Abstract
Objective
The heart contains a pool of c-kit+ progenitor cells which is believed to be able to rege-nerate. The differentiation of these progenitor cells is reliant on different physiological cues. Unraveling the underlying signals to direct differentiation of progenitor cells will be beneficial in controlling progenitor cell fate. In this regard, the role of the mitochondria in mediating cardiac progenitor cell fate remains unclear. Specifically, the association between changes in mitochondrial morphology with the differentiation status of c-kit+ CPCs remains elusive. In this study, we investigated the relationship between mitochondrial morphology and the differentiation status of c-kit+ progenitor cells.
Methods and Results
c-kit+ CPCs were isolated from 2-month-old male wild-type FVB mice. To activate differentiation, CPCs were incubated in α-minimal essential medium containing 10 nM dexamethasone for up to 7 days. To inhibit Drp1-mediated mitochondrial fragmentation, either 10 μM or 50 μM mdivi-1 was administered once at Day 0 and again at Day 2 of differentiation. To inhibit calcineurin, either 1 μM or 5 μM ciclosporin-A (CsA) was administered once at Day 0 and again at Day 2 of differentiation. Dexamethasone-induced differentiation of c-kit+ progenitor cells is aligned with fragmentation of the mitochondria via a calcineurin-Drp1 pathway. Pharmacologically inhibiting mitochondrial fragmentation retains the undifferentiated state of the c-kit+ progenitor cells.
The heart contains a heterogenous pool of cardiac progenitor cells (CPCs). These different populations of CPCs, such as the c-kit+ (1) and Sca1+ cells (2) have previously been demonstrated to exert a role in cardiac growth, development, and repair. c-kit+ cells functioning as cardiovas-cular precursors have been detected in adult human hearts undergoing hypertrophy, post-myocardial infarction and post-ischemia/reperfusion injury, albeit the number of the c-kit+ cells may differ depending on the cardiac condition (3) and origins in the different heart chambers (4). Infusion of CPCs into the injured myocardium has been demonstrated to result in cardiac restoration and improved function (5). Yet lineage tracing studies to track the differenti-ation of c-kit+ cells into cardiomyocytes have been contradic-ting, depending on the experimental system employed (6). Irrespective of the contradictory findings, studies conducted in general have suggested that c-kit+ CPCs can be found in the hearts of different animals ranging from the mice, pig, and sheep to the human heart at different developing stages (7). Similarly, regardless of whether the beneficial effects of CPCs infusion may purely be due to invoking certain indirect paracrine mechanisms (8), c-kit+ cells exert a certain level of role in functional cardiac regeneration and repair (9).
Recent studies conducted have alluded to the role of mitochondria, particularly the influence of mitochondrial dynamics in the differentiation process of induced pluripo-tent stem cells (iPSCs) (10) and the embryonic stem cells (ESCs) (11) whereby mitochondrial fusion, in general, has been demonstrated to be crucial for stem cell differen-tiation. The elongation of the mitochondria coincides with the development of cristae, reflecting increased mitochondrial activity in the differentiated cell (12). In the quiescent adult cell, the mitochondria exist in a dynamic equilibrium between an elongated (via mitochondrial fusion) versus a fragmented (via mitochondrial fission) phenotype (13, 14). The fusion of individual mitochondrions is governed by pro-fusion proteins such as Mitofusins 1 or 2 (Mfn1 or 2) positioned on the outer mitochondrial membrane (OMM) (15) and Optic Atrophy 1 (OPA1) on the inner mitochondrial membrane (IMM) (16). The fragmentation of the mitochondrion depends on the Dynamin-related Protein 1 (Drp1), which translocates from the cytosol to the mito-chondrion upon activation by calcineurin – a calcium and calmodulin dependent serine/threonine protein phosphatase (17). The change in mitochondrial morphology is affected by the physiological environment in which different cues such as intracellular calcium or ROS levels may tilt the mitochondrial morphology towards a fused or fragmented state. Similarly, the removal of dysfunctional mitochondria via autophagy/mitophagy or a shift in cellular metabolism affects the fusion and fission process of the mitochondria which ultimately dictate cellular fate.
In this study, we investigated the changes in mitochondrial morphology in the dexamethasone-mediated differentiation process of c-kit+ CPCs. We discovered that mitochondrial fragmentation precedes the differentiation of cardiac c-kit+ CPCs and inhibition of mitochondrial fragmentation abrogates the differentiation status of c-kit+ CPCs. In this regard, our observations highlight a potential role of mitochondrial morphology in the differentiation of cardiac c-kit+ CPCs, whereby pharmacological modulation of mitochondrial morphology may dictate cardiac progenitor cell fate in the heart.
All experimental procedures were performed in accordance with institutional guidelines and approved by the Ani-mal Experimentation Ethics Committee of the Chinese Uni-versity of Hong Kong. c-kit+ CPCs were isolated from 2-month-old male wild-type FVB mice. All c-kit+ adult CPCs were isolated and cultured as previously described (18). To activate differentiation, CPCs were incubated in α-mini-mal essential medium containing 10 nM dexamethasone for up to 7 days (19). To inhibit Drp1-mediated mitochondrial fragmentation, either 10 μM or 50 μM mdivi-1 (Sigma) was administered once at Day 0 and again at Day 2 of differentiation (20). To inhibit calcineurin, either 1 μM or 5 μM ciclosporin-A (CsA) (Sigma) was administered once at Day 0 and again at Day 2 of differentiation (17).
Cells were fixed, permeabilized, and blocked as previously described (19). CPCs were stained with antibodies against Tom20 (Santa Cruz Biotechnology), COXIV (Invi-trogen), and GATA-4 (Santa Cruz Biotechnology). Cells were incubated with Alexa Fluor 488 or 594 secondary antibodies (Life Technologies) followed by Hoechst 33342 (10 μg/ml, Life Technologies) to stain nuclei. Cells were imaged using a Carl Zeiss Axio Observer Z1, and Z-stacks were acquired using a high resolution AxioCam MRm digital camera, a 63× oil immersion objective, and Zeiss Axio-Vision 4.8 software (Carl Zeiss). The number of mitochondria within each image and the average size of each mitochondrion (total mitochondrial length over the total number of mitochondria) was quantified using ImageJ. A minimum of 80 cells per group were counted for each condition.
Samples were prepared in lysis buffer containing 50 mM Tris-HCl, 150 mM NaCl, 1 mM EGTA, 1 mM EDTA, 1% Triton X-100, and protease inhibitor mixture (Roche Applied Science) and run on Invitrogen NuPAGE Bis-Tris gels. The membranes were probed with the following antibodies: MitoProfile Total OXPHOS Rodent WB antibody mixture (MitoSciences), Opa1 (Abcam), Mfn1 (Santa Cruz Biotechnology), Mfn2 (Sigma), Drp1 (BD Biosciences), Fis1 (Sigma), Tubulin (Sigma), Drp1 (phospho S637) (Abcam). Membranes were imaged using a ChemiDoc XRS+ System (Bio-Rad).
The calcineurin phosphatase activity was measured by the dephosphorylation rate of a synthetic phosphopeptide substrate with a calcineurin assay kit (Enzo Life Sciences, Plymouth Meeting, Pa, USA) following the manufacturer’s instructions. The released free phosphate was then detected colorimetrically with the green reagent on a plate reader at 620 nm.
Incubation of c-kit+ CPCs with dexamethasone for a period of 7 days activates the differentiation process as evidenced by the loss of c-kit staining (Fig. 1A) and appearance of the cardiac myocyte lineage marker GATA-4 at Day 7 post-dexamethasone treatment (Fig. 1B). The 7 days of dexamethasone treatment also increases the expression of the mitochondrial respiratory subunits (Fig. 1C).
In addition to the loss of c-kit and the appearance of GATA-4 following differentiation, we observed a signifi-cant increase in the proportion of c-kit+ CPCs with fragmented mitochondria (Fig. 2A). In addition, the number of individual mitochondrions was significantly increased (Fig. 2B), whilst the average mitochondrial size was significantly reduced (Fig. 2C) following dexamethasone-mediated differentiation for 7 days. The fragmentation of the mito-c-hondria started as early as Day 2 following dexametha-sone treatment (Fig. 2D).
We next assessed the mitochondrial-shaping protein responsible for mediating the shift in mitochondrial morphology to a fragmented state during the differentiation process. We observed that the level of Drp1 was signifi-cantly upregulated at Day 7 post-differentiation (Fig. 3A). This increase in Drp1 protein expression started as early as Day 2 following dexamethasone treatment (Fig. 3B) whilst de-phosphorylation of Drp1 at the site of Ser637 (S637) occurred on both Days 2 and 4 post-dexamethasone treatment (Fig. 3C). As Drp1 activation is known to be mediated upstream by calcineurin, we employed the use of a calcineurin activity assay kit to determine calcineurin activity (Fig. 3D). We found that the calcineurin activity was increased significantly at Day 2 post-dexamethasone treat-ment. The calcineurin activity increased further at Day 4, albeit not significantly compared to Day 2. At Day 7, the calcineurin activity reduced to a level which was almost similar to Day 0.
As the fragmentation of the mitochondria correlates with an increase in total Drp1 levels, we investigated whether inhibiting Drp1 affects the differentiation of c-kit+ CPCs. We subjected the cells to 2 different doses of mdivi-1 −10 μM or 50 uM; once at Day 0 and again at Day 2. We found that the presence of 50 μM mdivi-1 significantly reduced the proportion of c-kit+ cells with predominantly fragmented mitochondria following 7 days of dexamethasone treatment (Fig. 4A and 4B). The proportion of GATA-4-positive c-kit+ CPCs at Day 7 were also significantly reduced following the 50 μM mdivi-1 treatment (Fig. 4A and 4C). 10 μM mdivi-1, however, failed to inhibit mitochondrial fragmentation and reduce the number of GATA-4 positive cells.
