Abstract
Actin dynamics play an essential role in myogenesis through multiple mechanisms, such as mechanotransduction, cell proliferation, and myogenic differentiation. Twinfilin-1 (TWF1), an actin-depolymerizing protein, is known to be required for the myogenic differentiation of progenitor cells. However, the mechanisms by which they epigenetically regulate TWF1 by microRNAs under muscle wasting conditions related to obesity are almost unknown. Here, we investigated the role of miR-103-3p in TWF1 expression, actin filament modulation, proliferation, and myogenic differentiation of progenitor cells. Palmitic acid, the most abundant saturated fatty acid (SFA) in the diet, reduced TWF1 expression and impeded myogenic differentiation of C2C12 myoblasts, while elevating miR-103-3p levels in myoblasts. Interestingly, miR-103-3p inhibited TWF1 expression by directly targeting its 3’UTR. Furthermore, ectopic expression of miR-103-3p reduced the expression of myogenic factors, i.e., MyoD and MyoG, and subsequently impaired myoblast differentiation. We demonstrated that miR-103-3p induction increased filamentous actin (F-actin) and facilitated the nuclear translocation of Yes-associated protein 1 (YAP1), thereby stimulating cell cycle progression and cell proliferation. Hence, this study suggests that epigenetic suppression of TWF1 by SFA-inducible miR-103-3p impairs myogenesis by enhancing the cell proliferation triggered by F-actin/YAP1.
Skeletal muscle is a highly dynamic tissue that contributes significantly to various body and cellular functions, including force generation, energy reservoirs, and metabolism [1]. Maintaining skeletal muscle requires myogenesis, which is essential for proper development, growth, and regeneration [2]. Skeletal myogenesis is a highly ordered and finely coordinated process that includes progenitor cell proliferation, myogenic factor activation, and differentiation [2]. Hence, dysregulation of skeletal myogenesis inevitably results in muscle wasting, characterized by a decline in muscle mass, strength, quality, and regeneration capacity [3]. During the last decade, several studies have discovered that excess consumption of saturated fatty acids (SFA) causes lipotoxicity, including mitochondrial dysfunction, oxidative stress, and apoptosis, through the ectopic accumulation of fat in the muscle and liver, which eventually leads to muscle wasting [4]. However, the mechanisms responsible for impaired myogenic differentiation and muscle wasting resulting from SFA remain largely unknown.
Diverse myogenic transcriptional programs in progenitor cells require timely control of cytoskeletal dynamics, such as assembly, disassembly, and rearrangement of cytoskeletons [5]. Actin is a pivotal player in the dynamic remodeling of the cytoskeleton, and its ability to polymerize and depolymerize is indispensable for controlling the mechanical support, structural changes, and mobility of cells [5,6]. Recent investigations have revealed that actin-binding proteins can orchestrate mechanotransduction, the conversion of mechanical and physical changes into biochemical signaling, through actin remodeling, thereby eliciting cellular responses related to the cell cycle and proliferation [7,8]. In particular, actin-binding proteins have been highlighted as critical regulators of Yes-associated protein 1 (YAP1) in mechanotransduction via modulation of the dynamics between globular actin (G-actin) and filamentous actin (F-actin) [9-11]. YAP1 is a transcriptional coactivator of the Hippo signaling pathway that mediates various cellular functions, including cell proliferation, differentiation, and migration [12]. Therefore, the accumulation of F-actin has been shown to enhance cell proliferation and inhibit the differentiation of progenitor cells through mechanotransduction-mediated YAP1 activation [13]. Previously, we demonstrated that Twinfilin-1 (TWF1), an actin-binding protein for actin depolymerization, is required for myogenic differentiation through the modulation of F-actin, YAP1 activation, and cell proliferation [14]. Nevertheless, how TWF1 is regulated under muscle-wasting conditions, such as obesity and oxidative stress, remains unexplored.
MicroRNAs (miRNAs) are endogenously expressed small non-coding RNAs that function as epigenetic regulators of gene expression in most biological events [15]. There is growing evidence that miRNAs are critical in cellular processes associated with myogenesis, including cell proliferation, cell cycle coordination, and differentiation [16]. Several studies also have shown that dysregulation of specific miRNAs is associated with the etiology of myopathies [17]. Dysregulated miRNAs by SFA or obesity have been connected to muscle wasting by aggravating oxidative stress, mitochondrial dysfunction, and endoplasmic reticulum stress [18,19]. However, the mechanisms by which SFA-inducible miRNAs inhibit myogenic differentiation are poorly understood. Recently, miR-103-3p, a member of the miR-103/107 family, was reported to be upregulated in many types of malignancies, including liver [20] and colorectal cancers [21]. Conversely, overexpression of miR-103-3p promotes cell proliferation, migration, and invasion [22]. Moreover, miR-103-3p has upregulated in the livers of leptin-deficient (ob/ob) and high-fat diet-fed obese mice [23-25] and in the serum of obese children [26] and patients with non-alcoholic fatty liver disease [27]. In a recent study, miR-103-3p levels in differentiating C2C12 myocytes were significantly lower than in undifferentiated proliferating myoblasts (GSE29286) [28]. Interestingly, in silico analysis of miR-103-3p for target prediction suggested that TWF1 is a possible target. Nonetheless, the role of miR-103-3p in myogenesis, particularly in the regulation of myogenic differentiation and TWF1 expression, has not yet been investigated.
In the present study, we found that SFA inversely modulated the expression of TWF1 and miR-103-3p in myogenic progenitor cells and that TWF1 is a direct target of miR-103-3p. Therefore, we further investigated the role of miR-103-3p in the regulation of myogenic differentiation because TWF1 is an essential factor in differentiation. Finally, we explored the mechanism underlying the action of miR-103-3p on F-actin-mediated YAP1 activation, cell cycle progression, and proliferation in myoblasts. The study highlights the role of miR-103-3p in myogenic differentiation, suggesting a possible mechanism for miRNA-mediated muscle wasting in obesity.
C2C12 myoblast, a mouse skeletal muscle cell line, was obtained from ATCC and cultured in a growth medium (GM; 10% fetal bovine serum in Dulbecco’s Modified Eagle Medium [DMEM] + 100 IU/ml penicillin/streptomycin) (Gibco). All the cells were conditioned in a 5% CO2 humidified atmosphere at 37°C. For the myogenic differentiation, the GM was switched to a differentiation medium (DM; 2% horse serum in DMEM + 100 IU/ml penicillin/streptomycin) (Gibco) after reaching 80%–90% confluence. For palmitic acid (PA) treatment, bovine serum albumin (BSA)-conjugated PA was prepared as previously described [29]. Cells were incubated in the GM in the presence of 100 μM PA or vehicle for 24 h before analyzing or inducing differentiation.
C2C12 cells were seeded in 35 mm dishes at a density of 1.3 × 105 cells/dish for 24 h before transfection. Lipofectamine 2000 (Invitrogen) was used to transfect TWF1 siRNA (siTWF1), miR-103-3p mimic, miR-103-3p inhibitor (antimiR-103), or scrambled control RNA (scRNA) at a final concentration of 200 nM (Genolution), according to the manufacturer’s instructions. Oligonucleotide sequences are listed in Supplementary Table 1.
Total RNA was isolated using QIAzol reagent (Qiagen) and purified using the miRNeasy Mini Kit (Qiagen). The concentration and quality of the RNAs were evaluated using a UV-1700 PharmaSpec spectrophotometer (Shimadzu) and 2% agarose gel electrophoresis. The isolated RNAs were reverse-transcribed into cDNAs using a miScript II RT Kit (Qiagen) with an Applied Biosystems Veriti Thermal Cycler (Thermo Fisher Scientific), according to the manufacturer’s instructions. To measure mRNAs and miRNA expression levels, qRT-PCR was performed using a LightCycler 480 (Roche Applied Science) with iTaq polymerase and SYBR Green I (Promega). The primers and conditions used for the qRT-PCR are presented in Supplementary Table 2. Additionally, U6 was used as an internal reference to determine the relative gene expression using the 2-ΔΔCt method.
The murine TWF1 3’UTR including miR-103-3p target sequence (ATCGGGC) was amplified by RT-PCR using the primers described in Supplementary Table 2 and subcloned into the pmirGLO vector (Promega) to construct the wild-type TWF1 luciferase reporter plasmid (TWF1wt). Mutation of miR-103-3p target sites in the TWF1 3’UTR was accomplished by site-directed mutagenesis following the manufacturer’s instructions (Promega) to construct a mutant type TWF1 1uciferase reporter plasmid (TWF1mut). Next, cells (3 × 104 cells/well) were seeded in 12-well plates to reach a confluency of 30%–40%. The miR-103-3p mimic or scRNA (70 nM) was co-transfected with wild-type (TWF1wt) or mutant (TWF1mut) reporter vectors (0.5 μg) using Lipofectamine 2000 (Invitrogen). After 24 h of transfection, firefly and Renilla luciferase activities were recorded using the Sirius L Single Tube Luminometer system (Berthold Technology) with a Dual-Luciferase Reporter Assay System 100 kit (Promega). The Renilla/firefly ratios were used to determine luciferase activity.
The cytoplasmic and nuclear fractions were prepared using NE-PER Nuclear and Cytoplasmic Extraction Reagents (Thermo Fisher Scientific) following the manufacturer’s instructions. The cytoplasmic and nuclear fractionations were subjected to immunoblotting, as described below.
