Abstract
The pathological hallmark of rheumatoid arthritis (RA) is a synovial pannus that comprises proliferating and invasive fibroblast-like synoviocytes, infiltrating inflammatory cells, and an associated neoangiogenic response. Animal models have been established to study these pathological features of human RA. Spontaneous and induced animal models of RA primarily reflect inflammatory aspects of the disease. Among various induced animal models, collagen-induced arthritis (CIA) and collagen antibody-induced arthritis (CAIA) models are widely used to study the pathogenesis of RA. Improved transplantation techniques for severe combined immunodeficiency (SCID) mouse models of RA can be used to evaluate the effectiveness of potential therapeutics in human tissues and cells. This review provides basic information on various animal models of RA, including CIA and CAIA. In addition, we describe a SCID mouse coimplantation model that can measure the long-distance migration of human RA synoviocytes and cartilage destruction induced by these cells.
Rheumatoid arthritis (RA) is a common autoimmune disorder that affects approximately 1% of the global population. It is characterized by tumor-like expansion of the synovium, angiogenesis, and destruction of the articular cartilage and bone [1]. Despite the advent of anticytokine therapies that ameliorate the inflammatory manifestations of RA, there is no curative treatment and the pathogenesis of the disease is not fully understood. In RA joints, various cell populations, including innate immune cells, adaptive immune cells, endothelial cells, and fibroblast-like synoviocytes (FLSs), are activated [1]. Resident synoviocytes participate in the chronic inflammatory response and joint destruction in RA, and in fact, these cells represent the major cell population in the invasive pannus [2]. RA-FLSs have the potential to secrete matrix-degrading enzymes (matrix metalloproteinase 3 [MMP3] and MMP9), pro-inflammatory cytokines (interleukin 1 [IL-1] and IL-6), chemokines (IL-8 and C-C motif chemokine ligand 2), and angiogenic factors (vascular endothelial growth factor and placental growth factor) [2]. Moreover, although RA synoviocytes are primary cells, they proliferate abnormally and display resistance to Fas-mediated apoptosis, similar to cancer cells [2,3]. During RA development, the phenotype of RA-FLSs is altered to an invasive and aggressive behavior, with increased migratory ability and reduced attachment-dependent growth, leading to articular cartilage destruction [2,4]. RA-FLSs can spread by migrating from the affected site to distant unaffected joints in immune-deficient mice [5]. In this regard, antimigratory agents targeting RA synoviocytes may be of therapeutic benefit. Despite the importance of FLSs in RA pathogenesis, no attempt has been made to develop an animal model capable of specifically identifying FLS migration and invasiveness. This review summarizes the immunological properties of mouse models of RA and describes humanized models that are capable of measuring the migration and invasiveness of human synoviocytes.
As RA is an autoimmune disease characterized by joint destruction, careful consideration is needed when choosing the correct animal model for different in vivo experiments. Therefore, careful analysis of specific aspects of the disease and specific knowledge targeted in each study must be considered when choosing an RA animal model. Animal models of RA can be broadly divided into induced and spontaneous models, including mutant and genetically altered strains. The induced models may be polyarthritic (systemic response), which is more likely to be severe, or monoarthritic (local response). Spontaneous models progress naturally and generally involve nonresolving, chronic conditions. In this study, we have summarized the involvement of immune cells and cytokines in these models (Table 1).
RA in animals is induced by treatment with agents, such as antigens, antibodies, adjuvants, and serum. Examples of induced mouse models of RA include collagen-induced arthritis (CIA), adjuvant-induced arthritis, proteoglycan-induced arthritis (PGIA), and collagen antibody-induced arthritis (CAIA).
CIA is the most frequently used animal model of RA because it shares both immunological and pathological features with human RA, including symmetric joint involvement, synovitis, and cartilage and bone destruction [2,5]. It is elicited in genetically susceptible mouse strains by immunization with type II collagen (CII) emulsified in complete Freund’s adjuvant (CFA). The requirement of T and B cells in the development of CIA is clear [6]. The autoimmune response to CII is characterized by both the stimulation of CII-specific T cells and secretion of specific immunogens and autoantigens [6]. Moreover, CD4+ T cells are believed to participate in the induction phase of the disease, supporting the activation of collagen-specific B cells [6,7].
