Abstract
Preeclampsia (PE) is a multisystem disorder that is an important cause of maternal and perinatal deaths. Currently, delivery is the only final treatment for PE. This practice is usually accompanied by premature birth, which inevitably increases neonatal morbidities. Aspirin is a non-selective non-steroidal anti-inflammatory drug that irreversibly inhibits cyclooxygenase enzymes involved in converting arachidonic acid to prostaglandins and thromboxane. Aspirin inhibits thromboxane A2 production via platelet aggregation, thereby increasing the prostacyclin/thromboxane A2 ratio and reducing platelet aggregation. Since the first case report of aspirin’s potential use during pregnancy was reported in 1978, many studies have attempted to confirm the effect of aspirin on PE, and the results have been controversial. However, this preventive strategy is generally accepted in clinical practice. As evidence for aspirin’s prevention of PE has been accumulating, a recent study investigated the effectiveness of aspirin at high doses of 150 mg, which is higher than before. However, there is an ongoing debate about how much aspirin should be used during pregnancy and when to start aspirin therapy. Guidelines for the use of prophylactic aspirin during pregnancy vary slightly among countries and groups. In this article, we review and summarize the evidence regarding the use of aspirin for PE prevention.
Preeclampsia (PE) is a multisystem disorder that is an important cause of maternal and perinatal deaths. PE accounts for 2–4% of pregnancies worldwide and annually causes 60,000 maternal fatalities worldwide [1]. This multisystem inflammatory syndrome is defined as high blood pressure with proteinuria, thrombocytopenia, renal failure, liver dysfunction, pulmonary edema, and cerebral or visual symptoms after 20 weeks of gestation [2]. One-third of all PE cases are accompanied by preterm birth, and its association with fetal growth restriction and premature birth can often have lifelong effects on children, including a higher risk of cerebral palsy, delayed neurodevelopment, respiratory problems, high blood pressure, kidney dysfunction, and insulin resistance [3,4]. Furthermore, mothers with a history of PE are at a higher risk of future hypertension and cardiovascular disease than mothers without PE in their pregnancies [5,6]. Currently, delivery is the only final treatment for PE. Moreover, this practice is usually accompanied by premature birth, which inevitably increases neonatal morbidities. Continuing research on the pathogenesis and causes of PE has led to an interest in new drugs for the treatment or prevention of the disease.
Aspirin is a widely accessible, affordable, and safe medication used for the treatment of pregnant women and newborns. Although a randomized trial evaluated prophylactic aspirin use to avoid PE, the ideal dose remains uncertain and debatable. Recently, the American College of Obstetricians and Gynecologists (ACOG) released guidelines for the use of aspirin during pregnancy, which include prophylaxis for women at high risk for PE and women with one or more of several moderate risk factors for PE [7,8]. The potential benefit of taking aspirin during pregnancy to reduce the risk of PE has been examined in numerous heterogeneous studies, with notable variations in the risk profiles of the included population, the dosage of the medication, the gestational age at which prophylaxis was started, and the definition of the disease [9]. In this article, we evaluated and summarized the research on aspirin’s use in preventing PE.
PE is divided into early- and late-onset PE types. Delivery before 34 weeks was considered early onset, whereas delivery after 34 weeks was considered late onset. As the treatment of preeclampsia involves placental expulsion following delivery, the placenta is recognized as the major pathophysiological cause of early- and late-onset PE [10].
Preeclampsia is regarded as a two-stage illness, occurring early in pregnancy, with the first stage being marked by abnormal trophoblast infiltration and modification of the uterine spiral arteries. Extravillous trophoblast (EVT) cell invasion marks the initiation of placentation. Before reaching the arteries and myometrium, cells infiltrate the decidual stroma. The muscular layer of the spiral arteries is removed during normal pregnancy, and the maternal endothelial cells are replaced with EVTs. As a result, these vessels widen and lose their capacity to become narrow. To maintain appropriate perfusion of the intervillous area, a low-resistance, high-flow circulatory system must be created by the remodeling of spiral arteries, which is a significant factor in placental formation [11]. Poor placentation is thought to cause placental ischemia, which increases the concentration of anti-angiogenic substances in the mother’s serum. To promote placental function, maternal vasoconstriction increases maternal blood pressure. Due to its antiangiogenic effects, poor placental perfusion also causes a decrease in the levels of placental factors (placental growth factor and vascular endothelial growth factor) in maternal serum [12].
Inadequate perfusion and placental ischemia evoke an inflammatory response that activates cyclooxygenase (COX) [13]. As a result, thromboxane A2 (TXA2) levels increase and endothelial cell prostacyclin (PGI2) levels decrease [13,14]. TXA2 induces platelet aggregation and vasoconstriction, whereas PGI2 counteracts these effects [15]. Therefore, this imbalance plays a significant role in the clinical symptoms of PE [16], and the inflammatory response contributes to the disease phenotype.
The primary mechanism by which aspirin inhibits prostaglandin synthesis is the irreversible inactivation of COX [17]. Prostaglandin H (PGH2) synthase-1, also known as COX-1, catalyzes the first stage in the conversion of arachidonic acid into PGH2. PGH2 is converted to thromboxane A2 and A3 by thromboxane synthase and to PGI2 by prostacyclin synthase. Lipoxygenase converts arachidonic acid into leukotrienes [18].
Salicylic acid is chemically converted into acetylsalicylic acid (aspirin) by acetylation with acetic anhydride. It is a non-selective non-steroidal anti-inflammatory drug because it permanently blocks the COX enzymes that transform arachidonic acid into prostaglandins and thromboxane. Aspirin binds to and acetylates serine residues in the active sites of cyclooxygenase enzymes, which reduces prostaglandin synthesis. It mostly inhibits the constitutive COX-1 enzyme but reduces the inhibition of the induced COX-2 enzyme. The duration of the effects of aspirin depends on the ability of cells to resynthesize COX [19]. COX-1 regulates basal levels of TXA2 and prostacyclin, both of which have opposing effects on endothelium and platelet function [20]. TXA2, a powerful vasoconstrictor and promoter of platelet aggregation, is released from the platelets. In contrast, prostacyclin is the major inhibitory prostanoid for platelet aggregation and is a potent vasodilator [21]. Therefore, the biological effects depend on the equilibrium between endothelial prostacyclin and platelet thromboxane. In contrast, COX-2 is expressed in inflammatory states and is a more important source of prostanoids in inflammatory and proliferative diseases [22]. Aspirin is 170 times more potent as an inhibitor of the COX-1 enzyme than COX-2. Aspirin reduced the effect of TXA2 on platelets by irreversibly acetylating COX-1 at low administration doses without affecting the vascular wall production of prostacyclin [23] (Fig. 1).
