Abstract
Background
As the application of radiotherapy to brain metastasis (BM) increases, the incidence of radiation necrosis (RN) as a late toxicity of radiotherapy also increases. However, no specific treatment for RN is indicated except long-term steroids. Here, we summarize the clinical results of bevacizumab (BEV) for RN.
Methods
Ten patients with RN who were treated with BEV monotherapy (7 mg/kg) were retrospectively reviewed. RN diagnosis was made using MRI with or without perfusion MRI. Radiological response was based on Response Assessment in Neuro-Oncology criteria for BM. The initial response was observed after 2 cycles every 2 weeks, and maintenance observed after 3 cycles every 3–6 weeks of increasing length intervals.
Results
The initial response of gadolinium (Gd) enhancement diameter maintained stable disease (SD) in 9 patients, and 1 patient showed partial response (PR). The initial fluid-attenuated inversion recovery (FLAIR) response showed PR in 4 patients and SD in 6 patients. The best radiological response was observed in 9 patients. Gd enhancement response was 6 PR and 3 SD between 15–43 weeks. Reduction of FLAIR showed PR in 5 patients and SD in 4 patients. Clinical improvement was observed in all but 1 patient. Five patients were maintained on protocol with durable response up to 23 cycles. However, 2 patients stopped treatment due to primary cancer progression, 1 patient received surgical removal from tumor recurrence, and 1 patient changed to systemic chemotherapy for new BM. Grade 3 intractable hypertension occurred in 1 patient who had already received antihypertensive medication.
The incidence of brain metastasis (BM) is increasing as cancer patient survival is prolonged by advanced cancer therapies, as up to 15% of cancer patients can be expected to develop BM during the course of disease [12]. BM treatment has also advanced over the past few decades, including stereotactic radiosurgery. Thus, whole brain radiotherapy (WBRT) is no longer a standard therapy, even for multiple BM [3]. Since the mid-1990s, stereotactic radiosurgery/radiotherapy (SRS/SRT), which delivers relatively high biologically equivalent doses to BM in a single session or 2–3 sessions, has been considered as an alternative to, or to be used in combination with, surgery or WBRT for single or oligo-metastatic BM [456]. Studies suggest that SRS/SRT could provide equal or more prolonged control of BM and improve patient overall survival (OS) than WBRT or surgery alone [78]. Furthermore, while SRS/SRT saves radiation doses to critical brain tissue in addition to prolonged OS, re-treatment of recurrent BM with SRS/SRT has been attempted in recent decades [91011121314]. However, the long-term results of high single fraction doses and re-irradiation reported a considerable incidence of radiation necrosis (RN) and cognitive dysfunction from brain atrophy [391516171819].
RN manifests as the necrosis of targeted white matter with peri-lesional edema from leaky blood vessels. Clinical manifestations are various neurological deficits according to the location of the lesion, which sometimes progress to clinical deterioration. Previously, RN diagnosis was problematic, as it is hard to distinguish from recurrent tumors on routine gadolinium (Gd)-enhanced MRI. Recently, dynamic susceptibility-weighted perfusion MRI has been used for differentiating RN from tumor recurrence. However, the reliability of perfusion MRI seems low, as multi-modal approaches such as magnetic resonance spectroscopy, positron emission tomography-computed tomography, and pathological assessment are often required [20].
Additionally, treatment for RN is not fully standardized. Steroids have been the most commonly prescribed treatment options for brain RN. However, their adverse effects over long-term use and lack of efficacy in some cases limit their general use for RN treatment. Treatment efficacy of anti-coagulation and anti-platelet agents as well as hyperbaric oxygen therapy is still controversial. As the pathophysiology of RN is composed of endothelial cell dysfunction with concomitant release of vasoactive substances such as vascular endothelial growth factor (VEGF) [21], bevacizumab (BEV), a monoclonal antibody against VEGF, has been highlighted to reduce vascular permeability and brain edema. Gonzales et al. [22] reported a dramatic reduction of MRI-diagnosed RN in both Gd-enhanced and fluid-attenuated inversion recovery (FLAIR) images with BEV alone or in combination with other chemotherapeutic agents in patients with malignant brain tumors. Later, Levin et al. [23] verified the effectiveness of BEV for RN in a randomized, double-blind placebo-controlled trial in 14 patients diagnosed with RN.
Currently, clinical results of BEV monotherapy for RN has not been reported in Korea. Herein, we present our clinical findings using BEV monotherapy for RN in 10 patients from a single institute.
