Abstract
Health impacts of particulate matter (PM) have become a global concern. PM tends to affect patients with allergic diseases, such as allergic rhinitis (AR), more severely. Many epidemiological studies have shown that PM increases outpatient clinic visits as well as the prevalence and severity of AR, while decreasing the quality of life of AR patients. However, the exact mechanisms underlying the effects of PM on the development and exacerbation of AR are still poorly understood. Various mechanisms are involved in the effects of PM on AR, including immunological response, oxidative stress, epithelial barrier dysfunction, allergic sensitization, and epigenetic modification. This article reviews epidemiological and clinical studies on the effects of PM on AR, and the mechanisms by which PM aggravates AR.
기술이 발전하고 산업이 고도화되면서 인류의 삶은 양적으로 팽창하고 편리해졌지만, 그와 동반된 환경오염으로 인해 인류는 커다란 건강 위험에 직면하게 되었다. 2016년 시행한 세계보건기구(World Health Organization, WHO) 연구에 의하면 세계 인구의 91% 이상이 공기질 권장기준치 이하의 지역에 살고 있으며, 대기오염에 의한 조기 사망이 연간 420만명에 이른다[1]. 우리나라 역시 공업화에 따른 자동차의 증가, 인접국의 산업화로 인해 대기오염이 심해지고 있다. 우리나라에서는 국립환경과학원을 중심으로 미세먼지에 대한 특성 분석 및 건강 관련 연구가 이루어졌으며[2], 미세먼지의 측정 기술이 대중화되고, 미세먼지 농도에 대한 정보를 인터넷 또는 스마트폰을 통해 쉽게 접근할 수 있어 의료인뿐만 아니라 일반인의 미세먼지에 대한 관심도 높아졌다. 최근에는 미세먼지의 건강 위해성이 밝혀지면서, 그 어느 때보다도 국민적 관심이 높다.
이러한 상황에서 미세먼지의 호흡기질환에의 영향에도 관심이 높아지고 있으며, 기존의 연구들을 통해 미세먼지가 다양한 호흡기질환의 발현과 악화의 원인이 된다고 밝혀져 있다. 알레르기비염은 대표적인 상부 호흡기알레르기 질환 중 하나로, 최근 미세먼지 및 대기오염 등 환경적인 요인이 작용하여 그 유병률이 지속적으로 증가하고 있는 추세이다[3]. 하지만 미세먼지가 알레르기비염에 미치는 영향에 대한 정확한 이해는 여전히 부족한 상황이며, 이에 본 종설에서는 미세먼지의 정의와 특성에 대해서 기술하고, 문헌 고찰을 통해 미세먼지가 알레르기비염에 미치는 영향과 기전에 대해 살펴보고자 한다.
대기오염은 인위적으로 배출된 오염 물질이 대기 중 한 가지 또는 그 이상 존재하여 오염 물질의 농도, 양 및 지속 시간이 어떤 지역 불특정 다수에게 불쾌감을 일으키거나 해당 지역에 공중보건상 위해를 끼치고 인간이나 동식물 등의 활동에 해를 주어 생활과 재산을 향유할 권리를 방해받는 상태로 정의된다[4]. 우리나라는 대기환경보전법 시행규칙을 통하여 대기오염 물질을 아황산가스, 일산화탄소 등 가스 물질과 먼지 같은 입자 물질을 포함하여 총 64종으로 정하고 있으며, 이 중 특히 인체나 생물에 유해하다고 알려진 35종을 특정대기유해물질로 정하여 관리하고 있다[5].
미세먼지는 대기 중에 떠다니는 작은 크기의 고체 입자와 액체 방울의 혼합물을 이르는 용어로, 크기에 따라 지름 10 μm 이하를 미세먼지(PM10), 2.5 μm 이하를 초미세먼지(PM2.5), 1.0 μm 이하를 극미세먼지(PM1.0)로 정의하고 있다[6]. 이는 흙먼지나 꽃가루 등 자연적으로 생성된 물질도 포함하지만 더 많은 부분은 교통수단이나 산업활동에 의해 생성되는 인위적 물질로, 탄소 성분, 이온 성분(염소, 질산, 암모늄, 나트륨 등), 금속 성분(비소, 납, 수은 등), 다환방향족 탄화수소(벤조피렌 등) 등 다양한 성분이 포함된다[6]. 초미세먼지의 경우 58.3%가 대기오염물질이 공기 중에서 반응해 형성한 황산염, 질산염 등이며 그 다음이 화석연료를 태우는 과정에서 발생하는 탄소류와 검댕(soot)이다[7].
미세먼지는 그 크기에 따라 침착 또는 흡수되는 부위가 다르고, 건강에 미치는 영향도 다르다고 알려져 있다. 미세먼지는 기류의 흐름에 따라 기도를 지나는 과정에서 기관지 또는 기관 분지에 직접적인 충돌 후 침착되며, 미세먼지 입자 크기와 무게로 인한 중력 침강 현상으로 인해 침착되기도 한다[8].
PM10은 지름 10 μm 이하인 미세먼지로 분쇄나 연삭 과정을 통해 발생하며, 꽃가루, 부유 먼지, 곰팡이 포자, 석탄재 등이 포함되어 있다. 흡입된 후에는 입자의 크기가 크기 때문에 주로 상기도인 비강이나 큰 기관지에 침착된다.
초미세먼지는 지름이 2.5 μm 이하인 미세먼지로 자동차 배기가스나 발전 시설, 산불, 산업 공정을 포함한 다양한 연소 과정을 통해 발생하게 된다. 주된 성분은 유기탄소, 질산이온, 황산이온이며, 자동차 연료에서 생성된 질소산화물, 아황산화물, 탄소산화물, 오존 등과 암모니움, 다환방향족 탄화수소 및 금속류(납, 니켈, 카드뮴, 구리, 망간, 비소 등)가 포함된다[9]. 그 밖에 건축, 철거 과정 또는 바람에 날린 토양 등에서 발생한 칼슘염, 바닷물에서 발생한 염화나트륨, 유기탄소 등이 포함된다. 초미세먼지는 PM10에 비해 상대적으로 크기가 작아 대기 중에 머무르는 시간이 더 길며, 소기도와 폐포까지 흡입되어 침착될 수 있다. 그 결과, PM2.5는 호흡기와 심혈관계 건강에 미치는 영향이 PM10에 비하여 더 크다고 알려져 있다[10,11]. 1.0 μm보다 작은 극미세먼지(PM1.0)는 금속이나 다른 유기화합물의 수송체로서 작용할 수 있는데, 상대적으로 폐포와 혈관에까지 더 잘 침투되어 건강에 미치는 영향이 클 것으로 추정되고 있다[12].