Elevated calcium has been known to activate calcineurin leading to de-phosphorylation of Drp1 and subsequent translocation from the cytosol to the mitochondria for execution of mitochondrial fragmentation. We sought to investigate whether modulating calcineurin upstream of Drp1 via CsA at 2 different doses (1 μM or 5 μM) impedes the differentiation of c-kit+ CPCs. Inhibition of calcineu-rin using 5 μM CsA but not 1 μM CsA, significantly reduced the proportion of c-kit+ CPCs with fragmented mitochondria (Fig. 5A and 5B). Consequently, the propo-rtion of GATA-4-positive c-kit+ CPCs was also signifi-cantly reduced by 5 μM CsA as opposed to using 1 μM CsA (Fig. 5A and 5C).
The study conducted here demonstrated that dexame-thasone-induced differentiation of c-kit+ CPCs is aligned with Drp1-mediated mitochondrial fragmentation. Inhibition of Drp1 using the small molecule inhibitor of Drp1 – mdivi-1 or upstream calcineurin via CsA impairs the differentiation process. Thus, our findings allude to the impor-tance of mitochondrial fragmentation in the dexamethasone-mediated differentiation process of cardiac c-kit+ CPCs.
Our study utilised dexamethasone for a total of 7 days to induce differentiation according to previous literature (19). Although not included in this study, we have also demonstrated that the dexamethasone-mediated differentiation process of c-kit+ CPCs led to upregulation of other cardiac lineage markers such as GATA-6, MEF2C, as well as enhanced mitochondrial oxygen consumption rates, consistent with our previous study (19). In the current study, dexamethasone-induced differentiation of c-kit+ CPCs promoted a reduction in levels of c-kit expression, an increase in GATA-4 expression aligned with Drp1-mediated fragmentation of the mitochondria. Our results are consistent with the findings of other studies whereby the use of pharmacological nitric oxide (NO) donors to increase mitochondrial numbers promotes maturation of fast-dividing mesoangioblasts into cardiomyocytes whilst decreasing mitochondrial content using respiratory chain inhibitors and chloramphenicol perturbs cardiomyocyte differentiation in slow-dividing populations (21). Rounding of the mitochondria was also observed in the aggregation stage of P19 cells differentiation into cardiomyocytes (22). Knock-down of Drp1 negatively influences 30-days neurogenesis of ESCs, coincident with a delayed reduction of Oct4 and Nanog during the mid-differentiation process (23). None-theless, the consensus derived from studies involving iPSCs and ESCs is that mitochondria in stem cells are immature, perinuclear-localized, fragmented, with fewer cristae (24). Upon differentiation to terminal cell types, the mitochondria become enlarged, elongated, and tubular concomitant with an increase in mitochondrial content (24). A low mito-chondrial mass has been associated with the undifferen-tiated and rapidly dividing state of ESCs and iPSCs (25, 26). Lowering MFN2 and OPA1 levels by gene trapping impaired differentiation of ESCs into beating cardiom-yocytes (26). Nuclear reprogramming by the stemness transcription factors - OCT3/4, SOX2, KLF4, and c-MYC restructured the mature tubular and cristae-rich somatic mitochondria in mouse embryonic fibroblasts (MEF) into immature spherical and cristae-poor structures in iPSC (27). The presence of these stemness transcription factors induced an increase in phosphorylation of ERK1/2 and DRP1-S579, thus driving Drp1-mediated mitochondrial fragmentation (28). Administration of 50 μM mdivi-1 on day 3 before the appearance of iPSC colonies in MEFs transduced with individual lentiviruses containing OCT4, SOX2, and KLF4 was sufficient to reduce somatic cell reprogramming by >95% 14 days post-viral transduction (25). Mfn2 has also been demonstrated to regulate mammalian postnatal male germ cell development by modulating both mitochondrial and ER functions – a process which is distinct from Mfn1 which only regulates mitochondrial fusion during neonatal pro-spermatogonia (29). A comparison between these different studies alluded to the fact that the discrepancy in the observation of mitochondrial fusion-fission during differentiation may be due to different confounding factors such as the source of progenitor cells, different agents used for induction of differentiation, contrasting differentiation markers, varying di-fferentiation durations, and desired terminal cell types.
Although the level of intracellular calcium (Ca2+) was not investigated in this study, electrical stimulation in human c-kit+ CPCs has been found to activate a Ca2+-dependent signaling pathway in which the increased intracellular Ca2+ activates the transcription of cardiospecific genes and proper alignment of the cells in a tissue-like structure, albeit a direct investigation into the lineage fate was not examined (30). An increase in free intracellular Ca2+ in canine c-kit+ CPCs revealed a prominent inwardly rectifying current identified as the intermediate conductance Ca2+‐ activated K+ current (KCa3.1) that activates progenitor cell proliferation, although this was not extrapolated to the differentiation of c-kit+ CPCs (31). During cardiomyogenesis, activation of the TGFβ receptor regulates TAK, which then acts on the transcription factors ATF2 and CREB through the Ca2+ responsive MAPK pathway (32). In addition, adipogenic differentiation mediated by a combination of dexamethasone, indomethacin, and insulin in human umbilical cord blood-derived MSCs (hUCB-MSCs) has been found to be enhanced by Ca2+ via negative regulation of the Wnt5a/β-catenin signaling pathway (33).
Downstream of the increase in intracellular calcium, calcineurin has been established to be a Drp1-activator (34). Calcineurin activated in a Ca2+-calmodulin dependent fashion dephosphorylates Drp1 at Ser637, thus mediating the translocation of Drp1 from the cytosol to the mitochondria for activation of the scission process (17). We found that inhibition of calcineurin via CsA abrogates mitochondrial fragmentation and impairs the differentiation process of c-kit+ cells. Mirroring our results, the calcineu-rin-NFAT signaling has also been demonstrated to converge on the Erk1/2 pathway to regulate Src expression, which is crucial for lineage specification in response to varying differentiation stimuli such as LIF-withdrawal or retinoic acid addition in mouse ESCs (mESC) (35). This finding was further supported by the finding that the alternative splicing regulator MBNL1 promotes generation of the atypical calcineurin Aβ variant CnAβ1, which is localized to the Golgi apparatus and regulates the intracellular localization and activation of the mTORC2 complex, thus driving differentiation in mESCs (36). Calcineurin signaling has also been associated with transforming growth factor (TGF)-β1, which drives the differentiation of neural crest stem cells (NCSCs) into smooth muscle (37).
There are certain limitations in our study, one of which is that our differentiation process was not extended beyond 7 days. Our current protocol of dexamethasone treatment for 7 days is sufficient to induce differentiation of c-kit+ CPCs as evidenced by the expression of different cardiac lineage markers (19), in line with the findings of other previous studies (38). Yet, the differentiation process in certain studies involving iPSC-CMs and ESC-CMs had an extended duration until approximately 12∼15 weeks, whereby the structural, electrophysiological, and mechanical properties of these late stage cells exhibit a higher similarity to that of an adult cardiomyocyte (39), although it should be noted that the maturation of the mitochondria remains inadequate and suboptimal compared to fetal development despite the increase in relative mitochondrial abundance or mass following extended culture (39).
Apart from dexamethasone, the differentiation process of c-kit+ CPCs can also be initiated by different cues such as (i) TGF-β, which has been shown to result in differentiation to myocytes, (ii) dexamethasone and VEGF for differentiation into endothelial cells and (iii) dexame-thasone together with growth factor PDGF BB or all-trans retinoic acid for differentiation into smooth muscle cells. Furthermore, the detected transcription factors for the formation of either myocytes, endothelial cells, or smooth muscle cells may also explain the varying levels of differentiation observed in different studies (40). In addition, studying the electrical activity of the differentiated cells will reveal more about the physiological attributes of the cells (30). Calcineurin inhibition using alternative CsA analog such as FK506 (Tacrolimus) or CN585 should be performed in future to confirm the association of calcineurin-mediated de-phosphorylation of Drp1 to induce mitochondrial fragmentation as there are calcineurin-independent effects of CsA which includes the cyclophilins (41, 42), cardiac actin expression (43), mitochondrial Ca2+ homeostasis (44), ROS elevation (45), ER stress (46) and even TGF-beta production (47). Pharmacologically inhibiting Drp1-mediated mitochondrial fragmentation by other alternatives such as P110 or genetic silencing of Drp1 will also help in further validating the results as mdivi-1 may have other Drp1-independent off-target effects such as inhibition of mitochondrial respiratory Com-plex I (48). Comparing the c-kit+ CPCs isolated from other strains of mice such as the heterozygous Drp1-KO mice will also allow further investigation into the relevance of Drp1-mediated mitochondrial fragmentation in the differentiation process of c-kit+ CPCs. Similarly, whether other pro-fission regulators such as Mff or MiD49/51 exert a role in the differentiation of c-kit+ CPCs remains to be determined.
Taken together, the findings from this study highlight the pertinence of mitochondrial morphology in differentiation status of progenitor cells and potentiates pharmacological manipulation of the mitochondria in controlling progenitor cells fate. This is particularly important in the wake of translating progenitor cell research in the fields of regenerative and cardiovascular medicine whereby an optimal spatial positioning and proper temporal differentiation of progenitor cell is crucial.
References
1. Fischer KM, Cottage CT, Wu W, Din S, Gude NA, Avitabile D, Quijada P, Collins BL, Fransioli J, Sussman MA. 2009; Enhancement of myocardial regeneration through genetic engineering of cardiac progenitor cells expressing Pim-1 kinase. Circulation. 120:2077–2087. DOI: 10.1161/CIRCULATIONAHA.109.884403. PMID: 19901187. PMCID: PMC2787902.