The main mechanism of action for low-dose aspirin to prevent PE is the restoration of the disproportionate PGI2/TXA2 ratio. In normal pregnancy, even if there is a normal increase in platelet TXA2, the effect is offset by an increase in PGI2 [24,25]. Therefore, complications related to this procedure do not occur. In contrast, during PE, circulating urine and placental PGI2 levels drop sharply [26,27], resulting in an imbalance in the PGI2/TXA2 ratio, leading to vasoconstriction.
Numerous studies conducted in the early 1950s have shown that aspirin effectively prevents cardiovascular diseases [28]. Goodlin et al. [29] proposed the use of low-dose aspirin (LDA) and unfractionated heparin to prevent recurrent PE in 1978. The first case report described the use of high-dose aspirin (600 mg) 3 times daily for the treatment of recurrent toxemia during pregnancy [29]. One year later, Crandon and Isherwood found that LDA administration during pregnancy may reduce the risk of PE only among primigravidae [30]. According to Masotti et al. [31], in the same year, 2–5 mg/kg of aspirin is likely to prevent platelet aggregation but only marginally decreases prostacyclin synthesis. Subsequent observational studies have revealed that aspirin use during pregnancy may influence PE development [32].
The first randomized trial evaluating the effect of aspirin on placental-mediated complications was reported by Beaufils et al. [33], in which 102 high-risk patients with PE and/or fetal growth retardation were randomly allocated to treatment with 300 mg of dipyridamole and 150 mg of aspirin daily from 12 weeks of gestational age or to the control group. The study found that the aspirin therapy group had a normal pregnancy rate that was twice as high as that of the control group. Subsequently, in 1991, several randomized, double-blind trials were conducted to examine the effectiveness of aspirin for these indications. A multicenter, randomized, double-blind trial (the efficacy of low-dose aspirin in preventing fetal growth retardation [EPREDA] trial) was conducted to determine whether LDA treatment (150 mg/day) could prevent the perinatal outcome of PE [34]. The EPREDA trial revealed a substantial difference between the treatment and placebo groups in terms of birth weight and the occurrence of prenatal growth retardation. In 1994, a large randomized study (the collaborative low-dose aspirin study in pregnancy trial) was conducted to investigate the effects of antenatal aspirin on the occurrence of PE and intrauterine growth restriction [35]. Between 12 and 32 weeks, 9,364 women with a history of preeclampsia or intrauterine growth restriction (IUGR) or at risk for PE were included. They were then randomized to receive either a placebo or a daily dose of 60 mg of aspirin. In the therapy group, there was a negligible 12% decrease in the likelihood of PE, and those who began prophylaxis before 20 weeks of gestation experienced a greater reduction in preeclampsia with aspirin use. However, the incidence of PE or IUGR in women did not significantly decrease with daily aspirin therapy (60 mg). Subsequently, Caritis et al. [36] conducted a double-blind randomized trial and found no benefit in prescribing aspirin 60 mg at 13–26 weeks of gestation. However, the patients included in their study generally applied a wide range of aspirin prescription indications, and >50% of the patients started aspirin therapy after 20 weeks of gestation (Table 1).
Previous studies have raised questions regarding aspirin use in obstetrics. A meta-analysis of the effectiveness of preventive aspirin in the high-risk group for PE was performed in 2001 by Duley et al. [37]. They discovered that the risk of developing PE was significantly reduced by 15% in the antiplatelet group (60–160 mg). Additionally, the risks of preterm birth and fetal or neonatal death were reduced by 8% and 14%, respectively [37]. In 2007, a meta-analysis of individual patient data on the impact of antiplatelet medications (including 24 randomized controlled trials with aspirin alone) on the risk of PE was conducted by Askie et al. [38]. The analysis showed a 10% reduction in the relative risk of developing PE, delivering before 34 weeks of gestation, and having unfavorable outcomes. However, the limitations of the study were that the definitions of PE used in the included studies varied widely and that the aspirin dose used in most studies was less than 100 mg. In 2010, meta-analyses of the combined data showed that PE and IUGR were significantly reduced in the group taking LDA from week 16 or earlier. However, when LDA was administered after 16 weeks, no significant reduction in PE or IUGR was observed. Severe PE was also significantly reduced in the group that received LDA in week 16 or earlier [39]. These results support the concept that deep placental abnormalities that manifest at or before 16 weeks of gestation are linked to severe and preterm manifestations of the disease and that LDA enhances deep placentation. In 2017, Meher et al. [40] published a meta-analysis of the data of individual participants. Based on 16 weeks of gestation, this meta-analysis assessed the impact of aspirin on PE and complications, depending on the initiation of treatment [40]. Their findings revealed no difference in the risk of PE and its consequences regardless of when antiplatelet therapy was started (<16 weeks or >16 weeks).
The disparate and diverse nature of earlier study findings has prompted researchers to conduct well-organized, large-scale studies. The ASPRE study was a double-blind, randomized, placebo-controlled trial involving multiple centers. The greatest heterogeneity in previous studies was observed in the selection of high-risk groups. Traditionally, high-risk screening has been used to identify the risk factors for PE and has been highlighted in most guidelines. However, with different definitions of high-risk factors, high-risk group settings were applied differently for each study, which was the biggest reason for the heterogeneity of each study result. In addition, this screening method has the major weakness of low accuracy.
In the ASPRE trial, singleton pregnant women were screened at 11–13 weeks of gestation using an algorithm that considers the following maternal factors: mean arterial pressure (MAP), uterine artery pulsatility index (UtA-PI), placental growth factor (PLGF), and pregnancy-associated plasma protein A. Screening was performed for 26,941 pregnancies [41]. This predictive algorithm was prospectively verified in an independent cohort before beginning randomized trials and demonstrated a predictive performance comparable to that observed in algorithm development studies. Women were considered to be at a high risk of preterm PE if their estimated risk was ≥100, with a positive screening rate of 11%.
Similar to the previous study, the ASPRE trial was also intended to determine whether aspirin use during pregnancy had a preventive effect on PE. The high-risk group was selected through a combined screening test in the first trimester of pregnancy, and aspirin was started in the first trimester of pregnancy. The main finding of this study was the prevalence of preterm PE (delivery before 37 weeks of gestation) in high-risk patients [41].
Based on research showing that 81 mg of aspirin per day had no effect on approximately 30% of women, this study led to higher doses of aspirin. High-risk women were assigned to either the aspirin group or the placebo group from to 11–14 weeks gestation to 36 weeks gestation. In the ASPRE trial, aspirin administration was set for bedtime because previous studies showed better control of ambulatory blood pressure when aspirin was administered at night [42].
