Abstract
Objective
The growth and development of the mandible strongly depend on modeling changes occurring at its ramus. Here, we investigated covariance patterns between the morphology of the ramus and the rest of the face.
Methods
Lateral cephalograms of 159 adults (55 males and 104 females) with no history of orthodontic treatment were collected. Geometric morphometrics with sliding semi-landmarks was used. The covariance between the ramus and face was investigated using a two-block partial least squares analysis (PLS). Sexual dimorphism and allometry were also assessed.
Results
Differences in the divergence of the face and anteroposterior relationship of the jaws accounted for 24.1% and 21.6% of shape variation in the sample, respectively. Shape variation was greater in the sagittal plane for males than for females (30.7% vs. 17.4%), whereas variation in the vertical plane was similar for both sexes (23.7% for males and 25.4% for females). Size-related allometric differences between the sexes accounted for the shape variation to a maximum of 6% regarding the face. Regarding the covariation between the shapes of the ramus and the rest of the face, wider and shorter rami were associated with a decreased lower anterior facial height as well as a prognathic mandible and maxilla (PLS 1, 45.5% of the covariance). Additionally, a more posteriorly inclined ramus in the lower region was correlated with a Class II pattern and flat mandibular plane.
Growth of the craniofacial complex is an extremely complicated process involving structural and spatial changes in all its units in different developmental periods and to different extents, rates, and directions. Historically, the mandibular condyle has been considered a primary growth center,1-3 which, under the influence of intrinsic factors, can control mandibular growth and displace the mandible inferiorly and anteriorly, thus defining the sagittal relationship of the jaws. In contrast, the theory of a functional matrix4,5 accounts for the mandibular condyle as a locus where only secondary, compensatory periosteal growth occurs, and mandibular growth is attributed to the morphologic effects of both capsular and periosteal matrices.
According to studies on animal and human mandibles, it is common knowledge that the mandible grows forward by bone deposition at the posterior border of the ramus rather than the anterior mandibular region.6-8 Simultaneously, the anterior border of the ramus is resorptive; therefore, the body of the mandible elongates.9 Following the aforementioned modeling of the ramus, coupled with modeling changes at the condylar neck, the mandible is translated downwards and forward relative to the cranial base by growing larger in an upward and backward direction.10
According to Enlow’s counterpart principle of craniofacial growth,11 the modeling process at the mandibular ramus results in the lengthening of the mandibular corpus and simultaneous anterior displacement of the mandible, both of which help to keep pace with the amount of anterior maxillary displacement due to growth. Simultaneously, since the expansion of the middle cranial fossa has unequally displaced forward the nasomaxillary complex and the mandible, different amounts of bone deposition and resorption at the posterior and anterior borders of the ramus lead to increased ramus width, which in turn displaces the mandible forward in a coordinated position with the maxilla.11
Traditional cephalometry is not the most appropriate method for quantifying and/or evaluating the shape of the craniofacial structures since the collection of landmarks, angles, ratios, and linear distances only provide partial and localized information based on the assumption that reference planes are stable.12,13 Instead, shape can be investigated with geometric morphometrics by statistically analyzing the coordinates of both traditional landmarks and semi-landmarks, the latter of which can enable the quantification of homologous curves instead of just points.14 Geometric morphometrics is applicable in orthodontics and can reveal substantial shape information by circumventing the important limiting conventions of traditional cephalometry.15
The ramus might play a vital, primary role in the development of the mandible and its anteroposterior relationship with the maxilla.16 Although orthodontic treatment plans often aim to modify the growth of the mandible, orthodontists may underestimate the ramus as an important mandibular module, either by downgrading its role compared to condylar growth or because of limitations of conventional cephalometry.16 If ramal morphology covaries with the anteroposterior position of the mandible, it could become an essential diagnostic element leading to better-informed treatment plans, which directly benefit patients. Therefore, this study aimed to investigate the covariation between the shape of the ramus and the rest of the face using geometric morphometrics in an adult population. According to Enlow’s counterpart theory11 as well as the growth mechanism of the mandible at its ramus, wider rami might be correlated with more prognathic mandibles.
The study sample was obtained from the Clinic of Orthodontics and Pediatric Dentistry archives at the Center of Dental Medicine, University of Zurich, Switzerland, between 2003 and 2016. Patients signed an informed consent form before treatment initiation, and the research project was approved by the Cantonal Ethics Committee Zurich (BASEC-Nr. 2020-03012). Since morphological covariation between the ramus and the rest of the face was to be investigated with the RV coefficient,17 the sample size estimation was based on the findings by Fruciano et al.,18 who reported relatively stable RV values with sample sizes greater than 100. Consequently, at least 100 individuals had to be included in this study. The sample was collected based on the following eligibility criteria: (1) adult patients aged > 18 years, (2) cephalometric radiographs of good quality in habitual occlusion with no functional shifts, (3) no previous orthodontic treatment, and (4) no craniofacial malformations, systemic diseases, syndromes, or myofunctional disorders.
Lateral cephalograms were scanned at a resolution of 150 dpi.19 They were then imported to Viewbox version 4.1.0.10 (dHAL software, Kifissia, Greece) for landmark acquisition. In total, 66 landmarks were digitized: 15 landmarks of types I and II and 51 semi-landmarks,20 which were organized into five curves and two datasets. The curves corresponded to the outlines of the posterior and anterior borders of the ramus, mandible, maxilla, and frontonasal area. These curves were organized into three datasets and depicted the morphology of the ramus (two curves: posterior and anterior borders of ramus), rest of the face (three curves: mandible, maxilla, and frontonasal area), and whole configuration (all curves; Figure 1). After landmark digitization, semi-landmarks were automatically placed along their adjusted curves in equidistant positions. Semi-landmarks were allowed to slide along their respective curves to minimize bending energy, with the average configuration as the reference shape.14 Each time, a new average configuration was derived from sliding and set as the new reference shape for subsequent sliding. This process was repeated three times.
Intra- and inter-rater measurement errors were evaluated with a Procrustes ANOVA on a set of 30 lateral cephalograms, each against the biological variation of the whole configuration both in shape (Procrustes coordinates) and size (centroid size). Intra-rater assessment was performed at two-week intervals, and inter-rater assessment was performed by two raters. Outliers were visually checked in all three datasets using boxplots for size and Procrustes or squared Mahalanobis distances for shape.21 The subsequent analyses and graphs were produced in MorphoJ.22
