Abstract
Background
Type 2 diabetic mellitus (T2DM) is an emerging global pandemic which is associated with lots of co-morbidities and reported vascular dysfunctions. T2DM associated vascular dysfunctions leads to vasculopathy in the form of altered peripheral vascular dynamics. Cold stress test (CST) is a reliable sympathetic reactivity test used for assessing vascular dysfunctions. In this study we are trying to quantify vascular dysfunctions in T2DM patients non invasively by various parameters of photoplethysmography (PPG) of cold stress test.
Methods
Case control study had done in referral health center AIIMS, Raipur. Parameters are recorded by finger-PPG before, during and after CST (1 min) in 2 groups, control (n = 20 healthy volunteers) and case (n = 20 diagnosed T2DM patients).
Results
Due to cold stress, PPG parameter peak amplitude was significantly decreased in both healthy and T2DM groups (p <0.001 and p <0.001, respectively). However, recovery trend of amplitude was significantly slow in T2DM compared to healthy subjects. Another PPG parameter peak to peak interval was significantly higher in healthy group compared to T2DM patients.
Diabetes mellitus is a known lifestyle disease that is rapidly emerging as a global pandemic associated with high rates of comorbidity. One of the main problems that receives the least attention in patients with type 2 diabetic mellitus (T2DM) is cardiovascular autonomic neuropathy, which is characterized by complications such as peripheral neuropathy and vasculopathy.1 Vascular derangement is often overlooked in patients with diabetes since the features of subtle vascular autonomic impairment might not be clinically evident until it adversely affects the quality of life of a patient. The occurrence of peripheral artery disease is tenfold higher in patients with T2DM.2 Atherosclerosis in peripheral vasculature leads to vasculopathy in terms of dysfunctional vascular dynamics.3 Increased arterial stiffness in patients with diabetes may be an important pathway linking diabetic vasculopathy and the associated increased cardiovascular morbidity and mortality. The risks of macro- and microvascular complications increase with diabetes duration, which explains why vasculopathy measurements help to predict cardiovascular disease development.4
Reactivity of the cardiovascular system in the cold stress test (CST) has the potential to noninvasively assess cardiovascular disease. CST has been routinely used as a part of Ewing’s battery of tests for assessing autonomic functions. Sympathetic reactivity is confirmed by increased diastolic blood pressure (BP),5 as acute sympathetic stimulation leads to constriction of the peripheral vasculature.6 Photoplethysmography (PPG), which is an optical and noninvasive measuring technique for observing pulse-volume changes in the microvascular bed of peripheral tissue, is based on quantifying the sympathetic response.7 Pulse volume parameters such as pulse transit time (PTT), which refers to the arterial pulse wave transmitted from the heart to the peripheral vessels,8 can be used to measure vascular compliance.9 The pulse wave velocity in the peripheral vasculature increases due to the vasoconstriction response to the CST, which explains why the PTT reaction might be useful in assessing vasculopathy10 and also in predicting cardiovascular disease development.4
This study evaluated the difference in vascular reactivity to cold stress between healthy subjects and patients with T2DM. Objective measures of pulse-volume parameters such as pulse timings, amplitude, upstroke phase, and PTT were used to quantify the responses.
The aim of this study was to determine the effect of T2DM on cardiovascular functions. To accomplish this aim, the specific objectives were as follows: (1) to quantify the sympathetic vascular response to cold stress using various PPG measures in both study groups. (2) To measure the pulse timings, amplitude, upstroke phase, and PTT in both groups. (3) To compare the sympathetic vascular reactivity parameters between the two groups.
This was a case-control study. Study subjects were recruited after obtaining ethical clearance from the institute (reference no: 61/IEC-AIIMSRPR/2016). The total sample size was 40, comprising 20 healthy volunteers (age: 18-60 years) as the control group and 20 patients with T2DM (age: 18-60 years) as the patient (case) group. Informed written consent was obtained from all of the study participants. Inclusion criteria for the recruitment of participants were diagnosed cases of patients with T2DM (presenting for less than 6 months) and healthy control subjects who were asymptomatic for cardiovascular and respiratory diseases. The exclusion criteria were a history of addiction such as in smoking and alcohol drinking, history of any chronic illness, hypertension, abnormal lipid profile, or other neurological disease condition, epilepsy, or taking medication.
