847069. PMCID: PMC4050556.


18. Wei X, Zhang Z, Zeng HH, Wang XF, Zhan W, Qiao N, Chang Z, Liu L, Fan C, Yang Z, Li X, Yang Y, Liu H. 2020. Regeneration of functional retinal ganglion cells by neuronal identity reprogramming. bioRxiv 203497 [Preprint]. Available from: https://doi.org/10.1101/2020.07.16.203497. cited 2020 Jul 16. DOI: 10.1101/2020.07.16.203497.


19. Feng Y, Zhu M, Dangelmajer S, Lee YM, Wijesekera O, Castellanos CX, Denduluri A, Chaichana KL, Li Q, Zhang H, Levchenko A, Guerrero-Cazares H, Quiñones-Hinojosa A. 2014; Hypoxia-cultured human adipose-derived mesenchymal stem cells are non-oncogenic and have enhanced viability, motility, and tropism to brain cancer. Cell Death Dis. 5:e1567. Erratum in: Cell Death Dis 2015;6:e1797. DOI: 10.1038/cddis.2015.176. PMID: 26111059. PMCID: PMC4669846.


Fig. 1
Schematic diagram of the study. Two co-culture techniques of organotypic retinas with ADSCs. (A) ADSCs seeded on 24-well-plate sharing the same culture media with the organotypic retina, for studying the cells’ paracrine effect. (B) ADSCs seeded on top of the retina with direct contact with RGCs for studying the cell’s direct contact effect. Blue is the tissue culture 24-well-plate. Black is the insert. Red is the retina. Green are ADSCs.
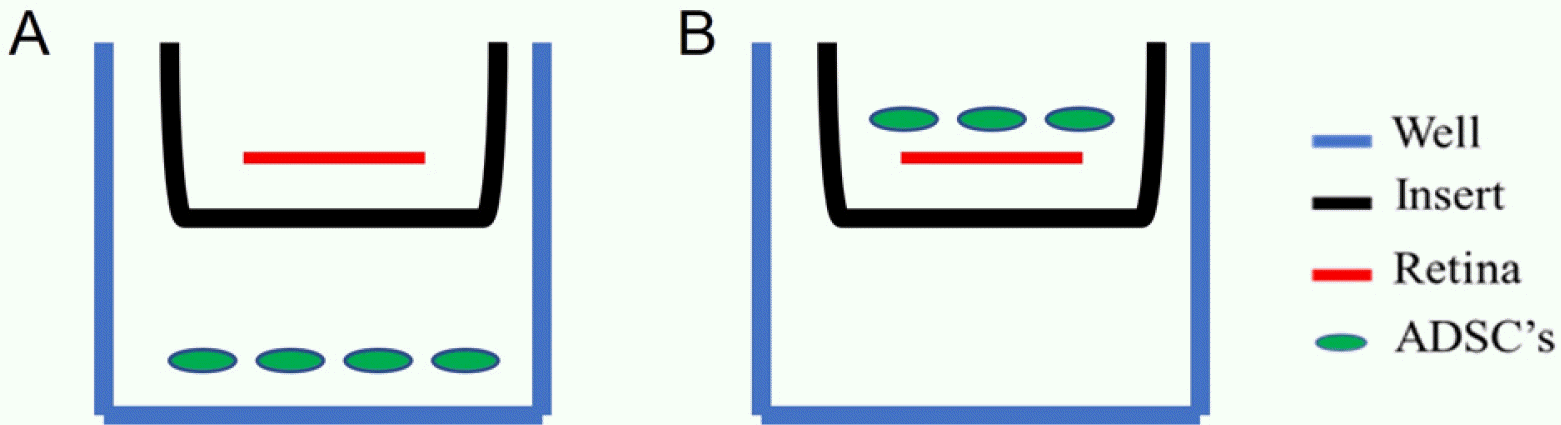
Fig. 2
Time depended effect of hypoxia on RGCs viability. Retinas were extracted and cultured as organotypic culture for different periods of times. Viability of RGCs was evaluated by staining for Brn3a. Repre-sentative pictures of retinas stained for Brn3a at (A) T0, (B) T24 hours, (C) T48 hours, (D) T72 hours. (E) Summary of time-dependent effect of hypoxic conditions on viability of RGCs. Bar=100 μm. Data are represented as mean±SEM. The experiment was conducted on 2 to 5 retinas per time point.