Information unrelated to shape was removed by a generalized partial least squares Procrustes superimposition (GLS) with orthogonal projection on the tangent plane. GLS was applied to the raw coordinates of the whole configuration of all individuals in the sample to acquire the Procrustes coordinates and the average shape of the sample in the shape space.
A principal component analysis (PCA) was then performed to investigate patterns of shape variation in the study sample. Principal components (PCs) that explained most of the shape variance and were biologically meaningful were considered.
Sexual dimorphism regarding shape was separately assessed in all three datasets using Procrustes distance and permutation tests on the mean values between males and females. Signs of separation between sexes were additionally sought in the shape space by visually inspecting the PC plots of the whole configuration.
Sexual dimorphism in size was tested by comparing the logarithm of centroid size (InCS) between males and females for all three datasets with an independent t-test. Furthermore, since the male-to-female ratio of the sample was approximately 1:2, static allometry was expected because males are, on average, larger than females.23 We assessed its significance with a multivariate regression analysis of shape (dependent variables: Procrustes coordinates) on size (independent variable: InCS) for all the datasets. To further control for allometry, new shape variables corrected for the effect of size were obtained for each dataset by exporting the residuals of the aforementioned respective multivariate regressions.24
Two-block partial least squares (PLS) analysis was performed to investigate any covariation patterns between the morphology of the ramus and the rest of the face. The dataset whole configuration was divided into two blocks: ramus and the rest of the face.25 Two separate Procrustes fits were applied to evaluate joint changes in shape within the ramus and the rest of the face and, consequently, investigate the covariation between the ramus and the rest of the face.17 The strength of the covariation between the datasets was quantified using the RV coefficient, which can take values between zero and one.17 PLS analyses were carried out with (Procrustes coordinates) and without (regression residuals) the allometric effect. Furthermore, to better control for the potential influence on the outcome due to the unbalanced male-to-female ratio, PLS analyses were repeated with only females (104 females, 35.5 (standard deviation [SD]) = 11.7 years old).26
The sample consisted of 159 individuals (55 males and 104 females) with a mean age of 34.4 years (SD = 11.4). Males were 32.4 (SD = 10.8) and females 35.5 (SD = 11.7) years, respectively. The Mann–Whitney U test did not identify any statistically significant age differences between males and females (U = 2,404, p = 0.1). The baseline cephalometric data for the entire sample are provided in Supplementary Table 1. No outliers were detected in terms of size and shape as far as all the datasets were concerned. The intra- and inter-rater measurement errors explained less than 1% of the total shape variance in the whole configuration for both shape and size.
The first four PCs accounted for 66.1% of the total shape variance of the whole configuration (PC1 24.1%, PC2 21.6%, PC3 11.5%, and PC4 8.9%). PC1 depicted shape differences corresponding to skeletal hyperdivergence and decreased facial depth, whereas a hypodivergent skeletal pattern with increased facial depth was evident in the opposite direction. PC2 described the shape differences in the sagittal plane between Class II and III skeletal patterns (Figure 2). Alveolar shape variability towards bimaxillary protrusion with a steeper mandibular plane or bimaxillary retrusion with a flatter mandibular plane was seen in PC3, whereas PC4 showed variability in the width of the ramus; wider rami were combined with bimaxillary protrusion and a flatter mandibular plane, whereas narrower rami were combined with bimaxillary retrusion and a steeper mandibular plane (Supplementary Figure 1).
Shape differences between males and females were detected in all datasets (9,999 permutations, whole configuration p = 0.0001, ramus p = 0.0102, rest of the face p = 0.0001; Table 1). Although males and females largely overlapped in the PC plots, some separation hints along PC1, PC2, and PC3 were evident (Figure 2 and Supplementary Figure 2). To visualize sexual dimorphism in shape, we performed additional PCA separately for males and females. Most of the variance was explained by shape differences in the sagittal plane in males (30.7%), whereas females mostly presented shape variation in the vertical plane (25.4%). However, PC1 and PC2 described the same shape variation in reversed order for males and females and jointly accounted for 54.4% and 42.8% of the total variance in males and females, respectively. PC3 explained only approximately 10% of the variance in both sexes, which showed changes in the gonial area for males and alveolar changes in females (Supplementary Figure 2).
For all datasets, significant size differences between the sexes were detected (p < 0.0001; Table 2). However, although regressions were highly statistically significant for all datasets (p ≤ 0.0028; Table 3), shape variance explained by allometry only ranged from 2.2% (ramus) to 6.0% (the rest of the face).
PLS analyses of all individuals with and without the allometric effect as well as only females produced similar results. Among the groups, the total covariance explained by PLS 1 ranged from 41.9% in the female group to 45.5% in all individuals with the allometric effect included. Results for PLS 2 only ranged from 25.1% in all individuals with the allometric effect removed to 25.5% in the female group and in the group of all individuals with the allometric effect included (Table 4). By inspecting the associated plots and outline drawings, slight shape changes between females and all individuals, with and without the allometric effect, were observed for PLS 1 (Figure 3 and Supplementary Figure 3). These slight shape changes between females and the other groups mostly referred to the sagittal plane, especially the anteroposterior position of the maxilla.
In all individuals with the allometric effect included (Figure 3), 45.5% (p < 0.0001) and 25.5% (p < 0.0001) of the total covariance between the ramus and the rest of the face was explained by PLS 1 and PLS 2, respectively. PLS 1 described covariance mainly between the width and height of the ramus, sagittal and vertical positions of the jaws, and thickness of the symphysis. In PLS 1, a wider and shorter ramus with an increased posterior curvature in the lower area (gonial area) was related to a decreased lower anterior facial height, a slightly prognathic mandible with a thicker symphysis, and a prognathic maxilla. PLS 2 depicted covariance pertaining to the position of the lower area of the ramus and changes in the sagittal relationship of the jaws and the inclination of the mandibular plane. A more posteriorly positioned ramus with an increased posterior curvature in the lower area (gonial area) was related to the Class II pattern and flatter mandibular plane.
