Abstract
Objective
This study aimed to compare the mechanical and thermal properties in the anterior and posterior segments of new and retrieved specimens of a commercially available multizone superelastic nickel-titanium (NiTi) archwire.
Methods
The following groups of 0.016 × 0.022-inch Bioforce NiTi archwires were compared a) anterior and b) posterior segments of new specimens and c) anterior and d) posterior segments of retrieved specimens. Six specimens were evaluated in each group, by three-point bending and bend and free recovery tests. Bending moduli (Eb) were calculated. Furthermore, the new specimens were evaluated with scanning electron microscopy/energy-dispersive X-ray spectrometry. A multiple linear regression model with a random intercept at the wire level was applied for data analysis.
Results
The forces in the posterior segments or new specimens were higher than those recorded in the anterior segments or retrieved specimens, respectively. Accordingly, Eb also varied. Higher austenite start and austenite finish (Af) temperatures were recorded in the anterior segments. No statistically significant differences were found for these temperatures between retrieved and new wires. The mean elemental composition was (weight percentage) Ni, 52.6 ± 0.5; Ti, 47.4 ± 0.5.
Conclusions
The existence of multiple force zones was confirmed in new and retrieved Bioforce archwires. The retrieved archwires demonstrated lower forces during the initial stages of deactivation in three-point bending tests, compared with new specimens. The Af temperature of these archwires may lie higher than the regular intraoral temperature. Even at 2 mm deflections, the forces recorded from these archwires may lie beyond biologically safe limits.
Nickel-titanium (NiTi) archwires are available in thermally activated form. Temperature variations induce the transformation between the austenite and martensite phases, although an intermediate rhombohedral phase (R-phase) may also occur. The shape memory effect results from the transition between these two crystallographic phases.1-4 Transition from one phase to another occurs within the “transition temperature range” (TTR).5-7 To obtain full advantage of the superelastic properties, TTR of shape memory alloys should be kept below body temperature, taking into account the temperature fluctuations in the oral cavity.8-11 These NiTi wires may produce lighter forces compared with non-thermally activated ones.12-15 The control of force magnitude is even more important for the anterior teeth, since the incisors have an increased risk of root resorption due to orthodontic forces.16
Hence, in the 1980s, many manufacturers developed multiforce NiTi archwires, i.e., archwires that exert forces of lower magnitude on the anterior teeth and higher magnitude on the posterior teeth. A precursor was composed of mechanically connected parts with different stiffnesses (DaulflexTM; Lancer Orthodontics, Carlsbad, CA, USA).17,18 Further research has demonstrated that selective wire heating by electric current may induce different superelastic properties. Therefore, direct electric resistance heat treatment was used by several manufacturers to develop multiforce NiTi archwires. With the introduction of new technologies, several multiforce or multizone NiTi archwires are currently commercially available.19 These archwires exert forces of lower magnitude in the anterior segment compared with the posterior segment.20 Moreover, they exert lower forces than the superelastic and heat-activated conventional NiTi archwires with the same cross section.20
The thermal properties of the heat-activated archwires may be explored using the following methods: 1) differential scanning calorimetry (DSC), 2) bend and free recovery tests (BFR), and 3) magnetization measurements.21-23 Furthermore, ASTM F2082 was developed to standardize active austenite finish (Af) temperature measurement techniques throughout the Nitinol industry.21
This study put forward the null hypothesis that the mechanical properties in bending and the thermal properties of anterior and posterior wire sections of a common multizone superelastic NiTi archwire are the same in as-received specimens and specimens retrieved after intraoral aging. This study aimed to compare the mechanical properties in bending and the transformation temperatures of a common multizone superelastic NiTi archwire between a) new and retrieved specimens and b) their anterior and posterior segments.
The following groups of 0.016 × 0.022-inch Bioforce (GAC, Dentsply Sirona, Charlotte, NC, USA) NiTi archwires were compared: a) anterior and b) posterior sections of new specimens and c) anterior and d) posterior sections of retrieved specimens (LOT K420, K1X9). Six archwire pieces, sectioned from different archwires, were measured in each configuration. The retrieved specimens were removed after 6.25 ± 0.87 (average ± standard deviation) months of intraoral service. Patients who met the following criteria were included in the study: (a) aged 11–20 years, (b) good overall and oral health (Modified Silness and Löe plaque index PI-M ≤ 3)24 and c) non-extraction orthodontic treatment needed with fixed appliances for both dental arches for at least 6 months after the insertion of the archwires. Patients with higher values of PI-M index or reduced periodontium were excluded. The sample comprised an equal number of male and female patients. The study was approved by the Ethics Committee of the School of Dentistry, National and Kapodistian University of Athens, 426/3.4.2020.
Three-point bending tests were conducted in the Orthodontic Measurement and Simulation System (OMSS). This device comprises two motor-driven positioning tables and two force/moment sensors capable of three-dimensionally registering forces and moments. The sensors are connected to a personal computer with a custom-developed control program to record the movements and simultaneously measure the multidimensional force/deflection (N/mm) or moment/degree curves (Nmm/degree). The measuring ranges amount to ± 15.00 N for forces and ± 450 Nmm for moments. The OMSS is installed in a temperature-controlled chamber during the measurement cycles for a constant temperature of 37 ± 1°C (VEM 03/400; Vötsch Heraeus, Hanau, Germany).25
The anterior (midline region) and the posterior (molar region) segments of the wires were cut off in 20 mm pieces and prepared for the three-point bending test. According to DIN EN ISO 15841,26 the distance between the support points was 10 mm.26 The wire pieces were deflected to a maximum of 3.3 mm by a thrust die, adjusted to the center of the support points (Figure 1). Care was taken to avoid slipping/flipping. This cycle was run twice with each of the 24 wire pieces and the mean value for each specimen was considered for statistical analysis. Wires were oriented edgewise (the smaller dimension was the height of the wire in the direction of displacement). The resulting force/deflection diagram was recorded by the OMSS. The forces on the unloading part of the force/deflection curve were registered and analyzed at deflections of 3.1 mm, 3.0 mm, 2.0 mm, and 1.0 mm.
Bending moduli (Eb) were calculated from the linear part of the three-point bending force-deflection curves with the formula:
where, ΔF and Δd are the differences in force and deflection, respectively, between the unloading points; L is the support span (10 mm); w is the width (in mm); and h is the archwire height (in mm). Deflection loads were obtained from the data at the linear part of the unloading curve between 0.1–0.5 mm.
The critical transformation temperature points of the austenite start (As) and Af were determined with BFR tests. The specimens were cooled in a custom-made, double-walled glass container “to its nominally fully martensitic phase”, deformed and placed on two support pins, and heated back (F12-ED; Julabo, Seelbach, Germany) to their fully austenitic phase (Figure 2).27 The bath of the cold mix (Centro Kühlerschutz, Centro, Karlsruhe, Germany, > 50% ethanediol) was cooled down to a minimum of –20°C and the wire piece remained in the bath for at least 3 minutes prior to testing. Subsequently, the measurement process was started by initializing the heating of the thermostat. During the gradual temperature increase, specimen displacement was monitored by a laser triangulation sensor (RF603; Riftek Sensors & Instruments, Minsk, Belarus) and plotted versus its temperature. Accordingly, the As and Af temperatures were determined from the temperature−displacement graph. The data acquired during heating and recovery (displacement and temperature) were saved as text files and imported into a Microsoft® Excel (Microsoft, Redmond, WA, USA) spreadsheet for plotting. A temperature/displacement graph was created for each wire piece using the spreadsheet tools to draw tangent lines to the linear portions of the curve.
