Abstract
Objectives
Nicotine is an ingredient of tobacco, and exposure to nicotine increases the risks of various cancers, including oral cancer. Previous studies have focused on the addictive properties of nicotine, but its carcinogenic mechanism has rarely been studied. We aimed to explore the key genes in the process through which nicotine promotes the occurrence and development of oral cancer via data mining and experimental verification.
Methods
This study involved three parts. First, key genes related to nicotine-related oral cancer were screened through data mining; second, the expression and clinical significance of a key gene in oral cancer tissues were verified by bioinformatics. Finally, the expression and clinical significance of the key gene in oral cancer were histologically investigated, and the effects of its expression on cell proliferation, invasion, and drug resistance were cytologically assessed.
Results
SERPINE1 was identified as the key gene, which was upregulated in nicotine-treated oral cells and may be an independent prognostic factor for oral cancer. SERPINE1 was enriched in various pathways, such as the tumor necrosis factor and apelin pathways, and was related to the infiltration of macrophages, CD4+T cells, and CD8+T cells. Overexpression of SERPINE1 was associated with N staging and may be involved in hypoxia, angiogenesis, and metastasis. Knockdown of SERPINE1 in oral cancer cells resulted in weakened cell proliferation and invasion ability and increased sensitivity to bleomycin and docetaxel.
Oral cancer is a malignant tumor that occurs in the oral cavity and adjacent tissues; it is the most common type of head and neck cancer (HNC), and its incidence is gradually increasing [1]. Despite the combined application of multiple treatment methods, the prognosis is still poor, with a 5-year survival rate of lessthan 50% [1]. The main risk factors for oral cancer are smoking, alcohol consumption, betel nut chewing, and human papillomavirus infection, among which smoking is an established factor [2]. Smoking affects human health in several ways and leads to the development of chronic diseases, such as cardiovascular disease and cancer [3].
Nicotine, the main active and addictive ingredient in tobacco (chemical formula C10H14N2), is an alkaloid found in the Nicotiana tabacum plant and is highly toxic [4]. Research has focused on its addictive properties, but rarely on its carcinogenic properties [5]. However, studies have shown that the possible carcinogenic effect of nicotine cannot be ignored. For example, nicotine may promote the progression of various cancers by acting on nicotinic choline receptors [6]. The activation of nicotinic acetylcholine receptors may stimulate cell proliferation and inhibit apoptosis, thus leading to tumorigenesis [7]. Moreover, nicotine exposure promotes cell metastasis and confers drug resistance in HNC [8]. Nevertheless, the carcinogenic mechanism of nicotine is very complex and still unclear.
Aberrant expression of some genes may mediate nicotine-induced carcinogenesis. For example, nicotine exposure can elevate the expression of α5 nicotinic acetylcholine receptors and survivin in lung cells, which plays an important role in the occurrence and development of lung adenocarcinoma [9]. Nicotine exposure can also induce the abnormal expression of PRX1 and the genes encoding for its interacting proteins CFL1 and PPP2R1A, thereby promoting the transformation of normal oral cells into cancer cells [10]. However, most of the literature has focused on single genes or pathways, which may lead to biases in our understanding of nicotine’s oncogenic mechanisms. Therefore, it is necessary to comprehensively study the molecular mechanisms of nicotine, which may improve our understanding of oral cancer prevention and treatment.
In this study, we aimed to explore dysregulated genes associated with nicotine-related oral cancer and screen key genes by data mining. Then, the expression and function of the key genes were further verified through big data analysis and experiments.
Datasets regarding nicotine-treated oral cells were retrieved from the Gene Expression Omnibus (GEO) database (https://ncbi.nlm.nih.gov/geo/). The differentially expressed genes (DEGs) between the nicotine-treated experimental and control groups were obtained by using the GEO2R [11]. The cut-off criteria of adjusted P-value <0.05 and log2 |fold-change| >1.5 were used for identification of the DEGs.
Gene Ontology (GO) and the Kyoto Encyclopedia of Genes and Genomes (KEGG) pathway analyses were conducted to annotate the functions of the DEGs. The DAVID database (https://david.ncifcrf.gov) was used. A P<0.05 was considered statistically significant.
To screen for the hub genes/proteins, the DEGs were submitted to STRING (https://cn.string-db.org) for calculation. The genes with a composite score of more than 0.4 were chosen. The Cytoscape was used for further calculation and visualization. Genes were sorted by degree and betweenness, respectively, and the top 15 genes were considered the hub genes. A Venn diagram was used to obtain the intersection of the two gene sets. The GEPIA tool (http://gepia.cancer-pku.cn/) based on The Cancer Genome Atlas (TCGA) database was used for evaluation.
The HNC cohort from the TCGA database was used. The associations of the key gene (SERPINE1) expression with the confounding factors were assessed. Univariate and multivariate Cox regression analysis was applied to verify the prognostic values of SERPINE1 and clinicopathological factors in HNC. The prognostic value and possible functions of SERPINE1 in HNC were also assessed. The GEO database, ProggeneV2 (http://www.progtools.net/gene/), and TNMplot (https://tnmplot.com/analysis/) were used.
The NetworkAnalyst tool (https://networkanalyst.ca) was used to predict the target genes of SERPINE1.
The TIMER (http://timer.cistrome.org/) and CIBERSORT (https://cibersortx.stanford.edu) algorithms were used to investigate whether SERPINE1 expression was related to the tumor immune microenvironment.
