Abstract
Optic pathway glioma (OPG) is a rare tumor located in optic nerve, optic tract, or optic chiasm. Treatment options for OPG include surgery, radiation therapy (RT), and chemotherapy. Although RT may provide favorable long-term outcomes in manner of either adjuvant or salvage aim, chemotherapy-first approach is increasingly performed due to possible late effects of RT. Proton beam RT may allow normal tissue sparing of radiation exposure compared to conventional X-ray treatment. Therefore, proton beam RT is expected to reduce complications from RT. This review discusses the recent updates on oncologic outcomes of OPG, late toxicities following RT, and compares the outcomes between X-ray treatment and proton beam RT.
Optic pathway glioma (OPG) arises in the optic nerve, optic tract, or optic chiasm, and it is a rare tumor that accounts for 5% of pediatric brain tumors [1]. Although most cases of OPG are considered pathologically low-grade, these tumors range from indolent to progressive [2]. Therefore, an appropriate multidisciplinary approach including observation, surgery, chemotherapy, and radiation therapy (RT) is required for treatment of this diverse condition. Historically, surgical excision followed by RT has resulted in favorable long-term outcomes [3].
Partial resection with adjuvant RT is performed more often than complete surgical resection due to the tumor location. Further, due to residual disease after resection, patients with OPG often demonstrate inferior outcomes among pediatric low-grade gliomas treated with RT [45]. Additionally, RT is administered as a salvage treatment for progressive deep-seated tumors. Recently, physicians have increasingly adopted a chemotherapy-first approach, deferring RT in fear of possible late effects of RT including pseudo-progression, visual disturbance, vasculopathy, endocrine deficiency, neurocognitive impairment, and secondary malignancy. However, recent technical advances in RT, especially intensity-modulated photon RT or proton beam RT (PBT), have enabled effective and safe treatment [6]. The physical advantages of PBT over photon RT (X-ray) is based on the “Bragg peak” which could minimize unnecessary radiation exposure of normal organs [678]. RT using a proton beam can effectively increase the therapeutic ratio by sparing the normal organs, especially in pediatric patients [78].
In this review, we summarize the recent literature regarding RT for treatment of OPG in terms of RT-related toxicities. We also particularly focus on comparison between photon RT and PBT.
Several retrospective series have been published for either pediatric low-grade gliomas in general or OPGs in specific (Table 1). A retrospective study with long-term outcomes after RT limited to OPG has shown favorable long-term survival outcomes of 92%–98% after 10 years [9]. Tsang et al. [9] reported outcomes of 89 patients treated with a total dose of 54 Gy with photon RT with a 10-year progression-free survival of 62%–68%. They reported that pre-RT chemotherapy was associated with inferior progression-free survival outcomes, whereas younger age (particularly in children aged <6 years) at RT was only related to overall survival outcomes. Forty-six patients with pre-RT chemotherapy had recurrent disease after RT administration. This subgroup may have aggressive tumor biology or tumor repopulation resulting in inferior progression-free survival outcomes. A prospective phase II trial, ACNS 0221, investigated conformal photon RT with a total dose of 54 Gy for low-grade glioma in 37/85 (44%) pediatric patients with hypothalamic or suprasellar tumors [10]. With a median follow-up of 5 years, progression-free survival and overall survival were 71% and 93%, respectively, comparable to earlier reports [111213]. Regarding RT techniques, Indelicato et al. [14] demonstrated similar outcomes following PBT compared to photon RT. Among 174 patients with low-grade glioma treated with 50.4–54.0 Gy relative biological effectiveness (RBE) PBT, there were 101 patients with OPG. With a median follow-up of 4.4 years, patients with OPG showed favorable outcomes of a 5-year local control rate, progression-free survival, and overall survival of 89%, 88%, and 93%, respectively. They also observed better local control and progression-free survival following 54 GyRBE than following <54 GyRBE (5-year local control, 91% vs. 67%; 5-year progression-free survival, 90% vs. 67%).
Although there is no comparative study between photon RT and PBT and follow-up period is relatively shorter in study of PBT than photon RT, oncologic outcomes (local control, progression-free survival, and overall survival) were comparable according to the RT modality as summarized in Table 1. In details, progression-free survival at 5 years following photon RT and PBT were 72%-84% [1516] and 88% [14], respectively. Considering the indolent nature of OPG, however, long-term follow-up should be warranted to compare the oncologic effectiveness of PBT compared with photon RT.
Based on current literature review, adopting upfront PBT (or photon RT) followed by chemotherapy cannot be determined. However, upfront PBT may be considered if tolerable toxicities which will be described in this review are expected. Further multidisciplinary discussion is needed considering individual patient and tumor characteristics.