Of the 26,941 pregnant women screened, 2,641 were at high risk for PE and were included. Of the included patients, 1,776 were randomly assigned to receive medication or a placebo. The primary outcome, preterm PE, had an onset in 13 patients (1.6%) in the aspirin group and 35 patients (4.3%) in the placebo group. Aspirin reduced the incidence of early onset PE, which required delivery before 34 weeks of gestation, by 82%. The subgroup analysis showed that the effect of aspirin on PE was prominent in nulliparous women. Prophylactic aspirin had no effect on term PE. There was no difference in the incidence of adverse neonatal outcomes or other adverse events between the two groups [41].
Several obstetrics and gynecology organizations have recommended the use of LDA to prevent PE in high-risk pregnant women. However, there is debate over the ideal aspirin dosage for preventing PE. In studies on aspirin for PE prophylaxis, the dose was varied from 60 to 150 mg/day. In 2011, according to the World Health Organization, women at high risk for PE should take LDA (75 mg) before 20 weeks of pregnancy [43]. In 2013, the ACOG issued a recommendation that began at the end of the first trimester: high-risk women were recommended to take 60–80 mg of aspirin daily [2,44]. In 2019, based on the findings of the meta-analysis, the United States Preventive Services Task Force (USPSTF) recommendation statement and the ACOG suggested that high-risk mothers for PE began taking a modest dose of prophylactic aspirin (81 mg) for 12 weeks [45]. A recent meta-analysis showed that aspirin had a dose-dependent effect depending on the timing of administration [46]. A dose-response effect on the prevention of PE and fetal growth restriction (FGR) was observed only when aspirin was started at a gestational age of less than 16 weeks. In terms of prophylaxis for PE and FGR, aspirin administered at a higher dose (100 mg) was considerably more efficient than aspirin administered at a lower dose (60 mg). Aspirin resistance or maternal body mass index (BMI) is assumed to be the cause of these dose-response effects, which can be overcome with higher dosages.
As PE is a placental-related disease, it is effective to administer aspirin from the early stages of placental development to prevent the disease. However, the optimal timing for aspirin use remains unclear. Chaemsaithong et al. [47] performed a meta-analysis to investigate the effect of LDA initiated in early pregnancy (less than 11 weeks) on the risk of hypertensive disorders during pregnancy. A total of 1,426 participants from eight randomized controlled trials were included in this meta-analysis. They found that early administration of LDA (less than 11 weeks gestation) did not reduce the risk of pregnancy complications [47]. On the same topic, Roberge et al. [48] also released a meta-analysis of randomized trials. They discovered that aspirin use from the time of pregnancy until at least 16 weeks was associated with significantly lower rates of PE and FGR. After 16 weeks of pregnancy, there was no discernible benefit of aspirin for PE or FGR [48]. According to the timing and dose of aspirin introduction, a recent meta-analysis that assessed the preventive use of aspirin examined the impact of aspirin on the prevention of preterm and term PE. It was concluded that aspirin reduced only the risk of preterm PE, not term PE and had a prophylactic effect only when started at <16 weeks of gestation and administered at a daily dose of ≥100 mg [48].
Preterm PE has relative risks of 8.7 and 9.1 for dichorionic twin pregnancies and monochorionic twin pregnancies, respectively, in women with multiple pregnancies compared with those with singleton pregnancies [49]. Professional organization guidelines identify multiple pregnancies as a risk factor for PE and advise aspirin prophylaxis in these circumstances.
However, contradictory recommendations result from disparate national guidelines exist for LDA prevention in twin pregnancies (Table 2). According to a systematic review and a meta-analysis of six randomized controlled trials with 898 multiple pregnancies, aspirin at doses between 60 mg and 100 mg significantly reduced the risk of PE and mild PE but not severe PE [50]. In the same year, PE decreased from 16% to 6% in a prospective study of twin pregnancies that randomly assigned women to take 100 mg/day of aspirin or a placebo before 16 weeks (a finding that was amplified in the twin pregnancy cohort with high human chorionic gonadotropin levels and a threshold of 29.96 iu/mL) [51]. Recently, this was a retrospective cohort study. It compared hypertensive outcomes in three groups of twins: no aspirin, aspirin 75 mg/day, and aspirin 150 mg/day. Although there are limitations to the retrospective study design, the incidence of hypertensive disorders in twin pregnancies with additional risk factors for PE was significantly lower in those receiving aspirin at 150 mg/day compared to 75 mg/day [52]. Many recommendations have suggested aspirin prophylaxis as a general measure during multiple gestations owing to an increased risk of PE in twin pregnancies. Even in nations with national standards, doctors do not advocate universal prophylaxis, and up to 15% of doctors decide to administer aspirin to all twins’ mothers [53]. However, a sufficiently powered randomized controlled study is urgently required to consider the paucity of information on the effectiveness of aspirin in avoiding preeclampsia in twins.
Although still debatable, screening for pregnant women who might benefit from aspirin to avoid preeclampsia is a significant topic. The most prevalent strategy is to assess the clinical risk factors early in pregnancy. The National Institute for Health and Care Excellence (NICE) guidelines on hypertension in pregnancy recommend that women at high risk of preeclampsia start taking low-dose aspirin at 12 weeks of gestation and continue taking it until birth. High-risk women were eligible if they had one of the following factors: hypertensive disease from a previous pregnancy, pre-existing hypertension, renal disease, diabetes mellitus, or an autoimmune disease. The same prophylactic aspirin therapy was administered to women with at least one moderate risk factor for preeclampsia. Factors indicating moderate risk were age >40 years, family history of PE, BMI >35 kg/m2, nulliparity, and an interpregnancy interval >10 years [54]. In 2013, the ACOG recommended that women at high risk for preeclampsia begin taking LDA in the late first trimester and continue daily [54]. Women who had experienced preterm birth at less than 34 weeks of gestation and early onset PE, or who had one or more previous pregnancies complicated by PE, were considered to be at high risk for preeclampsia. The following year, the USPSTF published guidelines for indications for the use of low-dose aspirin, and the list of indications was broader [55]. The USPSTF recommends that women with a history of PE in a previous pregnancy, type 1 or 2 diabetes, chronic hypertension, multiple pregnancies, autoimmune diseases, and kidney disease be administered low-dose aspirin prophylaxis at 81 mg/day between 12 weeks and 28 weeks of gestation (ideally at 16 weeks of gestation), which is continued every day until birth. Other factors associated with increased PE risk include nulliparity, a high pre-pregnancy BMI, family history of PE, and advanced maternal age (≥35 years). Additionally, black people have higher rates of PE and are at an increased risk of serious complications owing to various societal and health problems [55] (Table 2).