The CancerSEA database [12] was used to learn the roles of SERPINE1 in individual HNC cells.
The possible effect of SERPINE1 expression on the drug sensitivity was predicted by using The Genomics of Drug Sensitivity in Cancer (GDSC) database and the Gene Set Cancer Analysis [13] was used to help visualize the results.
The experiment was approved by the Ethics Committee of Guizhou Medical University. The tissue microarray was commercially purchased, and the consent of the patients had been obtained when collecting samples.
A tissue microarray of oral cancer patients (HOraC060PG01) was purchased from Shanghai Outdo Biotech Company. The characteristic of the patients was described previously [14]. SERPINE1 protein expression was measured by immunohistochemistry (IHC) assay. IHC staining was performed according to the manufacturer’s instructions. Protein staining intensity was scored from 0 to 3, with 0 indicating negative, 1 weak, 2 moderate, and 3 strong. The percentage of positively stained cells was scored from 0 to 4, where 1 represents (1%–25%), 2 (26%–50%), 3 (51%–75%), and 4 (76%–100%). Samples were scored as the product of percent staining and staining intensity, ranging from 0 to 12. A score of not less than 6 was considered “high” and the rest was considered “low.”
CAL27, SAS, HSC-3 (oral cancer cell lines), and DOK (human dysplastic oral keratinocyte) from American Type Culture Collection were stored in the laboratory. Cells were cultured in Dulbecco’s modified Eagle medium containing 10% fetal bovine serum at 37 °C in a humidified atmosphere containing 5% CO2. DOK was intermittently treated with a low dose of Nicotine (1 µM) for 6 months according to the reference [15]. The treated cells were named DOK/NIC.
SERPINE1 was overexpressed in oral cancer tissues. Thus, the loss-of-function strategy was used to explore its roles. The RNA interference technique was utilized. Stable SERPINE1-knockdown cell lines were established according to the methods described recently [14]. Two cancer cell lines, CAL27 and SAS, were chosen for further exploration. The cells with stable SERPINE1-silence were named sh-SERPINE1-CAL27 and sh-SERPINE1-SAS, whereas the relevant controls were named sh-NCCAL27 and sh-NC-SAS, respectively.
The mRNA and protein expressions of SERPINE1 in these cells were detected by qRT-PCR and Western blot assays (anti-PAI1 rabbit monoclonal antibody; Abcam). The cell proliferation was assayed by CCK-8 and colony formation test. The invasive abilities of the cells were evaluated by transwell invasion assay.
For continuous variables, differences between groups were assessed using t-tests, analysis of variance, or Wilcoxon rank-sum tests, depending on the specific type of data. If ratio comparisons were involved, the chi-square test was chosen. Overall survival curves were calculated using the Kaplan-Meier method, and differences in survival rates were determined using the log-rank test. Multivariate Cox regression analysis was performed when multiple possible clinical factors were considered. A P-value or false discovery rate (FDR) of less than 0.05 was considered statistically significant. The statistical analyses were performed using GraphPad Prism 5 (GraphPad Software Inc.)
GSE89923 [16], a gene expression profile that met the inclusion criteria, was identified. The dataset was retrieved and downloaded from the GEO database. The dataset contained six samples of normal human oral cells exposed to nicotine and six samples of control cells. They were analyzed on the GPL570 platform of Affymetrix Human Genome U133 Plus 2.0 Array. The DEGs were screened from a comparison of the two groups. Finally, 123 upregulated genes and 109 downregulated genes were identified through screening (Fig. 1A).
We performed a GO analysis to investigate the possible biological processes of the DEGs. The DEGs were enriched in hundreds of terms. Fig. 1B lists the top 20 terms, such as cell adhesion, epidermal development, neutrophil chemotaxis, epithelial cell differentiation, and neutrophil chemotaxis. KEGG analysis showed the pathway terms. Fig. 1C lists the top 20 terms, including pathways in cancer, interleukin (IL)-17 signaling pathway, tumor necrosis factor (TNF) signaling pathway, transcriptional misregulation in cancer, transforming growth factor (TGF)-β signaling pathway, and nuclear factor (NF)-kappa B signaling pathway.
The interactions of the DEGs were further explored using the STRING and Cytoscape software. In the network, genes were sorted by their degree and betweenness values (Fig. 1D and E, Table 1). The top 15 hub genes were selected separately for Venn analysis (Fig. 1F). The intersection contained eight genes: EGFR, CDH1, CXCL8, S100A7, SERPINE1, LCN2, EP300, and P13. The prognostic values of these genes in HNC were evaluated using the GEPIA tool. SERPINE1 was eventually identified as a key gene due to its possible effect on the prognosis of HNC patients and its high expression in HNC tissues (Table 1).
The TIMER database was used to analyze the expression of SERPINE1 in pan-cancer. As displayed in Fig. 2A, the expression of SERPINE1 was significantly higher in HNC, BRCA, COAD, ESCA, KIRC, READ, and other tumor tissues that in normal tissues, respectively. Next, survival curves based on the TCGA and GSE68585 data, respectively, showed that the overall survival time of the HNC patients bearing high SERPINE1 expression was shorter than that of patients harboring low SERPINE1 expression (Fig. 2B and C).
We evaluated the relationship between SERPINE1 expression and clinicopathological features in HNC. As shown in Table 2, the expression of SERPINE1 was significantly associated with the N-stage and the number of metastatic lymph nodes (P<0.05), indicating that SERPINE1 expression might have a correlation with lymph node metastasis (LNM). Multivariate Cox analysis of different variables in HNC patients revealed that SERPINE1 expression level was an independent prognostic factor for HNC (Fig. 2D). A plot from the TNMplot tool showed that high SERPINE1 expression might have a relationship with enhanced metastatic ability in oral cancer cells (Fig. 2E).