The surface morphology and elemental composition of new specimens were studied with a scanning electron microscope (SEM, Quanta 200; FEI, Hillsboro, OR, USA) and by EDS microanalysis employing a spectrometer (XFlash®6–10 Silicon Drift Detector and a slew window; Bruker, Berlin, Germany) under high vacuum, 20 kV accelerating voltage, 108 μA beam current, area scan mode (250 μm × 220 μm), 200 seconds acquisition time, and 1% dead time. Three measurements were taken and the results were averaged. Quantitative analysis of collected spectra was carried out by the dedicated software (ESPRIT ver.1.9; Bruker) employing atomic number, absorbance, and fluorescence correction factors.
The sample size was calculated based on a recent in vitro experiment, which measured the differences in the force magnitude between the posterior and the anterior segments of similar wires.19 The results showed that a sample of four pairs of measurements would be required for the test to detect the resulting difference with a power of 80%. However, the present study measured a total number of 12 retrieved and new wires. This sample size was additionally assessed using the software GPower 3.1 for Windows (https://www.psychologie.hhu.de/arbeitsgruppen/allgemeine-psychologie-und-arbeitspsychologie/gpower), which determined a power of over 95%.
Statistical analysis was performed using STATA 13.1 (StataCorp LLC, College Station, TX, USA). To investigate possible differences between the measurements by the type of wire (new or retrieved) and segment (posterior or anterior), a multiple linear regression model with a random intercept at the wire level was applied, using the type of wire and location of measurements as covariates. Interactions between the two covariates were also tested; the model with the interactions was selected when the interaction term was statistically significant. The level of statistical significance was set to 0.05.
Forces recorded from the posterior segment were on an average larger than from the anterior segment, regardless of activation; the forces were larger by 0.73 N (95% confidence interval [CI]: 0.67, 0.79) at 1 mm, 0.80 N (95% CI: 0.77, 0.84) at 2 mm, and 1.78 N (95% CI: 1.69, 1.86) at 3 mm, on new wires and by 1.19 N (95% CI: 0.99, 1.40) on retrieved wires; at 3.1 mm the forces were larger by 1.87 N (95% CI: 1.78, 1.96) larger on new wires and 1.32 N (95% CI: 1.11, 1.53) on retrieved wires (Figure 3, Tables 1 and 2).
No statistically significant differences were found between new and retrieved specimens for the unloading points of 1 mm and 2 mm. Contrarily, at 3 mm and 3.1 mm, statistically significant differences were demonstrated between retrieved and new wires (Tables 1 and 3). These differences depend on the location of measurement according to results from models with interaction. At 3 mm, the measurement on the anterior segment of the retrieved wires was on an average 0.18 N less than on the new wires and this difference ranges between 0.07–0.29 N (95% CI); on the posterior segment, the measurement of the retrieved wires was on an average 0.76 N less than on the new wires (95% CI: 0.53, 0.99). Results of similar magnitude and direction were obtained at 3.1 mm (Tables 1 and 3).
The Eb of the retrieved wires were lower by 2.67 (95% CI: 1.22, 4.11) compared with the Eb of new wires in their anterior segment, and by 0.67 (95% CI: 0.94, 3.83) in the posterior segment (Tables 1 and 3). The Eb of the posterior segments was on an average 2.17 (95% CI: 0.95, 3.38) larger than on the anterior segments in new wires, while the respective difference on the retrieved wires was 4.17 (95% CI: 1.23, 3.66) new wires (Tables 1 and 2).
The transformation temperature As obtained from the anterior segments was on average 3.86°C (95% CI: 2.88, 4.83) higher than from the posterior segments. Af in the anterior segments was on average 6.83°C (95% CI: 5.92, 7.73) higher than the posterior Af (Table 4).
No statistically significant difference was found in As and Af temperatures between retrieved and new wires, adjusting for the location of the measurements. Figure 4 depicts representative temperature−displacement graphs of anterior and posterior wire segments.
Backscattered electron image from the anterior segment of a new specimen without mean atomic number, implied a homogeneous structure (Figure 5). In the EDS spectrum, only Ni and Ti were detected (Figure 6). The mean elemental composition was determined as follows (percent weight): Ni = 52.6 ± 0.5 and Ti = 47.4 ± 0.5, which is a typical NiTi formulation.
Based on the results, the null hypothesis was rejected. Differences in specific mechanical and thermal properties were demonstrated between the anterior and posterior segments as well as between as-received and retrieved specimens of Bioforce NiTi orthodontic archwires.
The load-deflection properties of the orthodontic archwires are important parameters that determine the biological nature of tooth movement. The classic three-point bending test is a suitable method for determining these properties28 and is used for distinguishing superelastic wires.29 In all subgroups of wires, higher force magnitudes and Eb were observed in the posterior segments compared with the anterior counterparts (150–250% higher, depending on the deactivation point). A recent study demonstrated the existence of multiple force and torque zones in multizone NiTi wires.19 Similarly, another study reported that Bioforce archwires exert lower forces for a given amount of deflection in the anterior segment when compared to conventional NiTi archwires. These differences may be attributed to differences in wire composition or cold-working process during manufacturing.30
There is still no consensus in orthodontic literature on the optimal force to be applied during orthodontic tooth movement. A recent systematic review concluded that a force between 50 cN and 100 cN seems optimal.31 Different forces have been suggested to bring about the different types of orthodontic movements, such as 0.10–0.20 N for tooth intrusion and 0.35–0.60 N for tooth inclination, extrusion, or rotation.32 However, the recorded force magnitudes in the present experimental setup were higher, even at 2 mm deflections, and may lie beyond biologically safe limits. This finding was expected, since a rectangular NiTi archwire exerts high force magnitudes even with small deflections.33
The present configuration of the three-point bending test is similar to a previous investigation facilitating direct comparison of results.19 They reported similar force levels for other multiforce NiTi products of the same cross section and lower levels for multistrand products without force zones. Interestingly, a 0.017 × 0.025 nine-strand NiTi archwire presented biologically safer forces, despite its large cross section. However, the authors did not recommend the use of the tested multizone wires for tooth displacements more than 1.5 mm.19 Another study demonstrated a force difference between the posterior and anterior segments of multizone archwires to be at least 45%.34
Additionally, the present investigation compared new and retrieved wire pieces. The latter presented lower forces in all deactivation points, except for the last stages of deactivation. Specifically, the mean difference in force magnitude between new and retrieved archwires at the anterior segment and at the 3 mm unloading point was 0.18 N (7% of the force produced). The respective difference for the posterior segment was 0.76 N (17% of the force exerted by a new archwire).