2. Wang X, Hu Q, Nakamura Y, Lee J, Zhang G, From AH, Zhang J. 2006; The role of the sca-1+/CD31-cardiac progenitor cell population in postinfarction left ventricular remode-ling. Stem Cells. 24:1779–1788. DOI: 10.1634/stemcells.2005-0386. PMID: 16614004.


3. Li S, Guo K, Wu J, Guo Z, Li A. 2017; Altered expression of c-kit and nanog in a rat model of Adriamycin-induced chronic heart failure. Am J Cardiovasc Dis. 7:57–63.
4. Zakharova L, Nural-Guvener H, Nimlos J, Popovic S, Gaballa MA. 2013; Chronic heart failure is associated with transforming growth factor beta-dependent yield and functional decline in atrial explant-derived c-Kit+ cells. J Am Heart Assoc. 2:e000317. DOI: 10.1161/JAHA.113.000317. PMID: 24088507. PMCID: PMC3835238.


5. Zakharova L, Nural-Guvener H, Feehery L, Popovic-Sljukic S, Gaballa MA. 2015; Transplantation of epigenetically modified adult cardiac c-Kit+ cells retards remodeling and improves cardiac function in ischemic heart failure model. Stem Cells Transl Med. 4:1086–1096. DOI: 10.5966/sctm.2014-0290. PMID: 26240433. PMCID: PMC4542868.


6. Liu Q, Yang R, Huang X, Zhang H, He L, Zhang L, Tian X, Nie Y, Hu S, Yan Y, Zhang L, Qiao Z, Wang QD, Lui KO, Zhou B. 2016; Genetic lineage tracing identifies in situ Kit-expressing cardiomyocytes. Cell Res. 26:119–130. DOI: 10.1038/cr.2015.143. PMID: 26634606. PMCID: PMC4816131.


7. Castaldo C, Di Meglio F, Nurzynska D, Romano G, Maiello C, Bancone C, Müller P, Böhm M, Cotrufo M, Montagnani S. 2008; CD117-positive cells in adult human heart are localized in the subepicardium, and their activation is associated with laminin-1 and alpha6 integrin expression. Stem Cells. 26:1723–1731. DOI: 10.1634/stemcells.2007-0732. PMID: 18436868.
8. Wang X, Li Q, Hu Q, Suntharalingam P, From AH, Zhang J. 2014; Intra-myocardial injection of both growth factors and heart derived Sca-1+/CD31-cells attenuates post-MI LV remodeling more than does cell transplantation alone: neither intervention enhances functionally significant cardiomyocyte re-generation. PLoS One. 9:e95247. DOI: 10.1371/journal.pone.0095247. PMID: 24919180. PMCID: PMC4053321. PMID: 3d821a02a7b1403bb4406eb707c63445.
9. Ellison GM, Vicinanza C, Smith AJ, Aquila I, Leone A, Waring CD, Henning BJ, Stirparo GG, Papait R, Scarfò M, Agosti V, Viglietto G, Condorelli G, Indolfi C, Ottolenghi S, Torella D, Nadal-Ginard B. 2013; Adult c-kit(pos) cardiac stem cells are necessary and sufficient for functional cardiac regeneration and repair. Cell. 154:827–842. DOI: 10.1016/j.cell.2013.07.039. PMID: 23953114.


