Abstract
Objective
The purpose of this study was to determine the efficacy of a 3D-printed aneurysm simulation model (3DPM) in educating patients and improving physicians’ comprehension and performance.
Methods
This prospective study involved 40 patients who were diagnosed with unruptured intracranial aneurysms (UIAs) and scheduled for surgical clipping or endovascular coiling and randomly divided into two groups (the 3DPM group and the non-3DPM group). The 3DPM was used in preoperative consultation with patients and intraoperatively referenced by surgeons. The patients, 7 neurosurgical residents, and 10 surgeons completed questionnaires (5-point Likert scale) to determine the usefulness of the 3DPM.
Results
Patients in the 3DPM group had significantly higher scores in terms of their understanding of the disease (mean 4.85 vs. 3.95, p<0.001) and the treatment plan (mean 4.85 vs. 4.20, p=0.005) and reported higher satisfaction during consultation (5.0 vs. 4.60, p=0.036) than patients in the non-3DPM group. During patient consultation, 3DPMs were most useful in improving doctor–patient communication (mean 4.57, range 4-5). During clipping surgery, the models were most useful in assessing adjacent arteries (mean 4.9, range 4-5); during endovascular coiling, they were especially helpful in microcatheter shaping (mean 4.7, range 4-5).
Unruptured intracranial aneurysms (UIAs) are frequently observed in the neurosurgical department, with a prevalence of approximately 3% in the general population [9,23]. Since the rupture of UIAs is well recognized as a cause of subarachnoid hemorrhage or intracerebral hemorrhage, leading to fatal neurological aftereffects, it is currently treated with either surgical clipping or endovascular coiling [2,8].
The location, direction, shape, and size of the aneurysm as well as its relationship with adjacent arteries are important considerations in preoperative planning, meaning that three-dimensional (3D) comprehension of the target aneurysm is indispensable [13,16]. Although it has recently become simple and fast to reconstruct 3D images due to the development of imaging techniques, the ability to comprehend the actual anatomy and characteristics of aneurysms on a two-dimensional computer screen is limited [1,6,16]. In addition, a large percentage of patients are elderly, which makes it difficult to understand the disease and treatment plan based on digital images. Therefore, 3D printing technology has recently been used in many medical fields, including medication, surgical planning and instrumentation, and in the education of patients and neurosurgical trainees to improve performance and eliminate the limitations of digital imaging [5,13,14,16,21].
For the current report, we conducted a study using questionnaires to validate our assumption that a patient-specific 3D-printed aneurysm simulation model (3DPM) of the target aneurysm can improve the patient’s understanding of the condition and improve the understanding of both the surgical trainees and neurosurgeons, thus resulting in better performance and understanding.
This prospective study included 40 consecutive patients (20 with clipping surgery and 20 with endovascular coiling) who were diagnosed with UIAs and scheduled for surgical clipping or endovascular coiling between September 2021 and November 2021. Enrolled patients were randomly divided into two groups before admission: 20 patients in the 3D printing group (3DPM group) and 20 patients in the non-3D-printing group (non-3DPM group). The data were collected, including patient medical records (demographics, medical history and education), aneurysm geometry (location, aneurysm number, aneurysm size) and planned procedure (clipping or coiling).
In addition, 7 neurosurgical residents and 10 neurosurgeons (the surgeons performing the procedures, along with their surgical assistants) were required to complete the questionnaire to evaluate the efficacy of 3DPM.
The study was approved by the institutional review board of our institute (2021-08-040), and we obtained informed consent from all the enrolled patients.
The patient-specific 3DPM was made before hospitalization. Images were exported in standard digital imaging format from preoperative digital subtraction angiography (DSA) or magnetic resonance angiography (MRA) to the Mimics medical image processing software (20.0, Materialise, Leuven, Belgium). The field of interest was segmented and converted into the standard triangulated language file. The printing was started after the design by using an SLA 3D printer (Form2, Formlabs, Somerville, US). Then, the researcher manually removed the thin pillars supporting the 3DPM, completing the manufacturing process (Fig. 1).