The C2C12 cells were isolated with lysis buffer consisting of 1% phosphatase inhibitor cocktail II, 2% Trixon-X, and 0.2 mM PMSF (Sigma-Aldrich). All the proteins were quantitated using the Bradford assay with a UV-1700 PharmaSpec spectrophotometer (Shimadzu). The protein samples (20 µg/lane) were separated by electrophoresis and transferred onto nitrocellulose membranes (Amersham). After blocking for 1 h with 5% skimmed milk in TTBS (1% Tween 20 in TBS), the membranes were incubated overnight with specific antibodies (Supplementary Table 3) at 4°C. The next day, the blots were incubated with secondary antibodies (Supplementary Table 3) and developed using chemiluminescent Femto reagent (Thermo Fisher Scientific). Fusion Solo, an analytical scanning system, was used to visualize all blots, and immunoblot intensities were analyzed with Evolution Capt software (Vilber).
The C2C12 cells were transfected with indicated oligonucleotides, allowed to induce differentiation for five days, and the cells were then fixed with 4% paraformaldehyde for 10 min. After that, the cells were permeabilized with Triton X-100 (0.3%, 15 min) and blocked with BSA (3%, 2 h). The fixed cells were incubated overnight with myosin heavy chain (MyHC) antibody (1:100; DSHB) at 4°C. After washing three times with PBS, the cells were incubated with Alexa fluorescent 488 goat-anti mouse antibody (Thermo Fisher Scientific) for 1 h. For the F-actin staining, FITC-coupled phalloidin (P5282; Sigma) was used to stain the cells after fixation and permeabilization, as previously described [11]. The nuclei were counterstained with Hoechst 33342 (Thermo Fisher Scientific) for 15 min. Finally, the cells were imaged using a fluorescence microscope (Leica Microsystems). The differentiation index was defined as the percentage of nuclei expressed in MyHC-positive myotubes relative to the total number of nuclei. The fusion index was defined as the percentage of nuclei in elongated myotube structures (≥ 3 nuclei) relative to the total number of nuclei. ImageJ software (National Institutes of Health) was used to measure the number of myotubes, diameter, and MyHC-positive areas. The experiments were conducted at least three times, and five randomly selected fields were examined in each experiment.
Cell proliferation was measured by EdU assays using the Click-iT EdU Cell Proliferation Kit (Invitrogen), following the manufacturer’s protocol. The C2C12 myoblasts (104 cells/well) were seeded in eight-well chamber slides, transfected with the oligonucleotides, and incubated for 24 h. Next, EdU reagent (10 µM) was added to the cells and grown for 4 h in 37°C incubator. Further, 0.3 mL of Click-iT reaction cocktail was added and incubated for 20 min in the dark after fixation and permeabilization. The nuclei were then counterstained with Hoechst 33342 (Thermo Fisher Scientific) for 15 min and imaged under a Leica microscope. The ratio of EdU-positive cells to the total number of nuclei was calculated using ImageJ software (National Institutes of Health).
The cell cycle assay was conducted using cell cycle kit reagent (C03551; Beckman Coulter). The C2C12 cells were harvested and centrifuged at 3,000 rpm at 4°C for 5 min. The supernatants were discarded and the pellets were fixed with 70% ethanol at 4°C. After one day, the cells were washed three times with PBS buffer, centrifuged, and the cells were resuspended in a cell cycle kit reagent (500 µl) for 20 min. Flow cytometry was performed using the CytoFLEX unit (Beckman Coulter).
The tentative binding site of miRNAs on TWF1 3’UTR was analyzed using publicly available algorithms (Pictar: pictar.mdc-berlin.de, TargetScan: targetscan.org). All data are presented as the means ± standard errors of the mean (SEM) from at least three independent experiments. The statistical significance of differences between means was assessed using the one-way independent Student’s t-test for unpaired data.
It has been reported that TWF1 is crucial at the early stage of myogenic differentiation [14], and miR-103-3p is upregulated in obesity [23-25]. Therefore, we first investigated whether SFA modulates the expression of TWF1 and miR-103-3p in C2C12 myoblasts. According to a previous study [30], to suppress myogenic differentiation, C2C12 cells were treated with 100 µM of PA, the most abundant dietary SFA, for 24 h and allowed to differentiate for five days. As shown by immunocytochemistry (Fig. 1A, B), PA resulted in shorter MyHC-positive myotubes with fewer nuclei than vehicle-treated controls and markedly reduced the MyHC-positive area, differentiation index, and fusion index compared to controls. Moreover, PA significantly reduced the expression of myogenic transcription factors, such as MyoD and MyoG (Fig. 1C), demonstrating that PA suppressed myotube formation and myogenic factors expression. Interestingly, PA markedly reduced the protein levels of TWF1 by approximately 60% (Fig. 1C, D). In contrast, it dramatically increased the expression of miR-103-3p by more than five-fold (Fig. 1E). Thus, PA impaired myogenic differentiation and inversely regulated TWF1 and miR-103-3p expression in C2C12 cells.
To investigate whether miR-103-3p might regulate TWF1 expression, we analyzed the putative target genes of miR-103-3p using the target prediction algorithms of miRNAs, such as PicTar and TargetScan. Notably, miR-103-3p was predicted to target TWF1, whose expression in PA-treated myoblasts was inversely related to miR-103-3p expression. The results of comparative sequence analysis indicated that TWF1 3’UTR is complementary to the miR-103-3p seed sequence, which is evolutionarily conserved among mammals, including humans, mice, and rabbits (Fig. 2A). These data provide a robust rationale for examining whether TWF1 3’UTR has genuine target sites for miR-103-3p. Therefore, we cloned the TWF1 3’UTR (wild-type; TWF1wt) region or a three-base mutation (TWF1mut) at the tentative miR-103-3p seed binding site to a pmirGLO dual-luciferase reporter plasmid (Fig. 2B). The construct was transfected into C2C12 cells along with either the miR-103-3p mimic or scRNA, and luciferase activity was analyzed after 24 h (Fig. 2C). Co-transfection of miR-103 mimic with TWF1wt construct suppressed luciferase activity by approximately 40% when compared to scRNA controls (Fig. 2C). In contrast, mutation of critical three nucleotides in the miR-103-3p seed binding site of the TWF1 3’UTR resulted in the failure of the miR-103 mimic to suppress the luciferase activity of the reporter construct (Fig. 2C). To further confirm that TWF1 is indeed a valid target of miR-103-3p, we transfected C2C12 myoblasts with a miR-103 mimic or scRNA and analyzed TWF1 protein expression. As expected, the miR-103-3p mimic markedly reduced the protein level of TWF1 compared to scRNA (Fig. 2D). In a reciprocal experiment, inhibition of miR-103-3p using 2’-O-methyl antisense inhibitors of miR-103-3p (antimiR-103) led to full recovery of the endogenous TWF1 protein (Fig. 2D). These observations indicate that miR-103-3p functions as a negative regulator of TWF1 expression by directly targeting the TWF1 3’UTR.
As TWF1 is essential for myogenic factors’ expression and myoblast differentiation [14], we hypothesized that induction of miR-103-3p might suppress myogenic factors and inhibit myoblast differentiation by targeting TWF1. The expression of myogenic factors in C2C12 cells was determined on the third day of differentiation after transfection with scRNA, siTWF1, miR-103-3p mimic, or antimiR-103. Under our experimental conditions, the transfection of siTWF1 caused a dramatic reduction in the protein levels of TWF1 and myogenic factors, i.e., MyoD, MyoG, and MyHC, in C2C12 cells (Fig. 3). As expected, transfection of the miR-103-3p mimic resulted in significant decreases in the levels of TWF1 and myogenic factors (Fig. 3). Moreover, co-transfection with antimiR-103 and miR-103-3p mimic almost entirely rescued the suppressive effect of the miR-103-3p mimic on the expressions of TWF1 and myogenic factors (Fig. 3). Because the 3’UTRs of myogenic factors do not exhibit tentative binding sites for the miR-103-3p seed sequence based on bioinformatic analysis, the inhibitory effect of the miR-103-3p mimic on myogenic factors was mainly attributed to the reduction of TWF1.
We next assessed the function of miR-103-3p in myoblast differentiation because miR-103-3p suppressed myogenic factors’ expression. The C2C12 cells were transfected with scRNA, siTWF1, miR-103-3p mimic, or antimiR-103 and differentiated in DM for five days. Myoblast differentiation was evaluated by immunocytochemistry using the MyHC antibody, followed by quantitative image analysis. As shown in Fig. 4, myotube formation was significantly reduced in myoblasts when TWF1 was silenced using siTWF1. Notably, the miR-103-3p mimic clearly decreased the number and size of myotubes (Fig. 4). By calculating the number of nuclei within myotubes, we found that the miR-103-3p mimic exhibited fewer myotubes with fewer nuclei and had a lower differentiation index and fusion index than the scRNA controls. To further confirm the function of miR-103-3p in myogenic differentiation, C2C12 cells were co-transfected with scRNA or antimiR-103. The results showed that antimiR-103 dramatically rescued the suppressive effect of the miR-103-3p mimic on myoblast differentiation and myotube formation (Fig. 4). These results provide evidence that miR-103-3p impairs the differentiation of myoblasts into myotubes.