Using an electronic medical records database, we retrospectively identified 74 patients who received both brain radiation and BEV treatment between January 2010 and December 2019. From these, we excluded cases in which BEV was administered in combination with other chemotherapeutic agents to treat either systemic cancer or primary brain tumors. Ultimately, we confirmed 10 patients with RN after radiotherapy who had received BEV monotherapy for RN. This study was in accordance with the precepts established by the Helsinki Declaration and approved by the Institutional Review Board of the National Cancer Center of Korea (NCCCTS-2020-0122-0001).
Diagnosis of RN was based on conventional MRI with or without dynamic susceptibility-weighted contrast-enhanced perfusion MRI. Patients were required to have had a newly appeared or aggravated contrast-enhancing mass within the radiation field on MRI after brain radiation for BM. The following criteria has been considered as suggestive for RN: 1) increased T1 contrast enhancement located in the irradiated area with central hypointensity and increased peripheral edema; 2) substantial regression or stability (for ≥4 months) of enhancing areas on serial follow-up MRI scans without additional treatment; 3) a clear absence of perfusion in the absence of any nodular highly vascularized area within the contrast-enhanced lesion using perfusion MRI [24].
Fig. 1 shows a schematic describing the treatment protocol of BEV monotherapy for RN. All patients received at least 2 cycles of BEV (7 mg/kg) every 2 weeks. At 4 weeks, before the third cycle of BEV, we performed a routine contrast-enhanced MRI with T2/FLAIR imaging to evaluate treatment response and neurologic status of patients (the initial response). If patients had improved clinical and radiological status, patients entered into maintenance BEV treatment. The empirical maintenance protocol was to increase the BEV treatment period from 2 weeks to 3 weeks, 4 weeks, and 6 weeks of terminating BEV regimen every 3 cycles. MRI follow-up was performed before every interval-lengthening step. Patients were discontinued from the treatment protocol for any of the following events: 1) clinical or radiological progression; or 2) development of grade 3 or worse side effects according to Common Terminology Criteria for Adverse Events (CTCAE version 4.0; https://ctep.cancer.gov/protocolDevelopment/electronic_applications/ctc.htm). Another tentative BEV protocol (by author LYJ) was executed in this study at the same BEV dose (7 mg/kg). The initial response evaluation was the same at 6 weeks after 2 cycles of BEV every 2 weeks, and 2 more cycles of BEV were given if the initial response was positive, and then discontinued.
To accurately evaluate responses, we adopted the Response Assessment in Neuro-Oncology for Brain Metastasis (RANO-BM) criteria published in 2015 [25]. RANO-BM response assessment is based on the sum diameter of one-dimensional measurements, corticosteroid dosing, and clinical status. In brief, we measured the longest diameter of RN on T2-weighted FLAIR images and T1-weighted Gd enhancement images. A measurable lesion is ≥10 mm in longest diameter, and the sum of measurable lesions is used in the case of multiple RNs. Complete response (CR) is a disappearance of measurable lesions. Partial response (PR) is ≥30% decrease in the sum longest distance relative to baseline. Stable disease (SD) is <30% decrease relative to baseline but <20% increase in sum longest distance relative to nadir. Progressive disease (PD) is ≥20% increase in sum longest distance relative to nadir.
The response evaluation performed after 2 cycles of BEV monotherapy (4 weeks after the first BEV administration) was defined as “the initial response.” During follow-up on maintenance therapy, we assessed the “best radiological response” (BRR) among MRI evaluations before changing schedules or discontinuing BEV.
Demographic data for patients with BM and treated with BEV for RN are summarized in Table 1. Six patients were female and 4 patients were male. The median age at the time of BEV treatment was 54 years (range: 38–71). Primary cancer of treated BM consisted of non-small cell lung cancer (n=6), breast cancer (n=2), ovarian cancer (n=1), and malignant melanoma (n=1). Radiation therapy given for BM was a single session of SRS in 3 patients; a WBRT in 1 patient; and multiple sessions of combination WBRT, proton beam therapy, intensity-modulated radiation therapy, and SRS in 6 other patients. Apparent summated radiation doses ranged from 2,000 cGy/single fraction to 10,500 cGy/10+12 fractions. The mean time from the last radiotherapy to RN diagnosis was 22.9 months (range: 4–54 months). All patients had symptoms of RN, and 8 of them were treated with steroids (median dexamethasone dose of 2 mg p.o. bid) before BEV treatment. The other 2 patients who directly received BEV treatment refused steroids because of the side effects experienced from previous steroid use for their BM. Eight patients had a single RN lesion, 1 patient had 2 symptomatic RNs, and 1 patient had 3 RNs.