In the 3DPM group, the patient-specific 3DPM was used when explaining the disease and procedure and when obtaining informed consent the day before the procedure. In the non-3DPM group, preoperative DSA or MRA was used rather than 3DPM for explaining the procedure. In both groups, aneurysm character, neurovascular anatomy, disease prognosis, complication and outcome of each planned procedure was described. Then, the patients enrolled in both groups completed the questionnaire to evaluate their level of comprehension and satisfaction with the explanation and efficacy of 3DPM. The questionnaire consisted of questions rated on a 5-point Likert scale.
After the explanations using 3DPM, a questionnaire was completed by the neurosurgical residents and evaluated to determine the usefulness of 3DPM in resident training and patient–doctor communications.
The 3DPM was shown to neurosurgeons during clipping surgery or endovascular coiling of enrolled patients. During the procedures, various situations, such as patient positioning, surgical approach, clip application, microcatheter shaping or selection into the aneurysm, and coiling, were referred to. Instead of referring to the DSA image, the 3DPM was intermittently displayed with the same surgical view in the operative field whenever the operator wanted it. A questionnaire was also completed by both the surgeon and the assistant after the procedure to evaluate the efficacy of 3DPM during the whole process.
In addition, the process and cost of making the 3DPM were disclosed, and all the included patients and medical personnel questioned the expected cost of the 3DPM.
All statistical analyses were performed using SPSS version 18.0 (SPSS, Chicago, Illinois, US). Variables are expressed as the mean±standard deviation, mean (range) or number with percentage. Student’s t test or the Mann–Whitney U test were used to compare continuous variables and the scores of the questionnaire between two groups, while the chi-square test was used to compare categorical variables. A p value <0.05 was considered statistically significant.
The average duration of the 3D printing process was 5 hours and 9 minutes, consisting of 224 minutes for the printing process, 65 minutes for the washing and drying process, and 20 minutes for the manual cutting process. The average cost of the resin used for the process was 2.98 US dollars (USD) per case.
The baseline characteristics of the patients are summarized in Table 1. Each group consisted of 10 patients who underwent clipping surgery and 10 who underwent endovascular coiling. Points of the questionnaire from patients after preoperative consultations are shown in Table 2. The 3DPM group had higher points in understanding of the disease (question no. 1, mean 4.85 vs. 3.95, p=0.001) and understanding of the treatment plan (question no. 2, mean 4.85 vs. 4.20, p=0.005) and revealed higher satisfaction during the consultation (question no. 8, mean 5.0 vs. 4.60, p=0.036) than the non-3DPM group. All the patients in group A reported that the 3DPM was very helpful in understanding the shape, location and overall aspect of the disease. Patients in the non-3DPM group reported that the patient-specific 3DPM service would help them to better understand the characteristics of the aneurysm and the treatment plan (mean 4.60, range 2-5). All the patients in both groups expected that the 3DPM might increase the accuracy and safety of the surgery or endovascular process (mean 4.95 and 4.80, respectively).
Questionnaire results from neurosurgical residents are shown in Table 3. The 3DPM was especially helpful in understanding the shape (mean 4.14, range 3-5), direction (mean 4.43, range 3-5) and parent artery (mean 4.14, range 3-5) of the aneurysm. The model mostly helped to improve doctor–patient communication (mean 4.57, range 4-5).
Surgeons and surgical assistants participated in the actual procedures of both surgical clipping and endovascular coiling using 3DPM, and their questionnaire results are shown in Tables 4 and 5. During surgical clipping, the 3DPM was most useful in understanding the direction (mean 4.1, range 4-5) and adjacent arteries (mean 4.9, range 4-5) of the target aneurysm. In the coiling procedure, the 3DPM was mostly helpful in shaping (mean 4.7, range 4-5) and inserting (mean 4.5, range 4-5) the microcatheter into the aneurysm. In both the clipping and coiling procedures, surgeons reported that the 3DPM is clinically applicable (mean 4.6 and 4.4, respectively).