미세먼지가 건강에 영향을 미치는데 입자의 크기 이외에 중요한 요인으로, 미세먼지의 화학적 조성이 있다. 미세 먼지의 화학적 조성은 발생원에 따라 차이가 나며, 산업화 정도 및 교통량 등의 차이로 인해 지역별로 다르게 나타난다[13]. 미세먼지는 오염원으로부터 바로 발생하는 일차 입자와 이들 입자가 다른 물질과 화학반응을 일으켜 발생되는 이차 입자로 나누어 볼 수 있다. 일차 입자는 주로 자동차 배기가스, 엔진 윤활제, 타이어나 제동장치의 마모 또는 공장연료 연소에 의해 발생하며, 그 외 채석장, 건설 현장, 그리고 사막에서 발생한 먼지와 꽃가루 등 공기 중으로 직접 배출되는 것들에 의해 발생할 수 있다. 이차 입자는 대기 중에 이미 배출되어 있는 황산화물, 질산화물 및 암모니아 등의 오염 물질이 화학 반응을 통하여 생성되는 것을 말하며, 이에는 황산염, 질산염, 이산화황, 질소산화물, 암모니아, 그리고 유기 성분을 포함하는 에어로졸 등이 있다[14].
미세먼지는 건강에 대한 위해성으로 최근 많은 주목을 받고 있으며, 1980년대 후반부터 보고된 다양한 연구에서 연구가 시행된 국가, 연구 디자인 등이 각각 다르지만, 사망률의 증가, 심혈관 및 호흡기계 위해 영향, 암 질환 증가 등에 대한 관련성이 공통적으로 나타났다[4]. 미세먼지의 농도에 따른 상대적 사망 위험도를 보면 PM10이 20 μg/mm3 증가할 때마다 위험도는 0.4%-1.5% 높아지고, PM2.5의 경우 10 μg/mm3 증가할 때마다 위험도가 0.6%-1.2% 증가한다[15]. 다른 연구에서는 PM2.5의 발생을 줄여 대기질이 개선되면 인류의 평균 수명이 0.9년 증가될 것으로 보고한 바 있다[16]. 2000년 연구에서도 PM10이 10 μg/m3 증가할 때, 전체 사망률이 0.51% 증가한다고 보고하였으며[17], 미국 암학회가 2002년 미국 성인 50만명을 대상으로 시행한 연구에서도 PM10이 10 μg/m3 증가할 때, 전체 사망률이 4% 증가함을 보고하여[18] 미세먼지의 위험성이 구체적으로 기술된 바 있다. 기대여명 단축과 관련해서는 PM2.5 농도와 기대여명이 반비례함을 보고한 연구와[19], WHO가 수행한 메타분석 연구에서는 미세먼지에 연중 장기간 노출된 경우 PM2.5가 10 μg/m3 증가할 때마다 30세 이상 성인에서 22개월의 기대여명 손실이 발생하는 것으로 보고하였다[20].
이와 같이 대기오염은 운동 부족, 고염식, 고지질혈증, 약물 오남용과 같이 일반적으로 알려진 조절 가능한 질병 위험인자보다 질병 발생 위험도가 더 높으며[21], 질병 발생 외에도 조기 사망, 건강수명 감소와 노동생산성 저하에도 영향을 미친다[22]. 폐암 등에 대한 영향이 밝혀지면서 2013년 국제암연구소는 미세먼지를 1군 발암물질로 규정한 바 있으며[23], 이외에도 미세먼지는 중요한 내분비 교란물질로서 비만, 당뇨병과 같은 대사질환의 발병 및 악화에도 영향을 주는 것으로 알려져 있다[24,25].
미세먼지는 호흡기 증상을 유발 또는 증가시키고, 폐기능을 저하시킨다[26]. PM2.5에 해당하는 디젤분진(diesel exhaust particle, DEP) 급성 노출은 코와 눈의 자극, 폐기능 감소, 두통, 피곤감, 구역 등의 증상을 나타낸다[27]. 3일간 미세먼지 평균농도가 증가할 때 폐기능이 저하되고 기도 염증이 증가한다는 보고도 있다[28]. 또한, 천식이 잘 조절되지 않는 소아, 중증 지속성 천식에서 더 예민하게 반응하여, 일평균 미세먼지량 또는 시간당 최고치가 높을 때 최대호기유속이 더욱 감소하는 것으로 알려져 있다[28].
장기간 미세먼지 노출 역시 천식 환자의 증상 빈도를 높이고, 증상의 원활한 조절을 저해한다. 1년 평균미세먼지 농도의 증가가 천식 조절을 어렵게 한다고 알려져 있으며, 중증 발작이나 집중 치료가 필요한 상황으로 이어질 가능성이 높다[29]. 1년 평균농도가 12 μg/m3 증가할 때 입원이 19% 증가하고, 집중 치료의 필요성은 26% 증가되었다[30]. 우리나라에서 시행한 연구에서, 2000-2011년 만성 폐쇄성 폐질환 입원과 PM10 사이의 연관성을 확인한 결과, PM10이 10 μg/m3 증가할 때마다 만성 폐쇄성 폐질환 환자에서 입원이 2.7% (95% CI: 1.9-3.6) 증가하였고, 만성 폐쇄성 폐질환으로 인한 사망 또한 1.1% (95% CI: 0.8-1.4) 증가하였다[31]. 또한, 나이가 어릴수록 미세먼지 노출에 따른 천식 발생 위험이 증가하는데, 1세 미만에 노출될수록 모세기관지염의 위험도가 높아진다거나 7세에 천식 발생 위험이 증가한다는 보고가 있다[32,33].
대표적인 호흡기알레르기질환 중 하나인 알레르기비염은 콧물, 코막힘, 재채기, 코가려움증을 주증상으로 하는 질환이다. 알레르기비염의 유병률은 전세계적으로 성인에서 약 10%-30% 정도로 보고되고 있다. 우리나라에서도 16.2%로 보고된 바 있으나[34], 알레르기비염은 병원에서 진단되지 않고 자가로 치료하는 경우도 많아 실제로는 유병률이 더 높을 것으로 추정하고 있으며, 미세먼지나 배기가스 및 공장에서 배출되는 매연과 같은 대기오염 등의 환경적인 요인이 작용하여 비염 유병률은 점차 늘어나고 있는 추세이다[3,35].
미세먼지와 알레르기비염의 연관성에 대해서 다양한 연구 결과들이 보고된 바 있으며, 이는 알레르기비염에 대한 서로 다른 정의, 미세먼지 노출 정도 및 시기의 차이, 대상 연령, 그리고 지역적 차이 등 연구 설계의 차이에서 기인한다[36]. 대기오염 정도가 심해지면 알레르기비염 환자의 외래 방문 횟수가 늘어난다고 알려져 있다[37]. 스페인에서 1996과 2009년을 비교한 연구에서 알레르기비염 증상점수가 높아졌고, 이는 미세먼지와 관련된다고 보고하였으며[38], 스웨덴에서 시행한 연구에서도 자동차 배기가스 노출이 많아질수록 알레르기비염 유병률이 높아졌다[39]. 베이징에서 시행한 전향적 연구에서도 PM10 증가와 알레르기비염 진단 및 병원 방문 횟수 증가 사이에 상관관계를 보였다[40]. 우리나라에서 시행한 다기관 연구에서도 큰 도로 주변 대기오염이 알레르기항원 감작과 알레르기비염의 진단 및 폐기능 저하와 연관성이 높다는 점을 확인한 바 있으며[41], 서울에서 시행된 연구에서는 도로 주변에 거주하는 소아에서 자동차 배기가스 및 미세먼지 자극에 의한 알레르기비염 유병률에서 통계적 경향성을 확인하였다[42]. 장기간 노출될 경우 알레르기비염 빈도 뿐만 아니라 중증도 또한 증가하였으며, 유럽에서 시행한 연구에서 미세먼지에 장기간 노출되면 경증(odds ratio [OR], 1.20; 95% confidence interval [CI], 0.88-1.64), 중등도(OR, 1.53; 95% CI, 1.07-2.19), 중증(OR, 1.72; 95% CI, 1.23-2.41)으로 장기간의 미세먼지 노출 및 자극이 알레르기비염 중증도에 영향을 미칠 수 있다고 보고하였다[43].