Screening strategies based on clinical risk factors have been associated with poor outcomes. With the NICE method, the detection rate for all-PE was 30.4%, the rate for preterm PE was 40.8%, and the screening test-positive rate was 10.3% [56]. Screening based on the 2013 ACOG recommendation achieved detection rates of only 5% and 2% for preterm and term PE, respectively, with a 0.2% false-positive rate [57]. USPSTF recommendations with a more extensive list of clinical risk factors showed detection rates of 90% (95% confidence interval [CI]: 7,996) and 89% (95% CI, 84–94) for early and late PE, respectively. This was a significant improvement over other screening tests; however, the false positive rate (FPR) also increased by 64% [58]. Therefore, screening using a maternal risk factor approach performed poorly in identifying women at high risk for PE.
Significant efforts have been made to identify pertinent biomarkers that can predict PE in the first trimester of pregnancy. When predicting preeclampsia, combinations of numerous biomarkers outperform single biomarkers in terms of accuracy [59]. The Fetal Medicine Foundation (FMF) developed a multi-marker prediction algorithm, namely the first trimester combined test for PE. The FMF first trimester combined test uses the Bayes theorem to combine maternal a priori risk based on maternal characteristics, obstetrics, and medical history with a “triple test,” which entails the measurement of serum PLGF, MAP, and UtA-PI at gestational age 11–13+6 weeks [60]. Compared to the conventional approach, this method offers better screening performance [61]. Using the algorithm for the early PE, 90%, 75%, and 47% of the early, late, and term PE were estimated at an FPR of 10% [61,62]. This predictive model has also been used in the ASPRE study. The ASPRE trial, which screened 26,941 singleton pregnancies for preterm PE, achieved detection rates of 77% and 43% for preterm and term PE, respectively, with an FPR of 9.2% after adjusting for the effect of aspirin [63]. The largest study on the creation of an FMF first-trimester combination test utilizing a competitive risk model was conducted by Tan et al. in 2018 [64]. PE developed in 1,770 (2.9%) of the 61,174 pregnant women in this study. Combined screening predicted 90%, 75%, and 41% of early PE, preterm PE, and term PE cases, respectively, at a screen-positive rate of 10%. The screening performance was determined based on the racial origin of the woman. Using a risk cut-off of 1/100 for preterm PE in Caucasian women, the positive screening rate was 10%, and the detection rates were 88%, 69%, and 40% for early, preterm, and term PE, respectively. Using the same screening method and risk cutoff for women of African and Caribbean descent, the positive screening rate was 34%, and the detection rates were 100%, 92%, and 75% for early, preterm, and term PE, respectively [64].
The FMF algorithm performed during early pregnancy is the best method for screening for early PE. High-risk women with PE will be followed up regularly during pregnancy for the early detection of PE. Regular prenatal pregnancy management is also important for women who undergo early pregnancy screening and are deemed low-risk, because late preeclampsia can still occur in these women.
The value of sFlt-1 and PLGF in immediate PE prediction has already been demonstrated in numerous studies, and several studies have investigated the diagnosis of early- and late-onset PE using the sFlt-1/PLGF ratio [65,66]. The prediction of short-term outcome in pregnant women with suspected preeclampsia study was an international, prospective, observational study that examined the use of the sFlt-1/PLGF ratio to determine whether PE is present within 1 week and the presence of PE within 4 weeks in women with a clinical suspicion of PE. An international, prospective, observational study [67] with 80% sensitivity and 78.3% specificity showed that an sFlt-1/PLGF ratio (≤38) was 99.3% predictive of no PE in the following week. An sFlt-1/PLGF ratio greater than 38 had a positive predictive value (PPV) of 36.7% for predicting the occurrence of PE within 4 weeks. Similar results have been reported in 764 pregnant Asian women with suspected preeclampsia [67]. For sFlt-1/PLGF ratios ≤38, the negative predictive value to rule out preeclampsia within 1 week was 98.6% (95% CI, 97.2–99.4%), and the PPV for preeclampsia within 4 weeks was 30.3% (95% CI, 23.0–38.5%) when the sFlt-1/PLGF ratio was greater than 38. According to these studies, sFlt-1 and PLGF are helpful biomarkers for the short-term prediction and detection of preeclampsia progression in women with clinical signs and symptoms of the disorder and have high negative predictive values.
The use of sFlt-1 and PLGF in the risk classification of women at low risk of preeclampsia has been the subject of several studies. Using a competitive risk approach, FMF developed an algorithm that combined maternal factors, UtA-PI, MAP, and serum PLGF to assess pregnant women who were asymptomatic and at low risk of PE during the second and third trimesters [68]. In a study involving 16,254 women who underwent ultrasound examinations between weeks 19 and 24 as part of standard pregnancy treatment, this approach was proven to be effective. The proportion of PE cases at <32 weeks of pregnancy in the high-risk group was approximately 100% for screening based on maternal factors, MAP, UtA-PI, and PLGF. This indicates that the risk of preeclampsia can be assessed at 19–24 weeks of gestation in women in the low-risk group for preeclampsia during early pregnancy screening, and these women can be stratified into high-, intermediate-, and low-risk groups. Within 32 weeks, preeclampsia is more likely to develop in high-risk women; therefore, intensive monitoring is required. Intermediate-risk women require reevaluation at 32 weeks because of the possibility of preeclampsia between 32 and 36 weeks. Low-risk women only require standard antenatal care for up to 36 weeks [69].
It is now widely accepted that LDA is effective for the secondary prevention of PE in high-risk patients. Aspirin inhibits TXA2 production, thereby restoring the prostacyclin/TXA2 ratio and reducing platelet aggregation. The conventional method of classifying high-risk populations based on maternal traits has been ineffective. However, the effectiveness of screening significantly increases when maternal features are combined with biochemical and biophysical markers. As a result, it is important to identify high-risk pregnant women early, ideally with the aid of prediction algorithms. To identify high-risk women who respond best to aspirin prophylaxis to prevent preterm PE, combined screening using maternal variables, mean arterial pressure, uterine artery Doppler, and serum PLGF can be employed. Although the majority of national organizations advise high-risk women to take LDA (60–80 mg) to prevent PE, the majority of the most recent randomized trials with higher LDA dosages (100–150 mg in early pregnancy) had meaningful outcomes. Future research should assess the ideal LDA dosage and timing in women who have been specifically identified as being at a high risk of developing early onset PE. Growing data indicate that maternal organ dysfunction endures even after delivery, indicating that a history of PE is a distinct cardiovascular risk factor [65,66,70,71]. It is conceivable that aspirin could lower the rates of long-term cardiovascular mortality by avoiding preterm PE.
References
2. Hypertension in pregnancy. Report of the American College of Obstetricians and Gynecologists’ task force on hypertension in pregnancy. Obstet Gynecol. 2013; 122:1122–31.
3. Irving RJ, Belton NR, Elton RA, Walker BR. Adult cardiovascular risk factors in premature babies. Lancet. 2000; 355:2135–6.