All 8 patients (cases 1–7 and 9) that followed the suggested BEV monotherapy protocol above showed radiological and clinical responses at the initial response evaluation. Thus, they had proceeded to the maintenance protocol (Table 1). Meanwhile, 2 other patients (cases 8 and 10) were treated by a tentative protocol (by LYJ) of 4 cycles of BEV (same 7 mg/kg every 2 weeks) and the response evaluation. Thus, all patients received a minimum of 4 cycles of BEV, and the cycles of BEV administered varied from 4–23 cycles based on patient response, clinical condition, and compliance (Table 1).
Among the 8 patients that had continued on to maintenance therapy at a median 13 cycles of BEV, 4 patients were still receiving BEV monotherapy (cases 1, 2, 4, and 7) at the time of evaluation. Among these 4 patients, 1 patient (case 1) showed rebound phenomena 8 months after BEV-off, and restarted the BEV monotherapy protocol. All these 4 patients showed clinical improvement based on the Karnofsky Performance Status scale. Whereas 3 other patients stopped BEV treatment due to recurrent BM (case 3, Fig. 2) or primary cancer progression (cases 5 and 6), the remaining patient (case 9), who showed no discernible improvement of symptoms despite his radiological response, stopped BEV after 4 cycles on his own accord. Two patients who followed the tentative protocol of consecutive BEV 4 cycles discontinued BEV treatment with symptom resolution. One patient was followed for 2.5 years after BEV-off and maintained a stable status (case 8), but another patient (case 10), who changed systemic treatment regimens due to the emergence of new BM, suffered from recurrent symptoms 7 months after discontinuation of BEV.
We evaluated the radiological responses to identify any association with pretreatment MRI findings, although the number of cases was too small to determine statistical significance (Table 2). In 7 patients, magnetic resonance perfusion study was performed and 6 patients showed a definite decrease in regional cerebral blood volume (rCBV), with the remaining 1 patient (case 1) revealing no discernible rCBV change compared to contralateral normal brain. However, in this case, the left parieto-occipital enhancing lesion grew bigger in diameter from 1.8 cm to 3.1 cm, 1.5 years after BEV treatment.
The initial response evaluation for Gd enhancement response was SD in 9 patients and PR in 1 patient, whereas the FLAIR response was more prominent compared to the Gd enhancement response, with PR in 4 patients and SD in 6 patients. In 8 patients, the degree of Gd enhancement was decreased at the initial response evaluation, but 2 out of 3 patients with a hemorrhagic component failed to show decreases in Gd enhancement (cases 1 and 7). These 2 patients had no solid portion but mainly had cystic, necrotic, and hemorrhagic portions, and only the thin wall showed enhancement. The ratio of FLAIR high-signal intensity and Gd enhancement area (FLAIR/Gd ratio) was also obtained in terms of the product of the largest diameter and its perpendicular diameter (Supplementary Table 1 in the online-only Data Supplement). The median FLAIR/Gd ratio was 5.80 (range: 1.83–11.11). Interestingly, among 5 patients with higher FLAIR/Gd ratio, 4 showed PR FLAIR responses at the initial response evaluation (cases 10, 2, 9, and 8 in descending order of the ratio), whereas all 5 patients with lower FLAIR/Gd ratio revealed SD FLAIR response.
The BRR during follow-up was available for 9 patients except 1 patient who was lost to follow-up after the initial response evaluation (case 9). The sum of the longest Gd enhancement diameter was PR (≥30%) in 5 patients and SD in the remaining 4 patients. Interestingly, all 5 patients with Gd enhancement PR at the BRR evaluation had SD response at the initial evaluation (for example, case 2 in Fig. 3). Meanwhile, only 1 patient who showed Gd enhancement PR at the initial response evaluation turned SD due to discontinuation of BEV treatment during follow-up (case 10, Fig. 4). Among 4 patients with FLAIR PR at the initial response evaluation, 3 remained with PR and 1 was lost to follow-up. For 2 other patients who showed SD FLAIR response at the initial response evaluation, PR was achieved at the BRR evaluation.
The final response was obtained at the most recent time of study period or at the termination of BEV treatment, which was measurable in 9 patients (Table 2 and Fig. 5). The final response was maintained in 6 patients as the BRR, but in the other 3 patients, it was affected by primary cancer progression (case 6), recurrence (case 3), or newly developed BM (case 10) and progressed.