The expected appropriate cost of the 3DPM reported in each group is shown in Table 6.
3D printing technology is an essential technology in the neurosurgical field, not only in the cerebrovascular field but also in all other specialties, such as trauma, tumor and spine surgeries [4,7,10,12,15,19,20,22]. Our 3DPM showed direct visualization and great correlation with the actual anatomy of the patient’s vasculature, which was actually helpful intraoperatively. In addition to helping neurosurgeons’ anatomical comprehension, it is also useful in doctor–patient communication and training young neurosurgeons, which is shown in the results.
According to the surgeons, during surgical clipping, the 3DPM was very helpful in determining the exact correlation between the aneurysm and the parent artery or adjacent arteries, especially when adhesion was present between them. In a patient in the 3DPM group, we accurately demonstrated a large right middle cerebral artery (MCA) bifurcation aneurysm and suspected adhesion with the anterior temporal branch of the MCA and inferior trunk of the M2 segment, which was actually well matched and helpful during the clipping procedure (Fig. 2). Adhesion between the aneurysm and adjacent structures is one of the most important considerations in clipping surgery; and by considering the possible presence of adhesion, the surgeon lowers the risk of hemorrhagic and ischemic complications due to aneurysm rupture, kinking or traumatic tearing of near arteries [14,22,24].
The 3DPM gave a more precise understanding of the direction, size, and width of parent arteries, aneurysms, and their correlations during the coiling procedure, leading to easier selection, shaping and insertion of the microcatheter into the aneurysm, which is reflected in the results of the questionnaires completed by the surgeons who performed coiling. Microcatheter shaping is one of the most important factors for successful coiling procedures. However, one possible limitation during the procedure is the difficulty of imagining the exact 3D structure, direction, and depth of the target vessel and aneurysm using only the images on a two-dimensional computer screen [11]. Several researchers have presented the possibility of using the 3DPM for accurate microcatheter design during coiling procedures [11,18]. The planning and design of the microcatheter shape can be performed preoperatively by referring to 3DPM, and it was actually successfully matched intraoperatively. In most cases, the 3DPM was effective during the surgery because it improved the operator’s understanding of the morphology of aneurysms and parent arteries. However, as shown in the results, both surgical methods had low scores on the questionnaire regarding surgical skill improvement by 3DPM. Because surgical skill is improved with the surgeon’s experiences and trainings rather than the understanding of the aneurysm’s morphology, the results are presumed to reflect it.
During preoperative consultation, patients showed great interest in the simulation model, were more satisfied with its application and communication. Actually, the level of understanding of the disease and the treatment plan was statistically significantly higher in patients in the 3DPM group than in those in the non-3DPM group. Kim et al. presented a questionnaire study of 20 patients who observed the 3DPM while completing the informed consent form, and showed that the model was useful in preoperative patient consultation and doctor–patient communication [13]. The study showed a remarkable improvement in the level of understanding, which was larger than that in our present study. The cause of this difference is not definite, but it may be due to the difference in information delivery, method and communication skills of different participants at another institute. However, both studies showed the same result, that the 3DPM is fully applicable in preoperative patient education and consultation.
In particular, when dividing the enrolled patients into younger (age ≤60 years, 8 patients in the 3DPM group and 6 in the non-3DPM group) and older (age >60 years) groups, older patients had statistically significant improvement in understanding the disease (question no. 1) and the treatment plan (question no. 2) when younger patients did not (Table 7). Given that a large percentage of patients diagnosed with UIAs are elderly and because young patients tend to better understand information presented on a computer screen, the 3DPM is likely to seem more appropriate and useful for older patients.