A recent study demonstrated higher forces in new, non-multizone 0.016 NiTi archwires during the initial stages of deactivation, compared with retrieved specimens.35 This is in agreement with the present findings. Although this phenomenon is of doubtful clinical significance, it is an interesting future direction for research, since the cause remains obscure. Furthermore, studies have reported alterations in the mechanical and thermal properties of NiTi after clinical use. The hysteresis energy of NiTi gradually diminished after clinical use and X-ray diffraction analysis has shown irreversible austenite to martensite transformations.36
For the first time, the experiment results portray an intricate interplay between the mechanical and thermal properties of the Bioforce archwires. The BFR test is suitable for detecting the transformation temperatures of heat-activated NiTi archwires.37 The present study detected statistically significant differences in the As and Af transformation temperatures between the anterior and the posterior segments of Bioforce archwires in new and retrieved specimens. However, aging did not significantly affect the transformation temperatures. A recent study that evaluated several heat-activated non-multizone NiTi archwires using BFR and DSC, reported differences in BFR As/Af between archwires of different cross sections even from the same manufacturer.37 A further study conducted DSC for several rectangular multiforce NiTi wires, including Bioforce archwires. The recorded As temperatures ranged between 7.4–21.4°C in the anterior and 1.8–16.8°C in the posterior segments. Bioforce archwires demonstrated the highest As from the evaluated archwires. Af ranged between 22.7–38°C (30.2°C for Bioforce) in the anterior and 17.9–27.9°C (25.9°C for Bioforce) in the posterior segments.38 These temperatures were lower than those found in the present experiment, especially for the anterior segments of the tested archwires, which may be attributed to the inherent differences between the two techniques or the different archwire dimensions of the specimens used in the two protocols. Another possible explanation could involve the applied strain level. Studies that compare BFR and DSC show differences that may range between 4–6°C based on the testing methods.37 Similar results were demonstrated as long as the strain was ≤ 2.5% in the direction of the applied force;39-41 however, the transformation temperatures shifted to higher levels on the increasing strain,42 as in the present simulation model.
To obtain full advantage of the superelastic properties of the NiTi archwires, the TTR should be below the usual resting intraoral temperature (34°C)43 or even lower, since intraoral temperature fluctuates. Superelasticity is expected when the temperature rises above As and is fully expressed after the complete transformation from martensite to the austenite phase (Af).8 According to the present results, the Af temperature of these archwires during clinical use may lie higher than the average intraoral temperature, i.e., the wire segment ligated into the slots may not be fully austenitic after an increased activation. This is even more likely in the anterior wire sections, which showed increased Af. The clinical implication of this finding is related to the exerted force level that may fluctuate on consuming hot or cold beverages. In the BFR thermograms in the present experiment, the slope of the posterior segments was steeper compared with the slope of the anterior segments, in all subgroups, indicating a more rapid transformation during temperature fluctuations in this wire segment.
However, the transformation processes between austenite and martensite in NiTi orthodontic archwires is rather a complex mechanism.2 The R-phase appears to be an intermediate phase in the martensite–austenite transformation path in some NiTi products or in both the martensite–austenite and austenite–martensite paths in others.44 The austenitic transformations involving the R-phase begin below 0°C in superelastic NiTi alloys, but the nonsuperelastic NiTi is almost entirely martensite at room temperature and contains small amounts of austenite in the oral environment.2 Butler et al.40 did not report As values with DSC and stated that with the exact temperature at which austenite occurs is unclear with this method, due to overlapping between the completion of the R-phase and the onset of the austenitic phase.
The present experiment identified no differences in austenite transformation temperatures between new and retrieved specimens. Nevertheless, some irreversible transformation of austenite to martensite after the clinical use of NiTi archwires was demonstrated using X-ray diffraction analysis.36 Another recent study evaluated the thermal behavior of intraorally exposed and as-received copper-nickel-titanium wires with DSC. No difference was reported for all parameters (heating onset, endset, and enthalpy and cooling onset, endset, and enthalpy), with the exception of the retrieved 27°C wires that exhibited a significantly decreased heating enthalpy associated with the martensite to austenite transition. This indicates a lessening of the extent of phase transformation; however, the clinical significance of this reduction is unclear.45
Backscattered electron images showed the presence of elongated pores, a typical finding on orthodontic wire surface.46-48 This pattern is appended to extensive cold working during wiring.49 According to the present findings, the elemental composition (weight percentage) of the new Bioforce archwires was Ni, 52.6 ± 0.5 and Ti, 47.4 ± 0.5. Therefore, Bioforce archwires belong to the 55-Nitinol alloy family. These alloys present a higher shape memory effect and are comparatively easily processed by all forms of hot working than the 60-Nitinol forms.50
Even though the present study strengthens the evidence of the behavior of new and retrieved NiTi archwires, it has some limitations. Sectioned wires were used for testing. This cold working of the material may have affected the transformation temperature.27 Undoubtedly, differences exist in loading conditions between the oral cavity and the laboratory setup. Although highly reproducible, the three-point bend test is a laboratory simulation. The activation of the NiTi archwire, after its engagement into the bracket slot, is quite different than three-point bending tests in free NiTi wire segments. Moreover, the clinical extrapolation of the present findings on roughness is questionable. In vitro experiments may fail to reproduce intraoral factors that influence resistance to sliding, such as saliva, plaque, food debris, corrosion, temperature, and mastication.51
This study confirmed the existence of multiple force zones in new and retrieved Bioforce NiTi archwires. The Af temperature of these archwires may lie higher than the average intraoral temperature. Higher forces were recorded during the three-point bending test in new specimens, compared with retrieved (used intraorally for up to 6 months) specimens. Even at 2 mm deflections, the forces recorded from these archwires may lie beyond biologically safe limits.
ACKNOWLEDGEMENTS
We are grateful to Professor Spyros Zinelis (Department of Biomaterials, School of Dentistry, National and Kapodistrian University of Athens, Greece) for providing the equipment for the SEM/EDS analysis and for support and guidance while drafting this article. We thank Bitsaropoulos (Athens, Greece) for supplying the Bioforce archwires.
Notes
REFERENCES
1. Miura F, Mogi M, Ohura Y, Hamanaka H. 1986; The super-elastic property of the Japanese NiTi alloy wire for use in orthodontics. Am J Orthod Dentofacial Orthop. 90:1–10. DOI: 10.1016/0889-5406(86)90021-1. PMID: 3460342.
2. Bradley TG, Brantley WA, Culbertson BM. 1996; Differential scanning calorimetry (DSC) analyses of superelastic and nonsuperelastic nickel-titanium orthodontic wires. Am J Orthod Dentofacial Orthop. 109:589–97. DOI: 10.1016/S0889-5406(96)70070-7. PMID: 8659468.
3. Aghili H, Yasssaei S, Ahmadabadi MN, Joshan N. 2015; Load deflection characteristics of nickel titanium initial archwires. J Dent (Tehran). 12:695–704. PMID: 27148381. PMCID: PMC4854749.