Our previous studies have shown that TWF1 knockdown in myoblasts promoted cell proliferation by augmenting F-actin and translocating cytoplasmic YAP1 into the nucleus [14]. Therefore, we investigated whether the miR-103-3p mimic resulted in F-actin accumulation and YAP1 activation in C2C12 cells. As shown in Fig. 5A and B, the miR-103-3p mimic or siTWF1 transfection markedly enhanced F-actin levels by approximately 60% compared with scRNA controls. Considering that the total actin amount from each treatment remained equal in immunoblotting, the enhanced F-actin staining seems to be attributed to the impairment of actin depolymerization, resulting from the inhibition of TWF1 by miR-103-3p. Thus, miR-103-3p reduced TWF1 expression, consequently stimulating the formation of F-actin stress fibers in myoblasts. Accumulation of F-actin has been reported to inhibit the phosphorylation of YAP1, leading to its translocation to the nucleus, which activates transcription factors involved in cell proliferation [12,31]. Therefore, we examined whether miR-103-3p could promote the nuclear translocation of YAP1 in myoblasts by evaluating its phosphorylation (Ser127) and nuclear levels. As predicted, myoblasts transfected with the miR-103-3p mimic exhibited a drastic reduction in cytoplasmic phosphorylated YAP1 and increased nuclear YAP1 levels (Fig. 5C, D), suggesting that miR-103-3p could activate YAP1 by facilitating its nuclear translocation from the cytoplasm.
Because YAP1 plays a vital role in controlling cell cycle and proliferation, we determined whether the induction of miR-103-3p could be involved in cell cycle progression and proliferation. According to proliferation analysis using the EdU assay (Fig. 6A, B), siTWF1 transfection significantly increased EdU incorporation into myoblasts about 2.2-fold compared to scRNA controls. As expected, miR-103-3p transfection also substantially elevated EdU incorporation by 1.7-fold. However, co-transfection with antimiR-103 and miR-103-3p mimic completely abolished the effect of miR-103-3p on EdU incorporation, suggesting that miR-103-3p stimulates myoblast proliferation. Next, we analyzed the cell cycle in myoblasts to determine whether transfection with miR-103-3p enhances cell cycle progression. Flow cytometry analysis showed that miR-103-3p increased the proportion of cells in the S and G2/M phases and reduced the proportion of cells in the G0/G1 phase (Fig. 6C, D). Additionally, we further analyzed the expression levels of CCNB1, CCND1, and PCNA, which are known to induce cell proliferation and cell cycle progression [32], using qRT-PCR to confirm the effect of miR-103-3p on cell proliferation. As shown in Fig. 6E, the expression of these genes was dramatically upregulated in cells transfected with miR-103-3p mimic compared to that in the scRNA controls. Collectively, these results suggest that miR-103-3p increases myoblast proliferation by facilitating cell cycle progression.
Despite increasing evidence that miRNAs play a crucial role in skeletal myogenesis and regeneration [17,33], the mechanism by which miRNAs induced by SFA or obesity intervene in myogenic differentiation remains largely unknown. Recent advances in the understanding of cytoskeletal dynamics and mechanotransduction have enabled us to explore the significance of miRNA involved in actin remodeling and skeletal myogenesis. In this study, we demonstrated the role of SFA-inducible miR-103-3p in TWF1 expression and myogenic differentiation. Our findings provide the following key contributions to the current understanding: (i) PA reduces TWF1 expression while elevating miR-103-3p in C2C12 myoblasts; (ii) MiR-103-3p directly targets the 3’UTR of TWF1; and (iii) more importantly, the induction of miR-103-3p impaired myogenic differentiation of progenitor cells by activating YAP1 via F-actin accumulation, subsequently enhancing cell cycle progression and proliferation. Therefore, our study suggests that epigenetic regulation of TWF1 modulated by SFA-inducible miR-103-3p contributes to muscle wasting through the F-actin/YAP1 axis.
SFA has a detrimental effect on the differentiation of various progenitor cells; however, the underlying mechanisms by which SFA inhibits myogenic differentiation are not well understood. The present study is the first to show the suppression of TWF1 expression by PA, suggesting that dysregulation of actin-binding proteins by SFA could lead to impaired myogenesis. TWF1 has recently been discovered to be a highly conserved cytoplasmic actin-binding protein that belongs to the actin-depolymerizing factor homology family [34]. TWF1 plays a crucial role in actin cytoskeletal dynamics by capping the barbed ends on F-actin and sequestering G-actin monomer [35]. Therefore, the knockdown of TWF1 increases F-actin and cytoskeletal alteration [36], while overexpression of TWF1 diminishes F-actin by enhancing filament depolymerization [37]. Corresponding to a previous study [14], we demonstrated that the depletion of TWF1 using siTWF1 in myogenic progenitor cells promoted cell proliferation by facilitating the nuclear translocation of YAP1. Moreover, TWF1 silencing inhibited the expression of myogenic transcription factors and the differentiation of myoblasts. Given that PA suppressed TWF1 expression and provoked impaired myogenic differentiation, the reduction in TWF1 is considered a contributing factor in impaired myogenic differentiation by SFA, implying that dysregulation of TWF1 could cause sarcopenic obesity by linking fat accumulation to muscle wasting.
What is the mechanism by which TWF1 expression is suppressed in myoblasts in response to SFA? The present study focused on the epigenetic regulation of TWF1 expression, as several studies have indicated that miR-103-3p is upregulated in obesity and metabolic diseases [23-27], and bioinformatic analysis suggested that the 3’UTR of TWF1 is a tentative target of miR-103-3p (Fig. 2). Previous studies have shown an association between the upregulation of miR-103-3p and obesity [23-26]. In contrast, overexpression of miR-103-3p enhances the expression of adipogenic genes, which stimulates triglyceride accumulation in preadipocytes [38]. Our current study showed that PA alone could enhance miR-103-3p expression in myoblasts, although further studies are necessary to determine how SFA induces miR-103-3p expression. Above all, miR-103-3p suppressed TWF1 protein expression by directly targeting the TWF1 3’UTR. This is the first report demonstrating miRNA-mediated epigenetic regulation of the actin-binding protein TWF1. To date, only a few studies have implicated miRNAs in cytoskeletal dynamics and myogenic differentiation. In this aspect, the role of miR-103-3p in TWF1 expression suggests that the molecular mechanism of myogenic differentiation is regulated by miRNA that targets the molecules involved in actin dynamics.
During myogenesis, myoblast proliferation and differentiation into myotubes are inversely related; thus, cell cycle exit is a prerequisite for the onset of myoblast differentiation [2]. Impaired myogenic differentiation by SFA-inducible miR-103-3p was ascribed to the enhanced proliferation of progenitor cells. How miR-103-3p promotes cell cycle progression and proliferation in myoblasts? As a mechanotransduction mechanism, the actin cytoskeleton plays a critical role in regulating YAP1 in the Hippo pathway [39]. Phosphorylation of YAP1 by Hippo kinase increases the proteasomal degradation of YAP1 in the cytoplasm and reduces its nuclear translocation [40]. Hence, F-actin accumulation is known to inhibit Hippo kinase, thereby leading to the nuclear translocation of YAP1, which promotes cell proliferation and accelerates the cell cycle [31]. Accordingly, TWF1 siRNA markedly facilitated F-actin formation and nuclear YAP1 localization, thereby significantly promoting cell proliferation and cell cycle progression [11]. In this perspective, the most important finding of this study was that miR-103-3p increased F-actin by suppressing TWF1 expression (Fig. 5) and consequently facilitated the nuclear translocation of YAP1, resulting in myoblast proliferation through the transcriptional activation of proliferation-related genes (Fig. 6).
The present study is the first to show the suppression of TWF1 expression by SFA, implying that this could be a contributing factor to sarcopenic obesity by linking muscle wasting and fat accumulation. Furthermore, by demonstrating the role of miR-103-3p in TWF1 expression and myogenic differentiation, we revealed how SFA regulates TWF1 expression, mechanotransduction, cell proliferation, and myogenic differentiation. In conclusion, SFA-inducible miR-103-3p leads to the epigenetic suppression of TWF1 and inhibits myogenic differentiation by enhancing cell proliferation triggered by F-actin/YAP1. Therefore, our findings suggest that the underlying mechanisms of actin remodeling and skeletal muscle homeostasis might be epigenetically regulated in the context of obesity. Despite the many merits of this study, further improvements are still necessary to achieve even better results. Several other targets regulated by miR-103-3p should be investigated to better understand myogenesis. Furthermore, it is important to examine the potential diagnostic and therapeutic implications of miR-103-3p in animal models of muscle wasting.
Notes
SUPPLEMENTARY MATERIALS
Supplementary data including three tables can be found with this article online at https://doi.org/10.4196/kjpp.2023.27.3.277.
REFERENCES
1. Mukund K, Subramaniam S. 2020; Skeletal muscle: a review of molecular structure and function, in health and disease. Wiley Interdiscip Rev Syst Biol Med. 12:e1462. DOI: 10.1002/wsbm.1462. PMID: 31407867. PMCID: PMC6916202.