In females, PLS 1 explained 41.9% (p < 0.0001) of the covariance between the ramus and the rest of the face (Table 4). It depicted the covariance between the width of the ramus and the vertical dimension of the face, as well as the sagittal position of the mandible and the thickness of the symphysis. Wider rami in the upper posterior and lower anterior rims were related to a decreased lower anterior facial height and a prognathic mandible with an increased thickness of symphysis (Supplementary Figure 3).
The present study included an extensive range of skeletal patterns in adults. These morphological variations were revealed by PCA analyses, where PC1 described morphological variation in the vertical plane as the divergence of the maxillary and mandibular planes, while variation in the sagittal plane corresponding to Class II or III skeletal patterns was described by PC2. These results are in accordance with those of other orthodontic populations15,26-28 and, collectively, may suggest that facial variation originating from the vertical plane is predominant. Indeed, vertical variation, which is age- and size-related, may be the most significant source of facial variation in humans.29 Specifically, the overall facial pattern is established early, and skull proportions are determined by growth in the vertical plane, which involves posterior rotation of the mandible as far as anterior facial height variation in humans is concerned.26,30 However, if conventional superimpositions on cranial base structures are used, growth rotations are generally masked because of modeling within the jaws, which might impede diagnostic accuracy with conventional cephalometrics in the vertical plane.31 In this study, the adult orthodontic population was investigated using geometric morphometrics. Such growth rotations are expected to have been completed, along with growth in other planes in adults, which could minimize confounding arising from growth in different structures and developmental times.32 Furthermore, geometric morphometrics based on sliding semi-landmarks enabled the investigation of curves, which would have been impossible with conventional cephalometric measurements, irrespective of the reference planes.14 It is important to note that another common area of facial morphological variation is the gonial angle, which, in orthodontic populations, consistently contributes 8–10% to facial skeletal variation.15,27,28 This was also confirmed in the present investigation, since PC3 and PC4, except for alveolar variation, also depicted variations in the gonial area (PC3) and ramus width (PC4), amounting to 11.5% and 8.9%, respectively (Supplementary Figure 1). Ramus width is highly variable among humans, and variation is primarily located at the posterior border.33,34 According to Enlow’s counterpart theory,11 the mandibular ramus is the specific structural counterpart of the middle cranial fossa and pharynx; its horizontal growth can bridge the pharyngeal space35 and span the middle cranial fossa, resulting in proper intermaxillary fit. However, the lower correlation between the midline cranial base and mandibular ramus, compared to the correlation between the lateral basicranial elements and ramus, indicates that the lateral basicranial structures are more relevant than the midline cranial base.36 Indeed, in a morphometric study with a non-orthodontic adult population, the correlation between the petrosal part of the middle cranial fossa and the posterior border of the ramus was significantly higher than that between the midline cranial base and the posterior border of the ramus or the basicranium and the anterior border of the ramus.34 However, common developmental timing appears to strongly affect such integrations since the higher integration between the midline cranial base and the face in children shifts in adulthood; adults demonstrate higher integration between the lateral parts of the basicranium and the face.26
Since horizontal growth of the ramus might establish the anteroposterior position of the mandible and, consequently, its relationship with the rest of the face, this study investigated the integration of the ramus with the rest of the face. The curves used to capture the ramal shape were drawn along the posterior and anterior ramal margins, excluding the sigmoid notch region, which is biologically irrelevant to the anteroposterior position of the mandible (Figure 1). The shape patterns corresponding to the most covariance-explained (45.5%, p < 0.0001) pertained to the width and height of the ramus in relation to the anteroposterior position of the jaws, the thickness of the symphysis and divergence of the face. Specifically, the wider and shorter the ramus, the more prognathic the jaws were, and the thicker the symphysis and the more hypodivergent the faces were (Figure 3). These findings follow the well-discernible facial patterns in the vertical plane. Compared to hyperdivergent individuals, rami have been reported to be wider in hypodivergent individuals.37 Furthermore, compared with facial hyperdivergence, more elongated and anteriorly positioned maxilla and mandible,38 including increased symphyseal dimensions,39 appear in facial hypodivergence. Finally, the present results corroborate other morphometric findings stating that a narrower and more elongated ramus correlates with facial hyperdivergence, whereas a wider ramus correlates with a hypodivergent pattern.40
PLS 2, which explained 25.5% (p < 0.0001) of the covariance between the face and ramus, referred to the position of both posterior and anterior rims of the ramus at its caudal region, the sagittal jaw relationship, and the steepness of the mandibular plane (Figure 3). The more posteriorly the ramus was positioned in its lower region, the more Class II the jaw relationship and the flatter the mandibular plane. The posterior position of the ramus and the closed gonial angle leading to a flat mandibular plane may have led to a mandibular retrusive effect.41
Regarding the differences in the covariance patterns between the ramus and the rest of the face in only females, PLS 1 (41.9%, p < 0.0001) mainly described changes in the anteroposterior dimensions of the ramus in relation to facial divergence and the mandibular anteroposterior position. The wider the ramus, the more hypodivergent the face and the more prognathic the mandible. The covariance patterns described in this study were similar between males and females. However, sexual dimorphism of the mandible has been reported for both size and shape, and the ramus is a highly dimorphic area.42,43
From our findings, an increased ramal width correlates with a decreased lower anterior facial height and prognathic mandible and maxilla. In contrast, a posterior orientation of the ramus in its lower region correlates with a retrognathic mandible and a flat mandibular plane within a Class II skeletal pattern. The width, height, and inclination of the ramus are related to facial changes in both the sagittal and vertical planes.
Notes
AUTHOR CONTRIBUTIONS
Conception/design: VK, DJH. Data acquisition: MK, VK. Data analysis: VK. Data interpretation: VK, DJH, TE. Drafting and revising the manuscript: MK, VK, DJH, TE. Approval of the final version to be published: MK, VK, DJH, TE. Agreement to be accountable for all aspects of the work: MK, VK, DJH, TE.
REFERENCES
1. Charles SW. 1925; The temporomandibular joint and its influence on the growth of the mandible. Br Dent J. 46:845–55. https://cir.nii.ac.jp/crid/1573950400256289024.
2. Brodie AG. 1941; On the growth pattern of the human head. From the third month to the eighth year of life. Am J Anat. 68:209–62. https://doi.org/10.1002/aja.1000680204. DOI: 10.1002/aja.1000680204.