Various cytokines have been implicated in the pathogenesis of RA through time-dependent immune system activation [8]. At the time of the clinical onset of arthritis, proinflammatory mediators (tumor necrosis factor alpha [TNF-α], IL-1β, and IL-6) and anti-inflammatory mediators (IL-10, IL-1 receptor antagonist [IL-1Ra], and transforming growth factor-beta isoforms [TGF-β1, TGF-β2, and TGF-β3]) can be detected in CIA joints [8-10]. Because interferon gamma (IFN-γ) inhibits Th17 cell development, IFN-γ receptor-knockout mice develop CIA more readily [11]. IL-23 is also required for the induction of joint inflammation by mediators including IL-17 [12,13].
Virtually any animal species can be used to generate antigen-induced arthritis (AIA) models. In mice, ovalbumin and bovine serum albumin (BSA) are commonly used, and modified antigens (e.g., methylated antigens) can induce chronic arthritis [14]. Antigens, such as methylated BSA, are cationic substances that bind negatively to cartilage [14,15]. When antigens are injected into one or multiple joints, inflammation progresses quickly and complement is activated locally, resulting in cartilage destruction [14]. AIA is T cell-dependent in hypothymic nu/nu mice [14]. In a recent study, both IL-23p19–/– and IL-17Ra–/– mice displayed significantly milder arthritis than wild-type mice, demonstrating that AIA progression is dependent on IL-23 and IL-17Ra signaling [16,17].
PGIA is a progressive polyarthritis characterized by symmetrical synovitis, marginal erosion, pannus formation, and synovial infiltration of immune cells [18]. It is induced by the injection of human cartilage proteoglycans (PGs) into BALB/c (H-2d) mice, which exhibit remission and exacerbation. The first and fourth injections contain PG/CFA, the second and third injections contain PG/incomplete Freund’s adjuvant (IFA), and arthritis develops on approximately day 11 and reaches maximum severity 2 to 4 weeks after the last PG injection [19]. Mice with PGIA display CD4+ T cell responses along with an antibody response to PG, and immunoglobulin G (IgG) 2a antibody levels are correlated with disease severity [18]. Although PGIA is a predominantly Th1-type arthritis model, it has been reported that IL-4 deficiency increases the severity of PGIA [20]. In addition, treatment with IL-4 or IFN deficiency suppresses PGIA [21].
CAIA can be induced in many susceptible mouse strains, and clinical signs of arthritis generally appear a few days after antibody administration [22]. A cocktail of anti-CII monoclonal antibodies is administered to induce arthritis in the mice. Intraperitoneal injection of lipopolysaccharide increases disease severity and the incidence of arthritis; however, it is not essential for disease induction [22].
Disease initiation in CAIA models occurs mainly independently of B and T cells; however, immune cells modulate inflammation during the effector phase [23]. The main effector cells appear to be neutrophils and macrophages, which are activated by immune complexes formed in the joints via binding of CII antibodies to cartilage surfaces [23]. Typical pathological changes observed in RA, such as synovitis, pannus formation, and cartilage and bone destruction, are observed in the joints of animals with CAIA [24,25]. Although the cytokines involved in the pathogenesis of CAIA have not been studied in detail, the involvement of IL-1β, IL-6, and TNF is anticipated [24,26].
Genetic manipulations that affect the pathogenesis of arthritis can lead to arthritis in a variety of mice.
The first TNF transgenic mouse model was generated by Keffer et al. in 1991 [27]. They generated transgenic mice expressing wild-type and 3′-modified human TNF-α transgenes [27]. In this mouse model, synovial hyperplasia and inflammatory cell infiltration were observed in the joints after 3 to 4 weeks of age, and pannus formation, cartilage destruction, and bone erosion were observed in the mice at approximately 10 weeks of age [28,29]. This model highlights the importance of TNF-α in the pathogenesis of arthritis and can be used for the development of TNF inhibitors.