2. Chal J, Pourquié O. 2017; Making muscle: skeletal myogenesis in vivo and in vitro. Development. 144:2104–2122. DOI: 10.1242/dev.151035. PMID: 28634270.
3. Sartori R, Romanello V, Sandri M. 2021; Mechanisms of muscle atrophy and hypertrophy: implications in health and disease. Nat Commun. 12:330. DOI: 10.1038/s41467-020-20123-1. PMID: 33436614. PMCID: PMC7803748. PMID: 53db8cc5160a46c3bf9a31a615d5e847.


4. Akhmedov D, Berdeaux R. 2013; The effects of obesity on skeletal muscle regeneration. Front Physiol. 4:371. DOI: 10.3389/fphys.2013.00371. PMID: 24381559. PMCID: PMC3865699. PMID: bd56e511b6364b308390add5d21b4737.


5. Guerin CM, Kramer SG. 2009; Cytoskeletal remodeling during myotube assembly and guidance: coordinating the actin and microtubule networks. Commun Integr Biol. 2:452–457. DOI: 10.4161/cib.2.5.9158. PMID: 19907716. PMCID: PMC2775249.
6. Heng YW, Koh CG. 2010; Actin cytoskeleton dynamics and the cell division cycle. Int J Biochem Cell Biol. 42:1622–1633. DOI: 10.1016/j.biocel.2010.04.007. PMID: 20412868.


7. Watt KI, Goodman CA, Hornberger TA, Gregorevic P. 2018; The Hippo signaling pathway in the regulation of skeletal muscle mass and function. Exerc Sport Sci Rev. 46:92–96. DOI: 10.1249/JES.0000000000000142. PMID: 29346163. PMCID: PMC6319272.


8. Fischer M, Rikeit P, Knaus P, Coirault C. 2016; YAP-mediated mechanotransduction in skeletal muscle. Front Physiol. 7:41. DOI: 10.3389/fphys.2016.00041. PMID: 26909043. PMCID: PMC4754448. PMID: 5a6c62c8b18b4f119e329aff69bc2a6e.


9. Nguyen NU, Liang VR, Wang HV. 2014; Actin-associated protein palladin is required for migration behavior and differentiation potential of C2C12 myoblast cells. Biochem Biophys Res Commun. 452:728–733. DOI: 10.1016/j.bbrc.2014.08.143. PMID: 25194811.


10. Li H, Hou L, Zhang Y, Jiang F, Zhu Y, Li QX, Hu CY, Wang C. 2019; PFN2a suppresses C2C12 myogenic development by inhibiting proliferation and promoting apoptosis via the p53 pathway. Cells. 8:959. DOI: 10.3390/cells8090959. PMID: 31450751. PMCID: PMC6770762. PMID: 7a4c017f5f3f4526961f7fd9f6be2d19.


11. Nguyen MT, Min KH, Kim D, Park SY, Lee W. 2020; CFL2 is an essential mediator for myogenic differentiation in C2C12 myoblasts. Biochem Biophys Res Commun. 533:710–716. DOI: 10.1016/j.bbrc.2020.11.016. PMID: 33187645.


12. Dupont S, Morsut L, Aragona M, Enzo E, Giulitti S, Cordenonsi M, Zanconato F, Le Digabel J, Forcato M, Bicciato S, Elvassore N, Piccolo S. 2011; Role of YAP/TAZ in mechanotransduction. Nature. 474:179–183. DOI: 10.1038/nature10137. PMID: 21654799.


13. Aragona M, Panciera T, Manfrin A, Giulitti S, Michielin F, Elvassore N, Dupont S, Piccolo S. 2013; A mechanical checkpoint controls multicellular growth through YAP/TAZ regulation by actin-processing factors. Cell. 154:1047–1059. DOI: 10.1016/j.cell.2013.07.042. PMID: 23954413.


14. Nguyen MT, Won YH, Kwon TW, Lee W. 2022; Twinfilin-1 is an essential regulator of myogenic differentiation through the modulation of YAP in C2C12 myoblasts. Biochem Biophys Res Commun. 599:17–23. DOI: 10.1016/j.bbrc.2022.02.021. PMID: 35168059.


15. Saliminejad K, Khorram Khorshid HR, Soleymani Fard S, Ghaffari SH. 2019; An overview of microRNAs: biology, functions, therapeutics, and analysis methods. J Cell Physiol. 234:5451–5465. DOI: 10.1002/jcp.27486. PMID: 30471116.


16. Dowling L, Duseja A, Vilaca T, Walsh JS, Goljanek-Whysall K. 2022; MicroRNAs in obesity, sarcopenia, and commonalities for sarcopenic obesity: a systematic review. J Cachexia Sarcopenia Muscle. 13:68–85. DOI: 10.1002/jcsm.12878. PMID: 34984856. PMCID: PMC8818592. PMID: 39e3a1acc69341c09daa9bbe73df46d5.