3. Sicher H. 1947; The growth of the mandible. Am J Orthod. 33:30–5. https://doi.org/10.1016/0096-6347(47)90259-7. DOI: 10.1016/0096-6347(47)90259-7. PMID: 20278244.


4. Moss ML. 1960; Functional analysis of human mandibular growth. J Prosthet Dent. 10:1149–59. https://doi.org/10.1016/0022-3913(60)90228-6. DOI: 10.1016/0022-3913(60)90228-6.


5. Moss ML, Salentijn L. 1969; The primary role of functional matrices in facial growth. Am J Orthod. 55:566–77. https://doi.org/10.1016/0002-9416(69)90034-7. DOI: 10.1016/0002-9416(69)90034-7. PMID: 5253955.


6. Humphry GM. 1864. On the growth of the jaws. Cambridge University;Cambridge: https://books.google.co.kr/books/about/On_the_Growth_of_the_Jaws.html?id=iy0lzQEACAAJ&redir_esc=y. DOI: 10.1093/gmo/9781561592630.article.j601900.
7. Brash JC. 1924. Growth of the Jaws and Palate. The growth of the jaws, normal and abnormal, in health and disease: five lectures. Dental Board of the United Kingdom;London: p. 23–66. https://books.google.co.kr/books/about/The_Growth_of_the_Jaws_Normal_and_Abnorm.html?id=Z5QPzQEACAAJ&redir_esc=y. DOI: 10.1093/gmo/9781561592630.article.j601900.
8. Enlow DH, Harris DB. 1964; A study of the postnatal growth of the human mandible. Am J Orthod. 50:25–50. https://doi.org/10.1016/S0002-9416(64)80016-6. DOI: 10.1016/S0002-9416(64)80016-6.


9. Bang S, Enlow DH. 1967; Postnatal growth of the rabbit mandible. Arch Oral Biol. 12:993–8. https://doi.org/10.1016/0003-9969(67)90094-5. DOI: 10.1016/0003-9969(67)90094-5. PMID: 5231276.


10. Proffit WR, Fields HW, Sarver DM. 2007. Contemporary orthodontics. Mosby Elsevier;St. Louis: https://www.worldcat.org/ko/title/contemporary-orthodontics/oclc/769189433. DOI: 10.1002/j.0022-0337.2007.71.12.tb04437.x.
11. Enlow DH, Hans MG. 1996. Essentials of facial growth. Saunders;Philadelphia: https://www.amazon.com/Essentials-Facial-Growth-Donald-Enlow/dp/0721661068. DOI: 10.1097/00008505-199600540-00041.
12. Moyers RE, Bookstein FL. 1979; The inappropriateness of conventional cephalometrics. Am J Orthod. 75:599–617. https://doi.org/10.1016/0002-9416(79)90093-9. DOI: 10.1016/0002-9416(79)90093-9. PMID: 287374.


13. McIntyre GT, Mossey PA. 2003; Size and shape measurement in contemporary cephalometrics. Eur J Orthod. 25:231–42. https://doi.org/10.1093/ejo/25.3.231. DOI: 10.1093/ejo/25.3.231. PMID: 12831212.


14. Gunz P, Mitteroecker P. 2013; Semilandmarks: a method for quantifying curves and surfaces. Hystrix It J Mamm. 24:103–9. https://doi.org/10.4404/hystrix-24.1-6292.


15. Halazonetis DJ. 2004; Morphometrics for cephalometric diagnosis. Am J Orthod Dentofacial Orthop. 125:571–81. https://doi.org/10.1016/j.ajodo.2003.05.013. DOI: 10.1016/j.ajodo.2003.05.013. PMID: 15127026.


16. Enlow DH. 1983; JCO/interviews Dr. Donald H. Enlow on craniofacial growth. J Clin Orthod. 17:669–79. https://pubmed.ncbi.nlm.nih.gov/6586731/.
17. Klingenberg CP. 2009; Morphometric integration and modularity in configurations of landmarks: tools for evaluating a priori hypotheses. Evol Dev. 11:405–21. https://doi.org/10.1111/j.1525-142X.2009.00347.x. DOI: 10.1111/j.1525-142X.2009.00347.x. PMID: 19601974. PMCID: PMC2776930.


18. Fruciano C, Franchini P, Meyer A. 2013; Resampling-based approaches to study variation in morphological modularity. PLoS One. 8:e69376. https://doi.org/10.1371/journal.pone.0069376. DOI: 10.1371/journal.pone.0069376. PMID: 23874956. PMCID: PMC3712944. PMID: 3636493e64c9478da1df331b4ab3be8b.