IL-1 is an important inflammatory cytokine produced by a variety of cell types, including activated monocytes, macrophages, fibroblasts, and synovial cells [30]. In contrast, the IL-1 inhibitor IL-1Ra regulates the activity of IL-1 as an anti-inflammatory protein that binds to the IL-1 receptor [31,32]. It has been reported that IL-1Ra knockout mice develop spontaneous arthritis mediated by the amplification of Th17-dependent inflammation [32,33]. In a BALB/c background, polyarthritis develops starting at 5 weeks of age, and by 12 weeks of age, almost all mice are affected [33]. Histopathological analysis revealed marked synovial and periarticular inflammation, with articular destruction caused by the invasion of granulation tissues, closely resembling RA in humans [32]. Moreover, high levels of antibodies against IgG, CII, and double-stranded DNA were detectable in the sera of these mice [32,33].
The most commonly used mouse models are CIA and CAIA. CAIA bypasses the host’s generation of autoantibodies to CII; thus, it can be induced in mice that do not possess CIA-susceptible major histocompatibility complex haplotypes (H-2q and H-2r), such as BALB/c and C57BL/6 mice [22,24]. Therefore, CAIA can be induced in most mouse strains, including transgenic and knockout mice, with an arthritis incidence approaching 100% [22]. This model is ideal for screening and evaluating anti-inflammatory agents without the effects of CFA or IFA, which strongly affect the host’s immune response.
Table 2 presents the similarities and differences between the CIA and CAIA models, and a time comparison.
Mouse models of RA have been used for decades to study the immunopathogenesis of the disease and to explore therapeutic strategies. Nevertheless, differences in the immune systems of humans and mice have limited confidence in the success of disease treatments. To overcome this issue, severe combined immunodeficiency (SCID) mice have been used to investigate human tissues in autoimmune disease [34-37].
The use of SCID mice provides a novel model for studying tissues and cells from patients with RA [5,36]. In this review, we introduce a method that can measure cell migration and the ability to destroy human cartilage by applying synovial cells isolated from patients with RA in SCID mice.
FLSs, which are components of the synovial membrane, play a critical role in RA pathogenesis through aggressive migration and matrix invasion [2,4]. A model for measuring the migration of synoviocytes to the site of inflammation was established by subcutaneous induction of inflammation with CFA in SCID mice and then injecting synoviocytes from patients with RA around the site of inflammation [38]. One day after the CFA injection, RA-FLSs labeled with fluorescent dyes were implanted intradermally into the mice at a fixed distance (1.2 cm) from the injection site (Fig. 1). Five days after implantation, skin samples were obtained from the FLS-implanted site, CFA-injected site, and a region between these two sites (sites a, c, and b in Fig. 1). The migration of synoviocytes was analyzed according to the presence or absence of human leukocyte antigen class I (+) cells in each tissue site obtained at the end of the experiment [38].
RA starts in a few joints; however, as the disease progresses, it can affect all joints [5]. Interestingly, it has been reported that RA synoviocytes in a SCID mouse model can travel long distances through the bloodstream and migrate toward, attach to, and invade the distant cartilage matrix [5]. This model may be useful for elucidating the role and mechanism of an agent targeting activated synoviocytes in RA. In this section, we summarize the protocol for developing a synoviocyte migration and invasion model in SCID mice.
FLSs were prepared from synovial tissues of patients with RA [4,38]. Normal human cartilage was obtained from the nonarthritic knee joints of patients undergoing amputation [5,39]. Cartilage and RA synoviocytes in sponges were coimplanted into SCID mice at the primary site (6–8 weeks), and cartilage without synoviocytes was implanted at the contralateral site [5,35,39]. After 60 days, the implants were removed and either immediately embedded in a snap-frozen medium or fixed in 4% buffered formalin for paraffin embedding [5,37,39]. Using standard hematoxylin and eosin staining, each specimen was evaluated for invasion and perichondrocytic cartilage degradation in the implanted cartilage.
A procedural overview of the current model is shown in Fig. 2.
The careful selection, design, and implementation of animal models are important strategies for RA treatment. In this review article, we describe a variety of mouse models that are widely used or that exhibit pathologies similar to those of arthritis in humans. These models were divided into induced and genetically modified groups. This summary of the characteristics of each model will help researchers to select the best animal model for evaluating the effectiveness of experimental treatments for RA. Furthermore, humanized models are extremely important for investigating the role and mechanism of RA-FLSs targeting these cells.
Notes
Funding
This work was supported by grants from the National Research Foundation of Korea (NRF), funded by the Ministry of Education, Science, and Technology (2019R1A2C2010897).