10. Lees JG, Kong AM, Chen YC, Sivakumaran P, Hernández D, Pébay A, Harvey AJ, Gardner DK, Lim SY. 2019; Mitochon-drial fusion by M1 promotes embryoid body cardiac differentiation of human pluripotent stem cells. Stem Cells Int. 2019:6380135. DOI: 10.1155/2019/6380135. PMID: 31641358. PMCID: PMC6770295. PMID: 85ccbf95dccf420887dafb2aa41ba1df.


11. Lee JE, Seo BJ, Han MJ, Hong YJ, Hong K, Song H, Lee JW, Do JT. 2020; Changes in the expression of mitochondrial morphology-related genes during the differentiation of murine embryonic stem cells. Stem Cells Int. 2020:9369268. DOI: 10.1155/2020/9369268. PMID: 32399055. PMCID: PMC7204333. PMID: 08b215ff185940229f1bdf094d83ca55.


12. Varum S, Rodrigues AS, Moura MB, Momcilovic O, Easley CA 4th, Ramalho-Santos J, Van Houten B, Schatten G. 2011; Energy metabolism in human pluripotent stem cells and their differentiated counterparts. PLoS One. 6:e20914. DOI: 10.1371/journal.pone.0020914. PMID: 21698063. PMCID: PMC3117868. PMID: 3a763051317f43ffb33cf5da9b0515c6.


13. Ong SB, Hausenloy DJ. 2010; Mitochondrial morphology and cardiovascular disease. Cardiovasc Res. 88:16–29. DOI: 10.1093/cvr/cvq237. PMID: 20631158. PMCID: PMC2936127.


14. Ong SB, Hausenloy DJ. 2017; Mitochondrial dynamics as a therapeutic target for treating cardiac diseases. Handb Exp Phar-macol. 240:251–279. DOI: 10.1007/164_2016_7. PMID: 27844171.


15. Ishihara N, Eura Y, Mihara K. 2004; Mitofusin 1 and 2 play distinct roles in mitochondrial fusion reactions via GTPase ac-tivity. J Cell Sci. 117(Pt 26):6535–6546. DOI: 10.1242/jcs.01565. PMID: 15572413.


16. Olichon A, Baricault L, Gas N, Guillou E, Valette A, Belenguer P, Lenaers G. 2003; Loss of OPA1 perturbates the mitochondrial inner membrane structure and integrity, leading to cytochrome c release and apoptosis. J Biol Chem. 278:7743–7746. DOI: 10.1074/jbc.C200677200. PMID: 12509422.


17. Cereghetti GM, Stangherlin A, Martins de Brito O, Chang CR, Blackstone C, Bernardi P, Scorrano L. 2008; Dephospho-rylation by calcineurin regulates translocation of Drp1 to mito-chondria. Proc Natl Acad Sci U S A. 105:15803–15808. DOI: 10.1073/pnas.0808249105. PMID: 18838687. PMCID: PMC2572940.


18. Fransioli J, Bailey B, Gude NA, Cottage CT, Muraski JA, Emmanuel G, Wu W, Alvarez R, Rubio M, Ottolenghi S, Schaefer E, Sussman MA. 2008; Evolution of the c-kit-positive cell response to pathological challenge in the myocardium. Stem Cells. 26:1315–1324. DOI: 10.1634/stemcells.2007-0751. PMID: 18308948. PMCID: PMC4037162.


19. Orogo AM, Gonzalez ER, Kubli DA, Baptista IL, Ong SB, Prolla TA, Sussman MA, Murphy AN, Gustafsson ÅB. 2015; Accumulation of mitochondrial DNA mutations disrupts cardiac progenitor cell function and reduces survival. J Biol Chem. 290:22061–22075. Erratum in: J Biol Chem 2017;292:11348. DOI: 10.1074/jbc.A115.649657. PMID: 28687597. PMCID: PMC5500800.


20. Ong SB, Subrayan S, Lim SY, Yellon DM, Davidson SM, Hausenloy DJ. 2010; Inhibiting mitochondrial fission protects the heart against ischemia/reperfusion injury. Circulation. 121:2012–2022. DOI: 10.1161/CIRCULATIONAHA.109.906610. PMID: 20421521.


21. San Martin N, Cervera AM, Cordova C, Covarello D, McCreath KJ, Galvez BG. 2011; Mitochondria determine the differentiation potential of cardiac mesoangioblasts. Stem Cells. 29:1064–1074. DOI: 10.1002/stem.654. PMID: 21544900.


22. Jin J, Xuan QK, Zhou LJ, Shi CM, Song GX, Sheng YH, Qian LM. 2014; Dynamic mitochondrial changes during differentiation of P19 embryonic carcinoma cells into cardio-myocytes. Mol Med Rep. 10:761–766. DOI: 10.3892/mmr.2014.2315. PMID: 24920049.


23. Wang L, Ye X, Zhao Q, Zhou Z, Dan J, Zhu Y, Chen Q, Liu L. 2014; Drp1 is dispensable for mitochondria biogenesis in induction to pluripotency but required for differentiation of embryonic stem cells. Stem Cells Dev. 23:2422–2434. DOI: 10.1089/scd.2014.0059. PMID: 24937776.


24. Fu W, Liu Y, Yin H. 2019; Mitochondrial dynamics: biogenesis, fission, fusion, and mitophagy in the regulation of stem cell behaviors. Stem Cells Int. 2019:9757201. DOI: 10.1155/2019/9757201. PMID: 31089338. PMCID: PMC6476046. PMID: 798abb0e1ee64828bcce19bf5ea83351.


25. Vazquez-Martin A, Cufi S, Corominas-Faja B, Oliveras-Ferraros C, Vellon L, Menendez JA. 2012; Mitochondrial fusion by pharmacological manipulation impedes somatic cell reprogramming to pluripotency: new insight into the role of mitophagy in cell stemness. Aging (Albany NY). 4:393–401. DOI: 10.18632/aging.100465. PMID: 22713507. PMCID: PMC3409676.


26. Kasahara A, Cipolat S, Chen Y, Dorn GW 2nd, Scorrano L. 2013; Mitochondrial fusion directs cardiomyocyte differentia-tion via calcineurin and Notch signaling. Science. 342:734–737. DOI: 10.1126/science.1241359. PMID: 24091702.


27. Folmes CD, Nelson TJ, Martinez-Fernandez A, Arrell DK, Lindor JZ, Dzeja PP, Ikeda Y, Perez-Terzic C, Terzic A. 2011; Somatic oxidative bioenergetics transitions into pluripoten-cy-dependent glycolysis to facilitate nuclear reprogram-ming. Cell Metab. 14:264–271. DOI: 10.1016/j.cmet.2011.06.011. PMID: 21803296. PMCID: PMC3156138.


28. Prieto J, Seo AY, León M, Santacatterina F, Torresano L, Palomino-Schätzlein M, Giménez K, Vallet-Sánchez A, Ponsoda X, Pineda-Lucena A, Cuezva JM, Lippincott-Schwartz J, Torres J. 2018; MYC induces a hybrid energetics program early in cell reprogramming. Stem Cell Reports. 11:1479–1492. DOI: 10.1016/j.stemcr.2018.10.018. PMID: 30472011. PMCID: PMC6294174.