19. Halazonetis DJ. 2004; At what resolution should I scan cephalometric radiographs? Am J Orthod Dentofacial Orthop. 125:118–9. https://doi.org/10.1016/j.ajodo.2003.11.004. DOI: 10.1016/j.ajodo.2003.11.004. PMID: 14718889.


20. Bookstein FL. 1991. Morphometric tools for landmark data: geometry and biology. Cambridge University Press;Cambridge: https://doi.org/10.1017/CBO9780511573064. DOI: 10.1017/CBO9780511573064.


21. Klingenberg CP, Monteiro LR. 2005; Distances and directions in multidimensional shape spaces: implications for morphometric applications. Syst Biol. 54:678–88. https://doi.org/10.1080/10635150590947258. DOI: 10.1080/10635150590947258. PMID: 16126663.


22. Klingenberg CP. 2011; MorphoJ: an integrated software package for geometric morphometrics. Mol Ecol Resour. 11:353–7. https://doi.org/10.1111/j.1755-0998.2010.02924.x. DOI: 10.1111/j.1755-0998.2010.02924.x. PMID: 21429143.


23. Velemínská J, Bigoni L, Krajíček V, Borský J, Šmahelová D, Cagáňová V, et al. 2012; Surface facial modelling and allometry in relation to sexual dimorphism. Homo. 63:81–93. https://doi.org/10.1016/j.jchb.2012.02.002. DOI: 10.1016/j.jchb.2012.02.002. PMID: 22425585.


24. Klingenberg CP. 2016; Size, shape, and form: concepts of allometry in geometric morphometrics. Dev Genes Evol. 226:113–37. https://doi.org/10.1007/s00427-016-0539-2. DOI: 10.1007/s00427-016-0539-2. PMID: 27038023. PMCID: PMC4896994.


25. Rohlf FJ, Corti M. 2000; Use of two-block partial least-squares to study covariation in shape. Syst Biol. 49:740–53. https://doi.org/10.1080/106351500750049806. DOI: 10.1080/106351500750049806. PMID: 12116437.


26. Gkantidis N, Halazonetis DJ. 2011; Morphological integration between the cranial base and the face in children and adults. J Anat. 218:426–38. https://doi.org/10.1111/j.1469-7580.2011.01346.x. DOI: 10.1111/j.1469-7580.2011.01346.x. PMID: 21323666. PMCID: PMC3077525.


27. Wellens HL, Kuijpers-Jagtman AM, Halazonetis DJ. 2013; Geometric morphometric analysis of craniofacial variation, ontogeny and modularity in a cross-sectional sample of modern humans. J Anat. 222:397–409. https://doi.org/10.1111/joa.12027. DOI: 10.1111/joa.12027. PMID: 23425043. PMCID: PMC3610033.


28. Katsadouris A, Halazonetis DJ. 2017; Geometric morphometric analysis of craniofacial growth between the ages of 12 and 14 in normal humans. Eur J Orthod. 39:386–94. https://doi.org/10.1093/ejo/cjw070. DOI: 10.1093/ejo/cjw070. PMID: 27940444.


29. Zollikofer CP, Ponce De León MS. 2002; Visualizing patterns of craniofacial shape variation in Homo sapiens. Proc Biol Sci. 269:801–7. https://doi.org/10.1098/rspb.2002.1960. DOI: 10.1098/rspb.2002.1960. PMID: 11958711. PMCID: PMC1690963.


30. Bastir M, Rosas A. 2004; Facial heights: evolutionary relevance of postnatal ontogeny for facial orientation and skull morphology in humans and chimpanzees. J Hum Evol. 47:359–81. https://doi.org/10.1016/j.jhevol.2004.08.009. DOI: 10.1016/j.jhevol.2004.08.009. PMID: 15530353.


31. Nielsen IL. 1991; Vertical malocclusions: etiology, development, diagnosis and some aspects of treatment. Angle Orthod. 61:247–60. https://pubmed.ncbi.nlm.nih.gov/1763835/. Erratum in: Angle Orthod 1992;62:87. DOI: 10.1043/0003-3219(1991)061<0247:VMEDDA>2.0.CO;2. PMID: 1763835.
32. Manlove AE, Romeo G, Venugopalan SR. 2020; Craniofacial growth: current theories and influence on management. Oral Maxillofac Surg Clin North Am. 32:167–75. https://doi.org/10.1016/j.coms.2020.01.007. DOI: 10.1016/j.coms.2020.01.007. PMID: 32151371.


33. Enlow DH, Pfister C, Richardson E, Kuroda T. 1982; An analysis of Black and Caucasian craniofacial patterns. Angle Orthod. 52:279–87. https://pubmed.ncbi.nlm.nih.gov/6961829/. DOI: 10.1043/0003-3219(1982)052<0279:AAOBAC>2.0.CO;2. PMID: 6961829.
34. Bastir M, Rosas A, Kuroe K. 2004; Petrosal orientation and mandibular ramus breadth: evidence for an integrated petroso-mandibular developmental unit. Am J Phys Anthropol. 123:340–50. https://doi.org/10.1002/ajpa.10313. DOI: 10.1002/ajpa.10313. PMID: 15022362.


35. Smith RJ, Josell SD. 1984; The plan of the human face: a test of three general concepts. Am J Orthod. 85:103–8. https://doi.org/10.1016/0002-9416(84)90001-0. DOI: 10.1016/0002-9416(84)90001-0. PMID: 6594050.


36. Bastir M, Rosas A. 2005; Hierarchical nature of morphological integration and modularity in the human posterior face. Am J Phys Anthropol. 128:26–34. https://doi.org/10.1002/ajpa.20191. DOI: 10.1002/ajpa.20191. PMID: 15778978.