4. Moster D, Lie RT, Markestad T. Long-term medical and social consequences of preterm birth. N Engl J Med. 2008; 359:262–73.


5. Wu P, Haththotuwa R, Kwok CS, Babu A, Kotronias RA, Rushton C, et al. Preeclampsia and future cardiovascular health: a systematic review and meta-analysis. Circ Cardiovasc Qual Outcomes. 2017; 10:e003497.
6. Breetveld NM, Ghossein-Doha C, van Neer J, Sengers MJJM, Geerts L, van Kuijk SMJ, et al. Decreased endothelial function and increased subclinical heart failure in women several years after pre-eclampsia. Ultrasound Obstet Gynecol. 2018; 52:196–204.


7. ACOG committee opinion No. 743: low-dose aspirin use during pregnancy. Obstet Gynecol. 2018; 132:e44–52.
8. LeFevre ML; US Preventive Services Task Force. Low-dose aspirin use for the prevention of morbidity and mortality from preeclampsia: US preventive services task force recommendation statement. Ann Intern Med. 2014; 161:819–26.


9. Askie LM, Duley L, Henderson-Smart DJ, Stewart LA. PARIS Collaborative Group. Antiplatelet agents for prevention of pre-eclampsia: a meta-analysis of individual patient data. Lancet. 2007; 369:1791–8.


10. Staff AC, Benton SJ, von Dadelszen P, Roberts JM, Taylor RN, Powers RW, et al. Redefining preeclampsia using placenta-derived biomarkers. Hypertension. 2013; 61:932–42.


11. Phipps EA, Thadhani R, Benzing T, Karumanchi SA. Pre-eclampsia: pathogenesis, novel diagnostics and therapies. Nat Rev Nephrol. 2019; 15:275–89.


12. Herraiz I, Llurba E, Verlohren S, Galindo A. Spanish Group for the Study of Angiogenic Markers in Preeclampsia. Update on the diagnosis and prognosis of preeclampsia with the aid of the sFlt-1/ PlGF ratio in singleton pregnancies. Fetal Diagn Ther. 2018; 43:81–9.


13. Walsh SW. Eicosanoids in preeclampsia. Prostaglandins Leukot Essent Fatty Acids. 2004; 70:223–32.


14. Walsh SW. Preeclampsia: an imbalance in placental prostacyclin and thromboxane production. Am J Obstet Gynecol. 1985; 152:335–40.


15. Moncada S, Vane JR. Arachidonic acid metabolites and the interactions between platelets and blood-vessel walls. N Engl J Med. 1979; 300:1142–7.


16. Mills JL, DerSimonian R, Raymond E, Morrow JD, Roberts LJ 2nd, Clemens JD, et al. Prostacyclin and thromboxane changes predating clinical onset of preeclampsia: a multicenter prospective study. JAMA. 1999; 282:356–62.
18. Bosetti F. Arachidonic acid metabolism in brain physiology and pathology: lessons from genetically altered mouse models. J Neurochem. 2007; 102:577–86.


19. Patrono C. Aspirin and human platelets: from clinical trials to acetylation of cyclooxygenase and back. Trends Pharmacol Sci. 1989; 10:453–8.


20. Crofford LJ. COX-1 and COX-2 tissue expression: implications and predictions. J Rheumatol Suppl. 1997; 49:15–9.
21. Fitzpatrick FA. Cyclooxygenase enzymes: regulation and function. Curr Pharm Des. 2004; 10:577–88.


22. Dubois RN, Abramson SB, Crofford L, Gupta RA, Simon LS, Van De Putte LB, et al. Cyclooxygenase in biology and disease. FASEB J. 1998; 12:1063–73.


23. Clarke RJ, Mayo G, Price P, FitzGerald GA. Suppression of thromboxane A2 but not of systemic prostacyclin by controlled-release aspirin. N Engl J Med. 1991; 325:1137–41.


24. Lewis PJ, Boylan P, Friedman LA, Hensby CN, Downing I. Prostacyclin in pregnancy. Br Med J. 1980; 280:1581–2.


25. Fitzgerald DJ, Mayo G, Catella F, Entman SS, FitzGerald GA. Increased thromboxane biosynthesis in normal pregnancy is mainly derived from platelets. Am J Obstet Gynecol. 1987; 157:325–30.


26. Remuzzi G, Marchesi D, Zoja C, Muratore D, Mecca G, Misiani R, et al. Reduced umbilical and placental vascular prostacyclin in severe pre-eclampsia. Prostaglandins. 1980; 20:105–10.


27. Fitzgerald DJ, Entman SS, Mulloy K, FitzGerald GA. Decreased prostacyclin biosynthesis preceding the clinical manifestation of pregnancy-induced hypertension. Circulation. 1987; 75:956–63.


28. Craven LL. Prevention of coronary and cerebral thrombosis. Miss Valley Med J. 1956; 78:213–5.
29. Goodlin RC, Haesslein HO, Fleming J. Aspirin for the treatment of recurrent toxaemia. Lancet. 1978; 2:51.
30. Crandon AJ, Isherwood DM. Effect of aspirin on incidence of pre-eclampsia. Lancet. 1979; 1:1356.


31. Masotti G, Galanti G, Poggesi L, Abbate R, Neri Serneri GG. Differential inhibition of prostacyclin production and platelet aggregation by aspirin. Lancet. 1979; 2:1213–7.


32. Bussolino F, Benedetto C, Massobrio M, Camussi G. Maternal vascular prostacyclin activity in pre-eclampsia. Lancet. 1980; 2:702.


33. Beaufils M, Uzan S, Donsimoni R, Colau JC. Prevention of pre-eclampsia by early antiplatelet therapy. Lancet. 1985; 1:840–2.


34. Uzan S, Beaufils M, Breart G, Bazin B, Capitant C, Paris J. Prevention of fetal growth retardation with low-dose aspirin: findings of the EPREDA trial. Lancet. 1991; 337:1427–31.