4. Khier SE, Brantley WA, Fournelle RA. 1991; Bending properties of superelastic and nonsuperelastic nickel-titanium orthodontic wires. Am J Orthod Dentofacial Orthop. 99:310–8. DOI: 10.1016/0889-5406(91)70013-M. PMID: 2008890.
5. Andreasen G, Wass K, Chan KC. 1985; A review of superelastic and thermodynamic nitinol wire. Quintessence Int. 16:623–6. PMID: 3865259.
6. Kusy RP. 1997; A review of contemporary archwires: their properties and characteristics. Angle Orthod. 67:197–207. DOI: 10.1043/0003-3219(1997)067<0197:AROCAT>2.3.CO;2. PMID: 9188964.
7. Kusy RP, Whitley JQ. 2007; Thermal and mechanical characteristics of stainless steel, titanium-molybdenum, and nickel-titanium archwires. Am J Orthod Dentofacial Orthop. 131:229–37. DOI: 10.1016/j.ajodo.2005.05.054. PMID: 17276864.
8. Barwart O, Rollinger JM, Burger A. 1999; An evaluation of the transition temperature range of super-elastic orthodontic NiTi springs using differential scanning calorimetry. Eur J Orthod. 21:497–502. DOI: 10.1093/ejo/21.5.497. PMID: 10565090.
9. Brantley WA, Eliades T. 2001. Orthodontic materials: scientific and clinical aspects. Goerge Thieme Verlag;Stuttgart: DOI: 10.1055/b-002-43889.
10. Duerig TW Melton K, Stöckel D, Wayman C. 1990. Engineering aspects of shape memory alloys. Butterworth-Heinemann;London: p. 13–20. DOI: 10.1016/B978-0-7506-1009-4.50015-9.
11. Meling TR, Odegaard J. 2001; The effect of short-term temperature changes on superelastic nickel-titanium archwires activated in orthodontic bending. Am J Orthod Dentofacial Orthop. 119:263–73. DOI: 10.1067/mod.2001.112451. PMID: 11244421.
12. Bartzela TN, Senn C, Wichelhaus A. 2007; Load-deflection characteristics of superelastic nickel-titanium wires. Angle Orthod. 77:991–8. DOI: 10.2319/101206-423.1. PMID: 18004922.
13. Lombardo L, Toni G, Stefanoni F, Mollica F, Guarneri MP, Siciliani G. 2013; The effect of temperature on the mechanical behavior of nickel-titanium orthodontic initial archwires. Angle Orthod. 83:298–305. DOI: 10.2319/040612-287.1. PMID: 22908946. PMCID: PMC8793662.
14. Nakano H, Satoh K, Norris R, Jin T, Kamegai T, Ishikawa F, et al. 1999; Mechanical properties of several nickel-titanium alloy wires in three-point bending tests. Am J Orthod Dentofacial Orthop. 115:390–5. DOI: 10.1016/S0889-5406(99)70257-X. PMID: 10194282.
15. Sakima MT, Dalstra M, Melsen B. 2006; How does temperature influence the properties of rectangular nickel-titanium wires? Eur J Orthod. 28:282–91. DOI: 10.1093/ejo/cji079. PMID: 16199409.
16. Mirabella AD, Artun J. 1995; Risk factors for apical root resorption of maxillary anterior teeth in adult orthodontic patients. Am J Orthod Dentofacial Orthop. 108:48–55. DOI: 10.1016/S0889-5406(95)70065-X. PMID: 7598104.
17. Cannon JL. 1984; Dual-flex archwires. J Clin Orthod. 18:648–9. PMID: 6592172.
18. Thompson WJ. 1988; Combination anchorage technique: an update of current mechanics. Am J Orthod Dentofacial Orthop. 93:363–79. DOI: 10.1016/0889-5406(88)90095-9. PMID: 3284330.
19. Sanders E, Johannessen L, Nadal J, Jäger A, Bourauel C. 2022; Comparison of multiforce nickel-titanium wires to multistrand wires without force zones in bending and torque measurements. J Orofac Orthop. 83:382–94. DOI: 10.1007/s00056-021-00321-2. PMID: 34228142.
20. Lombardo L, Ceci M, Mollica F, Mazzanti V, Palone M, Siciliani G. 2019; Mechanical properties of multi-force vs. conventional NiTi archwires. J Orofac Orthop. 80:57–67. DOI: 10.1007/s00056-018-00164-4. PMID: 30610250.
21. ASTM International. 2016. ASTM F2082/F2082M-16. Standard test method for determination of transformation temperature of nickel-titanium shape memory alloys by bend and free recovery. ASTM;West Conshohocken: DOI: 10.1520/f2082_f2082m.
22. ASTM International. 2017. ASTM F2004-17. Standard test method for transformation temperature of nickel-titanium alloys by thermal analysis. ASTM;West Conshohocken: DOI: 10.1520/f2004-00.
23. Nespoli A, Villa E, Passaretti F, Albertini F, Cabassi R, Pasquale M, et al. 2014; Non-conventional techniques for the study of phase transitions in NiTi-based alloys. J Mater Eng Perform. 23:2491–7. DOI: 10.1007/s11665-014-1105-6.
24. Silness J, Loe H. 1964; Periodontal disease in pregnancy. II. Correlation between oral hygiene and periodontal condtion. Acta Odontol Scand. 22:121–35. DOI: 10.3109/00016356408993968. PMID: 14158464.
25. Bourauel C, Drescher D, Thier M. 1992; An experimental apparatus for the simulation of three-dimensional movements in orthodontics. J Biomed Eng. 14:371–8. DOI: 10.1016/0141-5425(92)90081-U. PMID: 1405553.
26. DIN Dentistry. 2020. Wires for use in orthodontics (ISO 15841:2014/Amd 1:2020). German version. Newark;iTeh, Inc.:
27. ASTM International. 2016. ASTM F2082-06. Standard test method for determination of transformation temperature of nickel-titanium shape memory alloys by bend and free recovery. ASTM;West Conshohocken: DOI: 10.1520/f2082_f2082m.
28. Sifakakis I, Eliades T. 2017; Laboratory evaluation of orthodontic biomechanics: the clinical applications revisited. Semin Orthod. 23:382–9. DOI: 10.1053/j.sodo.2017.07.008.
29. Krishnan V, Kumar KJ. 2004; Mechanical properties and surface characteristics of three archwire alloys. Angle Orthod. 74:825–31. DOI: 10.1043/0003-3219(2004)074<0825:MPASCO>2.0.CO;2. PMID: 15673147.
30. Pelton AR, Gong XY, Duerig T. 2004. Fatigue testing of diamond-shaped specimens. Paper presented at: International Conference on Shape Memory and Superelastic Technologies, SMST-2003. 2003 May 5-8; Pacific Grove, USA. SMST Society, Inc.;Menlo Park:
31. Theodorou CI, Kuijpers-Jagtman AM, Bronkhorst EM, Wagener FADTG. 2019; Optimal force magnitude for bodily orthodontic tooth movement with fixed appliances: a systematic review. Am J Orthod Dentofacial Orthop. 156:582–92. DOI: 10.1016/j.ajodo.2019.05.011. PMID: 31677666.