We retrospectively searched for possible adverse events associated with BEV treatments (Table 3). Grade 3 hypertension occurred in 1 patient already treated with anti-hypertensive medication as an intractable hypertension. We paused BEV treatment for one month as advised by their cardiologist (case 3) and it was resolved. Proteinuria was observed during routine urine analysis in 2 patients, but required no specific treatment on consultation (cases 1 and 3). Actually, case 3 patient had another problem of wound infection, which was not a surgical wound but a delayed healing of cellulitis of plegic low extremity. Although nephrologist recommend to continue BEV treatment with careful laboratory follow-up, we decided to stop BEV treatment. Both proteinuria and wound infection were spontaneously resolved, the patient suffered from rebound of RN with hemorrhage 6 months later. Gum bleeding occurred in 1 patient with pre-existing gingivitis upon toothbrushing (case 2). Retinal hemorrhage was identified in a patient who complained of “floater in eye” (case 7); however, the ophthalmologist could not differentiate between BEV treatment or spontaneous hemorrhage as the cause, and it resolved spontaneously without specific treatment.
RN, as a manifestation of long-term radiation effects, became clinically significant due to the prolonged survival of patients with BM and the frequent use of SRS or re-irradiation for treating BM over the past few decades. The true incidence of RN is hard to estimate because it can be affected by many factors such as the quality of neuroimaging, follow-up period after radiation, and RN definition (either radiological or histological aspects). Minniti et al. [24] reported a 24% incidence of RN among BM patients (14% symptomatic, 10% asymptomatic), for which they relied on imaging features. Chin et al. [26] reported a 7% incidence of RN based on pathological confirmation or temporal resolution.
Many RN risk factors have been identified, including tumor volume, prescribed dose, fraction size, and interval between re-irradiation. However, the threshold for safe re-irradiation in terms of cumulative dose or treatment volume has been suggested, but is yet to be confirmed [14272829]. A prospective controlled study as well as increased RN awareness in the neuro-oncology field is required for further evaluation.
Currently, oral corticosteroids are the preferred treatment option for brain RN, as steroids reduce inflammatory signals, which dramatically reduces brain edema. However, RN itself is not affected by corticosteroids. Thus, patients with RN require a relatively long period of steroid treatment with or without pausing or gradual dosage tapering, which can be accompanied by serious adverse effects such as iatrogenic Cushing's syndrome [20]. Treatment efficacy of anti-coagulation and anti-platelet agents and hyperbaric oxygen therapy is still controversial. Another issue is that RN is not particularly discernible from treatment-related changes of BM. Case 3 of our study, initially believed to have RN, was in fact, identified with a tumor recurrence 3 years after RN diagnosis and BEV treatment. Patients with RN also suffered from newly developed BM or progression of their primary systemic cancer. In this study, 2 patients died of primary systemic cancer progression and 1 patient stopped BEV due to the emergence of a new distal BM.
There are two hypotheses regarding the pathophysiology of RN: vascular injury and glial cell theory [20]. Radiation disrupts the blood-brain barrier and damages oligodendrocytes, resulting in ischemia, cell death, and leaky capillaries. This leads to increased production of VEGF. When released in the presence of hypoxia and necrosis, VEGF induces neo-angiogenesis made of leaky vessels, which results in perilesional edema and contrast extravasation [21]. Untreated RN can progress to small vessel occlusive disease and bleeding from friable vessels [23]. These concepts support the rationale that VEGF plays a prominent role in RN development. Based on these studies, BEV, a VEGF blocking agents, could play a key role in treating RN, thus proving the hypothesis of “normalization” of restricted blood flow [30]. Gonzales et al. [22] first reported their clinical experience with BEV in combination with or without other chemotherapeutic agents for malignant brain tumors while monitoring possible RN based on MRI findings. They observed a dramatic reduction in both T1-weighted Gd enhancement and FLAIR abnormal areas. Their institute (MD Anderson Cancer Center) later performed a randomized, double-blind, placebo-controlled trial of BEV monotherapy (7.5 mg/kg every 3 weeks) for brain RN [23]. All BEV-treated patients showed both radiological response and clinical improvement, whereas placebo-treated patients did not. Seventy one BEV-treated RN cases from 16 clinical studies and case reports were analyzed by Tye et al. [31], verifying a radiological response rate of 97% and clinical improvement rate of 79%. The largest series of BEV for RN was reported by Wang et al. [32], in which they used BEV in 17 patients with symptomatic RN, administered for a minimum of 2 cycles (7.5 mg/kg every 2 weeks) with a median of 4 BEV cycles. The average reduction of T1-weighted Gd enhancement and T2/FLAIR were 63% and 59%, respectively.