17. Zhao Y, Chen M, Lian D, Li Y, Li Y, Wang J, Deng S, Yu K, Lian Z. 2019; Non-coding RNA regulates the myogenesis of skeletal muscle satellite cells, injury repair and diseases. Cells. 8:988. DOI: 10.3390/cells8090988. PMID: 31461973. PMCID: PMC6769629. PMID: c274d319e33148a19d33df3e4507d3d0.


18. Sannicandro AJ, Soriano-Arroquia A, Goljanek-Whysall K. 2019; Micro(RNA)-managing muscle wasting. J Appl Physiol (1985). 127:619–632. Erratum in: J Appl Physiol (1985). 2019;127:1502. DOI: 10.1152/japplphysiol.00961.2018. PMID: 30991011.


19. Ji C, Guo X. 2019; The clinical potential of circulating microRNAs in obesity. Nat Rev Endocrinol. 15:731–743. DOI: 10.1038/s41574-019-0260-0. PMID: 31611648.


20. Xia W, Ni J, Zhuang J, Qian L, Wang P, Wang J. 2016; MiR-103 regulates hepatocellular carcinoma growth by targeting AKAP12. Int J Biochem Cell Biol. 71:1–11. DOI: 10.1016/j.biocel.2015.11.017. PMID: 26646106.


21. 2017; Correction: miR-103/107 promote metastasis of colorectal cancer by targeting the metastasis suppressors DAPK and KLF4. Cancer Res. 77:6788. Erratum for: Cancer Res. 2012;72:3631-3641. DOI: 10.1158/0008-5472.CAN-12-0667. PMID: 22593189.
22. Li K, Yuan C. 2020; MicroRNA-103 modulates tumor progression by targeting KLF7 in non-small cell lung cancer. Int J Mol Med. 46:1013–1028. DOI: 10.3892/ijmm.2020.4649. PMID: 32582959. PMCID: PMC7387085.


23. Trajkovski M, Hausser J, Soutschek J, Bhat B, Akin A, Zavolan M, Heim MH, Stoffel M. 2011; MicroRNAs 103 and 107 regulate insulin sensitivity. Nature. 474:649–653. DOI: 10.1038/nature10112. PMID: 21654750.


24. Näär AM. 2011; MiRs with a sweet tooth. Cell Metab. 14:149–150. DOI: 10.1016/j.cmet.2011.07.005. PMID: 21803284. PMCID: PMC3880780.


25. Rottiers V, Näär AM. 2012; MicroRNAs in metabolism and metabolic disorders. Nat Rev Mol Cell Biol. 13:239–250. Erratum in: Nat Rev Mol Cell Biol. 2012;13:1. DOI: 10.1038/nrm3313. PMID: 22436747. PMCID: PMC4021399.


26. Cui X, You L, Zhu L, Wang X, Zhou Y, Li Y, Wen J, Xia Y, Wang X, Ji C, Guo X. 2018; Change in circulating microRNA profile of obese children indicates future risk of adult diabetes. Metabolism. 78:95–105. DOI: 10.1016/j.metabol.2017.09.006. PMID: 28966078.


27. Xu Q, Li Y, Shang YF, Wang HL, Yao MX. 2015; miRNA-103: molecular link between insulin resistance and nonalcoholic fatty liver disease. World J Gastroenterol. 21:511–516. DOI: 10.3748/wjg.v21.i2.511. PMID: 25593466. PMCID: PMC4292282.


28. Yamamoto H, Morino K, Nishio Y, Ugi S, Yoshizaki T, Kashiwagi A, Maegawa H. 2012; MicroRNA-494 regulates mitochondrial biogenesis in skeletal muscle through mitochondrial transcription factor A and Forkhead box j3. Am J Physiol Endocrinol Metab. 303:E1419–E1427. DOI: 10.1152/ajpendo.00097.2012. PMID: 23047984.


29. Pike LS, Smift AL, Croteau NJ, Ferrick DA, Wu M. 2011; Inhibition of fatty acid oxidation by etomoxir impairs NADPH production and increases reactive oxygen species resulting in ATP depletion and cell death in human glioblastoma cells. Biochim Biophys Acta. 1807:726–734. DOI: 10.1016/j.bbabio.2010.10.022. PMID: 21692241.


30. Nguyen MT, Min KH, Lee W. 2020; MiR-96-5p induced by palmitic acid suppresses the myogenic differentiation of C2C12 myoblasts by targeting FHL1. Int J Mol Sci. 21:9445. DOI: 10.3390/ijms21249445. PMID: 33322515. PMCID: PMC7764195. PMID: 3d4f21be3da14dffb849999903560083.


31. Dupont S. 2016; Role of YAP/TAZ in cell-matrix adhesion-mediated signalling and mechanotransduction. Exp Cell Res. 343:42–53. DOI: 10.1016/j.yexcr.2015.10.034. PMID: 26524510.


32. Whitfield ML, George LK, Grant GD, Perou CM. 2006; Common markers of proliferation. Nat Rev Cancer. 6:99–106. DOI: 10.1038/nrc1802. PMID: 16491069.


33. Mok GF, Lozano-Velasco E, Münsterberg A. 2017; microRNAs in skeletal muscle development. Semin Cell Dev Biol. 72:67–76. DOI: 10.1016/j.semcdb.2017.10.032. PMID: 29102719.


34. Poukkula M, Kremneva E, Serlachius M, Lappalainen P. 2011; Actin-depolymerizing factor homology domain: a conserved fold performing diverse roles in cytoskeletal dynamics. Cytoskeleton (Hoboken). 68:471–490. DOI: 10.1002/cm.20530. PMID: 21850706.


35. Palmgren S, Vartiainen M, Lappalainen P. 2002; Twinfilin, a molecular mailman for actin monomers. J Cell Sci. 115(Pt 5):881–886. DOI: 10.1242/jcs.115.5.881. PMID: 11870207.


36. Johnston AB, Collins A, Goode BL. 2015; High-speed depolymerization at actin filament ends jointly catalysed by Twinfilin and Srv2/CAP. Nat Cell Biol. 17:1504–1511. DOI: 10.1038/ncb3252. PMID: 26458246. PMCID: PMC4808055.


37. Hakala M, Wioland H, Tolonen M, Kotila T, Jegou A, Romet-Lemonne G, Lappalainen P. 2021; Twinfilin uncaps filament barbed ends to promote turnover of lamellipodial actin networks. Nat Cell Biol. 23:147–159. Erratum in: Nat Cell Biol. 2021;23:437-438. DOI: 10.1038/s41556-020-00629-y. PMID: 33558729.


38. Xie H, Lim B, Lodish HF. 2009; MicroRNAs induced during adipogenesis that accelerate fat cell development are downregulated in obesity. Diabetes. 58:1050–1057. DOI: 10.2337/db08-1299. PMID: 19188425. PMCID: PMC2671055.


39. Mendez MG, Janmey PA. 2012; Transcription factor regulation by mechanical stress. Int J Biochem Cell Biol. 44:728–732. DOI: 10.1016/j.biocel.2012.02.003. PMID: 22387568. PMCID: PMC3445012.


40. Pan D. 2010; The hippo signaling pathway in development and cancer. Dev Cell. 19:491–505. DOI: 10.1016/j.devcel.2010.09.011. PMID: 20951342. PMCID: PMC3124840.