알레르기비염은 대기오염, 계절적 영향 등 환경적 요인뿐 아니라 개인의 유전자형에 따른 후생유전학적 영향도 받게 되며, 이에 따라 면역 세포나 점막 면역의 반응이 차이를 보이게 되는, 다양하고 복잡한 기전으로 나타나는 질환이다. 현재 미세먼지가 어떤 특정한 기전을 통하여 알레르기비염을 일으키거나 악화시킨다고 명확히 밝혀진 바는 없지만, 현재까지 보고된 다양한 기전에 대해 정리해보고자 한다(Table 1).
알레르기비염은 대표적인 Th2 면역반응에 의한 질환으로 잘 알려져 있으며, 미세먼지도 항원과 동시에 노출할 때, 기존의 Th2 면역반응을 촉진시켜, IgE 생산과 호산구의 증가를 유발한다는 것이 가장 기본적인 기전이다[44,45]. 미세먼지가 항원제시세포 등에 감지되면 염증 유전자의 발현을 상향 조절하고, 그 결과 비내 상피세포에서 염증성 사이토카인(IL-6, IL-8, GM-CSF, TNF α 등) 분비를 유발하게 된다[46-49]. 또한, 미세먼지는 Th2 반응을 담당하는 수지상세포의 CD80, CD86 등의 발현을 상향 조절하며, 생체 내에서 수지상세포 및 호염기구를 활성화하여 Th2 관련 사이토카인인 IL-4와 IL-5의 생성을 유도한다[44,45]. 마우스를 이용한 동물 실험에서 미세먼지 동시 자극이 Th2 반응을 더 강하게 유발하였으며, 기관지 폐포관류액(bronchoalveolar lavage fluid, BALF)에서의 Th2 사이토카인과 호산구의 증가를 확인하였다[50]. 또한, 돼지풀, 집먼지진드기에 DEP 자극을 추가한 연구에서 Th2 면역반응의 부가효과를 보여주었다[51].
최근 몇몇 연구에서 미세먼지와 Th17과 관련한 면역학적 변화를 보고하였으며, 마우스를 이용한 동물 실험에서 미세먼지가 Th17 염증과 비강 점막에서 호중구의 증가를 유발하였으며, 미세먼지의 다환방향족 탄화수소(polycyclic aromatic hydrocarbon)와 같은 aryl hydrocarbon receptor (AhR) ligand는 T세포의 AhR에 작용하여 Th17 세포의 분화를 유발하였다[52-54]. 소아천식 환자 대상 연구에서는 DEP에 노출되었을 때 6배 이상의 혈청 IL-17A가 측정되었고[55], 저자들의 연구에서도 집먼지진드기 감작 알레르기비염 마우스에 DEP를 노출한 경우 비염 증상 점수의 증가와, 기관지폐포세척액에서 호중구의 증가, 그리고 비강 점막에서 IL-17A의 증가를 보고한 바 있다(Fig. 1) [56].
대기오염물질에 의해 조직에 산화스트레스가 축적되고, 그 결과 염증반응과 세포 사멸을 유발하여 궁극적으로 알레르기비염을 발현시킨다는 가설이 제시된 바 있다[57]. 대기오염물질에 의해 비 IgE 매개 염증반응이 유발되어 superoxide anion, hydrogen peroxide, hydroxyl radicals 등의 활성산소종이 생성되고, 이는 조직 자체의 항산화기전(glutathione, superoxide dismutase)으로 산화환원반응에 의해 대사되어 중화된다[58]. 하지만 활성산소종의 양이 증가되어 조직 자체의 항산화기전으로 처리가 안되면 염증 단계가 발생하여 glutathione S-transferase, heme oxygenase 1 등의 항산화효소가 새로 생성되며, 이 과정에 nuclear factor erythroid 2-related factor2, activator protein 1, NF-κB 등의 전사인자가 관여한다[59]. 이러한 염증반응은 조직의 세포 단백을 산화시키고 DNA를 손상시켜 기도 손상을 일으키게 되며, 결과적으로 염증세포들이 동원되고 면역반응이 활성화되어 알레르기비염이 악화하게 된다[60-64].
기도 상피세포는 유해한 흡입 물질이나 외부 항원의 침입으로부터 점막하 조직을 보호하는 물리적 장벽 역할을 한다. 이러한 상피장벽기능은 세포 사이의 밀착연접(tight junction)과 부착연접(adherens junction)의 상태에 의해 결정되는데, 미세먼지 노출이 이들 연접의 변화를 일으켜 상피장벽기능이 떨어진다는 결과들이 보고되고 있다[65-67]. 미세먼지 노출로 알레르기비염 마우스 비점막의 밀착연접과 ZO-1 단백질 발현이 유의하게 감소하였으며, 기도과민성이 증가하고 비염 증상이 증가하였다[68]. 최근 보고된 연구에서도 비강상피세포에 PM2.5를 노출시킨 연구에서 전기저항성(transepithelial electrical resistance)이 유의하게 감소하고, fluorescein isothiocyanate 투과성은 유의하게 증가하였으며, claudin-1 mRNA와 claudin-1, occludin, ZO-1 등의 밀착연접단백질의 발현이 유의하게 감소하였고, 염증유발 사이토카인(IL-8, TIMP-1, TSLP)이 유의하게 증가하여, 미세먼지에 의한 상피장벽기능 저하를 알레르기비염 민감성을 높이는 기전으로 보고하였다[69].
미세먼지가 알레르겐에 대한 감작을 촉진한다는 것은 여러 역학연구와 실험실 연구 결과가 뒷받침하고 있다. 미세먼지가 보조제(adjuvant)로 작용하여 알레르기항원에 대한 감작을 증가시키게 되면, 그 결과 항원제시세포를 보다 활성화시키고, type 2 helper T-cells의 분화를 활성화하게 된다[70]. 프랑스에서 시행한 연구에서 미세먼지 농도와 꽃가루에 대한 감작이 유의한 연관성을 보였으며(OR, 1.35; 95% CI, 1.09-1.68) [71], 독일에서 시행한 출생코호트 연구에서도 미세먼지 노출은 개별 항원(OR, 1.45; 95% CI, 1.21-1.74)과 꽃가루(OR, 1.52; 95% CI, 1.23-1.87) 감작을 증가시켰다[72]. 또한, 메타분석에서도 미세먼지 노출이 흡입 알레르기항원 감작을 유의하게 증가시키는 것으로 확인된 바 있다[73].