37. Mehta S, Arqub SA, Sharma R, Patel N, Tadinada A, Upadhyay M, et al. 2022; Variability associated with mandibular ramus area thickness and depth in subjects with different growth patterns, gender, and growth status. Am J Orthod Dentofacial Orthop. 161:e223–34. https://doi.org/10.1016/j.ajodo.2021.10.006. DOI: 10.1016/j.ajodo.2021.10.006. PMID: 34802867.


38. Knigge RP, McNulty KP, Oh H, Hardin AM, Leary EV, Duren DL, et al. 2021; Geometric morphometric analysis of growth patterns among facial types. Am J Orthod Dentofacial Orthop. 160:430–41. https://doi.org/10.1016/j.ajodo.2020.04.038. DOI: 10.1016/j.ajodo.2020.04.038. PMID: 34175161. PMCID: PMC8405563.


39. Aki T, Nanda RS, Currier GF, Nanda SK. 1994; Assessment of symphysis morphology as a predictor of the direction of mandibular growth. Am J Orthod Dentofacial Orthop. 106:60–9. https://doi.org/10.1016/S0889-5406(94)70022-2. DOI: 10.1016/S0889-5406(94)70022-2. PMID: 8017351.


40. Alarcón JA, Bastir M, García-Espona I, Menéndez-Núñez M, Rosas A. 2014; Morphological integration of mandible and cranium: orthodontic implications. Arch Oral Biol. 59:22–9. https://doi.org/10.1016/j.archoralbio.2013.10.005. DOI: 10.1016/j.archoralbio.2013.10.005. PMID: 24192112.


41. Enlow DH, DiGangi D, McNamara JA Jr, Mina M. 1988; An evaluation of the morphogenic and anatomic effects of the functional regulator utilizing the counterpart analysis. Eur J Orthod. 10:192–202. https://doi.org/10.1093/ejo/10.3.192. DOI: 10.1093/ejo/10.3.192. PMID: 3181298.


42. Fan Y, Penington A, Kilpatrick N, Hardiman R, Schneider P, Clement J, et al. 2019; Quantification of mandibular sexual dimorphism during adolescence. J Anat. 234:709–17. https://doi.org/10.1111/joa.12949. DOI: 10.1111/joa.12949. PMID: 30834524. PMCID: PMC6481415.


43. Sharma M, Gorea RK, Gorea A, Abuderman A. 2016; A morphometric study of the human mandible in the Indian population for sex determination. Egypt J Forensic Sci. 6:165–9. https://doi.org/10.1016/j.ejfs.2015.01.002. DOI: 10.1016/j.ejfs.2015.01.002. PMID: df5aacfc90f04f129c073c794b316c23.