SERPINE1 may closely interact with 57 genes (Fig. 2F). A GO analysis and a KEGG pathway enrichment analysis were also conducted. The top GO terms included metabolic processes, growth, biological regulation, developmental processes, cellular processes, immune system processes, and cellular component tissue biogenesis (Fig. 2G). The KEGG terms mainly involved transcriptional misregulation in cancer, TGF-β signaling pathway, apelin signaling pathway, cell cycle, and thyroid hormone signaling pathway (Fig. 2H).
Evidence indicates that immune cells play an important role in tumor development, metastasis, and drug resistance [17]. We further explored the relationship between SERPINE1 and tumor immune cells. Different algorithms were used to calculate the relationship between the SERPINE1 expression levels and the infiltration of different immune cells.
As shown in Fig. 3A, significant associations were found between SERPINE1 expression and the infiltration levels of dendritic cells, macrophages, CD4+T cells, and CD8+T cells (P<0.05). The CIBERSORT algorithm showed that SERPINE1 expression might correlate with the infiltration levels of CD4+T cells, natural killer T cells, and macrophages in HNC samples (Fig. 3B). The above results confirmed that SERPINE1 might affect the infiltration of immune cells in the cancer microenvironment.
To further elucidate the possible roles of SERPINE1 in monocytes in HNC, the single-cell database CancerSEA was selected for assessment [12]. The results showed that SERPINE1 was positively correlated with metastasis (r=0.50, P<0.05), angiogenesis (r=0.35, P<0.05), hypoxia (r=0.42, P<0.05), and the epithelialmesenchymal transition (EMT; r=0.36, P<0.05) (Fig. 3C).
To predict the possible effect of SERPINE1 expression on the chemosensitivity of cancer cells, data from the GDSC database were evaluated. As shown in Fig. 3D, SERPINE1 expression was negatively correlated with the sensitivity of cancer cells to several agents, such as 17-AAG, bleomycin, and CHIR-99021. Among these drugs, bleomycin (r=–0.347, FDR<0.01) and docetaxel (r=–0.346, FDR<0.01) were clinically used for the treatment of oral cancer. Thus, they were chosen for further validation.
The mRNA expression of SERPINE1 was detected in nicotine-exposed oral cells and oral cancer cells. The results showed that SERPINE1 mRNA expression was significantly higher in DOK/NIC and oral cancer cell lines than in the control cells (Fig. 4A), suggesting that treatment with nicotine might upregulate SERPINE1 expression in oral cells and that SERPINE1 might be an oncogene for oral carcinoma.
A tissue microarray comprising oral cancer samples was used for detection. Fig. 4B showed that SERPINE1 protein expression was significantly higher than in oral cancer samples than in controls (P<0.05). SERPINE1 expression was markedly higher in samples with LNM than in samples without LNM (P<0.05) (Fig. 4C). No associations were identified in comparisons regarding age, sex, and the T-stages (Fig. 4D-G).
Both the mRNA and protein expression levels of SERPINE1 in the SERPINE1-silenced cells were markedly lower than in the control groups (P<0.05) (Fig. 5A and B). The cell proliferation assays showed that the cell viability (Fig. 5C) and colony formation capability (Fig. 5D) were significantly weakened in the SERPINE1-knockdown cells (sh-SERPINE1-CAL27 and sh-SERPINE1-SAS) relative to the controls (P<0.05). Moreover, the invasive ability of the SERPINE1-knockdown cells was significantly lower than that of the control cells (P<0.05) (Fig. 5E). The results implied that the overexpression of SERPINE1 might correlate with enhanced cell proliferation and invasive abilities of oral cancer cells.
To preliminarily verify the effect of SERPINE1 expression on the chemosensitivity of oral cancer cells, cells were treated with 10 μg/mL bleomycin and 10 nM docetaxel for 48 hours, respectively. The results showed that the cell viability of SERPINE1-silenced cells was significantly lower than that of the controls (P<0.05) (Fig. 5F), indicating that the overexpression of SERPINE1 might confer chemoresistance of oral cancer cells to chemotherapy drugs.
In the present study, SERPINE1 was identified as a key gene that might be involved in nicotine-induced oral carcinogenesis and cancer progression. The overexpression of SERPINE1 in oral cancer tissues might be correlated with LNM, which predicts poor prognosis. Thus, the downregulation of SERPINE1 in oral cancer cells resulted in weakened cell proliferation cell invasion abilities. Moreover, SERPINE1 silencing resulted in increased cell sensitivity to bleomycin and docetaxel.
SERPINE1, a plasminogen activator inhibitor (PAI-1) protein-coding gene, is located in the long arm of chromosome 7 (7q21.3-q22) and encodes the SERPINE1 protein, a member of the serine protease inhibitor (serpin) superfamily, which rapidly inhibits fibrinogenesis and mediates a variety of pathological processes such as inflammation and cancer [18]. SERPINE1 is highly expressed in a variety of tumor tissues [19]. This evidence is substantially in line with the results of the present study that SERPINE1 was overexpressed in oral cancer cells and was linked with poor clinical outcomes in patients.
In silico analyses showed that SERPINE1 expression might be associated with LNM in oral cancer. Single-cell functional analysis indicated that SERPINE1 expression was positively correlated with hypoxia, the EMT, angiogenesis, and metastasis. The occurrence of LNM in oral cancer patients usually predicts a poor prognosis [20]. Evidence has shown that hypoxia might result in the infiltration of relevant cells, including immune cells, in cancer tissues, which facilitates angiogenesis and thus expedites cancer metastasis [21]. In addition, hypoxia can induce the expression of relevant genes, and then stimulate cells to undergo the EMT through factors such as FGF1, thereby promoting angiogenesis [22]. Thus, there seems to be an inseparable relationship among hypoxia, EMT, and angiogenesis, which together promote the invasion and metastasis of cancer cells. Studies showed that a hypoxic environment can induce high expression of SERPINE1, which in turn promotes angiogenesis by activation of the EMT process, enhancing the proliferation, invasion, and invasion abilities of cancer cells [23,24]. In the present study, after silencing SERPINE1 expression in oral cancer cells, we observed significantly weakened cell proliferation and invasion ability. This result suggests that SERPINE1 expression enhances the malignant properties of oral cancer cells, while targeting SERPINE1 may significantly reverse the malignant phenotype of the cancer cells.