대기오염에 유사하게 노출이 된 경우에도 이로 인한 건강 영향은 개개인이 다르며, 성별이나 연령, 체질량지수 등 여러 인자가 영향을 미치는 것으로 보고되고 있다[74,75]. 대기오염물질이 건강에 미치는 영향이 개인의 유전 감수성에 따라 차이가 있음을 보여주는 기존의 유전적 연구들에서, 항산화기전에 관여하는 GSTM1, GSTT1, GSTP1 등의 효소 유전자와 TNF, TGF-β1 등 염증성 사이토카인의 유전자 변이가 유의한 연관성을 보여준 바 있다[76]. 후생유전학적 연구로는, 대기오염물질이 천식 경로에 관련된 유전자의 메틸화에 작용하는 근거들이 제시되고 있으며[77-79], 항산화기전에 관여하는 유전자가 후생유전학적 수정을 통해 폐포에서의 투과성은 증가되고, 점막 섬모운동을 통한 청소율은 저하되고, 포식세포의 기능이 떨어지면서 결과적으로 기도 염증을 일으킨다는 결과도 보고된 바 있다[80]. 또한, 면역조절 유전자인 Foxp3 유전자의 과메틸화를 통한 후생유전학적 수정이 조절 T세포의 기능을 약화시켜 면역반응을 유발한다는 보고도 있다[81,82].
미세먼지는 전세계적으로 조기 사망을 유발하며 기대여명을 단축하는 심각한 환경 위험인자이다. 또한 상하기도 호흡기알레르기질환에 영향을 미치며, 알레르기비염의 밝혀진 위험인자이다. 본 종설에서는 역학, 임상, 실험실 연구 결과를 통해 미세먼지의 알레르기비염에 대한 영향 및 관련 기전을 이해하고자 하였으며, 미세먼지는 면역학적 변화, 산화스트레스, 상피장벽기능 이상, 알레르기 감작, 후생유전학적 수정 등 다양한 기전을 통해 알레르기비염에 영향을 줄 것으로 생각된다(Fig. 2). 향후 미세먼지에 의한 비염 악화 기전을 규명하는 추가적인 연구와 기전에 따른 치료제 발굴 또한 필요할 것으로 사료된다.
ACKNOWLEDGMENTS
This research was supported by the Research Program funded Korea National Institute of Health (2021-ER1203-01) and SNUH fund (0420213160).
REFERENCES
1. World Health Organization. Ambient (outdoor) air pollution. [cited 2022 Oct 17]. Available from: URL: https://www.who.int/newsroom/fact-sheets/detail/ambient-(outdoor)-air-quality-and-health.
2. Air Quality Research Division. Study on the composition of PM2.5 in the yellow sand and fine particle. Incheon: National Institute of Environmental Research;2008.
3. Zou QY, Shen Y, Ke X, Hong SL, Kang HY. Exposure to air pollution and risk of prevalence of childhood allergic rhinitis: A meta-analysis. Int J Pediatr Otorhinolaryngol. 2018; 112:82–90.
4. Prüss-Üstün A, Corvalán CF; World Health Organization. Preventing disease through healthy environments: towards an estimate of the environmental burden of disease. [cited 2022 Oct 17]. Available from: URL: https://apps.who.int/iris/handle/10665/43457.
5. National Institute of Environmental Research (NIER). Annual report of air quality in Korea 2017. [cited 2022 Oct 17]. Available from: URL: https://www.airkorea.or.kr/web/detailViewDown?pMENU_NO=125.
6. World Health Organization. Ambient air pollution: a global assessment of exposure and burden of disease. [cited 2022 Oct 17]. Available from: URL: https://apps.who.int/iris/handle/10665/250141.
7. Myung JP. Health effects of particulate matter. Korean J Med. 2016; 91(2):106–13.
8. Asgharian B, Price OT, Oldham M, Chen LC, Saunders EL, Gordon T, et al. Computational modeling of nanoscale and microscale particle deposition, retention and dosimetry in the mouse respiratory tract. Inhal Toxicol. 2014; 26(14):829–42.
9. Kelly FJ, Fussell JC. Size, source and chemical composition as determinants of toxicity attributable to ambient particulate matter. Atmos Environ. 2012; 60:504–26.
10. Brook RD, Rajagopalan S, Pope CA 3rd, Brook JR, Bhatnagar A, Diez-Roux AV, et al. Particulate matter air pollution and cardiovascular disease: An update to the scientific statement from the American Heart Association. Circulation. 2010; 121(21):2331–78.
11. Ristovski ZD, Miljevic B, Surawski NC, Morawska L, Fong KM, Goh F, et al. Respiratory health effects of diesel particulate matter. Respirology. 2012; 17(2):201–12.
12. Utell MJ, Frampton MW. Acute health effects of ambient air pollution: The ultrafine particle hypothesis. J Aerosol Med. 2000; 13(4):355–9.
13. Kothai P, Saradhi IV, Pandit GG, Markwitz A, Puranik VD. Chemical characterization and source identification of particulate matter at an urban site of Navi Mumbai, India. Aerosol Air Qual Res. 2011; 11(5):560–9.
14. Begum BA, Biswas SK, Hopke PK. Source apportionment of air particulate matter by chemical mass balance (CMB) and comparison with positive matrix factorization (PMF) model. Aerosol Air Qual Res. 2007; 7(4):446–68.
15. Stieb DM, Judek S, Burnett RT. Meta-analysis of time-series studies of air pollution and mortality: Effects of gases and particles and the influence of cause of death, age, and season. J Air Waste Manag Assoc. 2002; 52(4):470–84.
16. Schwartz JD, Wang Y, Kloog I, Yitshak-Sade M, Dominici F, Zanobetti A. Estimating the effects of PM2.5 on life expectancy using causal modeling methods. Environ Health Perspect. 2018; 126(12):127002.
17. Samet JM, Dominici F, Curriero FC, Coursac I, Zeger SL. Fine particulate air pollution and mortality in 20 U.S. cities, 1987-1994. N Engl J Med. 2000; 343(24):1742–9.
18. Pope CA 3rd, Burnett RT, Thun MJ, Calle EE, Krewski D, Ito K, et al. Lung cancer, cardiopulmonary mortality, and long-term exposure to fine particulate air pollution. JAMA. 2002; 287(9):1132–41.
19. Pope CA 3rd, Ezzati M, Dockery DW. Fine-particulate air pollution and life expectancy in the United States. N Engl J Med. 2009; 360(4):376–86.
20. Aphekom. Summary report of the Aphekom project 2008–2011. [cited 2022 Oct 17]. Available from: URL: http://aphekom.org/c/document_library/get_file?uuid=5532fafa-921f-4ab1-9ed9-c0148f7da36a&groupId=10347.