Although pseudoprogression (psPD) after RT for high-grade gliomas has been well investigated, information on psPD after low-grade gliomas is limited. Several reports have described this phenomenon in 15% to 30% of patients after undergoing RT for low-grade gliomas. The underlying mechanism of psPD stems from RT-induced vascular damage [17]. A recent comparison study investigated serial magnetic resonance imaging between photon RT and PBT from 83 pediatric patients with low-grade gliomas. Higher rates of psPD after PBT were observed compared to those after photon RT: overall rates of psPD were 45% and 25%, respectively (p=0.048) [18]. The relative dose heterogeneity of passive scattering PBT compared to photon RT may be related to frequent “hotspots” resulting in increased vascular injuries and frequent psPD. Scanning PBT, an advanced PBT technique, deflects the proton beam using magnets positioned in the upstream of the nozzle and delivers treatment beams across the cross-sectional area of the target by controlling the beam path. A layer-by-layer dose painting of scanning PBT may provide a conformal dose distribution to the distal and proximal edges of the target [19]. Therefore, scanning PBT may reduce this late effect, which could affect the assessment of treatment response [19].
Because of the location of OPG, most patients with OPG usually experience long-term visual impairment following curative treatment, which could significantly affect quality of life [2021]. Although chemotherapy is adopted as a therapeutic approach for young patients to defer RT, chemotherapy could not successfully preserve visual acuity [2223]. Overall, 7%–17% of patients who undergo RT experience visual deficiency (Table 2) [1011121415162425]. Acharya et al. [16] evaluated long-term visual acuity in 41 children with OPG receiving X-ray and showed that incidence of decline in visual acuity was low; 11% and 14% for the better and worse eye, respectively. The decline occurred within the first 2 years after RT and was not related to progression of disease [16]. Recently, Hanania et al. [26] reported that early PBT as an initial or first-line salvage treatment was related to improved 8-year blindness-free survival rate of 100% compared to a 60% rate following chemotherapy. In this report, the visual outcomes of late PBT were not different from those of no PBT. Therefore, early PBT may preserve functional vision in appropriate patients before symptomatic progression. Serial follow-up of 20 patients with OPG demonstrated that surgery may play a major role in baseline function, whereas pre-RT chemotherapy may interrupt the improvement of visual disturbance following RT [27]. All patients treated with pre-RT chemotherapy received delayed RT due to symptomatic progression and they finally had significantly inferior visual acuity in their worse eye [27]. Therefore, an individualized approach incorporating surgery, RT, and chemotherapy is required to preserve long-term visual function in patients with OPG. Although current data support comparable visual function outcomes between X-ray and PBT, there is a chance for improvements in visual outcomes when early PBT approach is encouraged.
Vasculopathy, including cerebral vessel stenosis, Moyamoya disease, and stroke may occur in the late phase (over 10 years) and even in adulthood [28]. The development of vasculopathy highly depends on the location of the tumor. Patients with tumors located in the suprasellar region frequently developed vasculopathy around the Circle of Willis accounting for 9%–20% of incidence: 18%–19% of patients with OPG develop vasculopathy [29]. Tsang et al. [9] reported a 10-year incidence of 7% for clinically significant vasculopathy in 89 patients with OPG. In addition, they found no vasculopathy in any patients older than 10 years. Risk of vasculopathy may increase when RT is administered at young age. Merchant et al. [30] observed higher incidence of vasculopathy in patients <5 years than those >5 years at time of RT for low-grade glioma (12.5% vs. 3.8%).
Specifically, Moyamoya disease has been reported to develop in 5%–18% of patients treated with X-ray. Kestle et al. [31] reported that 18% of 28 patients with OPG were diagnosed with Moyamoya disease after 3.1–4.5 years of treatment. Additionally, 20%–30% of patients experienced stroke symptoms as a long-term sequela. Mueller et al. [32] reported a dose-response relationship of 5% hazard per 1 Gy in 325 patients with low grade gliomas. Radiation to the Circle of Willis may provoke stroke events [333435]. Campen et al. [34] observed stroke in 4.9% in 265 children and 12/13 patients were treated with RT to the Circle of Willis. Bowers et al. [35] also reported that OPG (odds ratio 4.14, 95% confidence interval of 1.08–15.87) was associated with frequent stroke events due to tumor geometry. Among 13/807 patients with brain tumor who experienced non-perioperative stroke, 3 patients had OPG [35]. Series limited to patients with OPG showed that 17%–18% of patients could experience stroke after X-ray [1231].