Subjects were familiarized with the experimental design and CST procedure on the day of the experiment. Subjects were instructed to refrain from caffeine intake for at least 12 hours before the procedure, and to eat a light meal no less than 2 hours beforehand. Experiments were conducted in a quiet room at an ambient temperature of 25-27°C with minimal external disturbances. Subjects rested in a supine position for 15 minutes, during which parameters such as BP, heart rate, and respiratory rate and PPG data were recorded. Heart rate was recorded using a lead II electrocardiogram (ECG) instrument and PPG was performed with the aid of an eight-channel digital physiograph machine (LabChart, AD Instruments, Bella Vista, Australia). Subjects were exposed to CSTs in a sitting position. Baseline PPG parameters were recorded in the sitting position before the CST was conducted. Cold water at 10°C was prepared in a container (the water temperature was maintained at 10°C in the autonomic function laboratory). The baseline BP and ECG were recorded before the hand was immersed in cold water. Subjects were asked to immerse the hand in water up to the wrist for 1 minute.11 BP was measured just before the hand was removed from the cold water (i.e., 1 minute after immersion). The hand was covered by a towel after being removed from the water. BP was measured again at 1.5 minutes and 4 minutes after the hand had been withdrawn from the cold water. PPG was performed on the right middle finger in a supine resting position for 5 minutes as a baseline measurement, and the data recording was uninterrupted throughout the CST in the sitting position. The recovery trend of cold stress (i.e., 5-min after the hand was withdrawn from cold water) was also investigated.
ECG and PPG data were analyzed simultaneously to obtain their beat-to-beat changes. These recordings included heart-rate variability (calculated over each minute until recovery time), peak systolic amplitude (height between peak point and foot), systolic peak to peak interval (PPI) (time difference between successive peaks), and crest time (CT); time taken to reach the peak point of the same pulse from the foot. The beat-to-beat changes in PTT were also calculated as the time delay between the R-wave peak on the ECG and the onset of the corresponding PPG pulse (Fig. 1). Resting steady recordings were evaluated for 5 minutes as baseline data.
All data were checked for conforming to a Gaussian distribution and reported as mean ± standard-deviation values. The data distribution was checked using the omnibus normality test from D’Agostino and Pearson, which revealed a normal distribution (p >0.05). Repeated-measures analysis of variance was used for both groups to compare significant trends of the PPG measurements during and after the CST (1-5 minutes) with respect to baseline. The independent t-test were used to compare the baseline and responses in PPG measurements during the CST between the groups. The significance criterion was set at p <0.05. All statistical analyses were performed using SPSS Software version 21.0 (IBM Corporation, Armonk, NY, USA).12
After screening of 30 patients with T2DM, 20 were finally included after confirming their adherence to the exclusion criteria and consent, while 20 healthy age- and sex-matched subjects were also recruited. PPG and ECG signals recorded during the experimental protocol are shown in Fig. 1.
Table 1 lists the demographic profile and baseline cardiovascular parameters of the patients with T2DM and healthy subjects. The patients with T2DM and healthy subjects had comparable age, weight, and sex, while the systolic BP and heart rate were significantly higher in the patients with T2DM. Table 2 lists the baseline PPG parameters of the patients with T2DM and healthy subjects, for which there were no significant differences.
Table 3 compares the responses of the PPG parameters in the patients with T2DM between baseline and during the CST. There was a significant decrease in peak amplitude immediately after immersing the hand in cold water, and it remained low throughout the CST (0.06 ± 0.03 vs. 0.03 ± 0.01 au, p = 0.001). PTT was significantly increased from baseline to during the CST (0.39 ± 0.05 vs. 0.42 ± 0.07 ms, p = 0.001); the upstroke phase changed significantly during and after 1 minute of recovery. Other PPG parameters such as CT and PPI did not differ significantly between baseline and during the CST.