21. Newby DE, Mannucci PM, Tell GS, Baccarelli AA, Brook RD, Donaldson K, et al. Expert position paper on air pollution and cardiovascular disease. Eur Heart J. 2015; 36(2):83–93b.
22. Zivin JG, Neidell M. Air pollution’s hidden impacts. Science. 2018; 359(6371):39–40.
23. Loomis D, Grosse Y, Lauby-Secretan B, El Ghissassi F, Bouvard V, Benbrahim-Tallaa L, et al. The carcinogenicity of outdoor air pollution. Lancet Oncol. 2013; 14(13):1262–3.
24. Coogan PF, White LF, Jerrett M, Brook RD, Su JG, Seto E, et al. Air pollution and incidence of hypertension and diabetes mellitus in black women living in Los Angeles. Circulation. 2012; 125(6):767–72.
25. Rajagopalan S, Brook RD. Air pollution and type 2 diabetes: Mechanistic insights. Diabetes. 2012; 61(12):3037–45.
26. O’Connor GT, Neas L, Vaughn B, Kattan M, Mitchell H, Crain EF, et al. Acute respiratory health effects of air pollution on children with asthma in US inner cities. J Allergy Clin Immunol. 2008; 121(5):1133–9.e1.
27. McCreanor J, Cullinan P, Nieuwenhuijsen MJ, Stewart-Evans J, Malliarou E, Jarup L, et al. Respiratory effects of exposure to diesel traffic in persons with asthma. N Engl J Med. 2007; 357(23):2348–58.
28. Liu L, Poon R, Chen L, Frescura AM, Montuschi P, Ciabattoni G, et al. Acute effects of air pollution on pulmonary function, airway inflammation, and oxidative stress in asthmatic children. Environ Health Perspec. 2009; 117(4):668–74.
29. Meng YY, Rull RP, Wilhelm M, Lombardi C, Balmes J, Ritz B. Outdoor air pollution and uncontrolled asthma in the San Joaquin Valley, California. J Epidemiol Community Health. 2010; 64(2):142–7.
30. Silverman RA, Ito K. Age-related association of fine particles and ozone with severe acute asthma in New York city. J Allergy Clin Immunol. 2010; 125(2):367–73.e5.
31. Kyung SY, Kim YS, Kim WJ, Park MS, Song JW, Yum H, et al. Guideline for the prevention and management of particulate matter/Asian dust particle induced adverse health effect on the patients with pulmonary diseases. J Korean Med Assoc. 2015; 58(11):1060–9.
32. Tétreault LF, Doucet M, Gamache P, Fournier M, Brand A, Kosatsky T, et al. Childhood exposure to ambient air pollutants and the onset of asthma: An administrative cohort study in Québec. Environ Health Perspect. 2016; 124(8):1276–82.
33. Zhou C, Baïz N, Zhang T, Banerjee S, Annesi-Maesano I; EDEN Mother-Child Cohort Study Group. Modifiable exposures to air pollutants related to asthma phenotypes in the first year of life in children of the EDEN mother-child cohort study. BMC Public Health. 2013; 13:506.
34. Rhee CS, Wee JH, Ahn JC, Lee WH, Tan KL, Ahn S, et al. Prevalence, risk factors and comorbidities of allergic rhinitis in South Korea: The fifth Korea national health and nutrition examination survey. Am J Rhinol Allergy. 2014; 28(2):e107–14.
35. Seo S, Kim D, Min S, Paul C, Yoo Y, Choung JT. GIS-based association between PM10 and allergic diseases in Seoul: Implications for health and environmental policy. Allergy Asthma Immunol Res. 2016; 8(1):32–40.
36. Yang SI. Particulate matter and childhood allergic diseases. Korean J Pediatr. 2019; 62(1):22–9.
37. Wang J, Lu M, An Z, Jiang J, Li J, Wang Y, et al. Associations between air pollution and outpatient visits for allergic rhinitis in Xinxiang, China. Environ Sci Pollut Res Int. 2020; 27(19):23565–74.
38. Cabrera M, Garzón García B, Moreno Grau S, Subiza J. Association between seasonal allergic rhinitis and air pollution, meteorological factors, and grass pollen counts in Madrid (1996 and 2009). J Investig Allergol Clin Immunol. 2019; 29(5):371–7.
39. Lindgren A, Stroh E, Nihlén U, Montnémery P, Axmon A, Jakobsson K. Traffic exposure associated with allergic asthma and allergic rhinitis in adults. A cross-sectional study in southern Sweden. Int J Health Geogr. 2009; 8:25.
40. Zhang F, Wang W, Lv J, Krafft T, Xu J. Time-series studies on air pollution and daily outpatient visits for allergic rhinitis in Beijing, China. Sci Total Environ. 2011; 409(13):2486–92.
41. Jung DY, Leem JH, Kim HC, Kim JH, Hwang SS, Lee JY, et al. Effect of traffic-related air pollution on allergic disease: Results of the children’s health and environmental research. Allergy Asthma Immunol Res. 2015; 7(4):359–66.
42. Min KD, Yi SJ, Kim HC, Leem JH, Kwon HJ, Hong S, et al. Association between exposure to traffic-related air pollution and pediatric allergic diseases based on modeled air pollution concentrations and traffic measures in Seoul, Korea: A comparative analysis. Environ Health. 2020; 19(1):6.
43. Burte E, Leynaert B, Marcon A, Bousquet J, Benmerad M, Bono R, et al. Long-term air pollution exposure is associated with increased severity of rhinitis in 2 European cohorts. J Allergy Clin Immunol. 2020; 145(3):834–42.e6.
44. Takano H, Yoshikawa T, Ichinose T, Miyabara Y, Imaoka K, Sagai M. Diesel exhaust particles enhance antigen-induced airway inflammation and local cytokine expression in mice. Am J Respir Crit Care Med. 1997; 156(1):36–42.
45. Fernvik E, Scharnweber T, Knopp D, Niessner R, Vargaftig BB, Peltre G. Effects of fractions of traffic particulate matter on TH2- cytokines, IgE levels, and bronchial hyperresponsiveness in mice. J Toxicol Environ Health A. 2002; 65(15):1025–45.
46. Kim JA, Cho JH, Park IH, Shin JM, Lee SA, Lee HM. Diesel exhaust particles upregulate interleukins IL-6 and IL-8 in nasal fibroblasts. PLoS One. 2016; 11(6):e0157058.
47. Wang YL, Gao W, Li Y, Wang YF. Concentration-dependent effects of PM2.5 mass on expressions of adhesion molecules and inflammatory cytokines in nasal mucosa of rats with allergic rhinitis. Eur Arch Otorhinolaryngol. 2017; 274(8):3221–9.
48. Guo ZQ, Dong WY, Xu J, Hong ZC, Zhao RW, Deng CR, et al. T-helper type 1-T-helper type 2 shift and nasal remodeling after fine particulate matter exposure in a rat model of allergic rhinitis. Am J Rhinol Allergy. 2017; 31(3):148–55.
49. Zhou L, Huang Y, Han Z, Wang J, Sun N, Zhang R, et al. Effects of rosmarinic acid on the inflammatory response in allergic rhinitis rat models after PM2.5 exposure. J Clin Lab Anal. 2022; 36(4):e24316.