Currently, there is a limited body of data to report vasculopathy limited to OPG following PBT. Instead, reports of vasculopathy after PBT associated with all brain tumors showed a slightly lower incidence (5%–10%), than those after X-ray treatment [142529363738]. Hall et al. [38] reported a 3-year incidence of serious vasculopathy as 2.6% in 644 children (mostly with craniopharyngioma, ependymoma, or low-grade glioma) treated with PBT. Maximum dose to the optic chiasm >54 GyRBE was related to serious vasculopathy. Bavle et al. [29] also found that 5/81 children (6.2%) who experienced vasculopathy following PBT for brain tumor received >45 GyRBE to the optic chiasm. Moreover, Moyamoya disease and stroke events occurred in 3%–6% and 1%–8% of patients, respectively, within the first 5 years after PBT [142529363738].
Considering tumor geometry and prescription RT dose, patients with OPG are at high-risk of vasculopathy, regardless of RT techniques (X-ray or PBT). Therefore, screening for early detection of any types of vasculopathy needs to be considered prior to symptomatic presentation.
Endocrinopathy in OPG following radiation is highly attributable to both the tumor itself located in the hypothalamic-pituitary axis and the high radiation dose to this region. In a 1990 report by Brauner et al. [39], treating OPG could induce growth hormone (GH) deficiency within 2 years. The incidence of endocrinopathy has been reported to be between 13% to 72% following X-ray treatment and 22%–31% following PBT (Table 2). Tao et al. [11] reported 72% of endocrinopathy in 29 OPG patients treated with photon RT; GH deficiency is the most frequent (59%) endocrinopathy followed by thyroid-stimulating hormone deficiency (48%), precocious puberty (38%), amenorrhea (24%), and adrenocorticotropic hormone deficiency or panhypopituitarism (21%). Granbenbauer et al. [24] also reported details of endocrinopathy following photon RT in 25 OPG patients; GH deficiency in 10 patients (40%) followed by delayed puberty or adrenocorticotropic hormone deficiency in 3 patients (10%), and thyroid stimulating hormone deficiency or panhypopituitarism (2%). In a series of PBT, Greenberger et al. [25] reported 31% of endocrinopathy in pediatric patients with low-grade glioma; GH deficiency and hypothyroidism were frequently observed. They also found the high risk dosage group ≥40 GyRBE was associated with increased incidence of any endocrine deficiency compared to <40 GyRBE (at 5-years, 70% vs. 10%). Since the dose to the hypothalamic-pituitary axis more than 40 Gy may be highly associated with endocrinopathy, neither PBT nor X-ray treatment can minimize the dose to the region considering the high dose (>50 Gy) required for tumor control.
Data on neurocognitive or neuropsychological outcomes is limited in patients with OPG [4041]. In 1998, a report from Cappelli et al. [12] showed that 34% of 53 patients with OPG have encountered intellectual impairments. Papini et al. [41] reported that patients with OPG had a moderate risk of neurocognitive impairment related to visual perception and cognitive proficiency. They examined the neuropsychological outcomes from longitudinal assessments of visual perception, general intelligence, and academic achievements in 12 pediatric patients with OPG.
There are several risk factors contributing to neurocognitive outcomes. Firstly, young age at RT is considered a risk factor for neurocognitive impairment after RT [1242]. In addition, serial measurements of intelligence quotients revealed that radiation dose to the left temporal lobe or hippocampus was highly related to neurocognitive decline [4344]. Potential benefit of intensity-modulated photon RT or PBT compared to traditional 3D-RT in intellectual outcomes may be found in dosimetric studies for other pediatric brain tumors. Qi et al. [45] modeled the effect of temporal lobe dose on intelligence quotient and demonstrated that a reduction of temporal lobe dose with 6 Gy in intensity-modulated photon RT compared to 3D-RT may result in an estimated IQ difference of an additional 3 points. Furthermore, Park et al. [46] observed that PBT had the greatest benefit in saving the temporal lobe and hippocampus for suprasellar germ cell tumor compared to intensity-modulated photon RT. Greenberger et al. [25] demonstrated no significant decline in neurocognitive function in 32 patients with OPG after PBT. Reduced radiation exposure by intensity-modulated photon RT compared to previous 2D or 3D-RT to the normal brain, especially to the temporal lobe may mitigate the previously reported neurocognitive function impairment (Fig. 1). Importantly, PBT may further reduce radiation to normal brain compared to intensity-modulated photon RT, expecting improved functional outcomes compared old series of OPG patients.
There are limited data on secondary malignancies that stem from RT in patients with OPG. A recent report found that 10-year cumulative incidence of secondary was 8% (11 of 89 patients) with a median interval of 9 years [9]. Considering the location and interval between RT and secondary malignancy, the authors identified 8 of 11 patients with subsequent cancer as true RT-induced malignancies. Several potential mechanisms of second neoplasms could exist, including malignant transformation of low-grade glioma, tumor related to NF-1 mutation, or true RT-effect [474849].