Author contributions
Conceptualization, Data curation, Resources, Software: all authors; Formal analysis: SAY, GHJ; Funding acquisition, Validation: SAY, JSK; Methodology, Investigation, Project administration, Visualization, Supervision: SAY; Writing-original draft: SAY; Writing-review & editing: SAY, JSK.
References
1. McInnes IB, Schett G. The pathogenesis of rheumatoid arthritis. N Engl J Med. 2011; 365:2205–19.
2. Bartok B, Firestein GS. Fibroblast-like synoviocytes: key effector cells in rheumatoid arthritis. Immunol Rev. 2010; 233:233–55.
3. Matsuno H, Yudoh K, Nakazawa F, Sawai T, Uzuki M, Nishioka K, et al. Antirheumatic effects of humanized anti-Fas monoclonal antibody in human rheumatoid arthritis/SCID mouse chimera. J Rheumatol. 2002; 29:1609–14.
4. Yoo SA, Park JH, Hwang SH, Oh SM, Lee S, Cicatiello V, et al. Placental growth factor-1 and -2 induce hyperplasia and invasiveness of primary rheumatoid synoviocytes. J Immunol. 2015; 194:2513–21.
5. Lefèvre S, Knedla A, Tennie C, Kampmann A, Wunrau C, Dinser R, et al. Synovial fibroblasts spread rheumatoid arthritis to unaffected joints. Nat Med. 2009; 15:1414–20.
6. Inglis JJ, Notley CA, Essex D, Wilson AW, Feldmann M, Anand P, et al. Collagen-induced arthritis as a model of hyperalgesia: functional and cellular analysis of the analgesic actions of tumor necrosis factor blockade. Arthritis Rheum. 2007; 56:4015–23.
7. Taneja V, Taneja N, Paisansinsup T, Behrens M, Griffiths M, Luthra H, et al. CD4 and CD8 T cells in susceptibility/protection to collagen-induced arthritis in HLA-DQ8-transgenic mice: implications for rheumatoid arthritis. J Immunol. 2002; 168:5867–75.
8. Marinova-Mutafchieva L, Gabay C, Funa K, Williams RO. Remission of collagen-induced arthritis is associated with high levels of transforming growth factor-beta expression in the joint. Clin Exp Immunol. 2006; 146:287–93.
9. Lee KM, Zhang Z, Achuthan A, Fleetwood AJ, Smith JE, Hamilton JA, et al. IL-23 in arthritic and inflammatory pain development in mice. Arthritis Res Ther. 2020; 22:123.
10. Kannan K, Ortmann RA, Kimpel D. Animal models of rheumatoid arthritis and their relevance to human disease. Pathophysiology. 2005; 12:167–81.
11. Chu CQ, Song Z, Mayton L, Wu B, Wooley PH. IFNgamma deficient C57BL/6 (H-2b) mice develop collagen induced arthritis with predominant usage of T cell receptor Vbeta6 and Vbeta8 in arthritic joints. Ann Rheum Dis. 2003; 62:983–90.
12. Murphy CA, Langrish CL, Chen Y, Blumenschein W, McClanahan T, Kastelein RA, et al. Divergent pro- and antiinflammatory roles for IL-23 and IL-12 in joint autoimmune inflammation. J Exp Med. 2003; 198:1951–7.
13. Lubberts E, Koenders MI, Oppers-Walgreen B, van den Bersselaar L, Coenen-de Roo CJ, Joosten LA, et al. Treatment with a neutralizing anti-murine interleukin-17 antibody after the onset of collagen-induced arthritis reduces joint inflammation, cartilage destruction, and bone erosion. Arthritis Rheum. 2004; 50:650–9.
14. Brackertz D, Mitchell GF, Mackay IR. Antigen-induced arthritis in mice: I. Induction of arthritis in various strains of mice. Arthritis Rheum. 1977; 20:841–50.
15. Bendele A. Animal models of rheumatoid arthritis. J Musculoskelet Neuronal Interact. 2001; 1:377–85.
16. Razawy W, Asmawidjaja PS, Mus AM, Salioska N, Davelaar N, Kops N, et al. CD4+ CCR6+ T cells, but not γδ T cells, are important for the IL-23R-dependent progression of antigen-induced inflammatory arthritis in mice. Eur J Immunol. 2020; 50:245–55.