32. Proffit W. Proffit W, Fields H, Larson B, Sarver D, editors. 2018. The biologic basis of orthodontic therapy. Contemporary orthodontics. 6th ed. Elsevier Mosby;Louis: p. 278.
33. Sifakakis I, Pandis N, Makou M, Eliades T, Bourauel C. 2010; A comparative assessment of the forces and moments generated at the maxillary incisors between conventional and self-ligating brackets using a reverse curve of Spee NiTi archwire. Aust Orthod J. 26:127–33. PMID: 21175021.
34. Ibe DM, Segner D. 1998; Superelastic materials displaying different force levels within one archwire. J Orofac Orthop. 59:29–38. DOI: 10.1007/BF01321553. PMID: 9505053.
35. Elayyan F, Silikas N, Bearn D. 2008; Ex vivo surface and mechanical properties of coated orthodontic archwires. Eur J Orthod. 30:661–7. DOI: 10.1093/ejo/cjn057. PMID: 19011166.
36. Bradley TG, Maslowski MJ, Toth JM, Monaghan P. 2003; Reduction of mechanical properties of NiTi wires due to clinical use. J Dent Res. 82(special issue A):Abstract 1538.
37. Obaisi NA, Galang-Boquiren MT, Evans CA, Tsay TG, Viana G, Berzins D, et al. 2016; Comparison of the transformation temperatures of heat-activated Nickel-Titanium orthodontic archwires by two different techniques. Dent Mater. 32:879–88. DOI: 10.1016/j.dental.2016.03.017. PMID: 27183921.
38. Mehta ASK. 2015. Thermomechanical characterization of variable force NiTi orthodontic archwires [Master's Theses]. Marquette University;Milwaukee:
39. Chen JT, Duerig TW, Pelton AR, Stöckel D. 1995; An apparatus to measure the shape memory properties of Nitinol tubes for medical applications. J Phys IV. 5(C8):1247–52. DOI: 10.1051/jp4/1995581247.
40. Butler J, Tiernan P, Tofail SAM, Gandhi A. 2010. Probing martensitic transition in Nitinol wire: a comparison of X‐ray diffraction and other techniques. Paper presented at: International Conference on Advances in Materials and Processing Technologies. AIP Conference Proceedings. 2010 Jul 21-22; Portland, USA. American Institute of Physics;Melville: DOI: 10.1063/1.3552318.
41. Norwich DW. 2010. A Study of the properties of a high temperature binary Nitinol alloy above and below its martensite to austenite transformation temperature. SAES Memry Corporation;Bethel:
42. Pelton AR, Dicello J, Miyazaki S. 2000; Optimisation of processing and properties of medical grade Nitinol wire. Minim Invasive Ther Allied Technol. 9:107–18. DOI: 10.3109/13645700009063057.
43. Longman CM, Pearson GJ. 1987; Variations in tooth surface temperature in the oral cavity during fluid intake. Biomaterials. 8:411–4. DOI: 10.1016/0142-9612(87)90016-0. PMID: 3676430.
44. Otsuka K. Duerig TW, Melton KN, Stöckel D, Wayman CM, editors. 1990. Introduction to the R-phase transition. Engineering aspects of shape memory alloys. Butterworth-Heinemann Ltd.;London: p. 36–45. DOI: 10.1016/B978-0-7506-1009-4.50007-X.
45. Biermann MC, Berzins DW, Bradley TG. 2007; Thermal analysis of as-received and clinically retrieved copper-nickel-titanium orthodontic archwires. Angle Orthod. 77:499–503. DOI: 10.2319/0003-3219(2007)077[0499:TAOAAC]2.0.CO;2. PMID: 17465660.
46. Amini F, Rakhshan V, Pousti M, Rahimi H, Shariati M, Aghamohamadi B. 2012; Variations in surface roughness of seven orthodontic archwires: an SEM-profilometry study. Korean J Orthod. 42:129–37. DOI: 10.4041/kjod.2012.42.3.129. PMID: 23112943. PMCID: PMC3481981.
47. Usui T, Iwata T, Miyake S, Otsuka T, Koizumi S, Shirakawa N, et al. 2018; Mechanical and frictional properties of aesthetic orthodontic wires obtained by hard chrome carbide plating. J Dent Sci. 13:151–9. DOI: 10.1016/j.jds.2017.07.003. PMID: 30895111. PMCID: PMC6388802. PMID: 9108157bf2c749f7a1488293882d7278.
48. Pelsue BM, Zinelis S, Bradley TG, Berzins DW, Eliades T, Eliades G. 2009; Structure, composition, and mechanical properties of Australian orthodontic wires. Angle Orthod. 79:97–101. DOI: 10.2319/022408-110.1. PMID: 19123699.
49. Brantley W. Brantley W, Eliades T, editors. 2001. Orthodontic wires. Orthodontic materials. Thieme;Stuttgart: p. 78–100. DOI: 10.1055/b-002-43889.
50. Thompson SA. 2000; An overview of nickel-titanium alloys used in dentistry. Int Endod J. 33:297–310. DOI: 10.1046/j.1365-2591.2000.00339.x. PMID: 11307203.
51. Kusy RP, Whitley JQ, Prewitt MJ. 1991; Comparison of the frictional coefficients for selected archwire-bracket slot combinations in the dry and wet states. Angle Orthod. 61:293–302. Erratum in: Angle Orthod 1993;63:164. DOI: 10.1043/0003-3219(1991)061<0293:COTFCF>2.0.CO;2. PMID: 1763840.
Figure 1
Representative wire segment during the three-point bending test on Orthodontic Measurement and Simulation System. The 0.022-inch side is seen.