It is worth noting that SERPINE1 expression was predicted to have an association with the sensitivity of cancer cells to a series of drugs. Among the significantly related drugs, bleomycin and docetaxel have been applied in the clinical treatment of HNC, and therefore they were selected for further verification. Bleomycin, which has been used in the treatment of HNC, can exert cytotoxic effects in cancer cells by inducing reactive oxygen species production; however, the aberrant expression of some proteins, such as coenzyme Q10, can increase the resistance of oral cancer cells to bleomycin [25]. Docetaxel has been used in first-line chemotherapy for HNC, as well as in the treatment of recurrent and metastatic HNC. Likewise, the emergence of resistance to docetaxel presents a challenge for the treatment of oral cancer [26]. The results of the present study showed that after silencing SERPINE1 in oral cancer cells, the sensitivity of the cells to both bleomycin and docetaxel significantly increased, suggesting that high expression of SERPINE1 may reduce the sensitivity of cancer cells to chemotherapy drugs.
To explore the carcinogenic mechanism of nicotine, a functional enrichment analysis of the DEGs related to nicotine-associated carcinogenesis was performed. These genes are enriched in many cancer-related signaling pathways, such as the IL-17 signaling pathway, TNF signaling pathway, and TGF-β signaling pathway. These pathways may play important roles in tumorigenesis and development. For instance, IL-17 promotes tumorigenesis by regulating beclin-1 ubiquitination [27]. The TNF signaling pathway mediates the mesenchymal transition of glioblastoma and plays a key role in its progression [28]. The data showed that several signaling pathways with different functions might be involved in the occurrence and development of nicotine-related oral cancer. The genes closely related to SERPINE1 were also functionally annotated and found to be enriched in multiple signaling pathways. These pathways were scattered across various aspects of cellular functions, and they were not observed to be concentrated in any area. The data suggest that SERPINE1 may exert biological activities in tumorigenesis and development through multiple different signaling pathways. However, its specific mechanism needs further experimental verification.
We noted that SERPINE1 expression in oral cancer may be associated with the infiltration of immune cells, including dendritic cells, macrophages, CD4+T cells, and CD8+T cells. The immune microenvironment was involved in the occurrence and progression of cancer. In general, CD8+T-cell infiltration indicates a better prognosis in several tumors [29], while overexpression of SERPINE1 predicts a poor prognosis. Nevertheless, a positive correlation between CD8+T and SERPINE1 expression was observed. The discrepancy might be because SERPINE1 promotes the infiltration of CD8+T cells as only part of its effect, and the influence of this partial effect on the overall prognosis was not sufficient to counteract its other effects (such as the infiltration of tumor-associated macrophages) that may be detrimental to the prognosis. Therefore, the factors influencing tumor prognosis should be comprehensively analyzed. These results suggest that there may be a correlation between SERPINE1 expression and the infiltration of various immune cells. However, the mechanism by which SERPINE1 affects immune cell infiltration is unclear and needs to be further clarified in future experimental studies.
Several limitations might exist in the present study. First, the experimental validation only involved in vitro assays. The roles of the genes were not verified in vivo. Second, the study focused on the effect of nicotine on the oral cavity. Future studies considering the larynx and hypopharynx may enhance our understanding of the carcinogenic effects and mechanisms of nicotine. Third, whether other carcinogens in tobacco such as benzopyrene and nitric oxide can also cause alterations in SERPINE1 expression is uncertain and was not addressed in this study. Future studies need to explore this issue because it would help to deepen our understanding of the mechanism of nicotine carcinogenesis. Fourth, although this study explored the correlation between nicotine exposure and SERPINE1 expression through a bioinformatics analysis and cytological verification, the causal relationship between them remains insufficiently understood, and the mechanism through which nicotine regulates SERPINE1 expression is still unclear. These problems should be taken into account in future experiments.
Despite its limitations, the present study has revealed that SERPINE1 might be a key gene that plays a crucial role in the genesis and development of nicotine-related oral cancer, raising the possibility that SERPINE1 could serve as a prognostic factor and a therapeutic target for oral carcinoma. Future experiments are needed to explore the molecular mechanisms in-depth.
ACKNOWLEDGMENTS
This study was partially supported by the Guizhou Science and Technology Project (ZK2022-044), and the Cultivation project of Affiliated Hospital of Guizhou Medical University (I-2020-10 and gyfybsky-2021-60).
REFERENCES
1. Rivera C. Essentials of oral cancer. Int J Clin Exp Pathol. 2015; Sep. 8(9):11884–94.
2. Auperin A. Epidemiology of head and neck cancers: an update. Curr Opin Oncol. 2020; May. 32(3):178–86.