29. Chen W, Sun Y, Sun Q, Zhang J, Jiang M, Chang C, Huang X, Wang C, Wang P, Zhang Z, Chen X, Wang Y. 2020; MFN2 plays a distinct role from MFN1 in regulating spermatogonial differentiation. Stem Cell Reports. 14:803–817. DOI: 10.1016/j.stemcr.2020.03.024. PMID: 32330448. PMCID: PMC7221103.
30. Maxwell JT, Wagner MB, Davis ME. 2016; Electrically induced calcium handling in cardiac progenitor cells. Stem Cells Int. 2016:8917380. DOI: 10.1155/2016/8917380. PMID: 27818693. PMCID: PMC5080514. PMID: 6e4d500b11d946cda1d3262f03033db1.


31. Vigneault P, Naud P, Qi X, Xiao J, Villeneuve L, Davis DR, Nattel S. 2018; Calcium-dependent potassium channels control proliferation of cardiac progenitor cells and bone marrow-derived mesenchymal stem cells. J Physiol. 596:2359–2379. DOI: 10.1113/JP275388. PMID: 29574723. PMCID: PMC6002232.


32. Park JS, Kim YS, Yoo MA. 2009; The role of p38b MAPK in age-related modulation of intestinal stem cell proliferation and differentiation in Drosophila. Aging (Albany NY). 1:637–651. DOI: 10.18632/aging.100054. PMID: 20157545. PMCID: PMC2806044.


33. Bae YK, Kwon JH, Kim M, Kim GH, Choi SJ, Oh W, Yang YS, Jin HJ, Jeon HB. 2018; Intracellular calcium determines the adipogenic differentiation potential of human umbilical cord blood-derived mesenchymal stem cells via the Wnt5a/β-catenin signaling pathway. Stem Cells Int. 2018:6545071. DOI: 10.1155/2018/6545071. PMID: 30123291. PMCID: PMC6079381. PMID: 920a99315261416d8d99ff8ab14608bc.
34. Cribbs JT, Strack S. 2007; Reversible phosphorylation of Drp1 by cyclic AMP-dependent protein kinase and calcineurin regulates mitochondrial fission and cell death. EMBO Rep. 8:939–944. DOI: 10.1038/sj.embor.7401062. PMID: 17721437. PMCID: PMC2002551.


35. Li X, Zhu L, Yang A, Lin J, Tang F, Jin S, Wei Z, Li J, Jin Y. 2011; Calcineurin-NFAT signaling critically regulates early lineage specification in mouse embryonic stem cells and embryos. Cell Stem Cell. 8:46–58. DOI: 10.1016/j.stem.2010.11.027. PMID: 21211781.


36. Gómez-Salinero JM, López-Olañeta MM, Ortiz-Sánchez P, Larrasa-Alonso J, Gatto A, Felkin LE, Barton PJR, Navarro-Lérida I, Ángel Del Pozo M, García-Pavía P, Sundararaman B, Giovinazo G, Yeo GW, Lara-Pezzi E. 2016; The calcineurin variant CnAβ1 controls mouse embryonic stem cell differen-tiation by directing mTORC2 membrane localization and activation. Cell Chem Biol. 23:1372–1382. DOI: 10.1016/j.chembiol.2016.09.010. PMID: 27746127.


37. Mann KM, Ray JL, Moon ES, Sass KM, Benson MR. 2004; Cal-cineurin initiates smooth muscle differentiation in neural crest stem cells. J Cell Biol. 165:483–491. DOI: 10.1083/jcb.200402105. PMID: 15148306. PMCID: PMC2172346.


38. Fischer KM, Din S, Gude N, Konstandin MH, Wu W, Quijada P, Sussman MA. 2011; Cardiac progenitor cell commitment is inhibited by nuclear Akt expression. Circ Res. 108:960–970. DOI: 10.1161/CIRCRESAHA.110.237156. PMID: 21350213. PMCID: PMC3082319.


39. Dai DF, Danoviz ME, Wiczer B, Laflamme MA, Tian R. 2017; Mitochondrial maturation in human pluripotent stem cell derived cardiomyocytes. Stem Cells Int. 2017:5153625. DOI: 10.1155/2017/5153625. PMID: 28421116. PMCID: PMC5380852. PMID: f512aa1509de4709ad70a39b9d16dd6b.


40. Choi IY, Lim H, Cho HJ, Oh Y, Chou BK, Bai H, Cheng L, Kim YJ, Hyun S, Kim H, Shin JH, Lee G. 2020; Transcrip-tional landscape of myogenesis from human pluripotent stem cells reveals a key role of TWIST1 in maintenance of skeletal muscle progenitors. Elife. 9:e46981. DOI: 10.7554/eLife.46981. PMID: 32011235. PMCID: PMC6996923. PMID: 2ec2845de8084974b69d4de181793812.


41. Harding MW, Handschumacher RE. 1988; Cyclophilin, a primary molecular target for cyclosporine. Structural and fun-ctional implications. Transplantation. 46(2 Suppl):29S–35S. DOI: 10.1097/00007890-198808001-00006. PMID: 3043795.
42. Nicolli A, Basso E, Petronilli V, Wenger RM, Bernardi P. 1996; Interactions of cyclophilin with the mitochondrial inner me-mbrane and regulation of the permeability transition pore, and cyclosporin A-sensitive channel. J Biol Chem. 271:2185–2192. DOI: 10.1074/jbc.271.4.2185. PMID: 8567677.


43. Paslaru L, Davidson S, Popescu I, Morange M. 2007; The effect of cyclosporine A on cardiomyocytes differentiation. J Cell Mol Med. 11:369–371. DOI: 10.1111/j.1582-4934.2007.00029.x. PMID: 17488483. PMCID: PMC3822835.


44. Montero M, Lobatón CD, Gutierrez-Fernández S, Moreno A, Alvarez J. 2004; Calcineurin-independent inhibition of mito-chondrial Ca2+ uptake by cyclosporin A. Br J Pharmacol. 141:263–268. DOI: 10.1038/sj.bjp.0705609. PMID: 14691054. PMCID: PMC1574196.


45. Nash A, Samoylova M, Leuthner T, Zhu M, Lin L, Meyer JN, Brennan TV. 2020; Effects of immunosuppressive medications on mitochondrial function. J Surg Res. 249:50–57. DOI: 10.1016/j.jss.2019.12.010. PMID: 31918330. PMCID: PMC7136143.


46. Yilmaz DE, Kirschner K, Demirci H, Himmerkus N, Bach-mann S, Mutig K. 2022; Immunosuppressive calcineurin inhibi-tor cyclosporine A induces proapoptotic endoplasmic reticulum stress in renal tubular cells. J Biol Chem. 298:101589. DOI: 10.1016/j.jbc.2022.101589. PMID: 35033536. PMCID: PMC8857494.
47. Hojo M, Morimoto T, Maluccio M, Asano T, Morimoto K, Lagman M, Shimbo T, Suthanthiran M. 1999; Cyclosporine indu-ces cancer progression by a cell-autonomous mechanism. Na-ture. 397:530–534. DOI: 10.1038/17401. PMID: 10028970.


48. Bordt EA, Clerc P, Roelofs BA, Saladino AJ, Tretter L, Adam-Vizi V, Cherok E, Khalil A, Yadava N, Ge SX, Francis TC, Kennedy NW, Picton LK, Kumar T, Uppuluri S, Miller AM, Itoh K, Karbowski M, Sesaki H, Hill RB, Polster BM. 2017; The putative Drp1 inhibitor mdivi-1 is a reversible mitochondrial complex I inhibitor that modulates reactive oxygen species. Dev Cell. 40:583–594.e6. DOI: 10.1016/j.devcel.2017.02.020. PMID: 28350990. PMCID: PMC5398851.