This retrospective study is limited due to the use of two different BEV protocols, even though the two protocols were identical in terms of BEV dosage levels, development of new BM, and primary cancer status. We anticipate prospective clinical trials that control these variables. Furthermore, as it has been reported that some RN might be regressed or resolved in time [33], BEV treatment should be given not just until complete resolution, but until a certain radiological response or clinical improvement. Rebound phenomena after discontinuation of BEV also warrants sophisticated long-term follow-up.
Acknowledgments
This work was supported by grants from the National Cancer Center, Korea (NCC-1910090-2).
References
1. Barnholtz-Sloan JS, Sloan AE, Davis FG, Vigneau FD, Lai P, Sawaya RE. Incidence proportions of brain metastases in patients diagnosed (1973 to 2001) in the Metropolitan Detroit Cancer Surveillance System. J Clin Oncol. 2004; 22:2865–2872. PMID: 15254054.
2. Schouten LJ, Rutten J, Huveneers HA, Twijnstra A. Incidence of brain metastases in a cohort of patients with carcinoma of the breast, colon, kidney, and lung and melanoma. Cancer. 2002; 94:2698–2705. PMID: 12173339.
3. Adler JR, Cox RS, Kaplan I, Martin DP. Stereotactic radiosurgical treatment of brain metastases. J Neurosurg. 1992; 76:444–449. PMID: 1738025.
4. Flickinger JC, Kondziolka D, Lunsford LD, et al. A multi-institutional experience with stereotactic radiosurgery for solitary brain metastasis. Int J Radiat Oncol Biol Phys. 1994; 28:797–802. PMID: 8138431.
5. Kondziolka D, Patel A, Lunsford LD, Kassam A, Flickinger JC. Stereotactic radiosurgery plus whole brain radiotherapy versus radiotherapy alone for patients with multiple brain metastases. Int J Radiat Oncol Biol Phys. 1999; 45:427–434. PMID: 10487566.
6. Alexander E 3rd, Moriarty TM, Davis RB, et al. Stereotactic radiosurgery for the definitive, noninvasive treatment of brain metastases. J Natl Cancer Inst. 1995; 87:34–40. PMID: 7666461.
7. Auchter RM, Lamond JP, Alexander E, et al. A multiinstitutional outcome and prognostic factor analysis of radiosurgery for resectable single brain metastasis. Int J Radiat Oncol Biol Phys. 1996; 35:27–35. PMID: 8641923.
8. Hasegawa T, Kondziolka D, Flickinger JC, Germanwala A, Lunsford LD. Brain metastases treated with radiosurgery alone: an alternative to whole brain radiotherapy? Neurosurgery. 2003; 52:1318–1326. PMID: 12762877.
9. Chen JC, Petrovich Z, Giannotta SL, Yu C, Apuzzo ML. Radiosurgical salvage therapy for patients presenting with recurrence of metastatic disease to the brain. Neurosurgery. 2000; 46:860–866. discussion 866-7. PMID: 10764259.
10. Davey P, O'Brien PF, Schwartz ML, Cooper PW. A phase I/II study of salvage radiosurgery in the treatment of recurrent brain metastases. Br J Neurosurg. 1994; 8:717–723. PMID: 7718169.
11. Hillard VH, Shih LL, Chin S, Moorthy CR, Benzil DL. Safety of multiple stereotactic radiosurgery treatments for multiple brain lesions. J Neurooncol. 2003; 63:271–278. PMID: 12892233.
12. Loeffler JS, Kooy HM, Wen PY, et al. The treatment of recurrent brain metastases with stereotactic radiosurgery. J Clin Oncol. 1990; 8:576–582. PMID: 2179476.
13. Noël G, Proudhom MA, Valery CA, et al. Radiosurgery for re-irradiation of brain metastasis: results in 54 patients. Radiother Oncol. 2001; 60:61–67. PMID: 11410305.
14. Shaw E, Scott C, Souhami L, et al. Radiosurgery for the treatment of previously irradiated recurrent primary brain tumors and brain metastases: initial report of radiation therapy oncology group protocol (90-05). Int J Radiat Oncol Biol Phys. 1996; 34:647–654. PMID: 8621289.
15. Cooper JS, Steinfeld AD, Lerch IA. Cerebral metastases: value of reirradiation in selected patients. Radiology. 1990; 174:883–885. PMID: 2305074.
16. DeAngelis LM, Delattre JY, Posner JB. Radiation-induced dementia in patients cured of brain metastases. Neurology. 1989; 39:789–796. PMID: 2725874.