3. Onor IO, Stirling DL, Williams SR, Bediako D, Borghol A, Harris MB, et al. Clinical effects of cigarette smoking: epidemiologic impact and review of pharmacotherapy options. Int J Environ Res Public Health. 2017; Sep. 14(10):1147.


4. Alkhatib R, Alkhatib B, Abdo N. Impact of exogenous nicotine on the morphological, physio-biochemical, and anatomical characteristics in Capsicum annuum. Int J Phytoremediation. 2022; 24(6):666–74.
5. Murphy SE. Biochemistry of nicotine metabolism and its relevance to lung cancer. J Biol Chem. 2021; Jan-Jun. 296:100722.


6. Dang N, Meng X, Song H. Nicotinic acetylcholine receptors and cancer. Biomed Rep. 2016; May. 4(5):515–8.


7. Chen J, Cheuk IW, Shin VY, Kwong A. Acetylcholine receptors: Key players in cancer development. Surg Oncol. 2019; Dec. 31:46–53.


8. Shimizu R, Ibaragi S, Eguchi T, Kuwajima D, Kodama S, Nishioka T, et al. Nicotine promotes lymph node metastasis and cetuximab resistance in head and neck squamous cell carcinoma. Int J Oncol. 2019; Jan. 54(1):283–94.


9. Zhang Y, Sun Y, Jia Y, Zhang Q, Zhu P, Ma X. α5-nAChR and survivin: two potential biological targets in lung adenocarcinoma. J Cell Physiol. 2021; Mar. 236(3):1787–97.


10. Qi M, Li L, Tang X, Lu Y, Wang M, Yang J, et al. Nicotine promotes the development of oral leukoplakia via regulating peroxiredoxin 1 and its binding proteins. Braz J Med Biol Res. 2021; May. 54(9):e10931.


11. Barrett T, Wilhite SE, Ledoux P, Evangelista C, Kim IF, Tomashevsky M, et al. NCBI GEO: archive for functional genomics data sets: update. Nucleic Acids Res. 2013; Jan. 41(Database issue):D991–5.
12. Yuan H, Yan M, Zhang G, Liu W, Deng C, Liao G, et al. CancerSEA: a cancer single-cell state atlas. Nucleic Acids Res. 2019; Jan. 47(D1):D900–8.


13. Li X, Yang A, Wen P, Yuan Y, Xiao Z, Shi H, et al. Nuclear receptor subfamily 3 group c member 2 (NR3C2) is downregulated due to hypermethylation and plays a tumor-suppressive role in colon cancer. Mol Cell Biochem. 2022; Nov. 477(11):2669–79.