Fig. 1
Differentiation of c-kit+ CPCs is mediated by dexamethasone incubation. Representative epifluorescence images and quantification of (A) c-kit and (B) GATA-4 in the c-kit+ CPCs at Day 0 and 7-days post-dexamethasone treatment (n=80 cells per group; *p<0.05). (C) Representative Western blot of mitochondrial respiratory subunits in the c-kit+ CPCs at Day 0 and 7-days post-dexamethasone treatment.
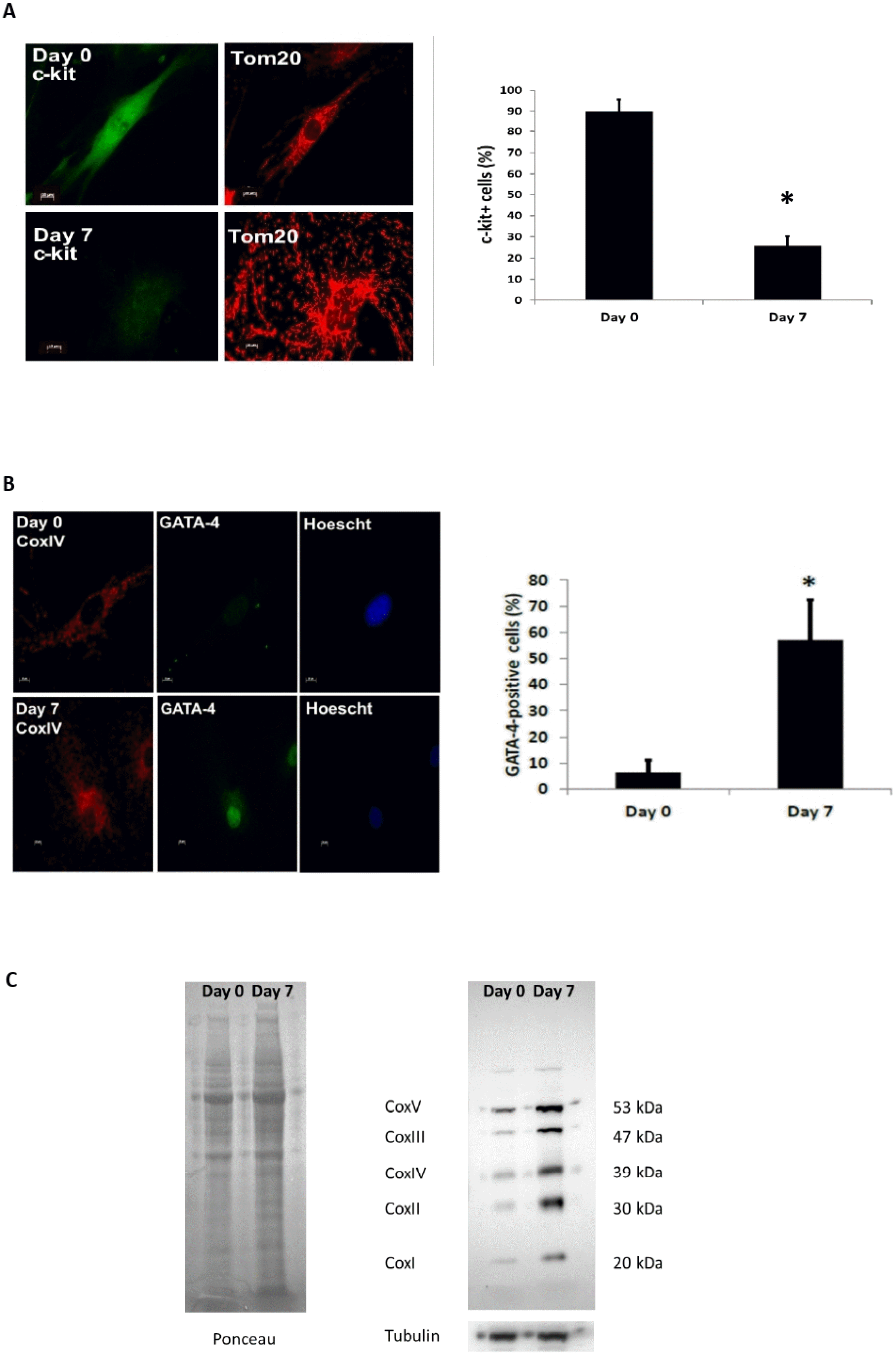
Fig. 2
Differentiation of c-kit+ CPCs promotes changes in mitochondrial morphology. Dexamethasone incubation for 7 days causes (A) fragmentation of the mitochondria in the c-kit+ CPCs, (B) an increase in mitochondrial number, and (C) a reduction in the average size of the individual mitochondrion (n=80 cells per group; *p<0.05). (D) Mitochondrial fragmentation occurred as early as Day 2 following dexamethasone treatment (n=80 cells per group; *p<0.05).
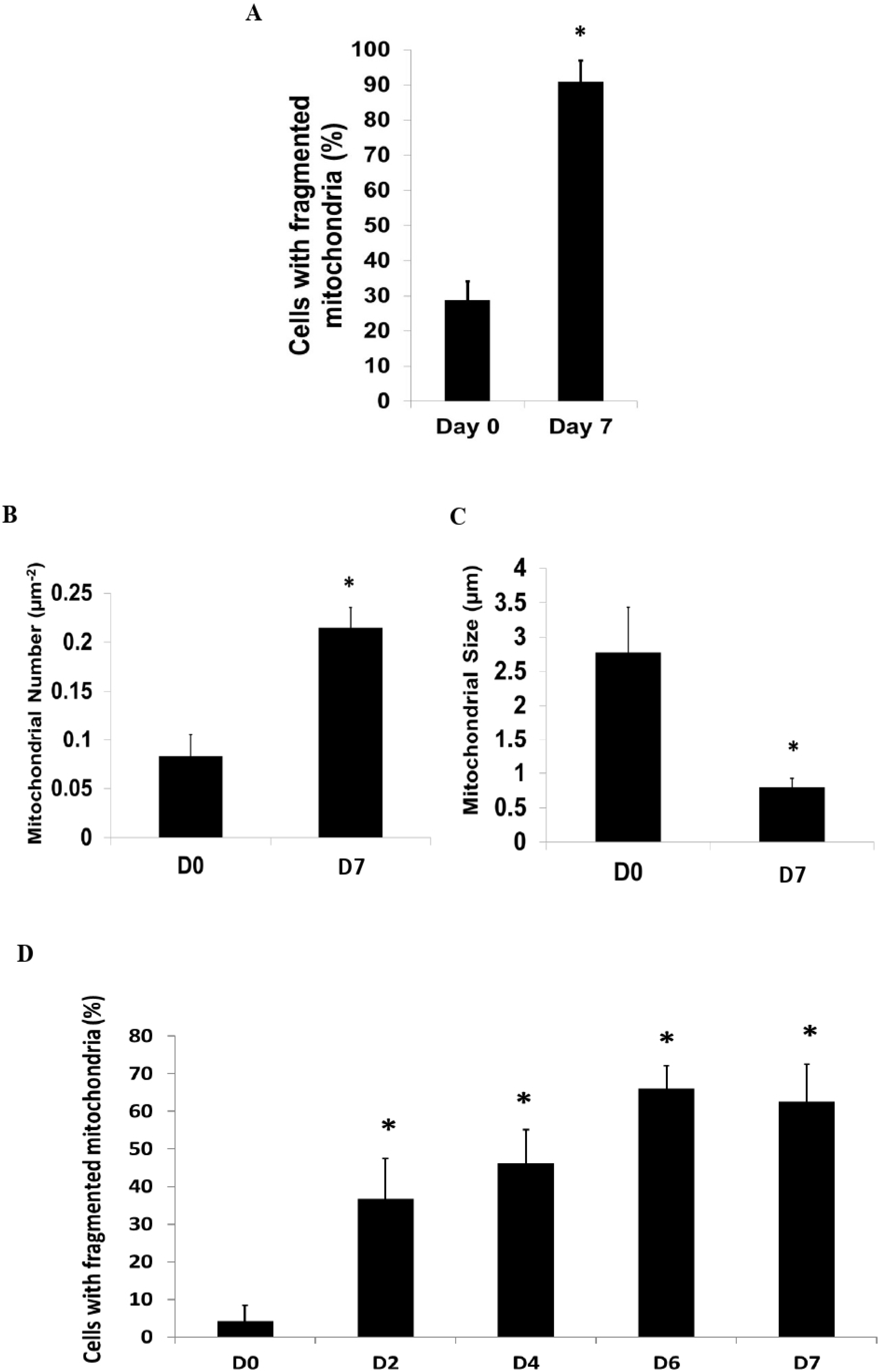
Fig. 3