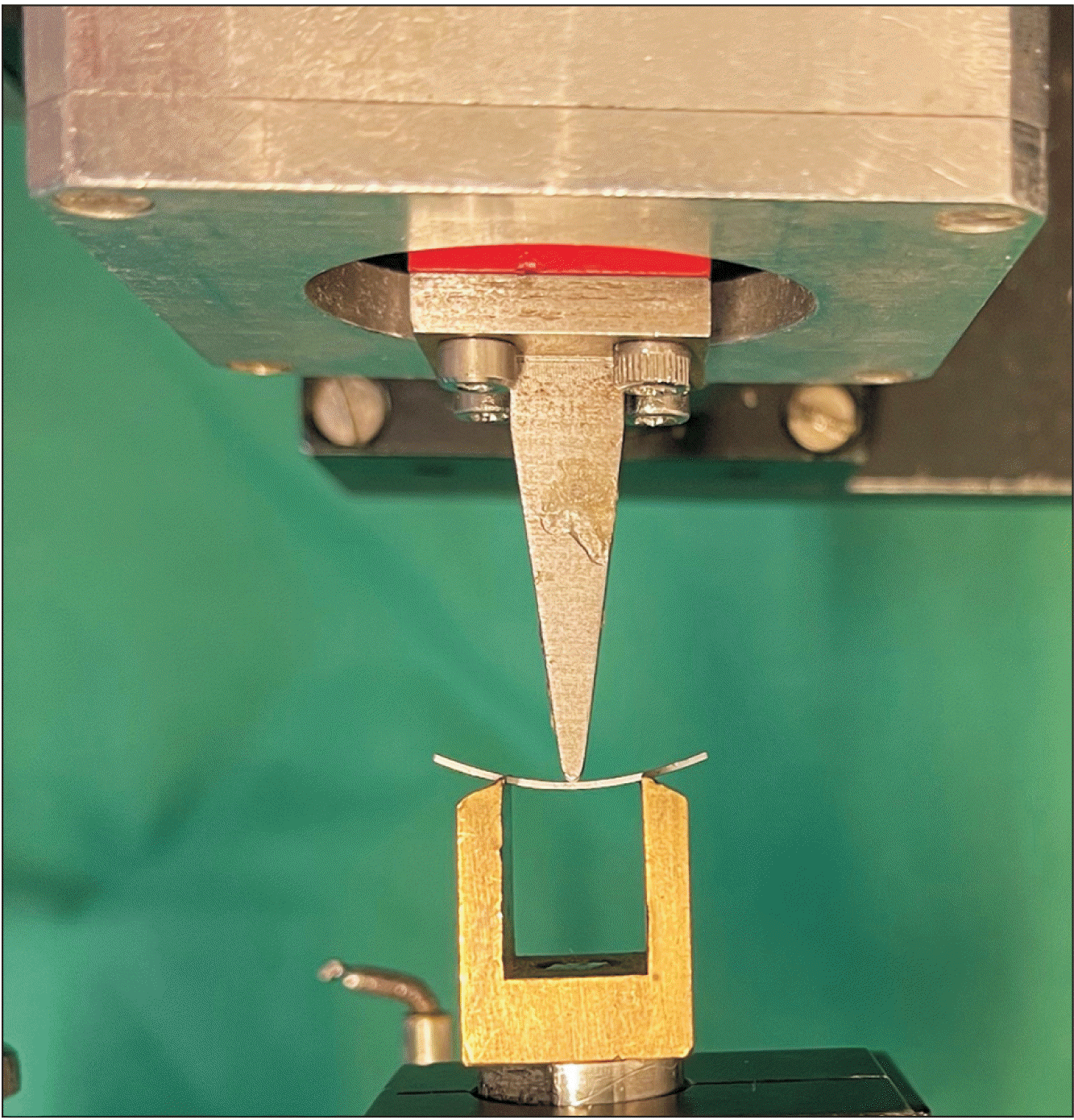
Figure 3
Three-point bending test. Representative curves of new and retrieved Bioforce archwires. The y-axis shows force in N corresponding to the displacement in mm in the x-axis during the bending test. The double-sided arrow indicates the linear part of the unloading curves used for calculating the bending moduli.
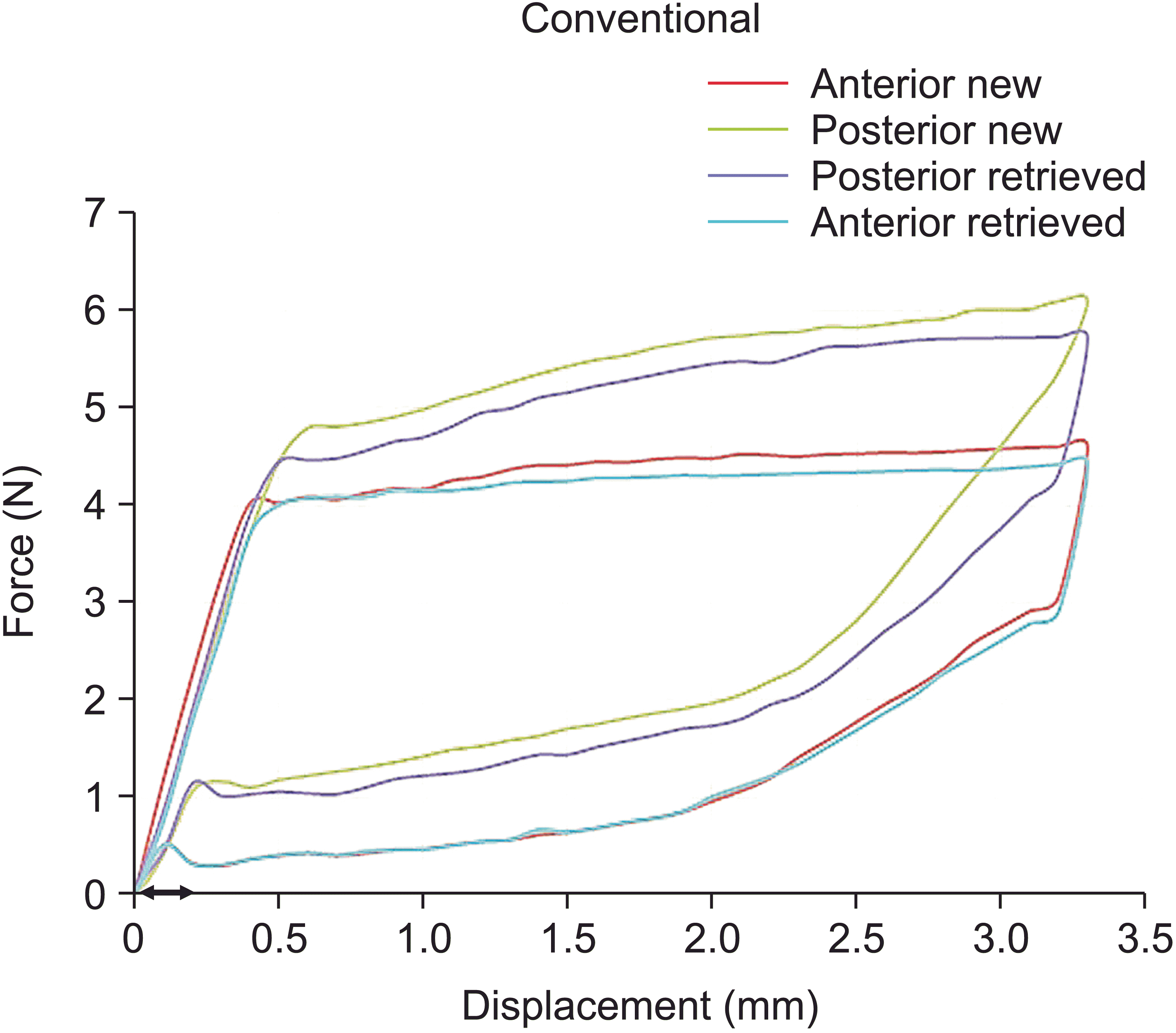
Figure 4
Bend and free recovery test. Typical temperature−displacement graphs of Bioforce archwires (anterior and posterior segments), depicting the austenite start and austenite finish transformation temperatures. The x-axis shows temperature in °C and the y-axis shows displacement in mm.