Figure 1
Lateral cephalogram presenting 15 landmarks, 51 semi-landmarks, and five curves organized in three datasets: ramus (green), rest of the face (blue), and whole configuration (green and blue). Fixed landmarks are denoted with squares: Nasion; Rhinion; the most posterior point of the frontonasal suture; the most inferior and posterior points on the alveolar portion of the premaxilla; posterior nasal spine; anterior nasal spine; Supradentale; the most superior and posterior points on the alveolar lingual portion; Infradentale; Menton; Antegonial notch; Gonion; the most posterior point of the condyle; the most inferior point on the anterior margin of ramus posterior to second molars, and Coronion. The semi-landmarks are denoted with circles.
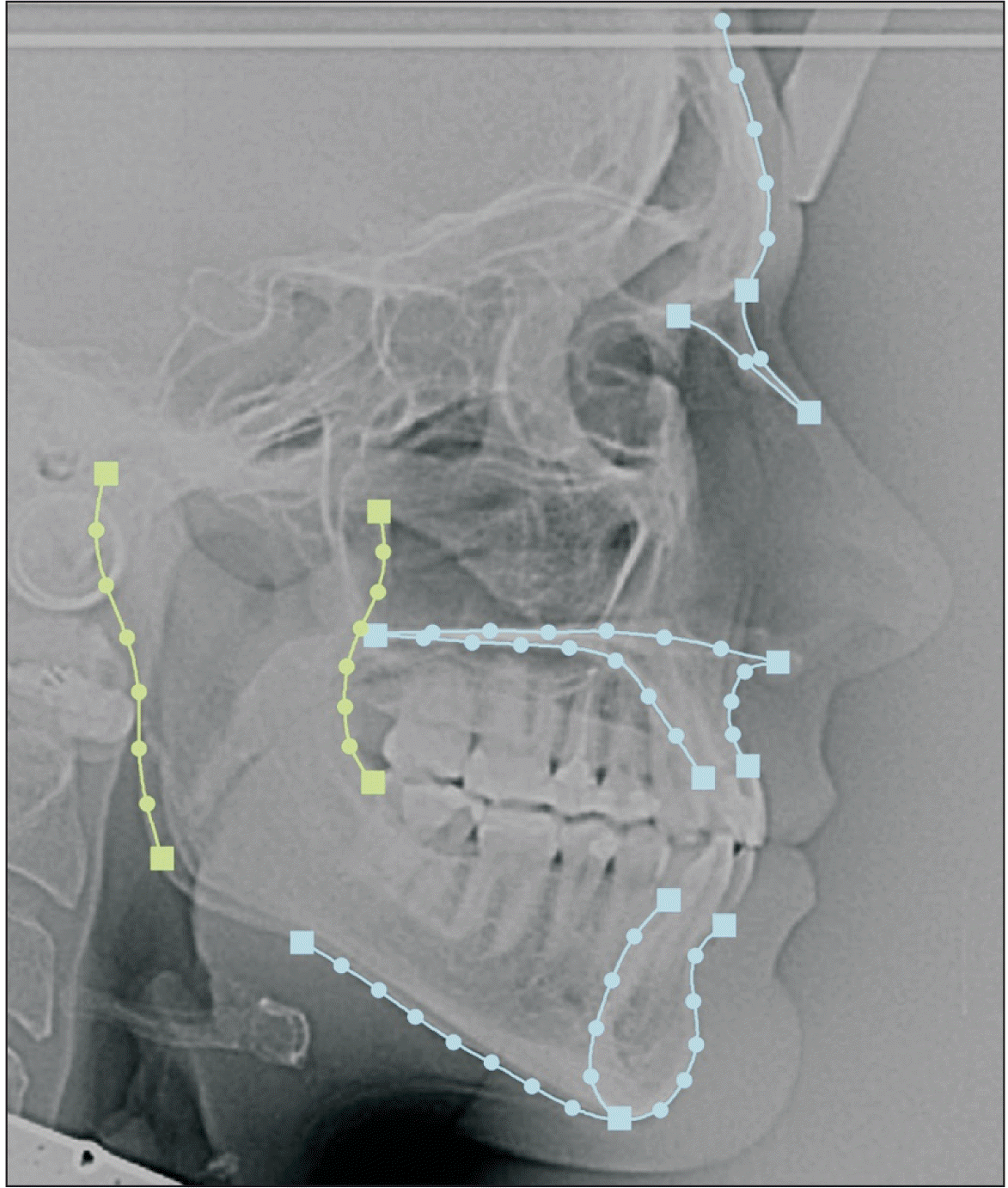
Figure 2
On the left, plots of PC1 and PC2 colored by sex; yellow dots represent females, and blue dots represent males. On the right, the shape changes from the average configuration of all individuals. The average configuration is colored black, and changes are represented in blue. Scaling in the positive and negative directions corresponds to the extreme values of the respective principal axes.
PC, principal component.
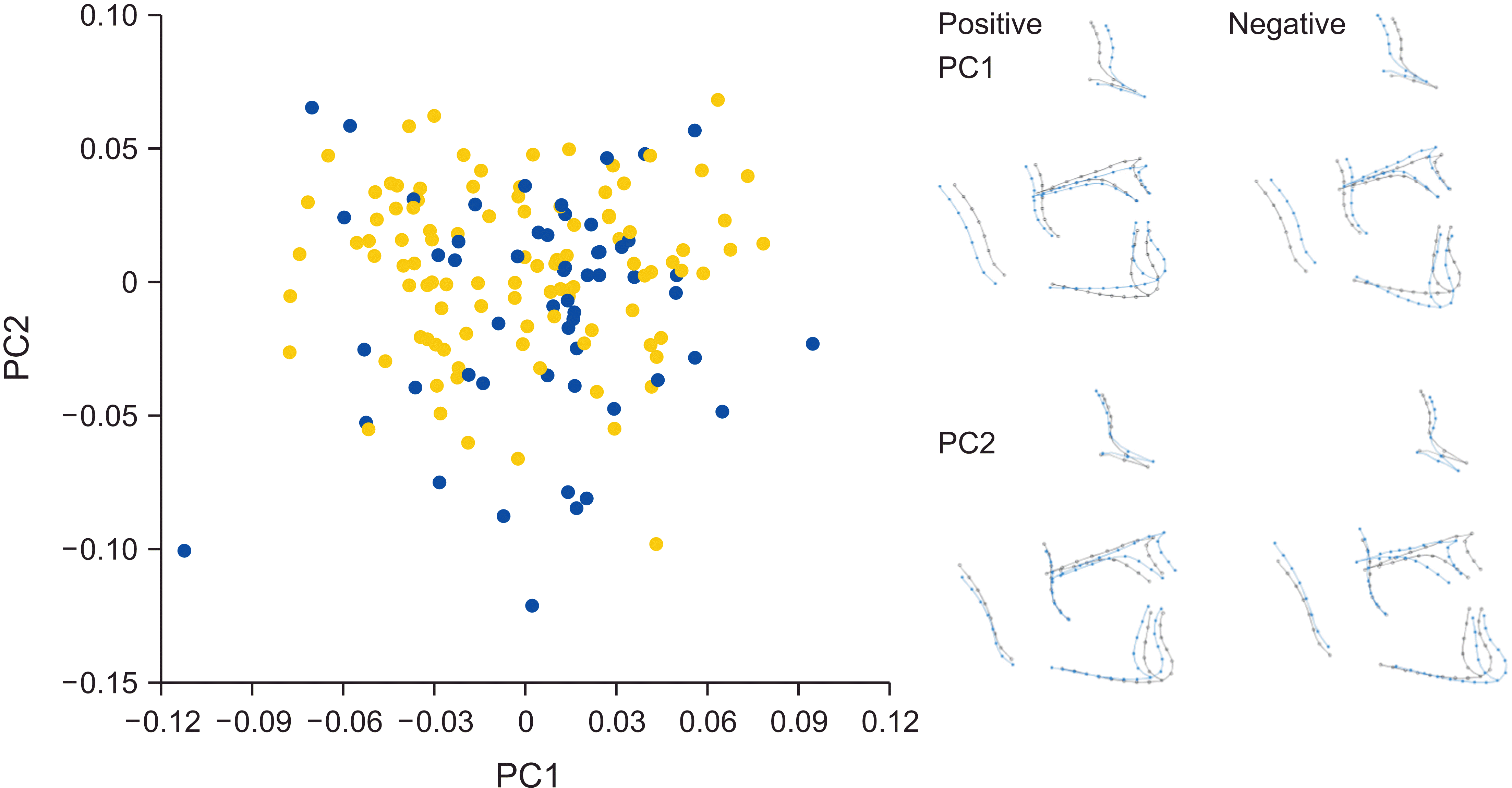
Figure 3
Plots of PLS scores for ramus (Block 1) and rest of the face (Block 2) explaining 45.5% and 25.5% of the total covariance for PLS 1 and PLS 2, respectively. Blue dots represent males, and yellow dots represent females. The associated outline drawings depict the covariance pattern between the aforementioned structures at a scale of 0.12 for Block 1 and 0.1 for Block 2. The average outline shape is depicted in black and shape changes in blue.