14. Feng S, Yuan W, Sun Z, Guo X, Ling J, Chang A, et al. SPP1 as a key gene in the lymph node metastasis and a potential predictor of poor prognosis in head and neck carcinoma. J Oral Pathol Med. 2022; Aug. 51(7):620–9.
15. Chang YW, Singh KP. Nicotine-induced oxidative stress contributes to EMT and stemness during neoplastic transformation through epigenetic modifications in human kidney epithelial cells. Toxicol Appl Pharmacol. 2019; Jul. 374:65–76.


16. Woo S, Gao H, Henderson D, Zacharias W, Liu G, Tran QT, et al. AKR1C1 as a biomarker for differentiating the biological effects of combustible from non-combustible tobacco products. Genes (Basel). 2017; May. 8(5):132.


17. Zhang T, Yu H, Dai X, Zhang X. CMTM6 and CMTM4 as two novel regulators of PD-L1 modulate the tumor microenvironment. Front Immunol. 2022; Jul. 13:971428.


18. Chen TY, Zhou M, Lin MQ, Liang ST, Yan Y, Wang SM, et al. Research progress on the SERPINE1 protein and chronic inflammatory diseases of the upper respiratory tract: a literature review. Int Arch Allergy Immunol. 2021; 182(11):1097–102.


19. Hu B, Chen Z, Wang X, Chen F, Song Z, Cao C. MicroRNA-148a-3p directly targets SERPINE1 to suppress EMT-Mediated colon adenocarcinoma progression. Cancer Manag Res. 2021; Aug. 13:6349–62.


20. Dai Y, Wang Z, Yan E, Li J, Ge H, Xiao N, et al. Development of a novel signature derived from single cell RNA-sequencing for preoperative prediction of lymph node metastasis in head and neck squamous cell carcinoma. Head Neck. 2022; Oct. 44(10):2171–80.
21. Sugiura K, Nakajima S, Kato I, Okubo-Sato M, Nakazawa Y, Mitsudo K, et al. Hypoxia and CD11b+ cell influx are strongly associated with lymph node metastasis of oral cancer. Anticancer Res. 2020; Dec. 40(12):6845–52.


22. Li Jp, Liu YJ, Zeng SH, Gao HJ, Chen YG, Zou X. Identification of COX4I2 as a hypoxia-associated gene acting through FGF1 to promote EMT and angiogenesis in CRC. Cell Mol Biol Lett. 2022; Sep. 27(1):76.
23. Teng B, Xie C, Zhao Y, Zeng Q, Zhan F, Feng Y, et al. Identification of MEDAG and SERPINE1 related to hypoxia in abdominal aortic aneurysm based on weighted gene coexpression network analysis. Front Physiol. 2022; Jul. 13:926508.
24. Yang JD, Ma L, Zhu Z. SERPINE1 as a cancer-promoting gene in gastric adenocarcinoma: facilitates tumour cell proliferation, migration, and invasion by regulating EMT. J Chemother. 2019; Nov-Dec. 31(7-8):408–18.


25. Yen HC, Li SH, Majima HJ, Huang YH, Chen Cp, Liu CC, et al. Upregulation of antioxidant enzymes and coenzyme Q(10) in a human oral cancer cell line with acquired bleomycin resistance. Free Radic Res. 2011; Jun. 45(6):707–16.
26. Cui J, Wang H, Zhang X, Sun X, Zhang J, Ma J. Exosomal miR-200c suppresses chemoresistance of docetaxel in tongue squamous cell carcinoma by suppressing TUBB3 and PPP2R1B. Aging (Albany NY). 2020; Apr. 12(8):6756–73.
27. Bie Q, Song H, Chen X, Yang X, Shi S, Zhang L, et al. IL-17B/IL-17RB signaling cascade contributes to self-renewal and tumorigenesis of cancer stem cells by regulating Beclin-1 ubiquitination. Oncogene. 2021; Mar. 40(12):2200–16.