50. Brandt EB, Biagini Myers JM, Acciani TH, Ryan PH, Sivaprasad U, Ruff B, et al. Exposure to allergen and diesel exhaust particles potentiates secondary allergen-specific memory responses, promoting asthma susceptibility. J Allergy Clin Immunol. 2015; 136(2):295–303.e7.
51. Diaz-Sanchez D, Tsien A, Fleming J, Saxon A. Combined diesel exhaust particulate and ragweed allergen challenge markedly enhances human in vivo nasal ragweed-specific IgE and skews cytokine production to a T helper cell 2-type pattern. J Immunol. 1997; 158(5):2406–13.
52. Wang P, Thevenot P, Saravia J, Ahlert T, Cormier SA. Radicalcontaining particles activate dendritic cells and enhance Th17 inflammation in a mouse model of asthma. Am J Respir Cell Mol Biol. 2011; 45(5):977–83.
53. van Voorhis M, Knopp S, Julliard W, Fechner JH, Zhang X, Schauer JJ, et al. Exposure to atmospheric particulate matter enhances Th17 polarization through the aryl hydrocarbon receptor. PLoS One. 2013; 8(12):e82545.
54. Bae JS, Oh SB, Kim J, Kim H, Kim JH, Kim EH, et al. Particulate matter exposure aggravates IL-17-induced eye and nose inflammation in an OVA/Poly(I:C) mouse model. Allergy Asthma Immunol Res. 2022; 14(1):59–72.
55. Brandt EB, Kovacic MB, Lee GB, Gibson AM, Acciani TH, Le Cras TD, et al. Diesel exhaust particle induction of IL-17A contributes to severe asthma. J Allergy Clin Immunol. 2013; 132(5):1194–204.e2.
56. Jung HJ, Ko YK, Shim WS, Kim HJ, Kim DY, Rhee CS, et al. Diesel exhaust particles increase nasal symptoms and IL-17A in house dust mite-induced allergic mice. Sci Rep. 2021; 11(1):16300.
57. Naclerio R, Ansotegui IJ, Bousquet J, Canonica GW, D’Amato G, Rosario N, et al. International expert consensus on the management of allergic rhinitis (AR) aggravated by air pollutants: Impact of air pollution on patients with AR: Current knowledge and future strategies. World Allergy Organ J. 2020; 13(3):100106.
58. de Kok TM, Driece HA, Hogervorst JG, Briedé JJ. Toxicological assessment of ambient and traffic-related particulate matter: A review of recent studies. Mutat Res. 2006; 613(2-3):103–22.
59. Gilliland FD, Li YF, Saxon A, Diaz-Sanchez D. Effect of glutathione-S-transferase M1 and P1 genotypes on xenobiotic enhancement of allergic responses: Randomised, placebo-controlled crossover study. Lancet. 2004; 363(9403):119–25.
60. Ciencewicki J, Trivedi S, Kleeberger SR. Oxidants and the pathogenesis of lung diseases. J Allergy Clin Immunol. 2008; 122(3):456–68. quiz 469-70.
61. Li N, Xia T, Nel AE. The role of oxidative stress in ambient particulate matter-induced lung diseases and its implications in the toxicity of engineered nanoparticles. Free Radic Biol Med. 2008; 44(9):1689–99.
62. Hong Z, Guo Z, Zhang R, Xu J, Dong W, Zhuang G, et al. Airborne fine particulate matter induces oxidative stress and inflammation in human nasal epithelial cells. Tohoku J Exp Med. 2016; 239(2):117–25.
63. Wang J, Guo Z, Zhang R, Han Z, Huang Y, Deng C, et al. Effects of N-acetylcysteine on oxidative stress and inflammation reactions in a rat model of allergic rhinitis after PM2.5 exposure. Biochem Biophys Res Commun. 2020; 533(3):275–81.
64. Piao CH, Fan Y, Nguyen TV, Shin HS, Kim HT, Song CH, et al. PM2.5 exacerbates oxidative stress and inflammatory response through the Nrf2/NF-κB signaling pathway in OVA-induced allergic rhinitis mouse model. Int J Mol Sci. 2021; 22(15):8173.
65. Steelant B, Farré R, Wawrzyniak P, Belmans J, Dekimpe E, Vanheel H, et al. Impaired barrier function in patients with house dust miteinduced allergic rhinitis is accompanied by decreased occludin and zonula occludens-1 expression. J Allergy Clin Immunol. 2016; 137(4):1043–53.e5.
66. Soyka MB, Wawrzyniak P, Eiwegger T, Holzmann D, Treis A, Wanke K, et al. Defective epithelial barrier in chronic rhinosinusitis: The regulation of tight junctions by IFN-γ and IL-4. J Allergy Clin Immunol. 2012; 130(5):1087–96.e10.
67. Park SK, Yeon SH, Choi MR, Choi SH, Lee SB, Rha KS, et al. Urban particulate matters may affect endoplasmic reticulum stress and tight junction disruption in nasal epithelial cells. Am J Rhinol Allergy. 2021; 35(6):817–29.
68. Fukuoka A, Matsushita K, Morikawa T, Takano H, Yoshimoto T. Diesel exhaust particles exacerbate allergic rhinitis in mice by disrupting the nasal epithelial barrier. Clin Exp Allergy. 2016; 46(1):142–52.
69. Xian M, Ma S, Wang K, Lou H, Wang Y, Zhang L, et al. Particulate matter 2.5 causes deficiency in barrier integrity in human nasal epithelial cells. Allergy Asthma Immunol Res. 2020; 12(1):56–71.
70. Gowers AM, Cullinan P, Ayres JG, Anderson HR, Strachan DP, Holgate ST, et al. Does outdoor air pollution induce new cases of asthma? Biological plausibility and evidence; a review. Respirology. 2012; 17(6):887–98.
71. Pénard-Morand C, Raherison C, Charpin D, Kopferschmitt C, Lavaud F, Caillaud D, et al. Long-term exposure to close-proximity air pollution and asthma and allergies in urban children. Eur Respir J. 2010; 36(1):33–40.
72. Morgenstern V, Zutavern A, Cyrys J, Brockow I, Koletzko S, Krämer U, et al. Atopic diseases, allergic sensitization, and exposure to traffic-related air pollution in children. Am J Respir Crit Care Med. 2008; 177(12):1331–7.
73. Bowatte G, Lodge C, Lowe AJ, Erbas B, Perret J, Abramson MJ, et al. The influence of childhood traffic-related air pollution exposure on asthma, allergy and sensitization: A systematic review and a meta-analysis of birth cohort studies. Allergy. 2015; 70(3):245–56.
74. Annesi-Maesano I, Agabiti N, Pistelli R, Couilliot MF, Forastiere F. Subpopulations at increased risk of adverse health outcomes from air pollution. Eur Respir J Suppl. 2003; 40:57s–63s.
75. Dong GH, Qian Z, Liu MM, Wang D, Ren WH, Fu Q, et al. Obesity enhanced respiratory health effects of ambient air pollution in Chinese children: The Seven Northeastern Cities study. Int J Obes (Lond). 2013; 37(1):94–100.