17. Cornelissen F, Mus AM, Asmawidjaja PS, van Hamburg JP, Tocker J, Lubberts E. Interleukin-23 is critical for full-blown expression of a non-autoimmune destructive arthritis and regulates interleukin-17A and RORgammat in gammadelta T cells. Arthritis Res Ther. 2009; 11:R194.
18. Glant TT, Mikecz K, Bartlett RR, Deák F, Thonar EJ, Williams JM, et al. Immunomodulation of proteoglycan-induced progressive polyarthritis by leflunomide. Immunopharmacology. 1992; 23:105–16.
19. Mikecz K, Glant TT, Poole AR. Immunity to cartilage proteoglycans in BALB/c mice with progressive polyarthritis and ankylosing spondylitis induced by injection of human cartilage proteoglycan. Arthritis Rheum. 1987; 30:306–18.
20. Kaplan C, Valdez JC, Chandrasekaran R, Eibel H, Mikecz K, Glant TT, et al. Th1 and Th2 cytokines regulate proteoglycan-specific autoantibody isotypes and arthritis. Arthritis Res. 2002; 4:54–8.
21. Doodes PD, Cao Y, Hamel KM, Wang Y, Rodeghero RL, Mikecz K, et al. IFN-gamma regulates the requirement for IL-17 in proteoglycan-induced arthritis. J Immunol. 2010; 184:1552–9.
22. Nandakumar KS, Holmdahl R. Efficient promotion of collagen antibody induced arthritis (CAIA) using four monoclonal antibodies specific for the major epitopes recognized in both collagen induced arthritis and rheumatoid arthritis. J Immunol Methods. 2005; 304:126–36.
23. Nandakumar KS, Bäcklund J, Vestberg M, Holmdahl R. Collagen type II (CII)-specific antibodies induce arthritis in the absence of T or B cells but the arthritis progression is enhanced by CII-reactive T cells. Arthritis Res Ther. 2004; 6:R544–50.
24. Moore AR, Allden S, Bourne T, Denis MC, Kranidioti K, Okoye R, et al. Collagen II antibody-induced arthritis in Tg1278TNFko mice: optimization of a novel model to assess treatments targeting human TNFα in rheumatoid arthritis. J Transl Med. 2014; 12:285.
25. Zhao T, Xie Z, Xi Y, Liu L, Li Z, Qin D. How to model rheumatoid arthritis in animals: from rodents to non-human primates. Front Immunol. 2022; 13:887460.
26. Wang C, Wang W, Jin X, Shen J, Hu W, Jiang T. Puerarin attenuates inflammation and oxidation in mice with collagen antibody-induced arthritis via TLR4/NF-κB signaling. Mol Med Rep. 2016; 14:1365–70.
27. Keffer J, Probert L, Cazlaris H, Georgopoulos S, Kaslaris E, Kioussis D, et al. Transgenic mice expressing human tumour necrosis factor: a predictive genetic model of arthritis. EMBO J. 1991; 10:4025–31.
28. Williams RO, Feldmann M, Maini RN. Cartilage destruction and bone erosion in arthritis: the role of tumour necrosis factor alpha. Ann Rheum Dis. 2000; 59(Suppl I):i75–80.
29. Joe B, Griffiths MM, Remmers EF, Wilder RL. Animal models of rheumatoid arthritis and related inflammation. Curr Rheumatol Rep. 1999; 1:139–48.
30. Kinne RW, Stuhlmüller B, Burmester GR. Cells of the synovium in rheumatoid arthritis. Macrophages. Arthritis Res Ther. 2007; 9:224.
31. Horai R, Nakajima A, Habiro K, Kotani M, Nakae S, Matsuki T, et al. TNF-alpha is crucial for the development of autoimmune arthritis in IL-1 receptor antagonist-deficient mice. J Clin Invest. 2004; 114:1603–11.
32. Horai R, Saijo S, Tanioka H, Nakae S, Sudo K, Okahara A, et al. Development of chronic inflammatory arthropathy resembling rheumatoid arthritis in interleukin 1 receptor antagonist-deficient mice. J Exp Med. 2000; 191:313–20.