Fig. 1
Palmitic acid (PA) inhibits myoblast differentiation while upregulating miR-103-3p expression.
C2C12 cells were pretreated with PA (100 µM) for 24 h before differentiation. (A) The cells were stained with an anti-MyHC antibody (green) and Hoechst 33342 nuclear dye (blue). Scale bar: 50 μm. (B) MyHC-positive areas, differentiation indices, and fusion indices were analyzed as described in the Materials and Methods. (C, D) Representative immunoblots of myogenic factors and TWF1 on day three of differentiation and analyzed densitometry. The immunoblot intensities were normalized to the amount of β-Actin. The values are shown as relative ratios, with the intensity of the normalized vehicle control set to one. (E) The expressions of miR-103-3p (miR-103) were determined after 24 h of PA treatment by qRT-PCR and normalized to the amount of U6. All data are shown as the mean ± SEM (n > 3), where the level of significance is represented as *p < 0.05; ***p < 0.001 vs. controls. MyHC, myosin heavy chain; TWF1, Twinfilin-1; qRT-PCR, real-time qualitative polymerase chain reaction.
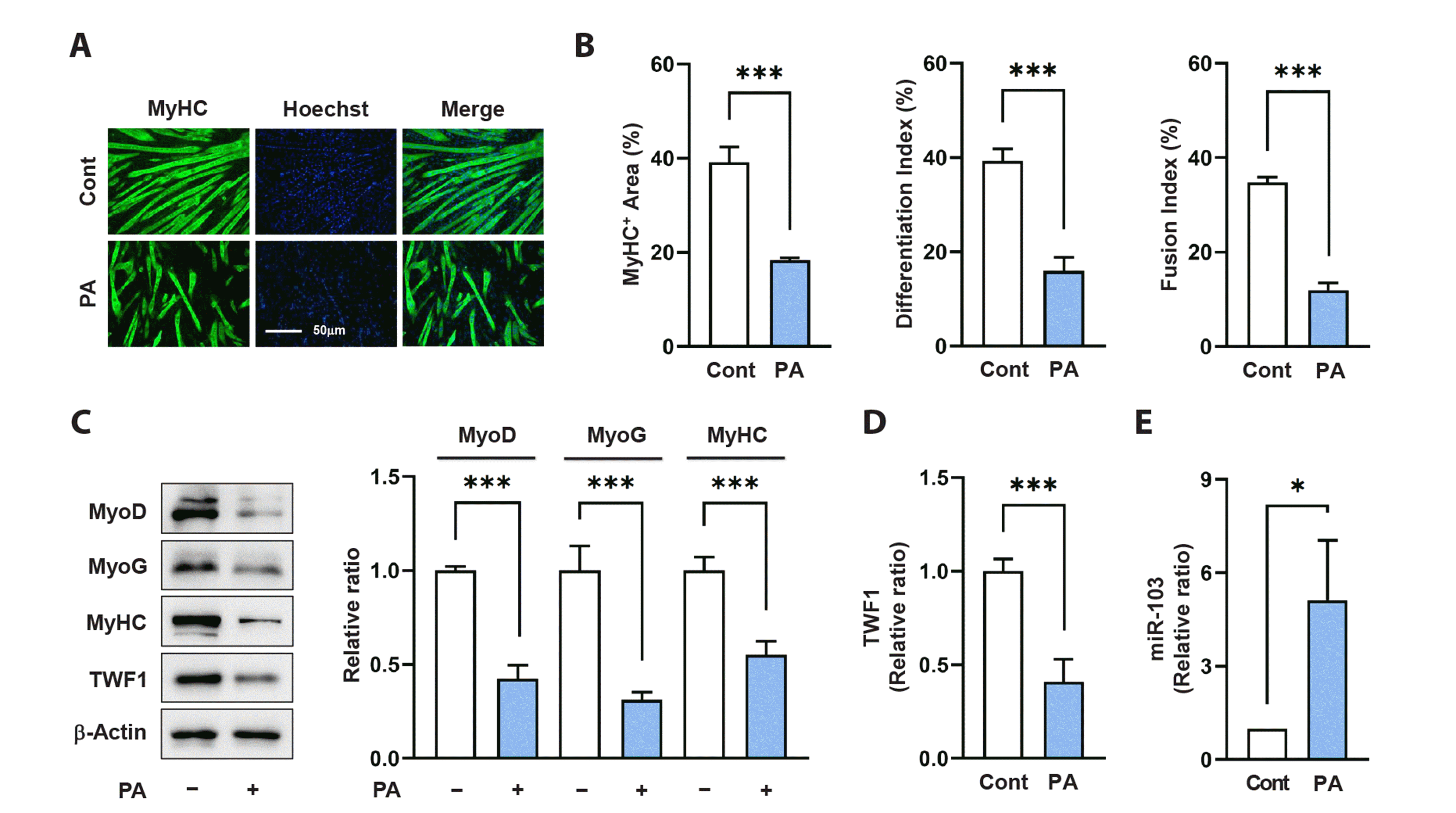
Fig. 2
MiR-103-3p suppresses TWF1 expression by targeting
TWF1 3’UTR. (A) The tentative miR-103-3p (miR-103) target site of the TWF1 3’UTR. (B) Schematic diagram of wild-type TWF1 3’UTR segment (TWF1wt) and mutant TWF1 3’UTR segment (TWF1mut) containing miR-103 target sites cloned in pmirGLO dual-luciferase reporter plasmids and the seed sequence of miR-103 target sites on the TWF1wt. (C) C2C12 cells were co-transfected with scRNA control or miR-103-3p mimic (miR-103) and either TWF1wt or TWF1mut. Luciferase activities were determined 24 h after transfection. (D) The protein levels of TWF1 in C2C12 cells were detected by immunoblotting after 24 h of transfection