As, austenite start temperature; Af, austenite finish temperature.
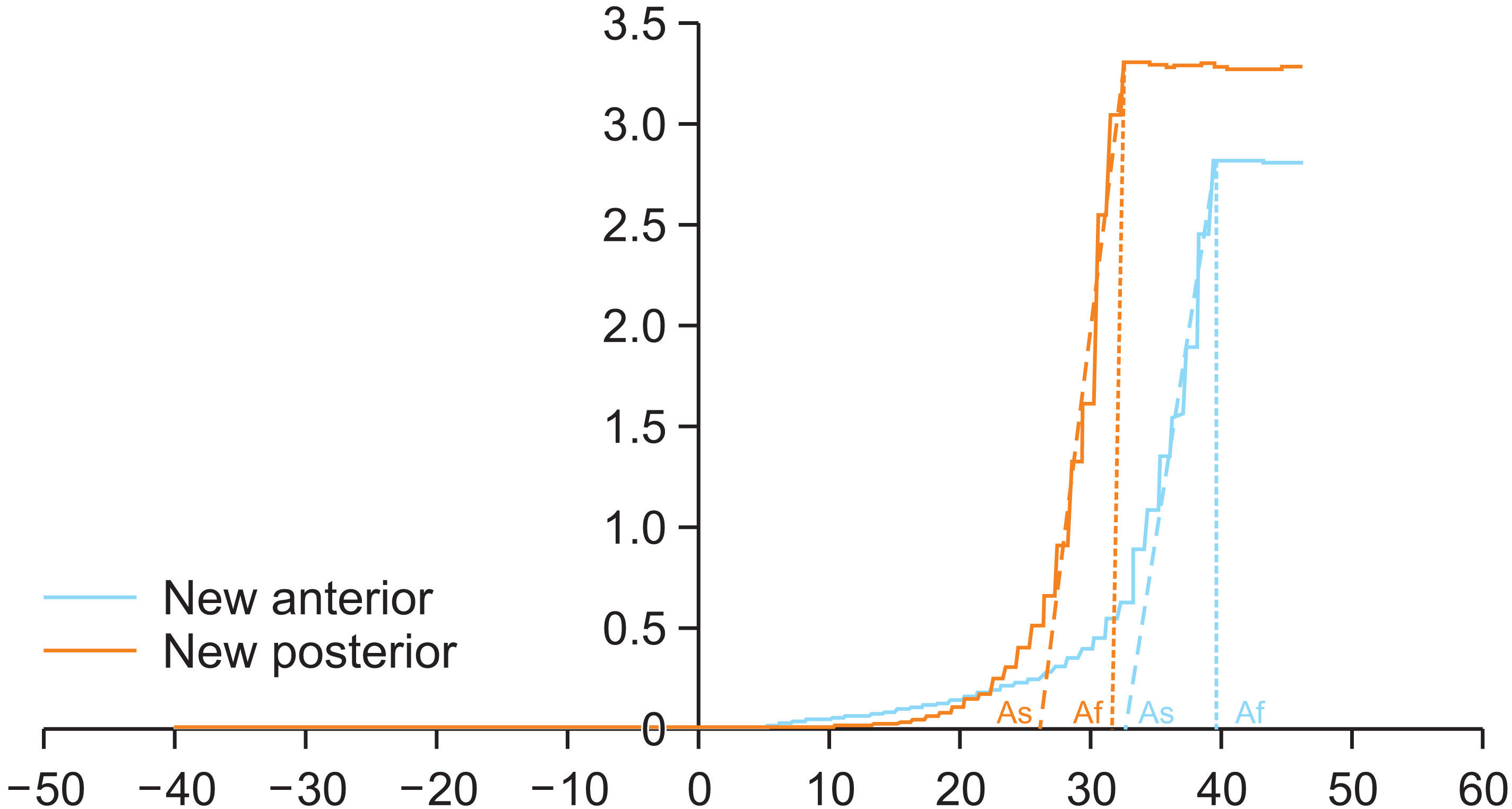
Figure 5
Representative backscattered electron image of the surface of a new wire. The long axis of the wire is oriented parallel to the horizontal axis of the image. The arrows point toward elongated pores along the horizontal axis (nominal magnification ×800).
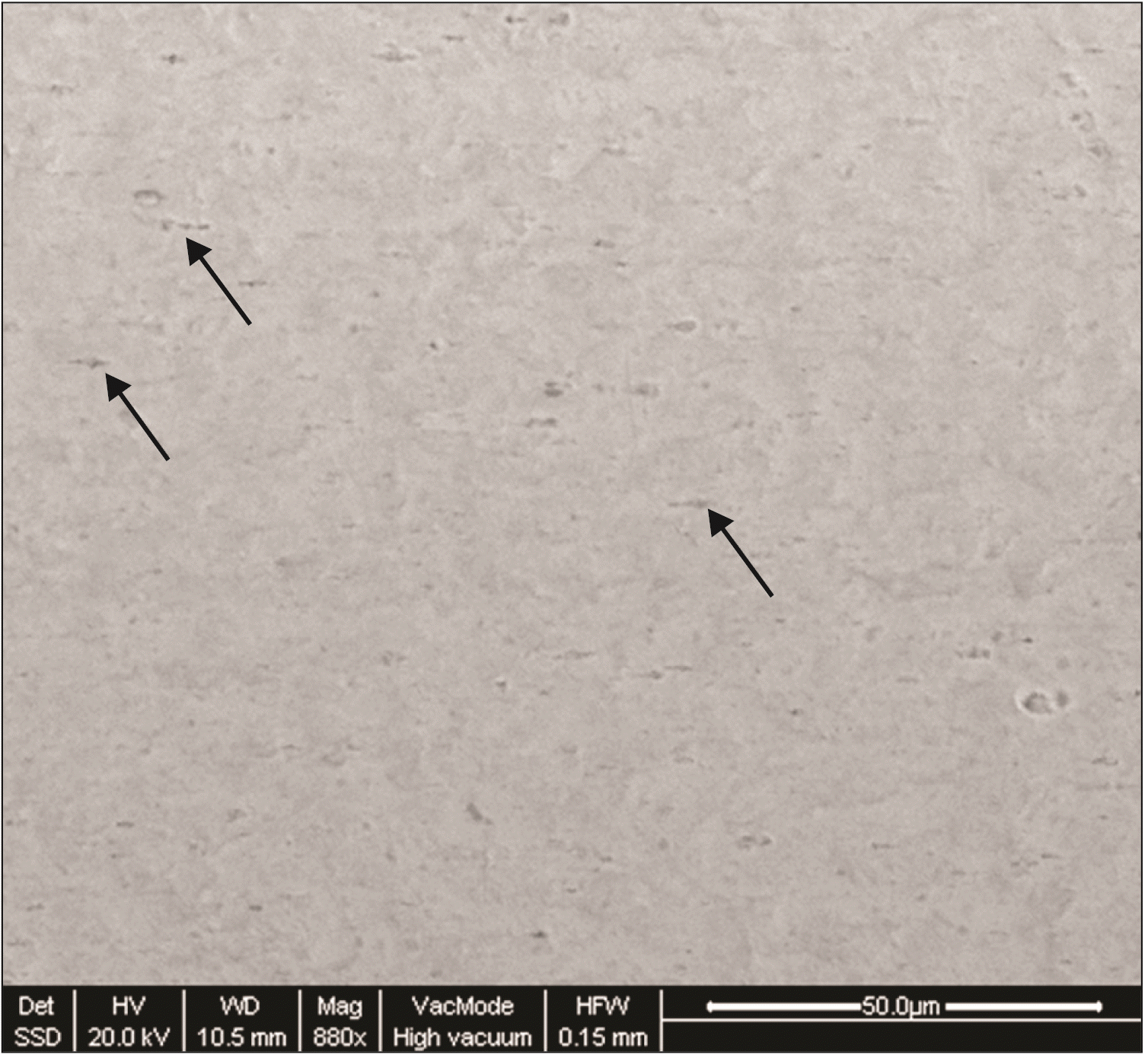
Figure 6
Representative energy dispersive X-ray spectra from the surface of the Bioforce archwire.