76. Li Y, Zhou J, Rui X, Zhou L, Mo X. PM2.5 exposure exacerbates allergic rhinitis in mice by increasing DNA methylation in the IFN-γ gene promoter in CD4+T cells via the ERK-DNMT pathway. Toxicol Lett. 2019; 301:98–107.
77. Sofer T, Baccarelli A, Cantone L, Coull B, Maity A, Lin X, et al. Exposure to airborne particulate matter is associated with methylation pattern in the asthma pathway. Epigenomics. 2013; 5(2):147–54.
78. Baccarelli A, Wright RO, Bollati V, Tarantini L, Litonjua AA, Suh HH, et al. Rapid DNA methylation changes after exposure to traffic particles. Am J Respir Crit Care Med. 2009; 179(7):572–8.
79. Perera F, Tang WY, Herbstman J, Tang D, Levin L, Miller R, et al. Relation of DNA methylation of 5’-CpG island of ACSL3 to transplacental exposure to airborne polycyclic aromatic hydrocarbons and childhood asthma. PLoS One. 2009; 4(2):e4488.
80. Madrigano J, Baccarelli A, Mittleman MA, Wright RO, Sparrow D, Vokonas PS, et al. Prolonged exposure to particulate pollution, genes associated with glutathione pathways, and DNA methylation in a cohort of older men. Environ Health Perspect. 2011; 119(7):977–82.
81. Brunst KJ, Leung YK, Ryan PH, Khurana Hershey GK, Levin L, Ji H, et al. Forkhead box protein 3 (FOXP3) hypermethylation is associated with diesel exhaust exposure and risk for childhood asthma. J Allergy Clin Immunol. 2013; 131(2):592–4.e1-3.
82. Kohli A, Garcia MA, Miller RL, Maher C, Humblet O, Hammond SK, et al. Secondhand smoke in combination with ambient air pollution exposure is associated with increasedx CpG methylation and decreased expression of IFN-γ in T effector cells and Foxp3 in T regulatory cells in children. Clin Epigenetics. 2012; 4(1):17.
Fig. 1.
Possible Th17-related immunological responses. A: Total symptom score. B: Differential counts in BALF. Significantly increased proportion of neutrophils in the BALF is seen in the HDM and DEP co-exposed group. C: Real-time PCR analysis of cytokines expression in the nasal mucosa. The transcriptional levels of IL-17A were increased in the HDM and DEP co-exposed group. Data are expressed as mean±standard error mean. *p<0.05; **p<0.01; ***p<0.001. BALF, bronchoalveolar lavage fluid; HDM, house dust mite; DEP, diesel exhaust particle; PCR, polymerase chain reaction; PBS, phosphate-buffered saline. Adapted from Jung et al. Sci Rep 2021;11(1):16300[56].
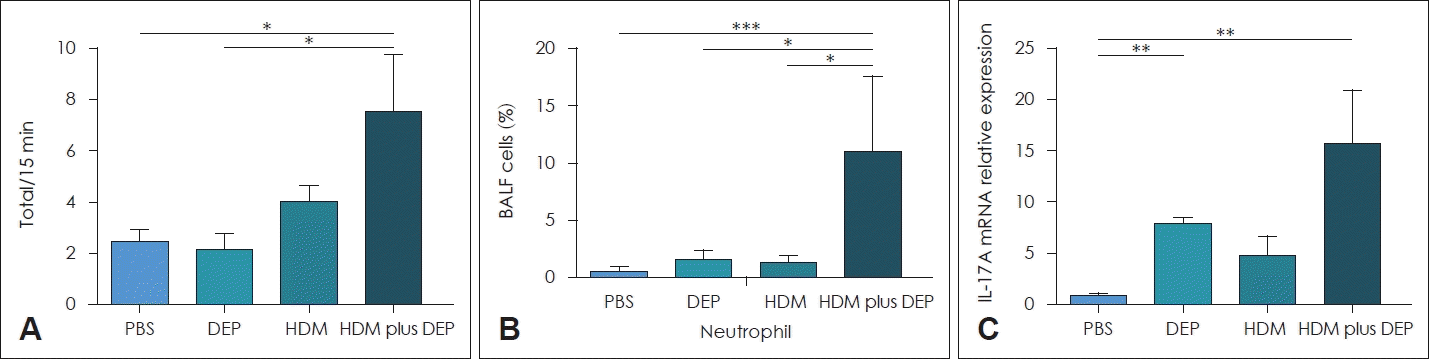
Fig. 2.
Effects of particulate matter on allergic rhinitis and potential mechanisms. HDM, house dust mite; PM, particulate matter; DEPs, diesel exhaust particles; PAHs, polycyclic aromatic hydrocarbons.
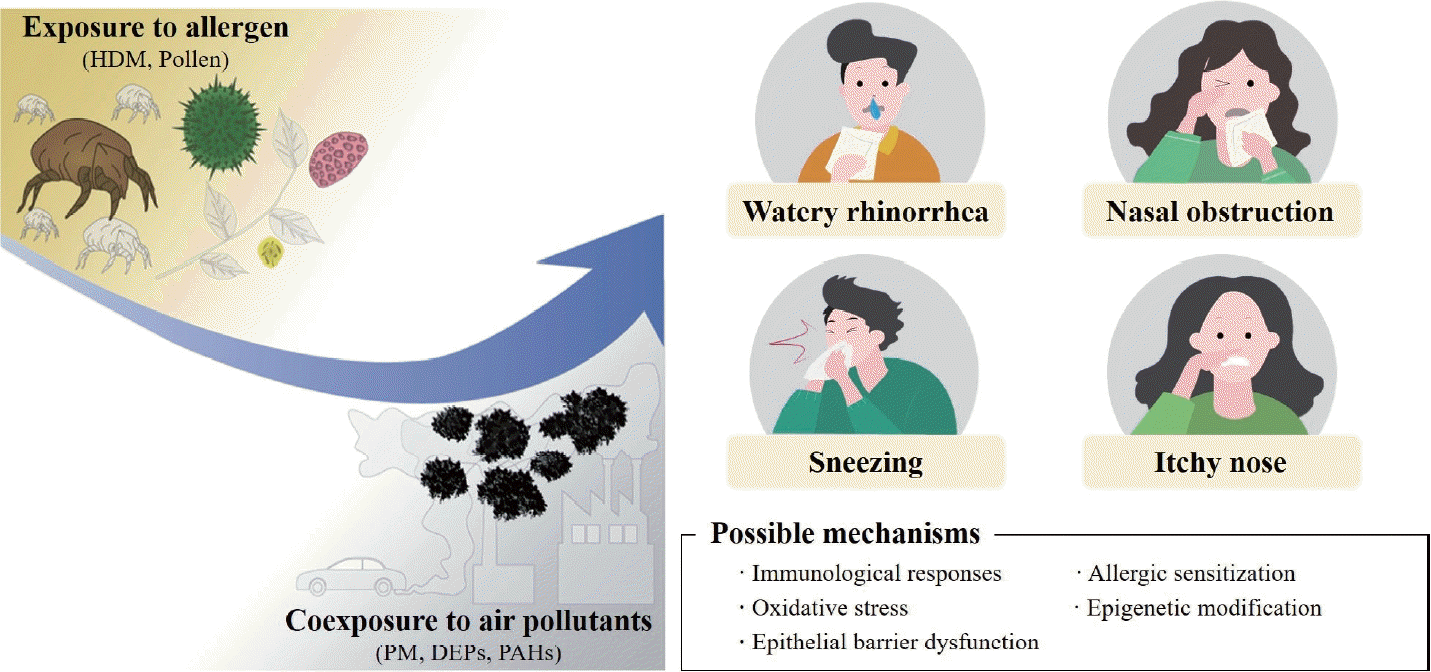
Table 1.