Additionally, in the current study, the expected cost of the 3DPM was determined through questionnaires administered to surgeons, trainees, and patients. The process and cost of making each 3DPM were reported to them before they completed the questionnaires. The average expected cost per 3DPM was 36 USD for patients and 42 USD for surgeons and trainees. Although this cost may not be the exact cost of the 3DPM, it is truly meaningful that patients are willing to pay for the service, which can be an important reference for hospitals in terms of medical charge when the service is commercialized.
To the best of our knowledge, the present study is the largest prospective questionnaire study, involving 40 patients, 7 neurosurgical residents, and 10 surgeons and assistants, that determined the efficacy of 3DPMs in different groups of patients undergoing neurosurgery. In our results, the 3DPM is applicable and useful for patient education and the physician’s understanding of surgical clipping and endovascular coiling of UIAs in both the preoperative and intraoperative periods.
Our study has several limitations. As our study models captured only the vasculature of the target aneurysm, they did not reflect the adjacent brain tissue or skeletal anatomy. Other researchers have described the use of a 3D-printed skull and vascular models in the preoperative planning and simulation of an operation, which may be more helpful in training young surgeons and residents [3,8,12,14,17,19,22].
3D printing technology transcends the limitations of two-dimensional computer screens, and it is fully applicable in patient education and physician simulations for surgical clipping or endovascular coiling of UIAs; the models are useful in both the preoperative and intraoperative periods. Based on the results of our study, it is expected that patient-specific 3DPMs will be increasingly used, enabling significant advances in patients’ and physicians’ understanding and treatment of UIAs.
ACKNOWLEDGMENTS
This work was supported by a National Research Foundation of Korea (NRF) grant funded by the Korean government (MSIT) (No. 2020R1G1A1013289).
REFERENCES
1. Błaszczyk M, Jabbar R, Szmyd B, Radek M. 3D printing of rapid, low-cost and patient-specific models of brain vasculature for use in preoperative planning in clipping of intracranial aneurysms. J Clin Med. 2021; Mar. 10(6):1201.
2. Brown RD, Broderick JP. Unruptured intracranial aneurysms: epidemiology, natural history, management options, and familial screening. Lancet Neurol. 2014; Apr. 13(4):393–404.
3. Chawla S, Devi S, Calvachi P, Gormley WB, Rueda-Esteban R. Evaluation of simulation models in neurosurgical training according to face, content, and construct validity: a systematic review. Acta Neurochir (Wien). 2022; Apr. 164(4):947–66.
4. Chen C, Cai L, Zheng W, Wang J, Guo X, Chen H. The efficacy of using 3D printing models in the treatment of fractures: a randomised clinical trial. BMC Musculoskelet Disord. 2019; Feb. 20(1):65.
5. Cogswell PM, Rischall MA, Alexander AE, Dickens HJ, Lanzino G, Morris JM. Intracranial vasculature 3D printing: review of techniques and manufacturing processes to inform clinical practice. 3D Print Med. 2020; Aug. 6(1):18.
6. Damon A, Clifton W, Valero-Moreno F, Quinones-Hinojosa A. Cost-effective method for 3-dimensional printing dynamic multiobject and patient-specific brain tumor models: technical note. World Neurosurg. 2020; Aug. 140:173–9.
7. Dho Y-S, Lee D, Ha T, Ji SY, Kim KM, Kang H, et al. Clinical application of patient-specific 3D printing brain tumor model production system for neurosurgery. Sci Rep. 2021; Mar. 11(1):7005.
8. Faraj MK, Hoz SS, Mohammad AJ. The use of three-dimensional anatomical patient-specific printed models in surgical clipping of intracranial aneurysm: a pilot study. Surg Neurol Int. 2020; Nov. 11:381.
9. Gabriel RA, Kim H, Sidney S, McCulloch CE, Singh V, Johnston SC, et al. Ten-year detection rate of brain arteriovenous malformations in a large, multiethnic, defined population. Stroke. 2010; Jan. 41(1):21–6.