Due to increased beamlets, beam-on time, and monitor units of intensity-modulated photon RT resulting in larger volumes of normal tissue exposure to low-dose radiation than 2D or 3D-RT, some previous reports raised concerns of increased risk of secondary malignancy [50]. However, long-term (11 years) follow-up data from a single center with 325 patients treated with intensity-modulated photon RT revealed that solid secondary malignancy developed in 11 patients and the 10- and 15-year cumulative incidence of secondary malignancy were 1.8% and 3.5%, respectively. Eight among 11 solid secondary malignancies developed within the intensity-modulated photon RT field, 1 within the intermediate-dose area, and only 1 was out-of-field [51]. Solid secondary malignancies included high-grade glioma (n= 3), osteosarcoma (n=3), colon cancer (n=2), thyroid cancer (n= 1), presumed sarcoma (n=1), and unknown primary (n=1). They found that most solid secondary malignancies developed in the high-dose area supporting the safety of intensity-modulated photon RT. In regard to PBT, scattering foil for field size adjustment could produce out-of-field neutron dissemination resulting in secondary ionizing radiation which could increase second malignancies compared to X-ray [50]. However, Brodin et al. [52] found a reduced risk of radiation-induced subsequent neoplasm compared to 3D-RT or intensity-modulated photon RT based on radio-biologic modeling even when the considering neutron effect. Also, Indelicato et al. [53] reported a 10-year cumulative incidence of 3.1% in 1,713 children treated with PBT and 1.7% in 1,676 children without germline mutation. They observed that all 5 high-grade gliomas (in children without genetic syndrome) developed in the high-dose region same as the intensity-modulated photon RT series [51]. A retrospective cohort study of 558 adult patients treated with PBT showed slightly reduced incidence of secondary neoplasm compared to matched cohort from the Surveillance, Epidemiology, and End Results cancer registry treated with X-rays (5.2% vs. 7.5%) [54]. Long-term follow-up data on PBT for OPG may help physicians to identify the protective effects of PBT compared to X-rays (even intensity-modulated photon RT) in the development of secondary neoplasms.
Although there is a lack of consensus regarding the optimal use of RT, the clinical integration of RT, either X-ray or PBT, has increased. Long-term tumor control rates following RT have shown favorable outcomes. However, RT for OPG is related to several late toxicities, including psPD, visual disturbance, vasculopathy, endocrinopathy, and neurocognitive decline. In this context, high-conformal RT techniques, such as intensity-modulated photon RT may minimize these late toxic events compared to traditional 2D or 3D-RT. Moreover, PBT may reduce the dose to normal structures compared to X-rays (2D or 3D-RT, and even intensity-modulated photon RT) owing to its physical characteristics. Therefore, comparable tumor control outcomes with reduced late toxicities from PBT (or intensity-modulated photon RT) could revisit the application of timely RT instead of deferring RT for the treatment of OPG.
Notes
Availability of Data and Material
Data sharing not applicable to this article as no datasets were generated or analyzed during the study.
References
1. Jahraus CD, Tarbell NJ. Optic pathway gliomas. Pediatr Blood Cancer. 2006; 46:586–596. PMID: 16411210.
2. Listernick R, Charrow J, Greenwald M, Mets M. Natural history of optic pathway tumors in children with neurofibromatosis type 1: a longitudinal study. J Pediatr. 1994; 125:63–66. PMID: 8021787.
3. Hoffman HJ, Soloniuk DS, Humphreys RP, Drake JM, Becker LE, De Lima BO, et al. Management and outcome of low-grade astrocytomas of the midline in children: a retrospective review. Neurosurgery. 1993; 33:964–971. PMID: 8134009.
4. Oh KS, Hung J, Robertson PL, Garton HJ, Muraszko KM, Sandler HM, et al. Outcomes of multidisciplinary management in pediatric low-grade gliomas. Int J Radiat Oncol Biol Phys. 2011; 81:e481–e488. PMID: 21470783.
5. Raikar SS, Halloran DR, Elliot M, McHugh M, Patel S, Gauvain KM. Outcomes of pediatric low-grade gliomas treated with radiation therapy: a single-institution study. J Pediatr Hematol Oncol. 2014; 36:e366–e370. PMID: 24714505.
6. Yock TI, Tarbell NJ. Technology insight: proton beam radiotherapy for treatment in pediatric brain tumors. Nat Clin Pract Oncol. 2004; 1:97–103. PMID: 16264827.
7. Gondi V, Yock TI, Mehta MP. Proton therapy for paediatric CNS tumours-improving treatment-related outcomes. Nat Rev Neurol. 2016; 12:334–345. PMID: 27197578.
8. Lim DH. Radiation therapy for pediatric brain tumors. J Korean Med Assoc. 2012; 55:447–453.
9. Tsang DS, Murphy ES, Merchant TE. Radiation therapy for optic pathway and hypothalamic low-grade gliomas in children. Int J Radiat Oncol Biol Phys. 2017; 99:642–651. PMID: 29280458.
10. Cherlow JM, Shaw DWW, Margraf LR, Bowers DC, Huang J, Fouladi M, et al. Conformal radiation therapy for pediatric patients with low-grade glioma: results from the children’s oncology group phase 2 study ACNS0221. Int J Radiat Oncol Biol Phys. 2019; 103:861–868. PMID: 30419305.
11. Tao ML, Barnes PD, Billett AL, Leong T, Shrieve DC, Scott RM, et al. Childhood optic chiasm gliomas: radiographic response following radiotherapy and long-term clinical outcome. Int J Radiat Oncol Biol Phys. 1997; 39:579–587. PMID: 9336136.