Table 4 compares the responses of the PPG parameters in the healthy subjects between baseline and during the CST. There was a significant decrease in peak amplitude immediately after immersing the hand in cold water (0.04 ± 0.02 vs. 0.03 ± 0.02 au, p = 0.001), and also in upstroke phase (0.85 ± 0.20 vs. 0.67 ± 0.13 aus−1, p = 0.001). There were significant increases in CT (176.55 ± 43.31 vs. 275.93 ± 187.17 ms, p = 0.029), PPI (0.80 ± 0.10 vs. 0.98 ± 0.38 ms, p = 0.034), and PTT (0.40 ± 0.03 vs. 0.43 ± 0.04 ms, p = 0.001) between baseline and during the CST.
The intergroup comparison between patients with T2DM and healthy subjects revealed a slow recovery trend toward baseline after the CST in patients with T2DM. The peak amplitudes of the patients increased significantly (p = 0.04) during the recovery period as compared with the healthy subjects. There was also a significant increase (p = 0.02) in the PPI recovery trend toward baseline after the CST in healthy subjects as compared with patients with T2DM.
The baseline BP and heart rate were significantly higher in the patients in our study than in the healthy subjects, suggestive of an increased autonomic sympathetic drive. Long-standing diabetes leads to micro- and macrolevel dysfunction in multiple organ systems. A severely compromised vascular autonomic nervous system may present with decreased BP response in the CST. As disease duration increases with uncontrolled factors such as glycated hemoglobin (HbA1c) and lipid profile, a diminished response of sympathetic reactivity may occur.1
BP and heart rate were also quantified during and after the CST, and were found to be significantly higher in the patients than in the healthy subjects. This was due to the increased direct drive of the heart by the sympathetic system, which is evidence of sympathetic nervous system activation.13
Simultaneous recordings of pulse volume indicated a significant decrease in peak amplitude and the PPG signal slope due to cold-stress-mediated sympathetic activation and the vasoconstriction response in both study groups. The decrease in both parameters was greater in the patients, reflecting their increased sympathetic nervous system activity. A significant decrease in the amplitude and slope of PPG signals during the CST was attributed to the vasoconstriction response produced by sympathetic stimulation.14 Our results indicated a slower recovery trend toward the baseline in patients with T2DM than in healthy subjects, which was manifested as an increased vascular constriction response to the sympathetic stimulation that was observed in the significant increase in baseline heart rate. This suggests that patients with T2DM have increased sympathetic tone in their blood vessels. The PPI recovery trend indicated significant changes in healthy subjects that suggested normal autonomic control as compared with the patients with T2DM. This may have been due to endothelial damage caused by a higher HbA1c level, which is found in patients with T2DM, resulting in stiffer arteries as compared with healthy subjects.
Baseline PTT did not differ significantly between the patient with T2DM and the healthy subjects. However, the pulse-wave velocity is significantly higher in patients with diabetes than in healthy subjects.15
The main limitation of our study was that only a small number of patients with T2DM was included. However, our exclusion criteria were very stringent, and the patients recruited were from a general heterogeneous group without any past history of autonomic deficits or cardiovascular disorders.
Measuring changes in pulse-volume parameters during the CST has been previously attempted in healthy subjects but not in patients with T2DM. Our study performed a detailed pulse-wave analysis and assessed differences in changes in PPG measurements between patients with T2DM and healthy subjects. The study results indicated reduced vascular reactivity in patients with T2DM, which was independently shown by the responses of PPG amplitude and PPI.
Our preliminary study suggests that the combined responses of pulse-volume parameters such as amplitude and PPI can be used to objectively quantify early vasculopathy in patients with T2DM through sympathetic reactivity to cold stress. Early diagnosis of vascular dysfunction can decrease the end organ damage and can reduce complications associated with diabetes.
ACKNOWLEDGMENTS
We thank the Department of Medicine, AIIMS Raipur, for referring patients for inclusion in our study.
REFERENCES
1. Khandelwal E, Jaryal AK, Deepak KK. Pattern and prevalence of cardiovascular autonomic neuropathy in diabetics visiting a tertiary care referral center in India. Indian J Physiol Pharmacol. 2011; 55:119–127.
2. Ding H, Triggle CR. Endothelial cell dysfunction and the vascular complications associated with type 2 diabetes: assessing the health of the endothelium. Vasc Health Risk Manag. 2005; 1:55–71.