10. Heinrich MA, Bansal R, Lammers T, Zhang YS, Michel Schiffelers R, Prakash J. 3D-bioprinted mini-brain: a glioblastoma model to study cellular interactions and therapeutics. Adv Mater. 2019; Apr. 31(14):e1806590.
11. Ishibashi T, Takao H, Suzuki T, Yuki I, Kaku S, Kan I, et al. Tailor-made shaping of microcatheters using three-dimensional printed vessel models for endovascular coil embolization. Comput Biol Med. 2016; Oct. 77:59–63.
12. Janes D, Boone D, Dubrowski A. “It’s only brain surgery”: using 3D printing and simulation to prepare rural physicians for the management of acute epidural hematoma. Cureus. 2020; Oct. 12(10):e11236.
13. Kim PS, Choi CH, Han IH, Lee JH, Choi HJ, Lee JI. Obtaining informed consent using patient specific 3D printing cerebral aneurysm model. J Korean Neurosurg Soc. 2019; Jul. 62(4):398–404.
14. Kimura T, Morita A, Nishimura K, Aiyama H, Itoh H, Fukaya S, et al. Simulation of and training for cerebral aneurysm clipping with 3-dimensional models. Neurosurgery. 2009; Oct. 65(4):719–25. discussion 725.
15. Kosterhon M, Neufurth M, Neulen A, Schäfer L, Conrad J, Kantelhardt SR, et al. Multicolor 3D printing of complex intracranial tumors in neurosurgery. J Vis Exp. 2020; Jan. (155).
16. Mashiko T, Otani K, Kawano R, Konno T, Kaneko N, Ito Y, et al. Development of three-dimensional hollow elastic model for cerebral aneurysm clipping simulation enabling rapid and low cost prototyping. World Neurosurg. 2015; Mar. 83(3):351–61.
17. Nagassa RG, McMenamin PG, Adams JW, Quayle MR, Rosenfeld JV. Advanced 3D printed model of middle cerebral artery aneurysms for neurosurgery simulation. 3D Print Med. 2019; Aug. 5(1):11.
18. Namba K, Higaki A, Kaneko N, Mashiko T, Nemoto S, Watanabe E. Microcatheter shaping for intracranial aneurysm coiling using the 3-dimensional printing rapid prototyping technology: preliminary result in the first 10 consecutive cases. World Neurosurg. 2015; Jul. 84(1):178–86.
19. Ploch CC, Mansi CSSA, Jayamohan J, Kuhl E. Using 3D printing to create personalized brain models for neurosurgical training and preoperative planning. World Neurosurg. 2016; Jun. 90:668–74.
20. Randazzo M, Pisapia JM, Singh N, Thawani JP. 3D printing in neurosurgery: a systematic review. Surg Neurol Int. 2016; Nov. 7(Suppl 33):S801–9.
21. Rengier F, Mehndiratta A, von Tengg-Kobligk H, Zechmann CM, Unterhinninghofen R, Kauczor H-U, et al. 3D printing based on imaging data: review of medical applications. Int J Comput Assist Radiol Surg. 2010; Jul. 5(4):335–41.
22. Ryan JR, Almefty KK, Nakaji P, Frakes DH. Cerebral aneurysm clipping surgery simulation using patient-specific 3D printing and silicone casting. World Neurosurg. 2016; Apr. 88:175–81.
23. Vlak MH, Algra A, Brandenburg R, Rinkel GJ. Prevalence of unruptured intracranial aneurysms, with emphasis on sex, age, comorbidity, country, and time period: a systematic review and meta-analysis. Lancet Neurol. 2011; Jul. 10(7):626–36.
24. Wang L, Ye X, Hao Q, Ma L, Chen X, Wang H, et al. Three-dimensional intracranial middle cerebral artery aneurysm models for aneurysm surgery and training. J Clin Neurosci. 2018; Apr. 50:77–82.
Fig. 1.