12. Cappelli C, Grill J, Raquin M, Pierre-Kahn A, Lellouch-Tubiana A, Terrier-Lacombe MJ, et al. Long-term follow up of 69 patients treated for optic pathway tumours before the chemotherapy era. Arch Dis Child. 1998; 79:334–338. PMID: 9875044.
13. Marcus KJ, Goumnerova L, Billett AL, Lavally B, Scott RM, Bishop K, et al. Stereotactic radiotherapy for localized low-grade gliomas in children: final results of a prospective trial. Int J Radiat Oncol Biol Phys. 2005; 61:374–379. PMID: 15667955.
14. Indelicato DJ, Rotondo RL, Uezono H, Sandler ES, Aldana PR, Ranalli NJ, et al. Outcomes following proton therapy for pediatric low-grade glioma. Int J Radiat Oncol Biol Phys. 2019; 104:149–156. PMID: 30684665.
15. Combs SE, Schulz-Ertner D, Moschos D, Thilmann C, Huber PE, Debus J. Fractionated stereotactic radiotherapy of optic pathway gliomas: tolerance and long-term outcome. Int J Radiat Oncol Biol Phys. 2005; 62:814–819. PMID: 15936565.
16. Acharya S, Quesada S, Coca K, Richardson C, Hoehn ME, Chiang J, et al. Long-term visual acuity outcomes after radiation therapy for sporadic optic pathway glioma. J Neurooncol. 2019; 144:603–610. PMID: 31414376.
17. Parvez K, Parvez A, Zadeh G. The diagnosis and treatment of pseudoprogression, radiation necrosis and brain tumor recurrence. Int J Mol Sci. 2014; 15:11832–11846. PMID: 24995696.
18. Ludmir EB, Mahajan A, Paulino AC, Jones JY, Ketonen LM, Su JM, et al. Increased risk of pseudoprogression among pediatric low-grade glioma patients treated with proton versus photon radiotherapy. Neuro Oncol. 2019; 21:686–695. PMID: 30753704.
19. Han Y. Current status of proton therapy techniques for lung cancer. Radiat Oncol J. 2019; 37:232–248. PMID: 31918460.
20. Wan MJ, Ullrich NJ, Manley PE, Kieran MW, Goumnerova LC, Heidary G. Long-term visual outcomes of optic pathway gliomas in pediatric patients without neurofibromatosis type 1. J Neurooncol. 2016; 129:173–178. PMID: 27311725.
21. Avery RA, Hardy KK. Vision specific quality of life in children with optic pathway gliomas. J Neurooncol. 2014; 116:341–347. PMID: 24197987.
22. Shofty B, Mauda-Havakuk M, Weizman L, Constantini S, Ben-Bashat D, Dvir R, et al. The effect of chemotherapy on optic pathway gliomas and their sub-components: a volumetric MR analysis study. Pediatr Blood Cancer. 2015; 62:1353–1359. PMID: 25858021.
23. Kelly JP, Weiss AH. Detection of tumor progression in optic pathway glioma with and without neurofibromatosis type 1. Neuro Oncol. 2013; 15:1560–1567. PMID: 24101736.
24. Grabenbauer GG, Schuchardt U, Buchfelder M, Rödel CM, Gusek G, Marx M, et al. Radiation therapy of optico-hypothalamic gliomas (OHG)--radiographic response, vision and late toxicity. Radiother Oncol. 2000; 54:239–245. PMID: 10738082.
25. Greenberger BA, Pulsifer MB, Ebb DH, MacDonald SM, Jones RM, Butler WE, et al. Clinical outcomes and late endocrine, neurocognitive, and visual profiles of proton radiation for pediatric low-grade gliomas. Int J Radiat Oncol Biol Phys. 2014; 89:1060–1068. PMID: 25035209.
26. Hanania AN, Paulino AC, Ludmir EB, Shah VS, Su JM, McGovern SL, et al. Early radiotherapy preserves vision in sporadic optic pathway glioma. Cancer. 2021; 127:2358–2367. PMID: 33739455.
27. Awdeh RM, Kiehna EN, Drewry RD, Kerr NC, Haik BG, Wu S, et al. Visual outcomes in pediatric optic pathway glioma after conformal radiation therapy. Int J Radiat Oncol Biol Phys. 2012; 84:46–51. PMID: 22607912.
28. Mueller S, Fullerton HJ, Stratton K, Leisenring W, Weathers RE, Stovall M, et al. Radiation, atherosclerotic risk factors, and stroke risk in survivors of pediatric cancer: a report from the Childhood Cancer Survivor Study. Int J Radiat Oncol Biol Phys. 2013; 86:649–655. PMID: 23680033.
29. Bavle A, Srinivasan A, Choudhry F, Anderson M, Confer M, Simpson H, et al. Systematic review of the incidence and risk factors for cerebral vasculopathy and stroke after cranial proton and photon radiation for childhood brain tumors. Neurooncol Pract. 2021; 8:31–39. PMID: 33664967.
30. Merchant TE, Kun LE, Wu S, Xiong X, Sanford RA, Boop FA. Phase II trial of conformal radiation therapy for pediatric low-grade glioma. J Clin Oncol. 2009; 27:3598–3604. PMID: 19581536.
31. Kestle JR, Hoffman HJ, Mock AR. Moyamoya phenomenon after radiation for optic glioma. J Neurosurg. 1993; 79:32–35. PMID: 8315466.
32. Mueller S, Sear K, Hills NK, Chettout N, Afghani S, Gastelum E, et al. Risk of first and recurrent stroke in childhood cancer survivors treated with cranial and cervical radiation therapy. Int J Radiat Oncol Biol Phys. 2013; 86:643–648. PMID: 23623405.