with scRNA control, miR-103-3p mimic (miR-103), or antimiR-103. The immunoblot intensities were normalized to the amount of β-Actin. The values are shown as relative ratios, with the intensity of the normalized scRNA control set to one. All data are shown as the mean ± SEM (n > 3), where the level of significance is represented as **p < 0.01; ***p < 0.001 vs. scRNA. TWF1, Twinfilin-1; CDS, coding sequence; ns, no significance.
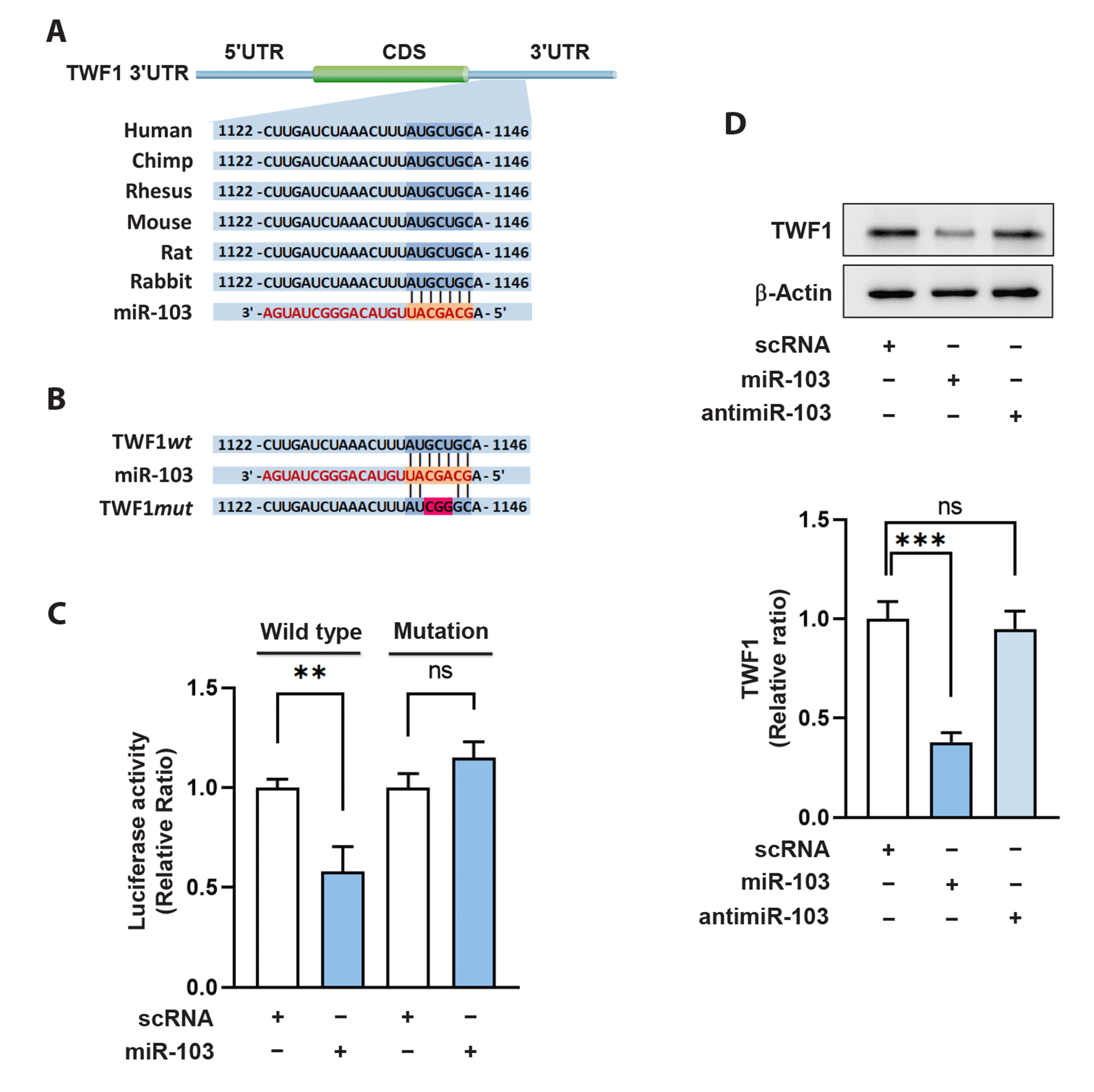
Fig. 3
MiR-103-3p inhibits the expressions of myogenic factors.
C2C12 cells were transfected with scRNA, siTWF1, miR-103-3p mimic (miR-103), or antimiR-103 and differentiated for three days. (A) Representative immunoblots from three independent experiments on day three of differentiation. (B) The immunoblot intensities were normalized to the amount of β-Actin. The values are shown as relative ratios, with the intensity of the normalized scRNA control set to one. All data are shown as the mean ± SEM (n > 3), where the level of significance is represented as **p < 0.01; ***p < 0.001 vs. scRNA. TWF1, Twinfilin-1; ns, no significance.
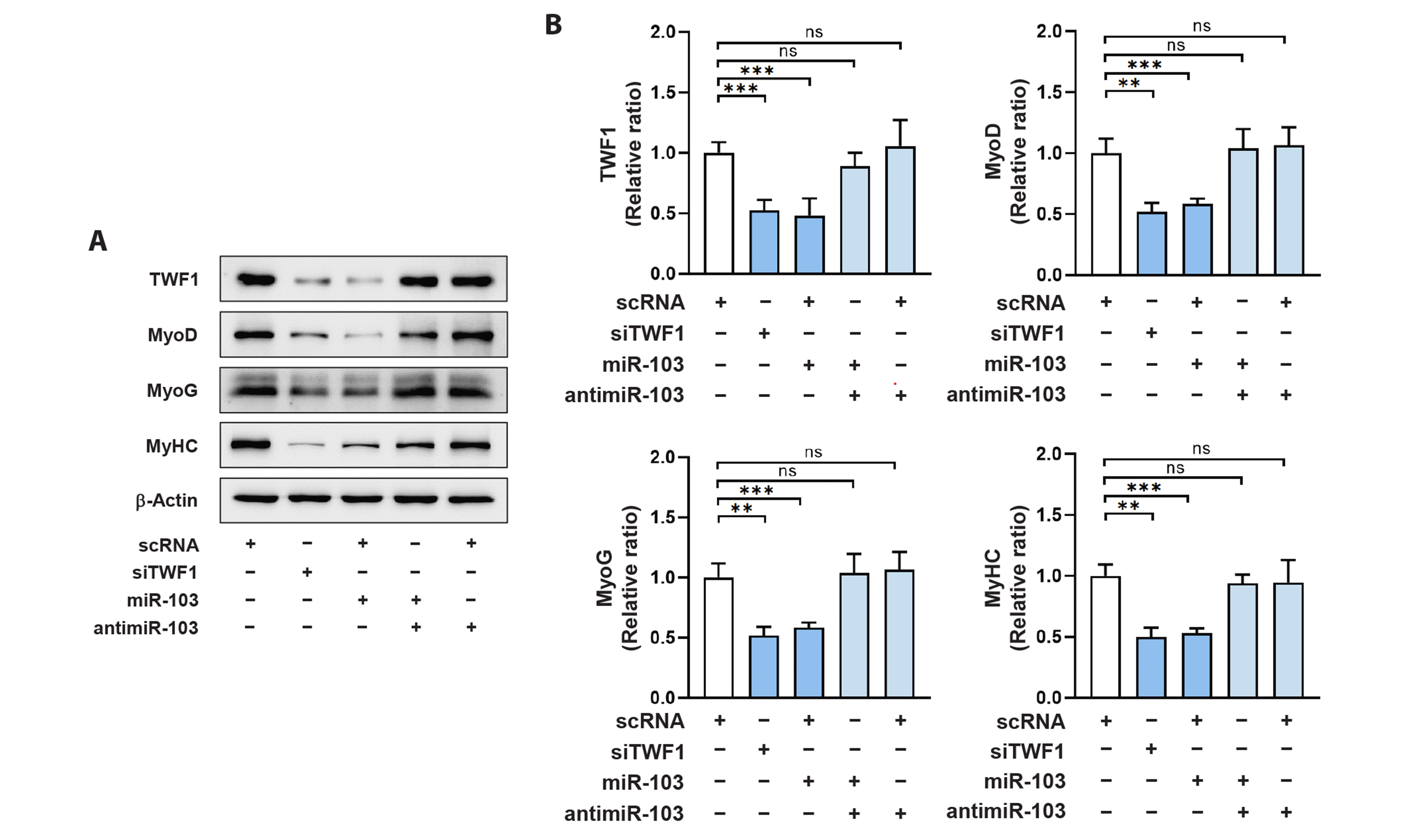
Fig. 4
MiR-103-3p impairs myogenic differentiation and myotube formation.
C2C12 cells were transfected with scRNA, siTWF1, miR-103-3p mimic (miR-103), or antimiR-103 and differentiated for five days. (A) The cells were stained with an anti-MyHC antibody (green) and Hoechst 33342 nuclear dye (blue). Scale bar: 50 μm. (B) MyHC-positive areas, differentiation indices, fusion indices, and myotube widths were analyzed as described in the Materials and Methods. All data are shown as the mean ± SEM (n > 3), where the level of significance is represented as ***p < 0.001 vs. scRNA. MyHC, myosin heavy chain; TWF1, Twinfilin-1; ns, no significance.