Mitochondrial fragmentation during c-kit differentiation is mediated by calcineurin-Drp1. (A) Representative Western blot images of mitochondrial-shaping proteins in c-kit+ CPCs at Day 0 and Day 7 following dexamethasone treatment. (B) Representative Western blot image of mitochondrial-shaping proteins following a time course of 7 days dexamethasone treatment. (C) Representative Western blot image profiling the changes in phosphorylated Drp1 at the S637 site following a time course of 7 days dexamethasone treatment. (D) Quantification of calcineurin activity during dexamethasone-differentiation of c-kit+ CPCs (n=3, *p<0.05 when compared with D0; †p<0.05 when compared with D7).
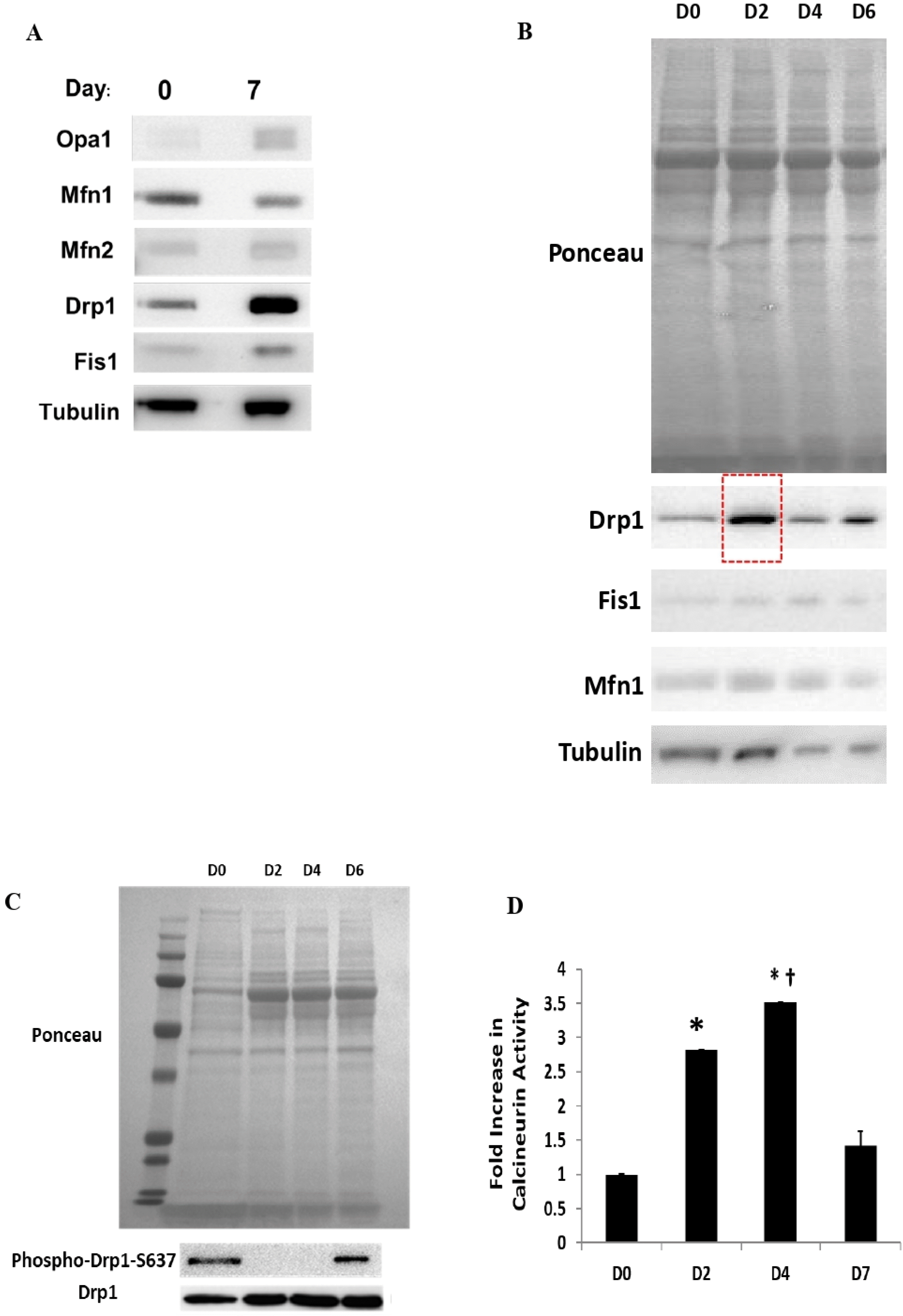
Fig. 4
Drp1 inhibition impairs mitochondrial fragmentation and c-kit differentiation. (A) Representative epifluorescence images of c-kit+ CPCs stained with antibodies against CoxIV (red), GATA-4 (green) with Hoescht (blue). (B) Quantification of c-kit+ CPCs with fragmented mitochondria