28. Yan T, Tan Y, Deng G, Sun Z, Liu B, Wang Y, et al. TGF-β induces GBM mesenchymal transition through upregulation of CLDN4 and nuclear translocation to activate TNF-α/NF-κB signal pathway. Cell Death Dis. 2022; Apr. 13(4):339.
29. Maimela NR, Liu S, Zhang Y. Fates of CD8+T cells in tumor microenvironment. Comput Struct Biotechnol J. 2018; Nov. 17:1–13.
Fig. 1.
(A) Heatmap of the differentially expressed genes (DEGs) screened from the GSE89923 dataset. The horizontal axis represents the names of the genes, and the right vertical axis represents the samples. Red represents the upregulated genes and blue represents the downregulated ones. (B, C) Enrichment analysis of the DEGs by Gene Ontology (GO; B) and Kyoto Encyclopedia of Genes and Genomes (KEGG; C). (D, E) The top 15 genes in a protein-protein interaction network of the DEGs are sorted by degree (D) and betweenness (E) values. (F) Venn analysis of the top 15 hub genes ranked by the degree and betweenness values, respectively. The intersection contained eight genes. IL, interleukin; TNF, tumor necrosis factor; TGF, transforming growth factor; NF, nuclear factor.
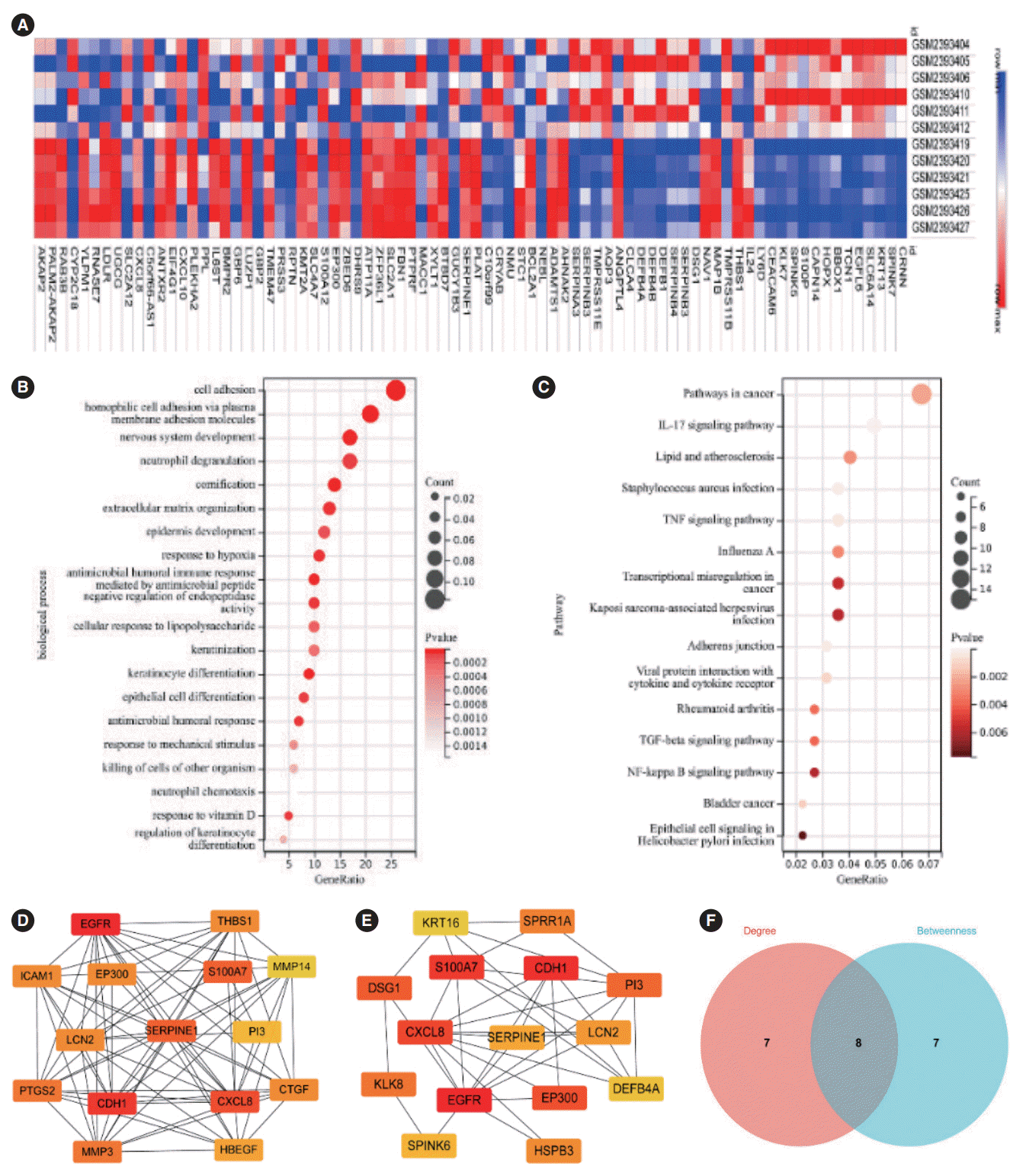
Fig. 2.
(A) The expression of SERPINE1 in pan-cancer based on The Cancer Genome Atlas (TCGA) database. SERPINE1 expression was significantly higher in head and neck cancer (HNC) tissues than in normal tissues (P<0.001). (B, C) The survival curves showed that HNC patients with high SERPINE1 expression had a shorter overall survival time than those with low SERPINE1 expression (P<0.05). (B) TCGA. (C) GSE65858. (D) Multivariate Cox regression analysis with different variables indicated that SERPINE1 was an independent prognostic factor for