(A) 3D reconstructed image using preoperative DSA of a patient with multiple aneurysms at the superior hypophyseal branch and bifurcation of the left internal carotid artery. (B) The preoperative images were converted into standard triangulated language files. (C) The patient’s 3DPM before manual work. (D) Final 3DPM after manual work to remove the columns attached to the model. DSA, digital subtraction angiography; 3DPM, 3D-printed aneurysm simulation model
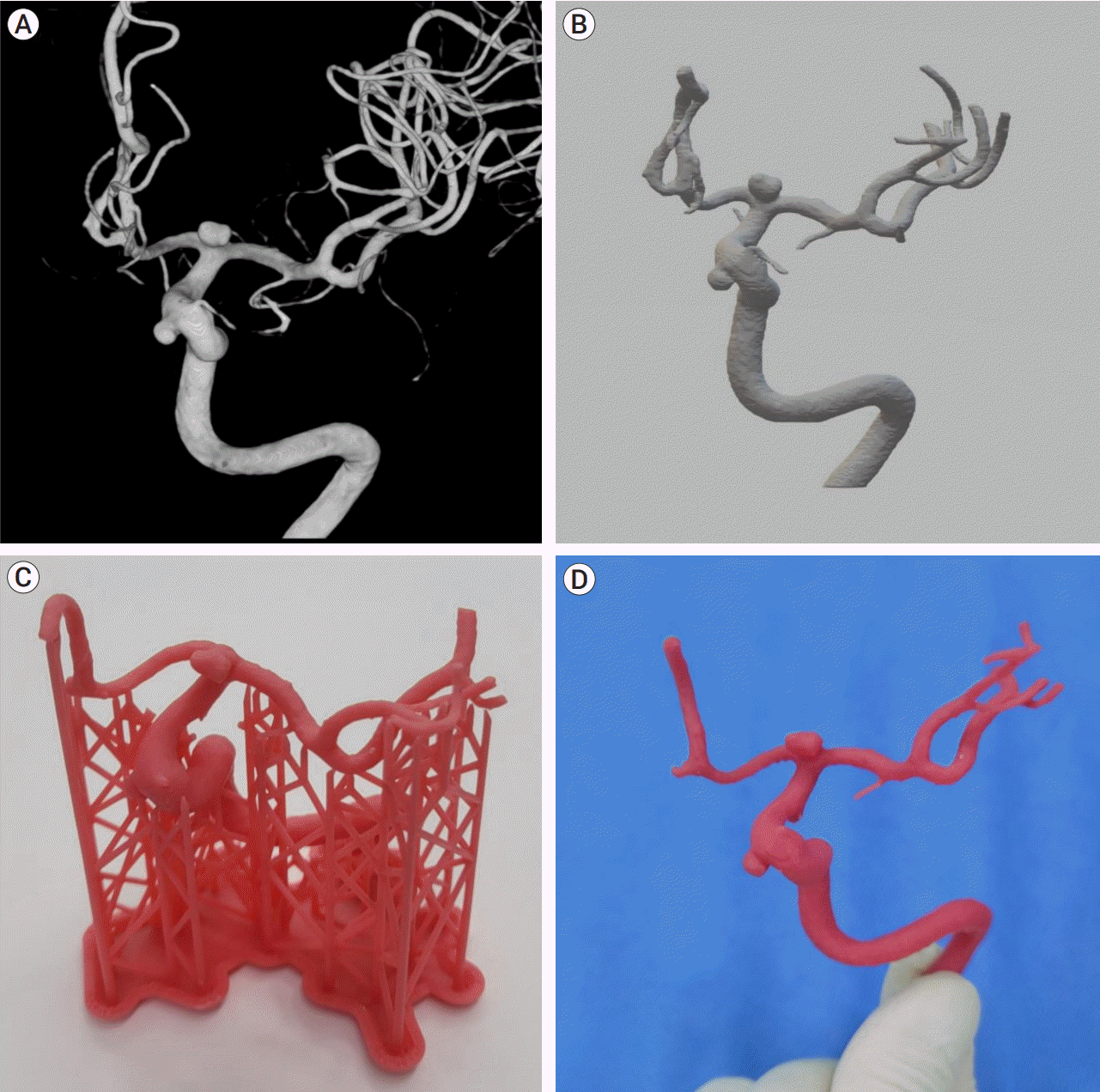
Fig. 2.