following mdivi-1 treatment (n=80; *p<0.05 when compared with D0; †p<0.05 when compared with D7 with 50 μM mdivi-1). (C) GATA-4-positive c-kit+ CPCs following 7 days dexamethasone treatment in the presence of mdivi-1 (n=80; *p<0.05 when compared with D0; †p<0.05 when compared with D7 with 50 μM mdivi-1).
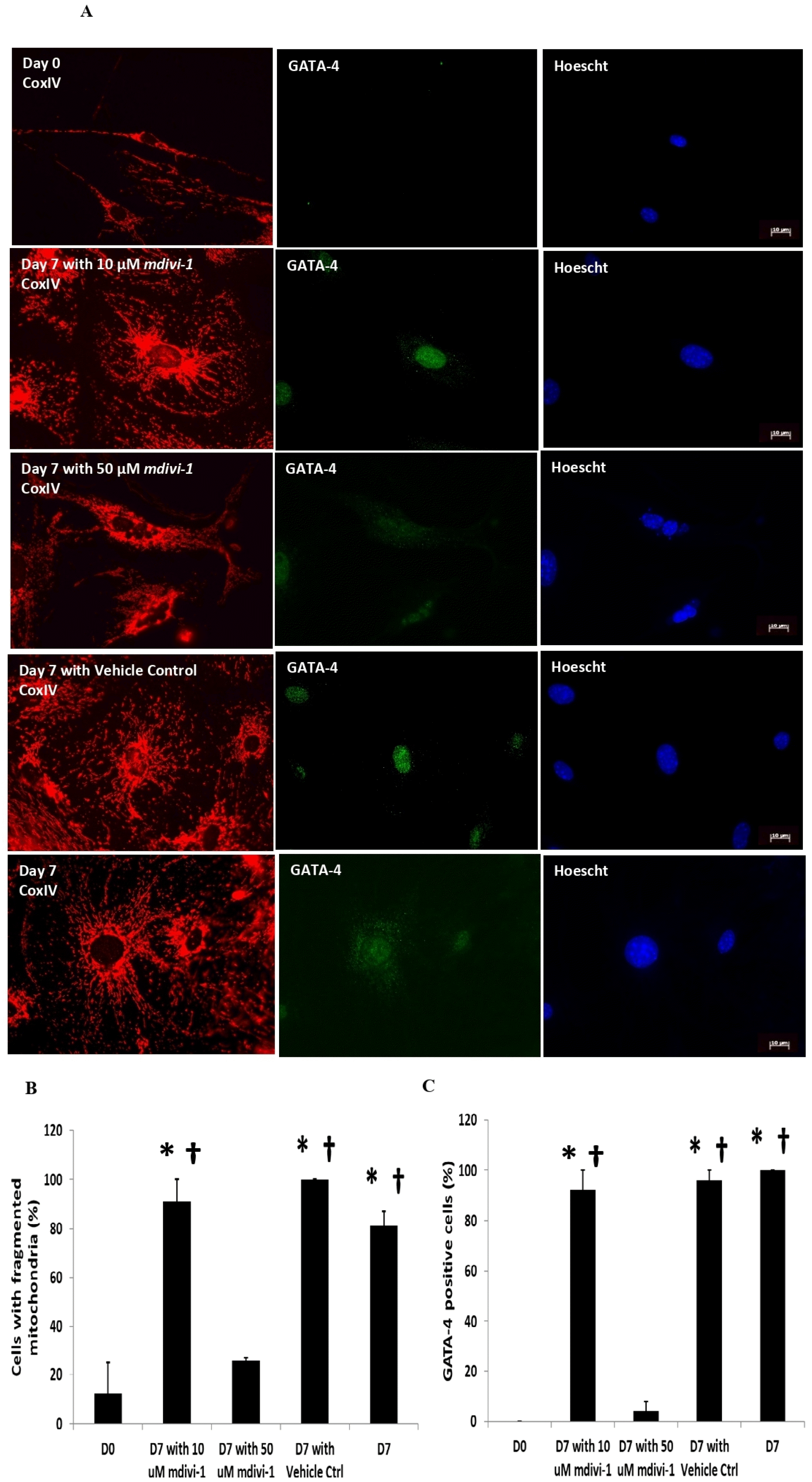
Fig. 5
Inhibition of calcineurin impairs mitochondrial fragmentation and differentiation of c-kit CPCs. (A) Representative epifluorescence images of c-kit+ CPCs stained with antibodies against CoxIV (red), GATA-4 (green) with Hoescht (blue). (B) Quantification of c-kit+ CPCs with fragmented mitochondria following CsA treatment (n=80; *p<0.05 when compared with D0; †p<0.05 when compared with D7 with 5 μM CsA). (C) GATA-4-positive c-kit+ CPCs following 7 days dexamethasone treatment in the presence of CsA (n=80; *p<0.05 when compared with D0; †p<0.05 when compared with D7 with 5 μM CsA).
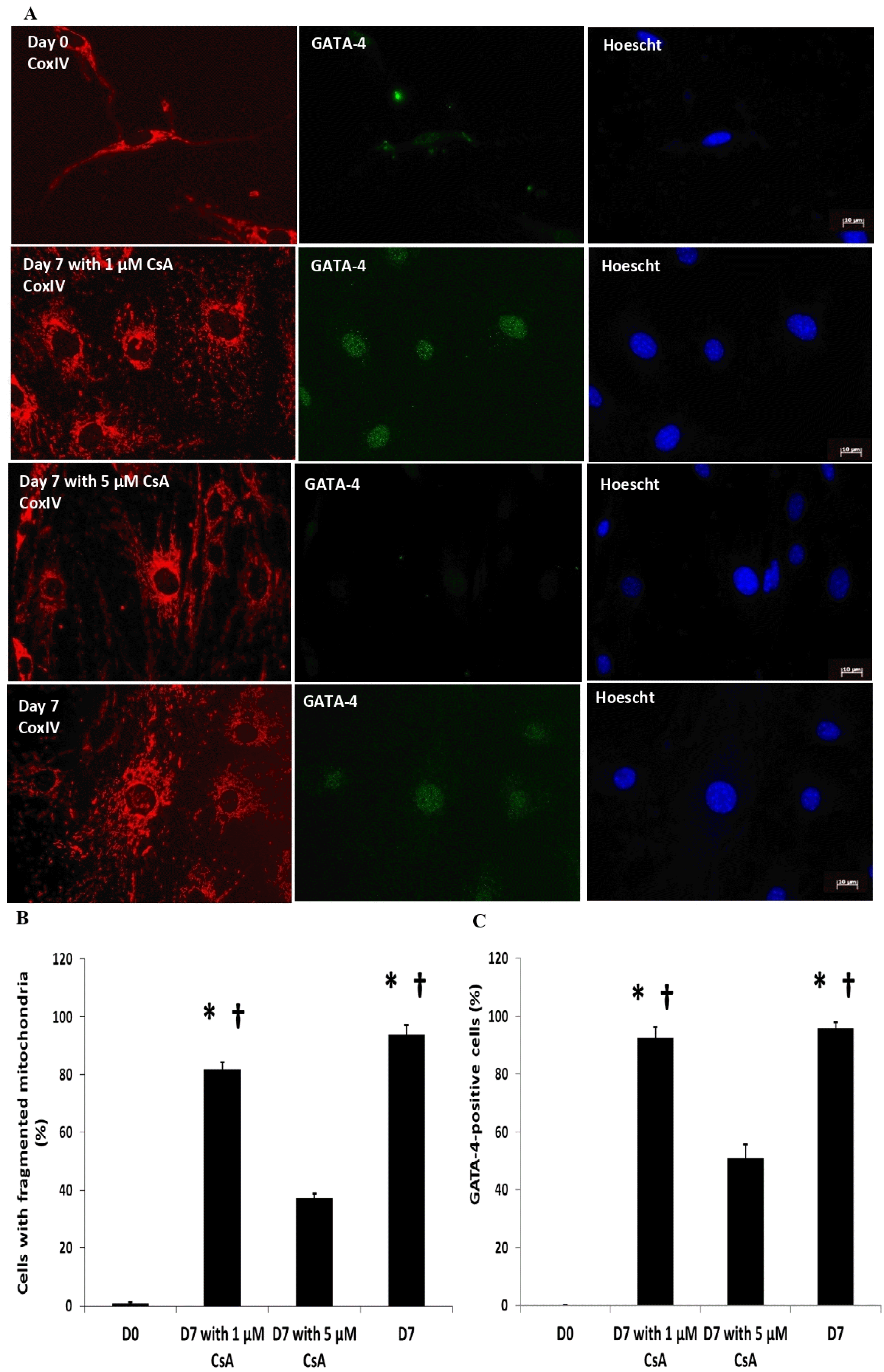