PLS, partial least squares.
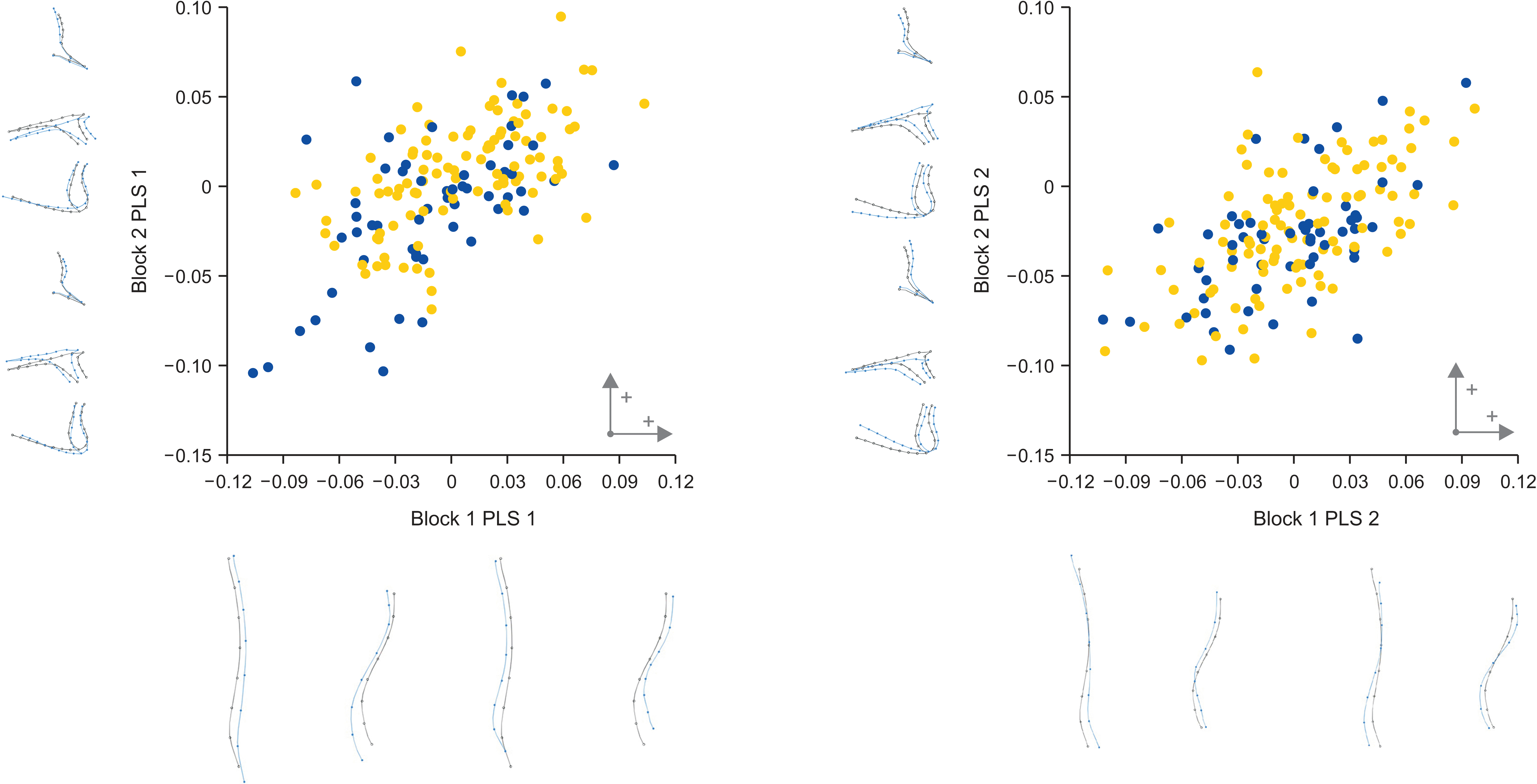
Table 1
Sexual dimorphism in shape between group means by sex
Total sum of squares | Within group sum of squares | Pseudo-F | p-value | |
---|---|---|---|---|
Whole configuration | 0.910 | 0.885 | 4.442 | 0.0001 |
Ramus | 1.208 | 1.185 | 3.047 | 0.0102 |
Rest of the face | 0.823 | 0.792 | 6.121 | 0.0001 |
Table 2
Sexual dimorphism in size between group means by sex
Table 3
Multivariate regression of shape (Procrustes coordinates) on size (InCS) to assess the significance of allometry
Variance explained | p-value from permutation | |
---|---|---|
Whole configuration |
3.4% | < 0.0001 |
Ramus | 2.2% | 0.0028 |
Rest of the face | 6.0% | < 0.0001 |
Table 4
Two-block PLS analyses between ramus and rest of the face
RV coefficient | p-value from permutation | Score | Correlation r | Covariance explained | p-value from permutation | |
---|---|---|---|---|---|---|
Allometric effect not removed | 0.22 | < 0.0001*** | PLS 1 | 0.59 | 45.5% | < 0.0001*** |
PLS 2 | 0.59 | 25.5% | < 0.0001*** | |||
Allometric effect removed | 0.19 | < 0.0001*** | PLS 1 | 0.51 | 43.7% | < 0.0001*** |
PLS 2 | 0.61 | 25.1% | < 0.0001*** | |||
Females only | 0.22 | < 0.0001*** | PLS 1 | 0.69 | 41.9% | < 0.0001*** |
PLS 2 | 0.54 | 25.5% | < 0.0001*** |