35. CLASP Collaborative Group. CLASP: a randomised trial of low-dose aspirin for the prevention and treatment of pre-eclampsia among 9364 pregnant women. Lancet. 1994; 343:619–29.
36. Caritis S, Sibai B, Hauth J, Lindheimer MD, Klebanoff M, Thom E, et al. Low-dose aspirin to prevent preeclampsia in women at high risk. National Institute of Child Health and Human Development Network of Maternal-Fetal Medicine Units. N Engl J Med. 1998; 338:701–5.


37. Duley L, Henderson-Smart D, Knight M, King J. Antiplatelet drugs for prevention of pre-eclampsia and its consequences: systematic review. BMJ. 2001; 322:329–33.


38. Askie LM, Duley L, Henderson-Smart DJ, Stewart LA; PARIS Collaborative Group. Antiplatelet agents for prevention of pre-eclampsia: a meta-analysis of individual patient data. Lancet. 2007; 369:1791–8.


39. Bujold E, Roberge S, Lacasse Y, Bureau M, Audibert F, Marcoux S, et al. Prevention of preeclampsia and intrauterine growth restriction with aspirin started in early pregnancy: a meta-analysis. Obstet Gynecol. 2010; 116:402–14.
40. Meher S, Duley L, Hunter K, Askie L. Antiplatelet therapy before or after 16 weeks’ gestation for preventing preeclampsia: an individual participant data meta-analysis. Am J Obstet Gynecol. 2017; 216:121–8e2.


41. Rolnik DL, Wright D, Poon LC, O’Gorman N, Syngelaki A, de Paco Matallana C, et al. Aspirin versus placebo in pregnancies at high risk for preterm preeclampsia. N Engl J Med. 2017; 377:613–22.


42. Ayala DE, Ucieda R, Hermida RC. Chronotherapy with low-dose aspirin for prevention of complications in pregnancy. Chronobiol Int. 2013; 30:260–79.


43. World Health Organization (WHO). WHO recommendations or prevention and treatment of pre-eclampsia and eclampsia [Internet]. Geneva: WHO;c2011. [cited 2011 Nov 2 ]. Available from: https://www.who.int/publications/item/9789241548335
.
44. Lisonkova S, Joseph KS. Incidence of preeclampsia: risk factors and outcomes associated with early- versus late-onset disease. Am J Obstet Gynecol. 2013; 209:544e1–544.e12.


45. American College of Obstetricians and Gynecologists. ACOG practice bulletin No. 203: chronic hypertension in pregnancy. Obstet Gynecol. 2019; 133:e26–50.
46. Roberge S, Nicolaides K, Demers S, Hyett J, Chaillet N, Bujold E. The role of aspirin dose on the prevention of preeclampsia and fetal growth restriction: systematic review and meta-analysis. Am J Obstet Gynecol. 2017; 216:110–20e6.


47. Chaemsaithong P, Cuenca-Gomez D, Plana MN, Gil MM, Poon LC. Does low-dose aspirin initiated before 11 weeks’ gestation reduce the rate of preeclampsia? Am J Obstet Gynecol. 2020; 222:437–50.


48. Roberge S, Bujold E, Nicolaides KH. Aspirin for the prevention of preterm and term preeclampsia: systematic review and metaanalysis. Am J Obstet Gynecol. 2018; 218:287–93e1.


49. Francisco C, Wright D, Benkő Z, Syngelaki A, Nicolaides KH. Hidden high rate of pre-eclampsia in twin compared with singleton pregnancy. Ultrasound Obstet Gynecol. 2017; 50:88–92.


50. Bergeron TS, Roberge S, Carpentier C, Sibai B, McCaw-Binns A, Bujold E. Prevention of Preeclampsia with aspirin in multiple gestations: a systematic review and meta-analysis. Am J Perinatol. 2016; 33:605–10.


51. Euser AG, Metz TD, Allshouse AA, Heyborne KD. Low-dose aspirin for pre-eclampsia prevention in twins with elevated human chorionic gonadotropin. J Perinatol. 2016; 36:601–5.


52. Kalafat E, Shirazi A, Thilaganathan B, Khalil A. The role of aspirin in prevention of preeclampsia in twin pregnancies: does the dose matter? Am J Obstet Gynecol. 2020; 223:457–8.


53. Gent J, Nanda S, Khalil A, Sharp A. Antenatal management of multiple pregnancies within the UK: a survey of practice. Eur J Obstet Gynecol Reprod Biol. 2020; 254:74–8.


54. National Collaborating Centre for Women’s and Children’s Health (UK). Hypertension in pregnancy: the management of hypertensive disorders during pregnancy [Internet]. London: RCOG Press;c2010. [cited 2019 Nov 18]. Available from: http://www.ncbi.nlm.nih.gov/books/NBK62652/
.
55. Henderson JT, Vesco KK, Senger CA, Thomas RG, Redmond N. Aspirin use to prevent preeclampsia and related morbidity and mortality: updated evidence report and systematic review for the US preventive services task force. JAMA. 2021; 326:1192–206.


56. O’Gorman N, Wright D, Poon LC, Rolnik DL, Syngelaki A, de Alvarado M, et al. Multicenter screening for pre-eclampsia by maternal factors and biomarkers at 11–13 weeks’ gestation: comparison with NICE guidelines and ACOG recommendations. Ultrasound Obstet Gynecol. 2017; 49:756–60.


57. American College of Obstetricians and Gynecologists. Task force on hypertension in pregnancy. Hypertension in pregnancy. Report of the American College of Obstetricians and Gynecologists’ task force on hypertension in pregnancy. Obstet Gynecol. 2013; 122:1122–31.
58. Tan MY, Wright D, Syngelaki A, Akolekar R, Cicero S, Janga D, et al. Comparison of diagnostic accuracy of early screening for pre-eclampsia by NICE guidelines and a method combining maternal factors and biomarkers: results of SPREE. Ultrasound Obstet Gynecol. 2018; 51:743–50.


59. Poon LC, Nicolaides KH. Early prediction of preeclampsia. Obstet Gynecol Int. 2014; 2014:297397.


60. Akolekar R, Syngelaki A, Poon L, Wright D, Nicolaides KH. Competing risks model in early screening for preeclampsia by biophysical and biochemical markers. Fetal Diagn Ther. 2013; 33:8–15.


61. Chaemsaithong P, Sahota DS, Poon LC. First trimester preeclampsia screening and prediction. Am J Obstet Gynecol. 2022; 226:S1071–97e2.


62. O’Gorman N, Wright D, Syngelaki A, Akolekar R, Wright A, Poon LC, et al. Competing risks model in screening for preeclampsia by maternal factors and biomarkers at 11–13 weeks gestation. Am J Obstet Gynecol. 2016; 214:103e1–103.e12.