3. Cooper ME, Bonnet F, Oldfield M, Jandeleit-Dahm K. Mechanisms of diabetic vasculopathy: an overview. Am J Hypertens. 2001; 14:475–486.


4. Stehouwer CD, Henry RM, Ferreira I. Arterial stiffness in diabetes and the metabolic syndrome: a pathway to cardiovascular disease. Diabetologia. 2008; 51:527–539.


5. Ewing DJ, Clarke BF. Diagnosis and management of diabetic autonomic neuropathy. Br Med J (Clin Res Ed). 1982; 285:916–918.


6. Yamamoto K, Iwase S, Mano T. Responses of muscle sympathetic nerve activity and cardiac output to the cold pressor test. Jpn J Physiol. 1992; 42:239–252.


7. Allen J. Photoplethysmography and its application in clinical physiological measurement. Physiol Meas. 2007; 28:R1–R39.


8. Naschitz JE, Bezobchuk S, Mussafia-Priselac R, Sundick S, Dreyfuss D, Khorshidi I, et al. Pulse transit time by R-wave-gated infrared photoplethysmography: review of the literature and personal experience. J Clin Monit Comput. 2004; 18:333–342.


9. Foo JY, Lim CS. Pulse transit time as an indirect marker for variations in cardiovascular related reactivity. Technol Health Care. 2006; 14:97–108.


10. Geleris P, Stavrati A, Boudoulas H. Effect of cold, isometric exercise, and combination of both on aortic pulse in healthy subjects. Am J Cardiol. 2004; 93:265–257.


11. Pistorius MA, Briant C, Planchon B. Determination of reference temperatures for a cold test in digital plethysmography. J Mal Vasc. 1994; 19:195–198.
12. IBM C. IBM SPSS statistics for Windows [Internet]. Armonk (NY), USA: IBM Corp;c2012. [accessed 2022 Jul 18]. Available from: https://www.ibm.com/products/spss-statistics.
13. Moriyama K, Ifuku H. Assessment of cardiac contractility during a cold pressor test by using (dP/dt)/P of carotid artery pulses. Eur J Appl Physiol. 2007; 100:185–191.