33. El-Fayech C, Haddy N, Allodji RS, Veres C, Diop F, Kahlouche A, et al. Cerebrovascular diseases in childhood cancer survivors: role of the radiation dose to Willis circle arteries. Int J Radiat Oncol Biol Phys. 2017; 97:278–286. PMID: 28068236.
34. Campen CJ, Kranick SM, Kasner SE, Kessler SK, Zimmerman RA, Lustig R, et al. Cranial irradiation increases risk of stroke in pediatric brain tumor survivors. Stroke. 2012; 43:3035–3040. PMID: 22968468.


35. Bowers DC, Mulne AF, Reisch JS, Elterman RD, Munoz L, Booth T, et al. Nonperioperative strokes in children with central nervous system tumors. Cancer. 2002; 94:1094–1101. PMID: 11920480.


36. Lee KA, O’Sullivan C, Daly P, Pears J, Owens C, Timmermann B, et al. Proton therapy in paediatric oncology: an Irish perspective. Ir J Med Sci. 2017; 186:577–582. PMID: 27744643.


37. Kralik SF, Watson GA, Shih CS, Ho CY, Finke W, Buchsbaum J. Radiation-induced large vessel cerebral vasculopathy in pediatric patients with brain tumors treated with proton radiation therapy. Int J Radiat Oncol Biol Phys. 2017; 99:817–824. PMID: 28867358.


38. Hall MD, Bradley JA, Rotondo RL, Hanel R, Shah C, Morris CG, et al. Risk of radiation vasculopathy and stroke in pediatric patients treated with proton therapy for brain and skull base tumors. Int J Radiat Oncol Biol Phys. 2018; 101:854–859. PMID: 29730064.


39. Brauner R, Malandry F, Rappaport R, Zucker JM, Kalifa C, Pierre-Kahn A, et al. Growth and endocrine disorders in optic glioma. Eur J Pediatr. 1990; 149:825–828. PMID: 2226566.


40. Lacaze E, Kieffer V, Streri A, Lorenzi C, Gentaz E, Habrand JL, et al. Neuropsychological outcome in children with optic pathway tumours when first-line treatment is chemotherapy. Br J Cancer. 2003; 89:2038–2044. PMID: 14647135.


41. Papini C, Dineen RA, Walker DA, Thomas S, Pitchford NJ. Neuropsychological outcomes of children with optic pathway glioma. Sci Rep. 2020; 10:3344. PMID: 32094393.


42. Janss AJ, Grundy R, Cnaan A, Savino PJ, Packer RJ, Zackai EH, et al. Optic pathway and hypothalamic/chiasmatic gliomas in children younger than age 5 years with a 6-year follow-up. Cancer. 1995; 75:1051–1059. PMID: 7842408.


43. Jalali R, Mallick I, Dutta D, Goswami S, Gupta T, Munshi A, et al. Factors influencing neurocognitive outcomes in young patients with benign and low-grade brain tumors treated with stereotactic conformal radiotherapy. Int J Radiat Oncol Biol Phys. 2010; 77:974–979. PMID: 19864079.


44. Redmond KJ, Mahone EM, Terezakis S, Ishaq O, Ford E, McNutt T, et al. Association between radiation dose to neuronal progenitor cell niches and temporal lobes and performance on neuropsychological testing in children: a prospective study. Neuro Oncol. 2013; 15:360–369. PMID: 23322748.


45. Qi XS, Stinauer M, Rogers B, Madden JR, Wilkening GN, Liu AK. Potential for improved intelligence quotient using volumetric modulated arc therapy compared with conventional 3-dimensional conformal radiation for whole-ventricular radiation in children. Int J Radiat Oncol Biol Phys. 2012; 84:1206–1211. PMID: 22516805.