Fig. 5
MiR-103-3p facilitates F-actin accumulation and nuclear YAP1 localization.
C2C12 cells were transfected with scRNA, siTWF1, or miR-103-3p mimic (miR-103) and cultured for 24 h. (A) The transfected cells were labeled with FITC-phalloidin (green) for F-actin staining and Hoechst 33342 (blue) for nucleus staining. Representative images from three independent experiments are shown. Scale bar: 25 μm. (B) Phalloidin intensities were analyzed by the ImageJ program and presented as relative ratios, with the intensity of the scRNA control set to one. (C) Representative immunoblots of YAP1 and phosphorylated YAP1 (pYAP1) protein in the cytoplasmic and nuclear fractions are shown. α-Tubulin and Lamin B were used as the cytoplasm and nucleus markers, respectively. β-Actin was used for loading control. (D) The immunoblot intensities were normalized to the amount of β-Actin or α-Tubulin, and the values are shown as relative ratios, with the intensity of the normalized scRNA control set to one. All data are shown as the mean ± SEM (n > 3), where the level of significance is represented as **p < 0.01; ***p < 0.001 vs. scRNA. F-actin, filamentous actin; YAP1, Yes-associated protein 1; TWF1, Twinfilin-1; ns, no significance.
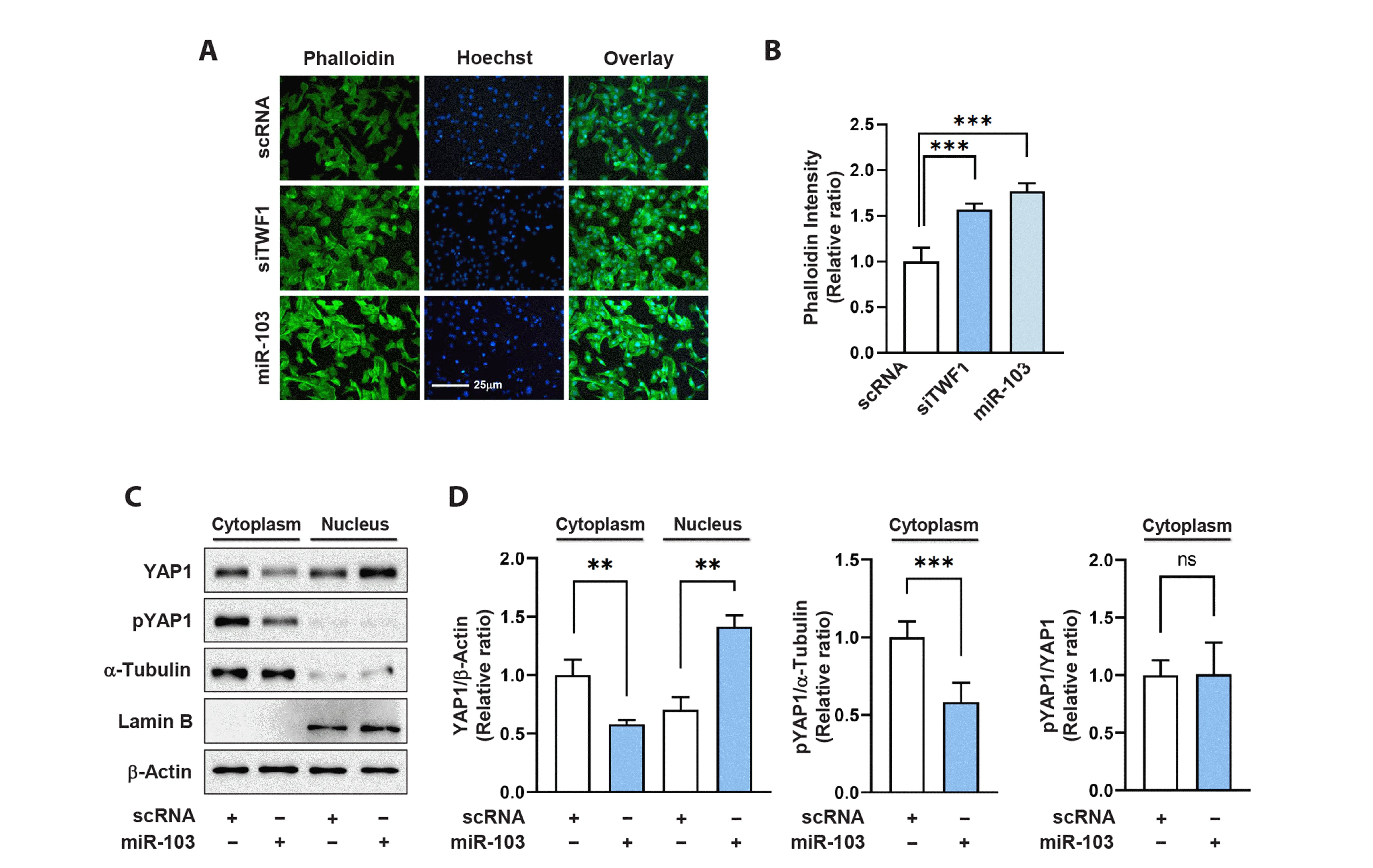
Fig. 6
MiR-103-3p enhances myoblasts proliferation.
C2C12 cells were transfected with scRNA, siTWF1, miR-103-3p mimic (miR-103), or antimiR-103 and cultured for 24 h. (A) The cells undergoing DNA replication were labeled with EdU (green), and Hoechst 33342 (blue) was used for nuclei staining. Scale bar: 50 μm. (B) The proportion of EdU-positive cells was measured using ImageJ software. (C, D) Flow cytometry with a scatter plot of cell cycle analysis after transfection with scRNA or miR-103-3p mimic (miR-103). (E) Relative expression levels of PCNA, CCNB1, and CCND1 were analyzed by qRT-PCR and normalized to U6 levels. The values are shown as relative ratios, with the intensity of the scRNA control set to one. All data are shown as the mean ± SEM (n > 3), where the level of significance is represented as *p < 0.05; **p < 0.01; ***p < 0.001 vs. scRNA. TWF1, Twinfilin-1; EdU, ethynyl deoxyuridine; qRT-PCR, real-time qualitative polymerase chain reaction; ns, no significance.
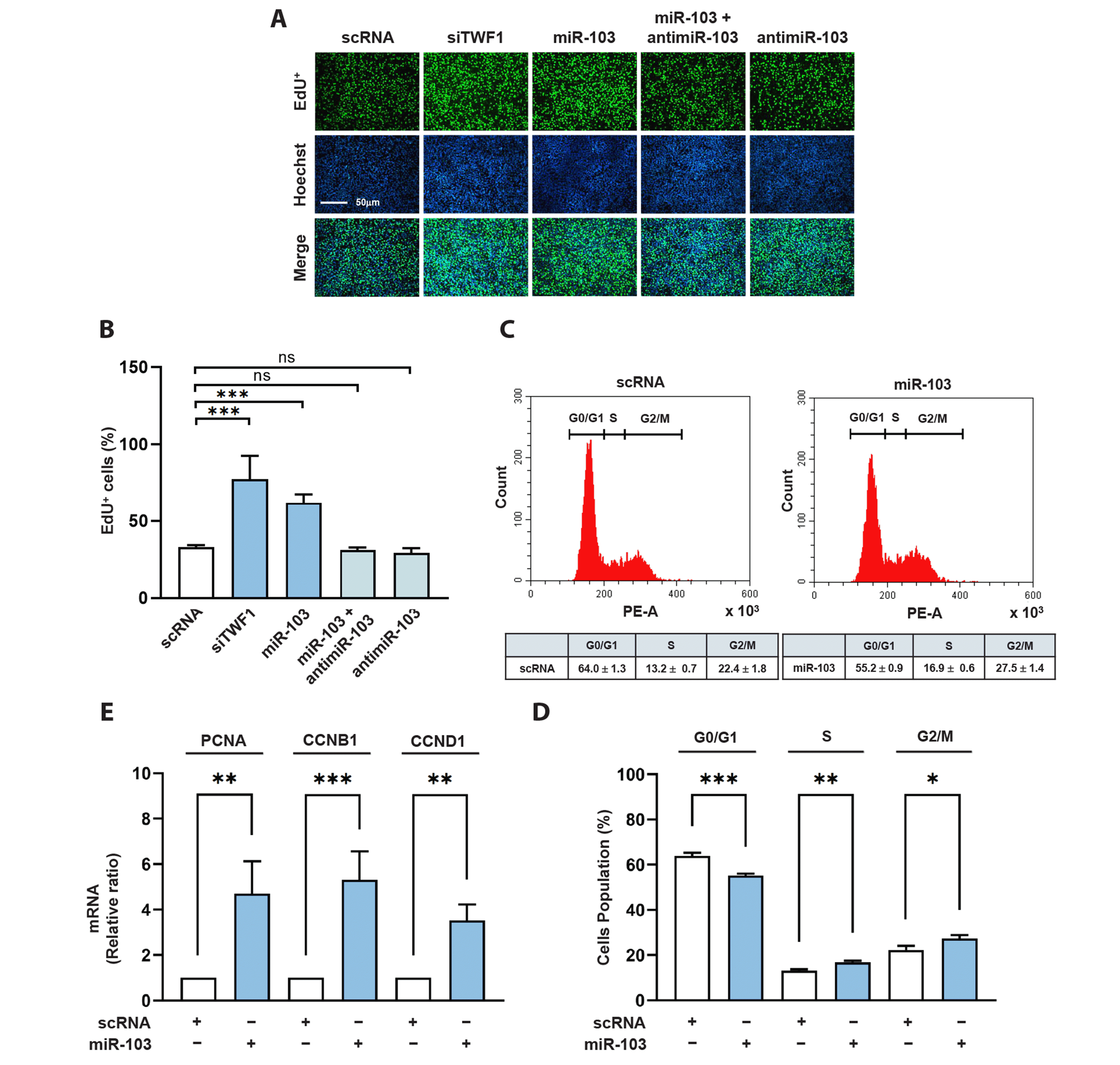