Fig. 1.
Schematic representation of various PPG measures derived from a digital physiograph after simultaneously recording ECG and PPG signals. PPI, peak to peak interval; PTT, pulse transit time; PPG, photoplethysmography; ECG, electrocardiography.
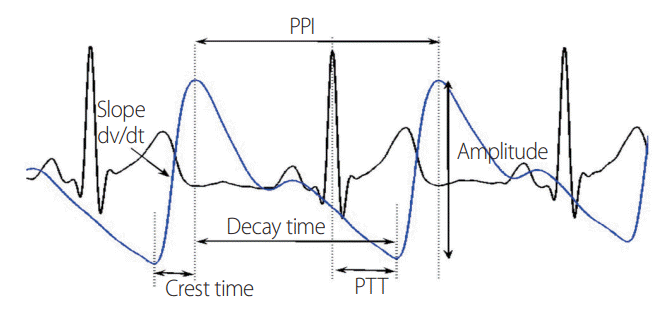
Table 1.
Demographic profile and baseline cardio vascular parameters in T2DM patient and healthy group
Parameter | T2DM patients (n = 20) | Healthy subjects (n = 20) | p |
---|---|---|---|
Age (years) | 45.04 ± 5.10 | 43 ± 3.94 | 0.17 |
Weight (kg) | 69.45 ± 12.99 | 61.67 ± 10.87 | 0.06 |
Height (cm) | 167.16 ± 7.77 | 163.93 ± 4.05 | 0.11 |
Systolic blood pressure (mmHg) | 133.09 ± 13.65 | 122.8 ± 12.95 | 0.019a |
Diastolic blood pressure (mmHg) | 89.28 ± 8.04 | 88.50 ± 9.11 | 0.77 |
Heart rate (per minute) | 82.32 ± 11.24 | 70.60 ± 9.32 | 0.009a |
HbA1c | 8.03 ± 1.88 | 5.32 ± 0.40 | 0.001a |
FBS | 124.82 ± 25.48 | 79.77 ± 7.04 | 0.001a |
Table 2.
Baseline PPG parameters in T2DM patient and healthy group
Table 3.
Comparison of baseline and CST response of PPG parameters in T2DM patient group
Parameters | Baseline | During | 1 minute | 3 minutes | 5 minutes |
---|---|---|---|---|---|
Crest time (ms) | 174.95 ± 36.42 | 210.83 ± 108.86 | 206.54 ± 48.66 | 177.53 ± 34.96 | 181.66 ± 42.55 |
p-value | 0.137 | 0.002a | 0.110 | 0.184 | |
Peak amplitude (au) | 0.06 ± 0.03 | 0.03 ± 0.01 | 0.05 ± 0.03 | 0.06 ± 0.03 | 0.06 ± 0.03 |
p-value | 0.001a | 0.001a | 0.876 | 0.966 | |
PPI (ms) | 0.79 ± 0.09 | 0.82 ± 0.18 | 0.85 ± 0.11 | 0.80 ± 0.11 | 0.80 ± 0.11 |
p-value | 0.396 | 0.002a | 0.226 | 0.190 | |
Upstroke-slope (aus-1) | 0.99 ± 0.39 | 0.70 ± 0.12 | 0.84 ± 0.26 | 0.97 ± 0.39 | 0.97 ± 0.38 |
p-value | 0.002a | 0.003a | 0.392 | 0.527 | |
PTT (ms) | 0.39 ± 0.05 | 0.42 ± 0.07 | 0.42 ± 0.06 | 0.40 ± 0.05 | 0.39 ± 0.05 |
p-value | 0.001a | 0.001a | 0.017a | 0.045a |
Table 4.
Comparison of baseline and CST response of PPG parameters in healthy subject group
Parameters | Baseline | During | 1 minute | 3 minutes | 5 minutes |
---|---|---|---|---|---|
Crest time (ms) | 176.55 ± 43.31 | 275.93±187.17 | 240.80 ± 99.77 | 178.22 ± 49.64 | 177.85 ± 43.69 |
p-value | 0.029a | 0.008a | 0.812 | 0.824 | |
Peak amplitude (au) | 0.04 ± 0.02 | 0.03 ± 0.02 | 0.04 ± 0.01 | 0.04 ± 0.02 | 0.04 ± 0.02 |
p-value | 0.001a | 0.265 | 0.539 | 0.542 | |
PPI (ms) | 0.80 ± 0.10 | 0.98 ± 0.38 | 0.91 ± 0.18 | 0.82 ± 0.09 | 0.82 ± 0.09 |
p-value | 0.034a | 0.003a | 0.155 | 0.144 | |
Upstroke-slope (aus-1) | 0.85 ± 0.20 | 0.67 ± 0.13 | 0.75 ± 0.15 | 0.82 ± 0.18 | 0.83 ± 0.20 |
p-value | 0.001a | 0.003a | 0.122 | 0.337 | |
PTT (ms) | 0.40 ± 0.03 | 0.43 ± 0.04 | 0.44 ± 0.04 | 0.40 ± 0.04 | 0.39 ± 0.03 |
p-value | 0.001a | 0.001a | 0.269 | 0.079 |