17. Dritschilo A, Bruckman JE, Cassady JR, Belli JA. Tolerance of brain to multiple courses of radiation therapy. I. Clinical experiences. Br J Radiol. 1981; 54:782–786. PMID: 7296205.
18. Nieder C, Leicht A, Motaref B, Nestle U, Niewald M, Schnabel K. Late radiation toxicity after whole brain radiotherapy: the influence of antiepileptic drugs. Am J Clin Oncol. 1999; 22:573–579. PMID: 10597741.
19. Vigliani MC, Duyckaerts C, Hauw JJ, Poisson M, Magdelenat H, Delattre JY. Dementia following treatment of brain tumors with radiotherapy administered alone or in combination with nitrosourea-based chemotherapy: a clinical and pathological study. J Neurooncol. 1999; 41:137–149. PMID: 10222434.
20. Vellayappan B, Tan CL, Yong C, et al. Diagnosis and management of radiation necrosis in patients with brain metastases. Front Oncol. 2018; 8:395. PMID: 30324090.
21. Kim JH, Chung YG, Kim CY, Kim HK, Lee HK. Upregulation of VEGF and FGF2 in normal rat brain after experimental intraoperative radiation therapy. J Korean Med Sci. 2004; 19:879–886. PMID: 15608402.
22. Gonzalez J, Kumar AJ, Conrad CA, Levin VA. Effect of bevacizumab on radiation necrosis of the brain. Int J Radiat Oncol Biol Phys. 2007; 67:323–326. PMID: 17236958.
23. Levin VA, Bidaut L, Hou P, et al. Randomized double-blind placebo-controlled trial of bevacizumab therapy for radiation necrosis of the central nervous system. Int J Radiat Oncol Biol Phys. 2011; 79:1487–1495. PMID: 20399573.
24. Minniti G, Clarke E, Lanzetta G, et al. Stereotactic radiosurgery for brain metastases: analysis of outcome and risk of brain radionecrosis. Radiat Oncol. 2011; 6:48. PMID: 21575163.
25. Lin NU, Lee EQ, Aoyama H, et al. Response assessment criteria for brain metastases: proposal from the RANO group. Lancet Oncol. 2015; 16:e270–e278. PMID: 26065612.
26. Chin LS, Ma L, DiBiase S. Radiation necrosis following gamma knife surgery: a case-controlled comparison of treatment parameters and long-term clinical follow up. J Neurosurg. 2001; 94:899–904. PMID: 11409517.
27. Ang KK, Price RE, Stephens LC, et al. The tolerance of primate spinal cord to re-irradiation. Int J Radiat Oncol Biol Phys. 1993; 25:459–464. PMID: 8436524.
28. Gwak HS, Yoo HJ, Youn SM, Lee DH, Kim MS, Rhee CH. Radiosurgery for recurrent brain metastases after whole-brain radiotherapy : factors affecting radiation-induced neurological dysfunction. J Korean Neurosurg Soc. 2009; 45:275–283. PMID: 19516944.
29. Kilburn JM, Ellis TL, Lovato JF, et al. Local control and toxicity outcomes in brainstem metastases treated with single fraction radiosurgery: is there a volume threshold for toxicity? J Neurooncol. 2014; 117:167–174. PMID: 24504497.
30. Thompson EM, Frenkel EP, Neuwelt EA. The paradoxical effect of bevacizumab in the therapy of malignant gliomas. Neurology. 2011; 76:87–93. PMID: 21205697.
31. Tye K, Engelhard HH, Slavin KV, et al. An analysis of radiation necrosis of the central nervous system treated with bevacizumab. J Neurooncol. 2014; 117:321–327. PMID: 24504500.
32. Wang Y, Pan L, Sheng X, et al. Reversal of cerebral radiation necrosis with bevacizumab treatment in 17 Chinese patients. Eur J Med Res. 2012; 17:25. PMID: 22913802.
33. Wang YX, King AD, Zhou H, et al. Evolution of radiation-induced brain injury: MR imaging-based study. Radiology. 2010; 254:210–218. PMID: 20019142.
Supplementary Materials
The online-only Data Supplement is available with this article at https://doi.org/10.14791/btrt.2020.8.e11.
Supplementary Table 1
The ratio of FLAIR high-signal intensity and Gd enhancement area (FLAIR/Gd ratio) in terms of the product of the largest diameter and its perpendicular diameter
Fig. 1
Schematic presentation of bevacizumab (BEV) monotherapy protocol for radiation necrosis. Patients with clinical suspicion of symptomatic radiation necrosis based on contrast-enhanced MRI underwent dynamic susceptibility perfusion imaging for confirmation. After 2 cycles of BEV (7 mg/kg every 2 weeks), the first follow-up images were collected to assess the radiologic response with BEV. If a response to treatment was observed, maintenance BEV therapy was started (7 mg/kg every 3 weeks) and serial follow-up images were obtained every 3 cycles with intervals lengthening up to 6 weeks. After a total of 11 cycles of BEV, patients were removed from BEV monotherapy and progress was monitored.