46. Park J, Park Y, Lee SU, Kim T, Choi YK, Kim JY. Differential dosimetric benefit of proton beam therapy over intensity modulated radiotherapy for a variety of targets in patients with intracranial germ cell tumors. Radiat Oncol. 2015; 10:135. PMID: 26112360.


47. Rotta JM, de Oliveira MF, Reis RC, Botelho RV. Malignant transformation of low-grade gliomas in patients undergoing adjuvant therapy. Acta Neurol Belg. 2017; 117:235–239. PMID: 27271289.


48. Broniscer A, Baker SJ, West AN, Fraser MM, Proko E, Kocak M, et al. Clinical and molecular characteristics of malignant transformation of low-grade glioma in children. J Clin Oncol. 2007; 25:682–689. PMID: 17308273.


49. Deng MY, Sturm D, Pfaff E, Sill M, Stichel D, Balasubramanian GP, et al. Radiation-induced gliomas represent H3-/IDH-wild type pediatric gliomas with recurrent PDGFRA amplification and loss of CDKN2A/B. Nat Commun. 2021; 12:5530. PMID: 34545083.


50. Hall EJ. Intensity-modulated radiation therapy, protons, and the risk of second cancers. Int J Radiat Oncol Biol Phys. 2006; 65:1–7. PMID: 16618572.


51. Tringale KR, Casey DL, Niyazov G, Lavery JA, Moskowitz C, Friedman DN, et al. Second cancer risk in childhood cancer survivors treated with intensity-modulated radiation therapy: an updated analysis of more than 10 years of follow-up. Pediatr Blood Cancer. 2022; 69:e29600. PMID: 35234340.


52. Brodin NP, Munck Af Rosenschöld P, Aznar MC, Kiil-Berthelsen A, Vogelius IR, Nilsson P, et al. Radiobiological risk estimates of adverse events and secondary cancer for proton and photon radiation therapy of pediatric medulloblastoma. Acta Oncol. 2011; 50:806–816. PMID: 21767178.


53. Indelicato DJ, Bates JE, Mailhot Vega RB, Rotondo RL, Hoppe BS, Morris CG, et al. Second tumor risk in children treated with proton therapy. Pediatr Blood Cancer. 2021; 68:e28941. PMID: 33565257.


54. Chung CS, Yock TI, Nelson K, Xu Y, Keating NL, Tarbell NJ. Incidence of second malignancies among patients treated with proton versus photon radiation. Int J Radiat Oncol Biol Phys. 2013; 87:46–52. PMID: 23778197.