63. Rolnik DL, Wright D, Poon LCY, Syngelaki A, O’Gorman N, de Paco Matallana C, et al. ASPRE trial: performance of screening for preterm pre-eclampsia. Ultrasound Obstet Gynecol. 2017; 50:492–5.


64. Tan MY, Syngelaki A, Poon LC, Rolnik DL, O’Gorman N, Delgado JL, et al. Screening for pre-eclampsia by maternal factors and biomarkers at 11–13 weeks’ gestation. Ultrasound Obstet Gynecol. 2018; 52:186–95.


65. Agrawal S, Cerdeira AS, Redman C, Vatish M. Meta-analysis and systematic review to assess the role of soluble FMS-like tyrosine kinase-1 and placenta growth factor ratio in prediction of preeclampsia: the SaPPPhirE study. Hypertension. 2018; 71:306–16.


66. Cerdeira AS, Agrawal S, Staff AC, Redman CW, Vatish M. Angiogenic factors: potential to change clinical practice in pre-eclampsia? BJOG. 2018; 125:1389–95.


67. Bian X, Biswas A, Huang X, Lee KJ, Li TK, Masuyama H, et al. Short-term prediction of adverse outcomes using the sFlt-1 (soluble fms-like tyrosine kinase 1)/PlGF (placental growth factor) ratio in Asian women with suspected preeclampsia. Hypertension. 2019; 74:164–72.


68. Gallo DM, Wright D, Casanova C, Campanero M, Nicolaides KH. Competing risks model in screening for preeclampsia by maternal factors and biomarkers at 19–24 weeks’ gestation. Am J Obstet Gynecol. 2016; 214:619e1–17.


69. Litwinska M, Syngelaki A, Wright A, Wright D, Nicolaides KH. Management of pregnancies after combined screening for pre-eclampsia at 19–24 weeks’ gestation. Ultrasound Obstet Gynecol. 2018; 52:365–72.
70. Salihu HM, Garcia BY, Dongarwar D, Maiyegun SO, Yusuf KK, Agili DEA. Maternal pre-pregnancy underweight and the risk of small-for-gestational-age in Asian-American ethnic groups. Obstet Gynecol Sci. 2021; 64:496–505.


71. Wadhwani P, Saha PK, Kalra JK, Gainder S, Sundaram V. A study to compare maternal and perinatal outcome in early vs. late onset preeclampsia. Obstet Gynecol Sci. 2020; 63:270–7.


72. Ebrashy A, Ibrahim M, Marzook A, Yousef D. Usefulness of aspirin therapy in high-risk pregnant women with abnormal uterine artery Doppler ultrasound at 14–16 weeks pregnancy: randomized controlled clinical trial. Croat Med J. 2005; 46:826–31.
73. Goffinet F, Bréart G, Uzan S. ECPPA: randomised trial of low dose aspirin for the prevention of maternal and fetal complications in high risk pregnant women. Br J Obstet Gynaecol. 1996; 103:719–20.


74. Golding J. Jamaica Low Dose Aspirin Study Group. A randomised trial of low dose aspirin for primiparae in pregnancy. Br J Obstet Gynaecol. 1998; 105:293–9.


75. Hermida RC, Ayala DE, Fernández JR, Mojón A, Alonso I, Silva I, et al. Administration time-dependent effects of aspirin in women at differing risk for preeclampsia. Hypertension. 1999; 34:1016–23.


76. Michael CA, Walters BNJ. Low-dose aspirin in the prevention of pre-eclampsia: current evaluation. Maternal Physiology and Pathology. 1992; 183–9.
77. Morris JM, Fay RA, Ellwood DA, Cook CM, Devonald KJ. A randomized controlled trial of aspirin in patients with abnormal uterine artery blood flow. Obstet Gynecol. 1996; 87:74–8.


78. Villa PM, Kajantie E, Räikkönen K, Pesonen AK, Hämäläinen E, Vainio M, et al. Aspirin in the prevention of pre-eclampsia in high-risk women: a randomised placebo-controlled PREDO trial and a meta-analysis of randomised trials. BJOG. 2013; 120:64–74.