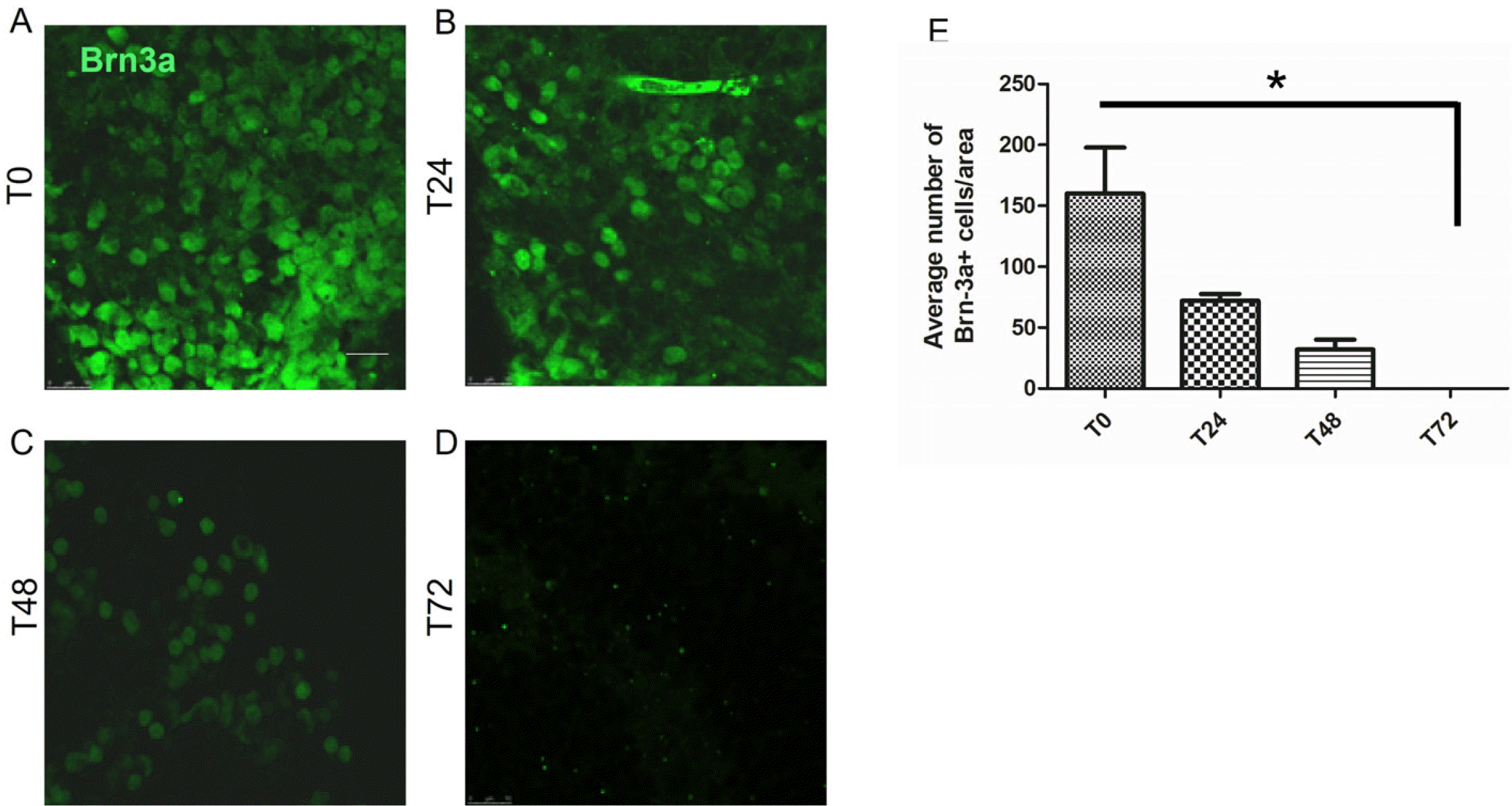
Fig. 3
Representative picture of Brn3a staining at 48 hours. (A) Control organotypic retinas cultured in same conditions without ADSCs. (B) Retinas treated with ADSCs seeded with direct contact on top of the RGCs. (C) Retinas treated with ADSCs seeded on tissue culture plate below the insert. Graphic summary of ADSCs regenerative potential at 48 hours. (D) Retinas treated with ADSCs on top of the retina and below the retina exhibited significantly more viable Brn3a+RGCs as compared to controls of retinas cultured for 48 hours with no treatment. Bar=100 μm. Data are represented as mean± SEM.