Experimental studies on the impact of PM on allergic rhinitis
Suggested possible mechanisms | Authors, year | Subjects (animal, tissue) | Exposure | Outcome measure | Main results |
---|---|---|---|---|---|
Immunological response | Kim, et al., [46] 2016 | Nasal fibroblast | DEP | Cytokine and chemokine array (levels of IL-6, IL-8), mRNA and protein expression levels of IL-6, IL-8, Signaling pathways of p-38, Akt, NF-κB | Increased expressions of IL-6 and IL-8 in nasal fibroblasts at mRNA and protein levels, induced phosphorylation of p38, Akt, and NF-κB |
Wang, et al., [47] 2017 | SD rats sensitized to OVA | PM2.5 | Nasal symptoms, serum IgE, ICAM-1, VCAM-1 expressions, eosinophil infiltration, expression of IFN-γ, IL-4, IL-5, IL-33, TSLP | Greater numbers of WBCs, increased IgE level, elevated levels of ICAM-1, VCAM-1, EOS, IFN-γ, IL-4, IL-5, IL-33, and TSLP | |
Guo, et al., [48] 2017 | SD rats sensitized to OVA | PM2.5 | Nasal symptoms, levels of Th1-Th2 cytokines, degree of eosinophilia in nasal lavage fluid, mRNA expressions of transcription factors GATA-3 and T-bet in the nasal mucosa, hyperplasia of goblet cells | Th1-Th2 shift and nasal remodeling, increased number of sneezes and nasal rubs, decreased IFN-γ, increased IL-4 and IL-13 expressions as well as the number of eosinophils in nasal lavage fluid, upregulated mRNA expression of GATA-3 in the nasal mucosa, downregulated T-bet | |
Jung, et al., [56] 2021 | BALB/c mice sensitized to HDM | DEP | Nasal symptoms, serum total and HDM-specific Igs, eosinophil infiltration in the nasal mucosa, cytological profiles in BALF, cytokine levels in the nasal mucosa and spleen cell culture | Increased allergic symptom scores, reduced total IgE and HDM-specific IgE and IgG1 levels, decreased eosinophil infiltration in the nasal mucosa, increased proportion of neutrophils in BALF, increased IL-17A level in the nasal mucosa | |
Bae, et al., [54] 2022 | BALB/c mice sensitized to OVA/Poly (I:C) | TiO2 particle | Nasal symptoms, inflammatory parameters (IL-4, IL-17, IFN-γ) | Increased nasal rubbing and sneezing counts, increased infiltration of neutrophils and IL-17A+cells in the nasal mucosa | |
Zhou, et al., [49] 2022 | SD rats sensitized to OVA | PM2.5 | Nasal symptoms, expression of IL-4, IL-13, INF-γ, OVA-sIgE, histopathological changes in nasal mucosa | Worsened allergic nasal symptoms, histopathologically, aggravated disorganized nasal mucosal epithelium, cell exfoliation, eosinophilic infiltration of lamina propria, gland swelling, and submucosal vascular congestion, increased IL-4, IL-13, decreased level of IFN-γ | |
Oxidative stress | Hong, et al., [62] 2016 | Nasal epithelial cells | PM2.5 | Cell morphology, intracellular levels of ROS, intracellular superoxide dismutase, catalase, glutathione peroxidase activities, mRNA levels of GM-CSF, TNF-α, IL-13, eotaxin, IL-6, IL-8, Nrf2, Histone H1, β-actin | Decreased the viability of RPMI 2650 cells, increased the levels of intracellular ROS and the nuclear translocation of Nrf2, decreased the activities of superoxide dismutase, catalase and glutathione peroxidase, increased the production of GM-CSF, TNF-α, IL-13 and eotaxin |
Wang, et al., [63] 2020 | SD rats sensitized to OVA | PM2.5 | Nasal symptoms, Redox level in nasal mucosa, Th1/Th2-related serum cytokines, nasal mucosal histopathology | Aggravated Th2-dominant allergic inflammation, redox imbalance, accompanied by nasal epithelial cell stripping and eosinophil infiltration | |
Piao, et al., [64] 2021 | BALB/c mice sensitized to OVA | PM2.5 | Oxidative stress and inflammatory response via the Nrf2/NF-κB signaling pathway, histological, serum, and protein analyses | Increased expression of inflammatory cytokine and reduced release of Treg cytokine, induced oxidative through malondialdehyde production, inhibited activity of the Nrf2 signaling pathway, enhanced PM2.5 phagocytosis by alveolar macrophages via activating the NF-κB signaling pathway | |
Epithelial barrier dysfunction | Fukuoka, et al., [68] 2016 | BALB/c mice sensitized to ragweed pollen | DEP | Frequency of sneezing, expression of a tight junction protein, zonula occludens-1, examination of paracellular permeability in RPMI 2650 cells | Increased frequency of sneezing, reduced ZO-1 expression in RPMI 2650 cells, disrupted nasal mucosal tight junctions in vivo |
Xian, et al., [69] 2020 | Nasal epithelial cells | PM2.5 | Transepithelial resistance, permeability of FITC, expression of tight junction, proinflammatory cytokines | Decreased transepithelial resistance, increased FITC permeability, decreased expression of claudin-1 mRNA, claudin-1, occludin and ZO-1 protein, increased production of the cytokines IL-8, TIMP-1 and TSLP | |
Park, et al., [67] 2021 | Nasal epithelial cells | Urban PM | Tight junction and endoplasmic reticulum stress marker expression, tight junction integrity and nasal epithelial barrier function by transepithelial electric resistance and paracellular flux | Impaired nasal epithelial barrier, by decreased protein expression of tight junction and endoplasmic reticulum stress markers in human nasal epithelial cells. reduced transepithelial electrical resistance, increased FITC-dextran permeability | |
DNA methylation | Li, et al., [76] 2019 | BALB/c mice sensitized to OVA | PM2.5 | Nasal symptoms, pathological changes, DNA methylation level of the IFN-γ gene promoter and activation of the ERK-DNMT pathway | Severe rhinitis symptoms, decreased percentage of Th1 T cells, increased DNA methylation of IFN-γ gene promoter in CD4+T cells, increased activation of ERK-DNMT pathway in CD4+ T cells |