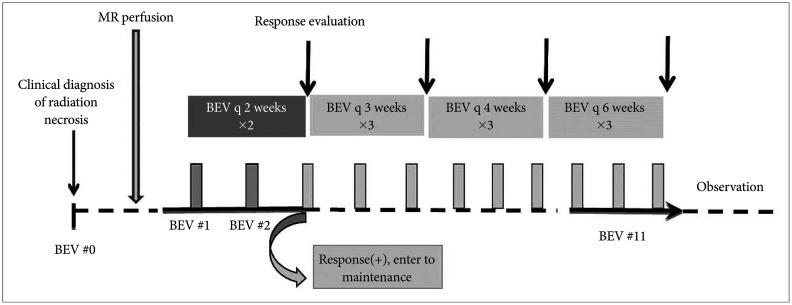
Fig. 2
Progression of radiation necrosis with hemorrhagic portion after bevacizumab (BEV) treatment on gadolinium enhancement (upper row) and fluid-attenuated inversion recovery (FLAIR) magnetic resonance imaging (lower row) (Case 3). A: A 63-year-old woman with brain metastasis from non-small cell lung cancer received two stereotactic radiosurgeries of 3,000 cGy in three fractions 23 months prior to radiation necrosis diagnosis. Intra-lesional hemorrhage was noticed on the FLAIR image. B: The initial response showed a decrease in both gadolinium enhancement and FLAIR image-defined brain edema. C: The first progression with hemorrhage occurred 6 months after BEV-off due to proteinuria and delayed wound healing during the maintenance. D: The second re-bleeding, 9 months after BEV-off due to intractable hypertension following an additional 9 cycles of BEV. E: The patient received a craniotomy for regrowth of brain metastasis with hemorrhage 11 months after the second BEV-off.

Fig. 3
Typical radiological response of radiation necrosis to bevacizumab (BEV) monotherapy on gadolinium enhancement (upper row) and fluid-attenuated inversion recovery (FLAIR) magnetic resonance imaging (lower row) (Case 2). A: A 38-year-old woman diagnosed with brain metastasis from breast cancer received 3 series of stereotactic radiosurgery/radiotherapy at a total dose of 7,800 cGy over four years prior to the diagnosis of radiation necrosis. B: The initial response after 2 cycles of BEV (7 mg/kg every 2 weeks) showed reduced enhancement and dramatic decrease of FLAIR high-signal intensity area. C: The best radiological response during maintenance therapy (5 BEV cycles) revealed a further decrease of the enhancement and FLAIR high-signal intensity area.
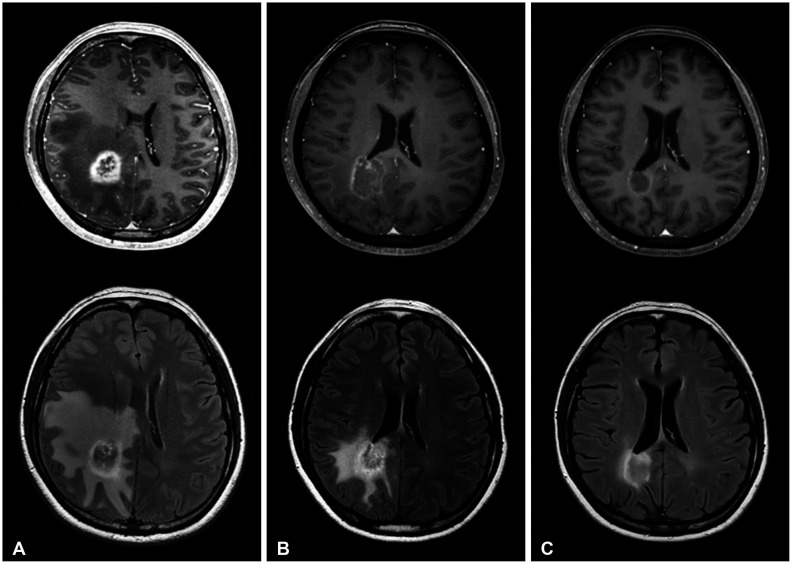
Fig. 4
Serial follow-up images of gadolinium-enhancement (upper row) and FLAIR (lower row) (Case 10): Pretreatment (A), the initial response (B), the best radiological response during follow-up (C), rebound phenomena 7 months after BEV-off (D), and after changing systemic chemotherapy for new brain metastasis (E). FLAIR, fluid-attenuated inversion recovery; BEV, bevacizumab.