79. Zhao YM, Xiao LP, Hu H, Yang XN, Xu YQ, Guo LM. Low-dose aspirin prescribed at bed time for the prevention of pre-eclampsia in high-risk pregnant women. Reprod Contracept. 2012; 32:355–9.
Fig. 1
Cyclooxygenase mechanism of action and aspirin mechanism of action. COX, cyclooxygenase; PGH2, prostaglandin H2; PGl2, prostaglandin I2; PGD2, prostaglandin D2; PGE2, prostaglandin E2; PGF2, prostaglandin.
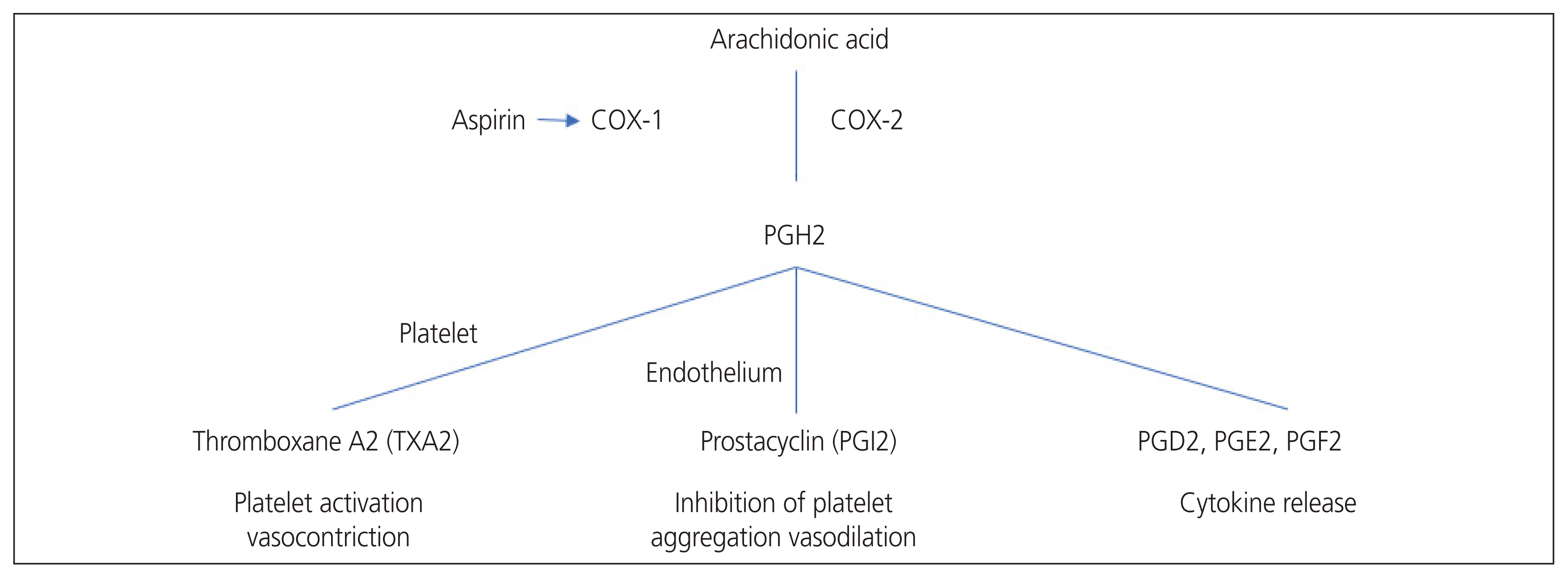
Table 1
Characteristics of randomized trials that assessed the use of low-dose aspirin to prevent preeclampsia in the early stages of pregnancy
Authors, year | N | Inclusion criteria | Aspirin | Control group | Onset (weeks) | Outcomes |
---|---|---|---|---|---|---|
Ayala et al. [42] (2013) | 350 | History, risk factor | 100 mg | Placebo | 13 | PE, preterm delivery, stillbirth, IUGR |
Beaufils et al. [33] (1985) | 102 | History, risk factors | 150 mg | No treatment | 14 | PE, gestational HTN, severe PE, birth weight, IUGR |
CLASP [35] (1994) | 9,309 | History, risk factors | 60 mg | Placebo | 12–32 | PE, IUGR, stillbirth |
Caritis et al. [36] (1998) | 523 | High risk | 60 mg | Placebo | ≤16 | PE, preterm birth, SGA, abruptio placenta |
Ebrashy et al. [72] (2005) | 139 | Abnormal uterine artery Doppler plus history, risk factors | 75 mg | No treatment | 14–16 | Preterm PE, PE, IUGR, preterm delivery |
Goffinet [73] (1996) | 970 | History, risk factors | 60 mg | Placebo | 12–32 | PE, preterm delivery, IUGR, birthweight, stillbirth |
Golding et al. [74] (1998) | 6,049 | Nulliparity | 60 mg | Placebo | 12–32 | Gestational HTN, PE, eclampsia, birthweight, preterm birth |
Hermida et al. [75] (1999) | 350 | History, risk factors | 100 mg | Placebo | 12–16 | IUGR, preterm birth, abruptio placenta |
Michael and Walters [76] (1992) | 110 | Chronic HTN | 100 mg | Placebo | 16 | PE, gestational HTN |
Morris et al. [77] (1996) | 102 | Nulliparous women with abnormal uteroplacental resistance at 18 weeks of gestation | 100 mg | Placebo | 17–19 | Fetal growth restriction, PE, preterm birth, perinatal death |
Rolnik et al. [63] (2017) | 1,620 | A high risk for preterm PE according to the screening algorithm | 150 mg | Placebo | 11–14 | Preterm PE, PE, gestational HTN, SGA, stillbirth, abruption, preterm birth, poor fetal growth |
Villa et al. [78] (2013) | 121 | Abnormal uterine artery Doppler plus history | 100 mg | Placebo | 12–14 | PE, gestational HTN, birthweight, SGA |
Zhao et al. [79] (2012) | 237 | Hypertension, history, risk factors | 75 mg | Placebo | 13–16 | PE, preterm birth, SGA, stillbirth, delivery mode, gestational age at delivery |
Table 2
Maternal risk factors for preeclampsia according to professional organizations
ACOG 2018 (United States of America) [7] | NICE 2019 (United Kingdom) [54] | SOGC 2014 (Canada) [80] | SOMANZ 2014 [81] | WHO 2011 [43] |
---|---|---|---|---|
High-risk factors | High-risk factors | High-risk factors | Risk factors | Risk factors |
Previous pregnancy with PE | Previous pregnancy with PE | Previous pregnancy with PE | Nulliparity | Previous pregnancy with PE |
Chronic hypertension | Chronic hypertension | Antiphospholipid syndrome | Multiple gestation | Diabetes |
Systemic lupus erythematosus | Autoimmune disease | Preexisting diabetes mellitus | Previous pregnancy with PE | Chronic hypertension |
Systemic lupus erythematosus | Type 1 or type 2 diabetes mellitus | Renal disease or proteinuria | Family history of PE | Renal disease |
Renal disease | Chronic kidney disease | Chronic hypertension or booking diastolic BP ≥90 mmHg | Overweight | Autoimmune disease |
Multiple gestation | Antiphospholipid syndrome | Moderate risk factors | Obesity (BMI ≥30 kg/m2) | Multifetal pregnancy |
Antiphospholipid syndrome | Moderate risk factors | Age ≥40 yrs | Age ≥40 yrs | |
Moderate risk factors | Nulliparity | Family history of PE (mother or sister) | Systolic BP >130 mmHg or diastolic BP >80 mmHg | |
Nulliparity | Age ≥40 yrs | Lower maternal birthweight or preterm delivery | Antiphospholipid syndrome | |
Age ≥35 yrs | Interpregnancy interval >10 yrs | Heritable thrombophilia | Preexisting diabetes mellitus | |
Interpregnancy interval >10 yrs | BMI at first visit >35 kg/m2 | Nonsmoking | Underlying renal disease | |
BMI >30 kg/m2 | Family history of PE | Increased prepregnancy triglyceride | Chronic autoimmune disease | |
Family history of PE (mother or sister) | Multiple gestation | Previous miscarriage of <10 weeks with same partner | Interpregnancy interval >10 yrs | |
History of SGA or adverse outcome | Cocaine and methamphetamine use | |||
Sociodemographic characteristics (African American race of low socioeconomic status) | Booking systolic of BP ≥130 mmHg or diastolic BP of ≥90 mmHg | |||
Vaginal bleeding in early pregnancy | ||||
Gestational trophoblastic disease | ||||
Abnormal PAPP-A or free beta-hCG |
ACOG, American College of Obstetricians and Gynecologists; NICE, National Institute for Health and Care Excellence; SOGC, Society of Obstetricians and Gynecologists of Canada; SOMANZ, Society of Obstetric Medicine of Australia and New Zealand; WHO, World Health Organizatio; PE, preeclampsia; BP, blood pressure; BMI, body mass index; SGA, small-for-gestational-age; PAPP-A, pregnancy-associated plasma protein A; hCG, human chorionic gonadotropin.