Ni, nickel; Ti, titanium.
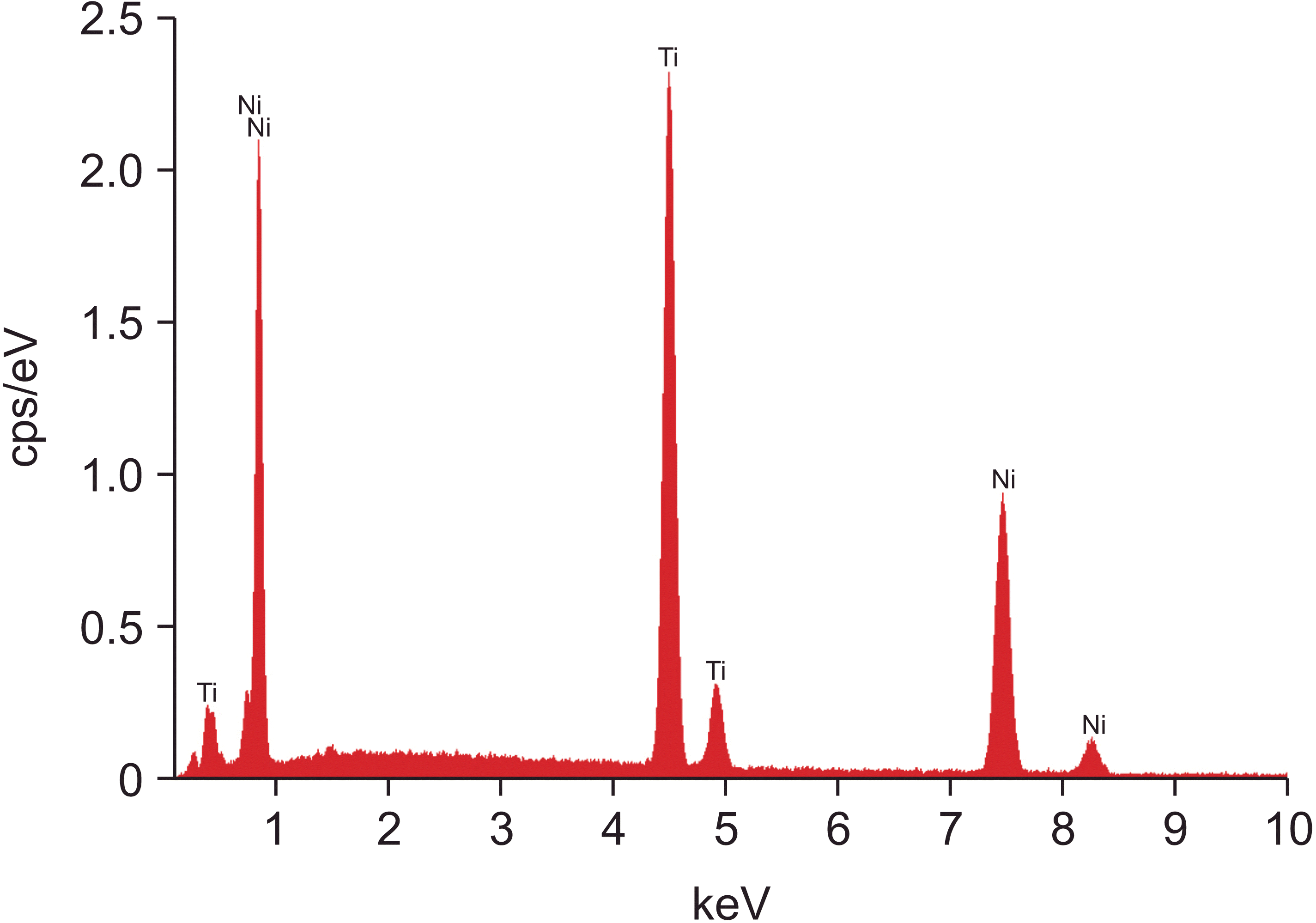
Table 1
Descriptive statistics of measurements by type of wire (retrieved or new) and by location of measurement (anterior or posterior)
Table 2
Results of 3-point bending tests
Measured variables | MD between posterior-anterior (N) | 95% CI | p-value |
---|---|---|---|
1 mm activation | 0.73 | (0.67, 0.79)* | < 0.001 |
2 mm activation | 0.80 | (0.77, 0.84)* | < 0.001 |
3 mm activation | |||
New wires | 1.78 | (1.69, 1.86)* | < 0.001 |
Retrieved wires | 1.19 | (0.99, 1.40)* | < 0.001 |
3.1 mm activation | |||
New wires | 1.87 | (1.78, 1.96)* | < 0.001 |
Retrieved wires | 1.32 | (1.11, 1.53)* | < 0.001 |
Bending modulus | |||
New wires | 2.17 | (0.95, 3.38)* | < 0.001 |
Retrieved wires | 4.17 | (1.23, 3.66)* | 0.023 |
Table 3
Results of 3-point bending tests
Measured variables | MD between retrieved-new (N) | 95% CI | p-value |
---|---|---|---|
1 mm activation | −0.06 | (−0.15, 0.02) | 0.152 |
2 mm activation | −0.03 | (−0.10, 0.05) | 0.499 |
3 mm activation | |||
Anterior segment | −0.18 | (−0.29, −0.07)* | 0.002 |
Posterior segment | −0.76 | (−0.99, −0.53)* | < 0.001 |
3.1 mm activation | |||
Anterior segment | −0.20 | (−0.31, −0.09)* | < 0.001 |
Posterior segment | −0.75 | (−0.98, −0.52)* | < 0.001 |
Bending modulus | |||
Anterior segment | −2.67 | (−4.11, −1.22)* | < 0.001 |
Posterior segment | −0.67 | (−3.83, −0.94)* | 0.023 |