33. Nakae S, Saijo S, Horai R, Sudo K, Mori S, Iwakura Y. IL-17 production from activated T cells is required for the spontaneous development of destructive arthritis in mice deficient in IL-1 receptor antagonist. Proc Natl Acad Sci U S A. 2003; 100:5986–90.
34. Duchosal MA. SCID mice in the study of human autoimmune diseases. Springer Semin Immunopathol. 1992; 14:159–77.
35. Elkon KB, Ashany D. The SCID mouse as a vehicle to study autoimmunity. Br J Rheumatol. 1993; 32:4–12.
36. Schinnerling K, Rosas C, Soto L, Thomas R, Aguillón JC. Humanized mouse models of rheumatoid arthritis for studies on immunopathogenesis and preclinical testing of cell-based therapies. Front Immunol. 2019; 10:203.
37. Müller-Ladner U, Kriegsmann J, Franklin BN, Matsumoto S, Geiler T, Gay RE, et al. Synovial fibroblasts of patients with rheumatoid arthritis attach to and invade normal human cartilage when engrafted into SCID mice. Am J Pathol. 1996; 149:1607–15.
38. You S, Yoo SA, Choi S, Kim JY, Park SJ, Ji JD, et al. Identification of key regulators for the migration and invasion of rheumatoid synoviocytes through a systems approach. Proc Natl Acad Sci U S A. 2014; 111:550–5.
39. Dang J, Zhu S, Wang J. A protocol for humanized synovitis mice model. Am J Clin Exp Immunol. 2019; 8:47–52.
Fig. 1.
Schematic of model using synoviocyte migration in severe combined immunodeficiency mice. Skin inflammation was induced by subcutaneously injecting CFA (120 µg) into site a. One day after the CFA injection, rheumatoid arthritis-FLSs were implanted intradermally in site c. Five days after human FLSs implantation, skin samples were obtained from site b. CFA, complete Freund’s adjuvant; FLS, fibroblast-like synoviocyte.
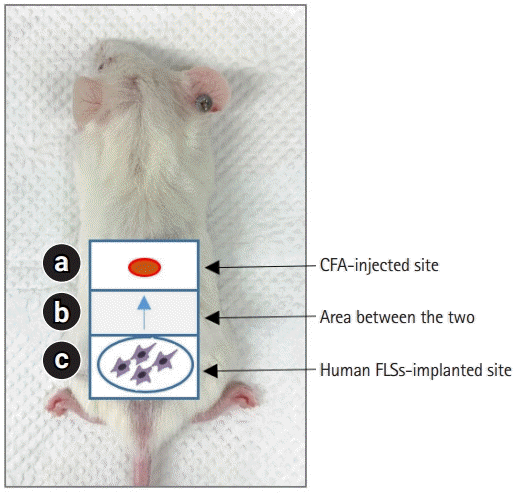
Fig. 2.
Schematic of model using synoviocyte migration and invasion SCID. Cartilage and rheumatoid arthritis-FLSs were coimplanted into SCID mice at the primary site and cartilage without cells was implanted at the contralateral site. After 60 days, the implants were removed, embedded in paraffin, and stained with hematoxylin and eosin (H&E). Implant evaluation was performed using H&E-stained sections to determine invasion and perichondrocytic cartilage degradation. SCID, severe combined immunodeficiency; FLS, fibroblast-like synoviocyte.
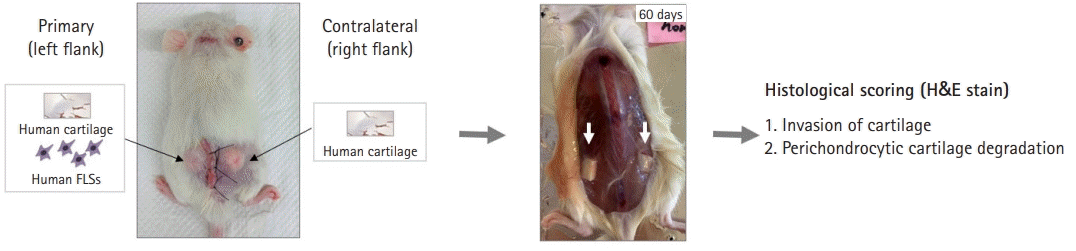
Table 1.
Involvement of immune cells and cytokines in mouse models of arthritis
Table 2.
Similarities and differences between animal models of CIA and CAIA