Fig. 1
Plan comparison according to radiation therapy (RT) modality. RT planning using 2-dimensional photon RT (A), 3-diensional conformal photon RT (B), intensity-modulated photon RT (C), and proton beam RT (D). Proton beam have better dose conformity to target area and lower radiation dose distribution to normal brain (e.g., temporal lobe) than photon RT.
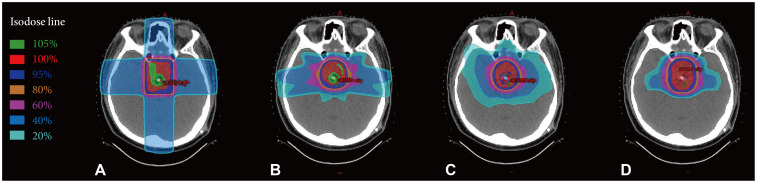
Table 1
Clinical outcomes for OPG following RT
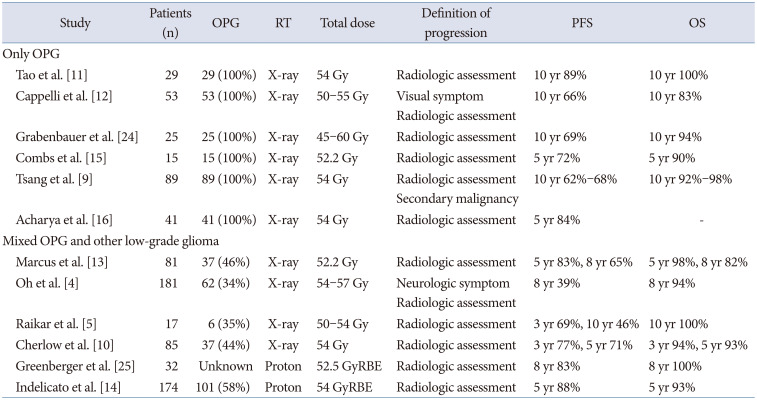
Study | Patients (n) | OPG | RT | Total dose | Definition of progression | PFS | OS | |
---|---|---|---|---|---|---|---|---|
Only OPG | ||||||||
Tao et al. [11] | 29 | 29 (100%) | X-ray | 54 Gy | Radiologic assessment | 10 yr 89% | 10 yr 100% | |
Cappelli et al. [12] | 53 | 53 (100%) | X-ray | 50–55 Gy | Visual symptom | 10 yr 66% | 10 yr 83% | |
Radiologic assessment | ||||||||
Grabenbauer et al. [24] | 25 | 25 (100%) | X-ray | 45–60 Gy | Radiologic assessment | 10 yr 69% | 10 yr 94% | |
Combs et al. [15] | 15 | 15 (100%) | X-ray | 52.2 Gy | Radiologic assessment | 5 yr 72% | 5 yr 90% | |
Tsang et al. [9] | 89 | 89 (100%) | X-ray | 54 Gy | Radiologic assessment | 10 yr 62%–68% | 10 yr 92%–98% | |
Secondary malignancy | ||||||||
Acharya et al. [16] | 41 | 41 (100%) | X-ray | 54 Gy | Radiologic assessment | 5 yr 84% | - | |
Mixed OPG and other low-grade glioma | ||||||||
Marcus et al. [13] | 81 | 37 (46%) | X-ray | 52.2 Gy | Radiologic assessment | 5 yr 83%, 8 yr 65% | 5 yr 98%, 8 yr 82% | |
Oh et al. [4] | 181 | 62 (34%) | X-ray | 54–57 Gy | Neurologic symptom | 8 yr 39% | 8 yr 94% | |
Radiologic assessment | ||||||||
Raikar et al. [5] | 17 | 6 (35%) | X-ray | 50–54 Gy | Radiologic assessment | 3 yr 69%, 10 yr 46% | 10 yr 100% | |
Cherlow et al. [10] | 85 | 37 (44%) | X-ray | 54 Gy | Radiologic assessment | 3 yr 77%, 5 yr 71% | 3 yr 94%, 5 yr 93% | |
Greenberger et al. [25] | 32 | Unknown | Proton | 52.5 GyRBE | Radiologic assessment | 8 yr 83% | 8 yr 100% | |
Indelicato et al. [14] | 174 | 101 (58%) | Proton | 54 GyRBE | Radiologic assessment | 5 yr 88% | 5 yr 93% |
Table 2
Toxicities outcomes following RT
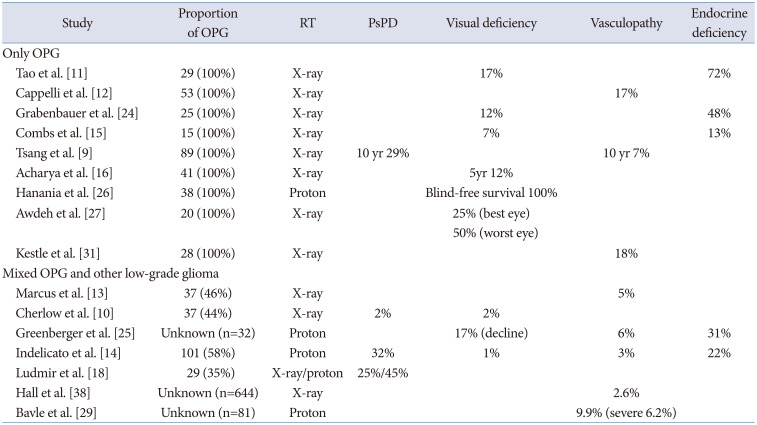
Study | Proportion of OPG | RT | PsPD | Visual deficiency | Vasculopathy | Endocrine deficiency | |
---|---|---|---|---|---|---|---|
Only OPG | |||||||
Tao et al. [11] | 29 (100%) | X-ray | 17% | 72% | |||
Cappelli et al. [12] | 53 (100%) | X-ray | 17% | ||||
Grabenbauer et al. [24] | 25 (100%) | X-ray | 12% | 48% | |||
Combs et al. [15] | 15 (100%) | X-ray | 7% | 13% | |||
Tsang et al. [9] | 89 (100%) | X-ray | 10 yr 29% | 10 yr 7% | |||
Acharya et al. [16] | 41 (100%) | X-ray | 5yr 12% | ||||
Hanania et al. [26] | 38 (100%) | Proton | Blind-free survival 100% | ||||
Awdeh et al. [27] | 20 (100%) | X-ray | 25% (best eye) | ||||
50% (worst eye) | |||||||
Kestle et al. [31] | 28 (100%) | X-ray | 18% | ||||
Mixed OPG and other low-grade glioma | |||||||
Marcus et al. [13] | 37 (46%) | X-ray | 5% | ||||
Cherlow et al. [10] | 37 (44%) | X-ray | 2% | 2% | |||
Greenberger et al. [25] | Unknown (n=32) | Proton | 17% (decline) | 6% | 31% | ||
Indelicato et al. [14] | 101 (58%) | Proton | 32% | 1% | 3% | 22% | |
Ludmir et al. [18] | 29 (35%) | X-ray/proton | 25%/45% | ||||
Hall et al. [38] | Unknown (n=644) | X-ray | 2.6% | ||||
Bavle et al. [29] | Unknown (n=81) | Proton | 9.9% (severe 6.2%) |