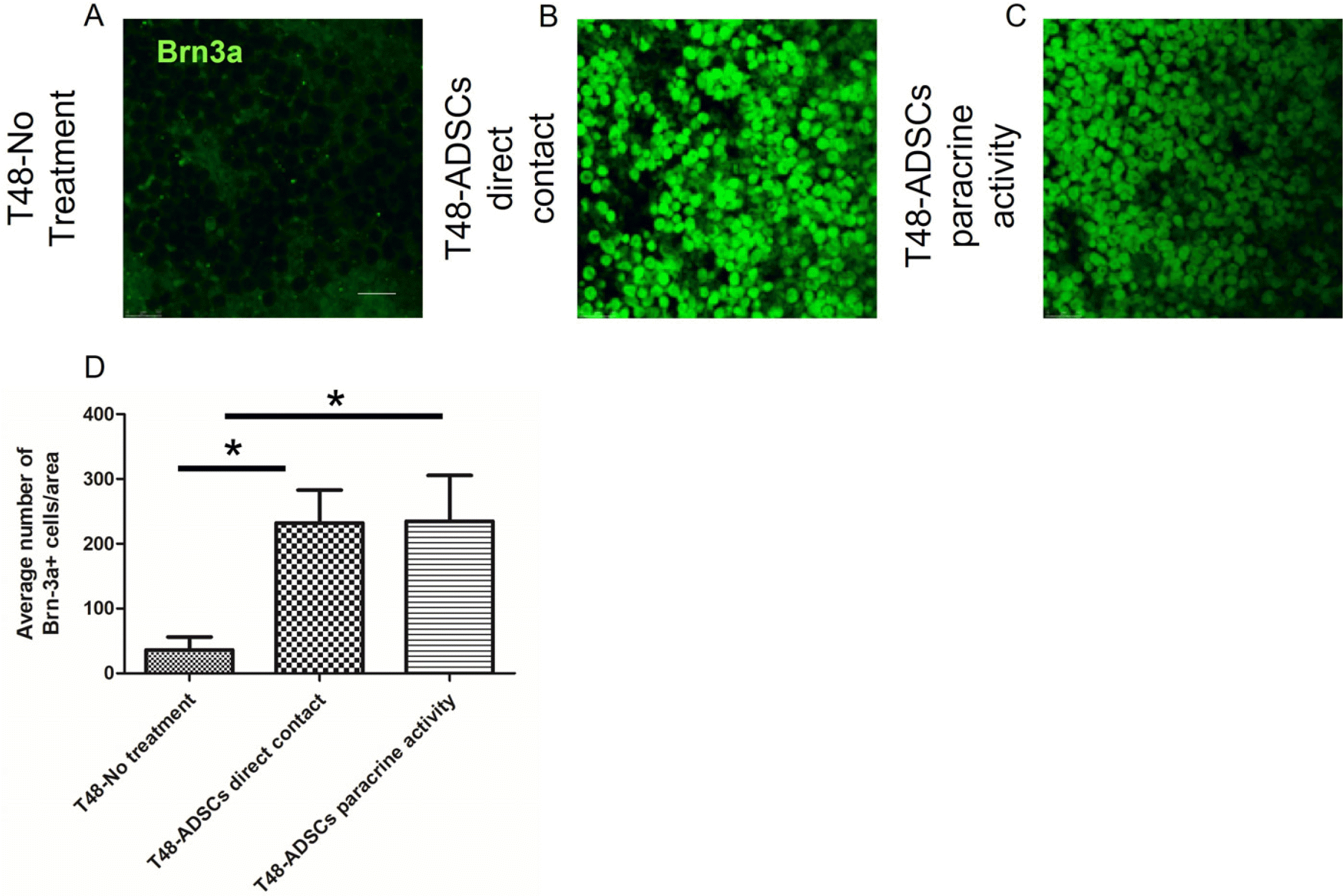