The 3DPM of a patient with a right MCA bifurcation aneurysm (A), showing a strong correlation with intraoperative microscopic findings (B). Adhesion to the inferior trunk of the M2 segment (black arrow) and the anterior temporal branch of the MCA (white arrow). 3DPM, 3D-printed aneurysm simulation model; MCA, middle cerebral artery
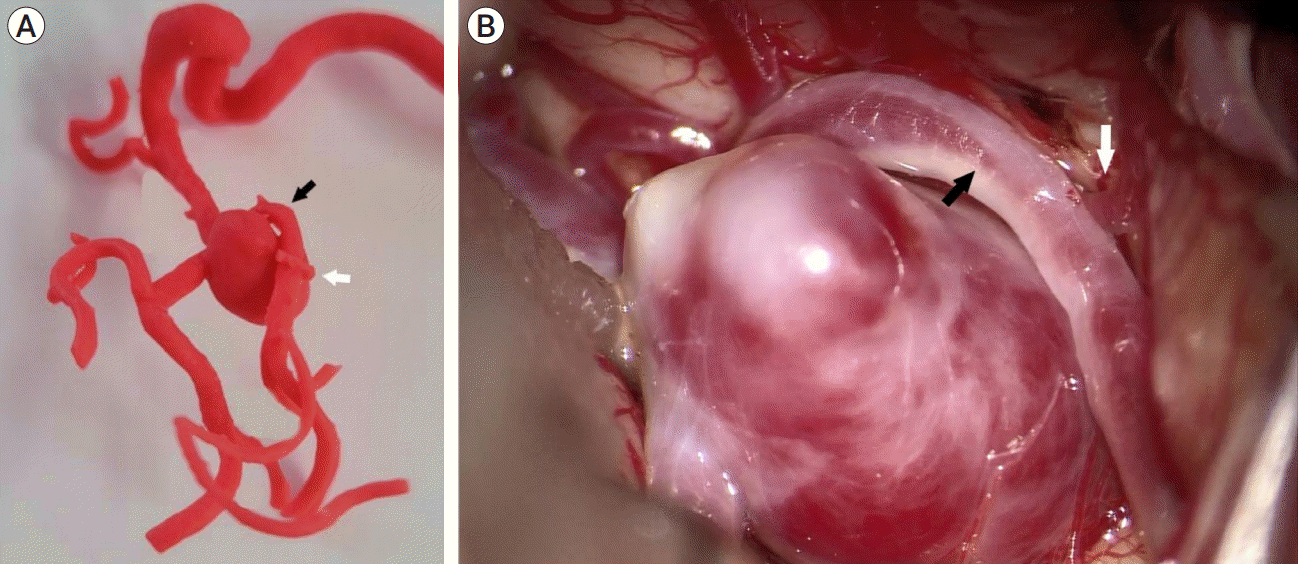
Table 1.
Baseline characteristics of the enrolled patients
Table 2.
Questionnaire filled out by patients after preoperative consultation
Table 3.
Result of the questionnaire filled out by neurosurgical residents
Table 4.
Result of the questionnaire filled out by operators of coiling procedure
Table 5.
Result of the questionnaire filled out by operators of clipping surgery
Table 6.
Results of the questionnaires about the price of the service
No. | Question |
Mean price |
|
---|---|---|---|
Patients | Physicians | ||
1 | How much do you think is the appropriate fee for the 3D printing service? | 44,000 KRW, 36 USD | 50,000 KRW, 42 USD |
Table 7.
Differences in scores of understanding between patients over 60 and under