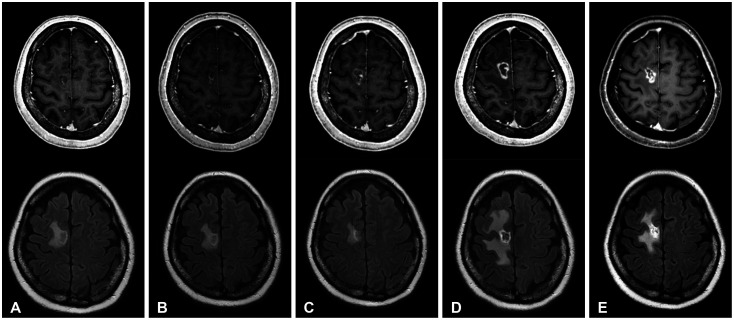
Fig. 5
Change of the sum of longest diameter in terms of gadolinium-enhancement (A) and FLAIR (B) of pretreatment, the initial response, and the BRR during follow-up based on RANO-BM criteria (see details in Methods). FLAIR, fluid-attenuated inversion recovery; BRR, best radiological response; RANO-BM, Response Assessment in Neuro-Oncology for Brain Metastasis.
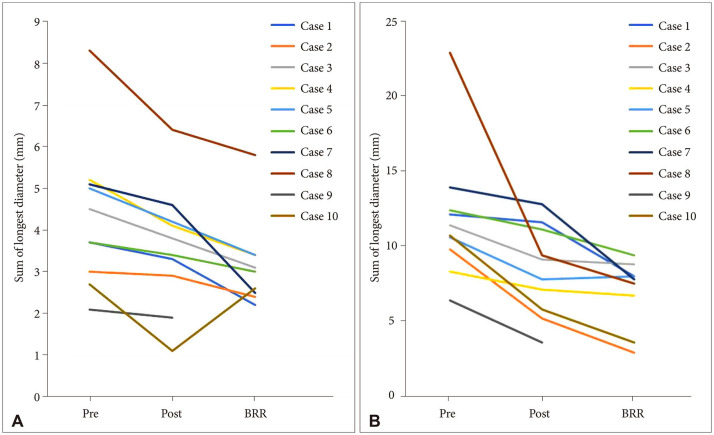
Table 1
Clinical characteristics and BEV response in patients with symptomatic RN
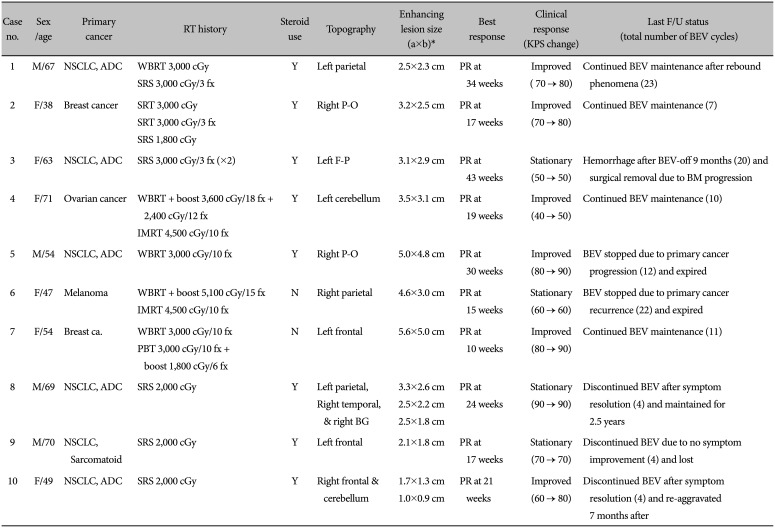
*a×b: the largest diameter × its perpendicular diameter. BEV, bevacizumab; RN, radiation necrosis; NSCLC, non-small cell lung cancer; ADC, adenocarcinoma; WBRT, whole brain radiotherapy; SRS, stereotactic radiosurgery; SRT, stereotactic radiotherapy; IMRT, intensity-modulated radiation therapy; PBT, proton beam therapy; BG, basal ganglia; PR, partial response; KPS, Karnofsky Performance Status; BM, brain metastasis; fx, fraction
Table 2
Pretreatment MRI findings and radiological response