HNC (P<0.05). (E) TNMplot presented that high expression of SERPINE1 was associated with metastasis (P<0.05). (F) The predicted targets of SERPINE1. The interaction plot showed 57 target genes that may be closely related to SERPINE1. (G, H) Gene Ontology (GO; G) and Kyoto Encyclopedia of Genes and Genomes (KEGG; H) enrichment analyses of the 57 genes. TPM, transcripts per million; HR, hazard ratio; CI, confidence interval; pT, pathological tumor; pN, pathological N; pTNM, pathological tumor-node-metastasis; TGF, transforming growth factor. *P<0.05; **P<0.01; ***P<0.001.
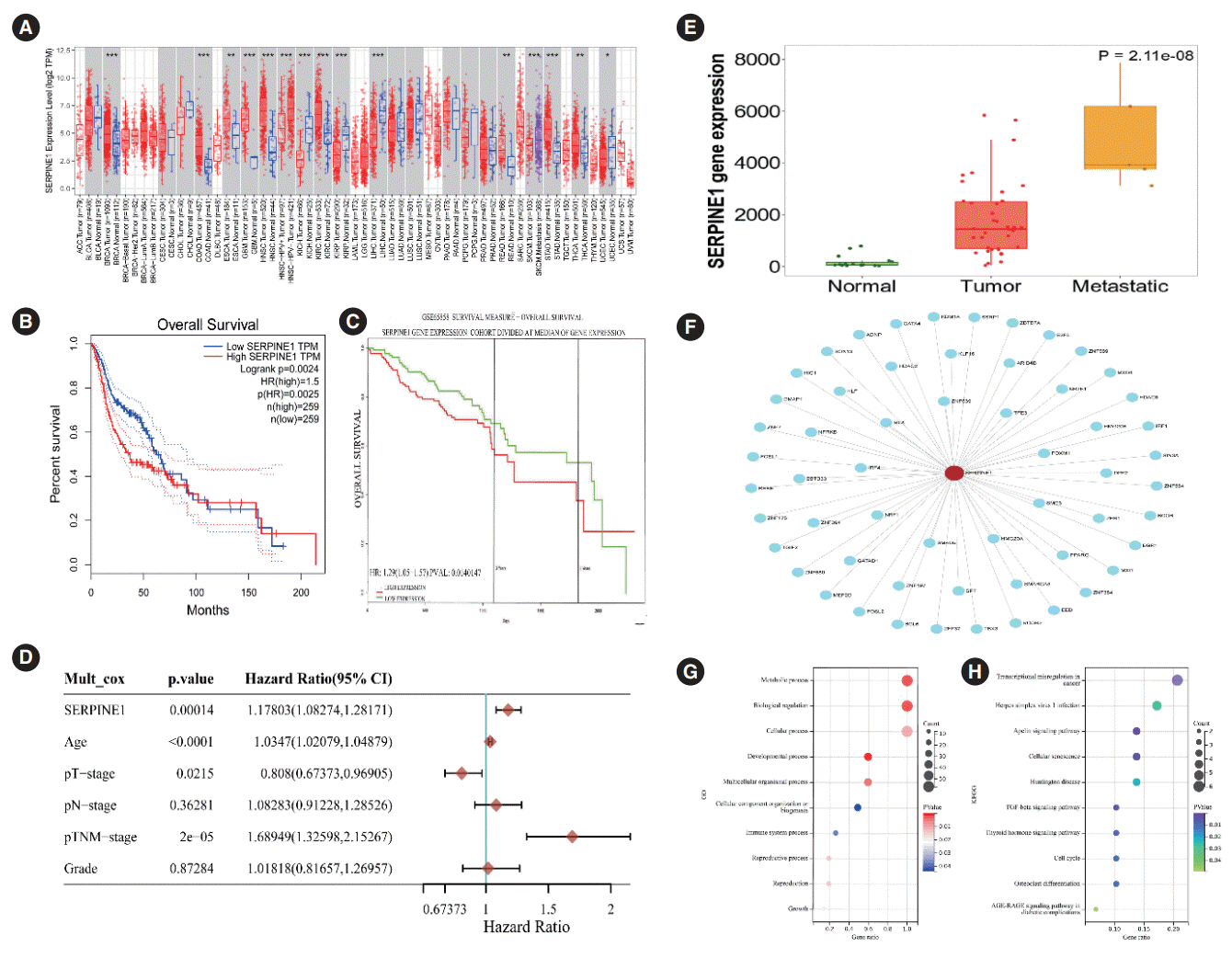
Fig. 3.
(A) The association between SERPINE1 expression and tumor purity, as well as the infiltration levels of several immune cells (TIMER algorithm). (B) Analysis of the relationship between the expression of SERPINE1 and the infiltration levels of 22 types of immune cells by the Cibersort algorithm. The darker color indicates a higher correlation (*P<0.05). (C) The correlation of SERPINE1 expression with malignant phenotypes in head and neck cancer tissues. Scatter plots showed positive correlations between SERPINE1 expression and malignant phenotypes, such as (C1) metastasis, (C2) hypoxia, (C3) the epithelial-mesenchymal transition, and (C4) angiogenesis. (D) The relationship between SERPINE1 expression and the drug sensitivity of cancer cells. Red represents a positive correlation, while blue stands for a negative correlation. TPM, transcripts per million; TCGA, The Cancer Genome Atlas; HNSC, Head and Neck squamous cell carcinoma; NK, natural killer; EMT, epithelial-mesenchymal transition; GDSC, Genomics of Drug Sensitivity in Cancer; mRNA, messenger RNA; FDR, false discovery rate.
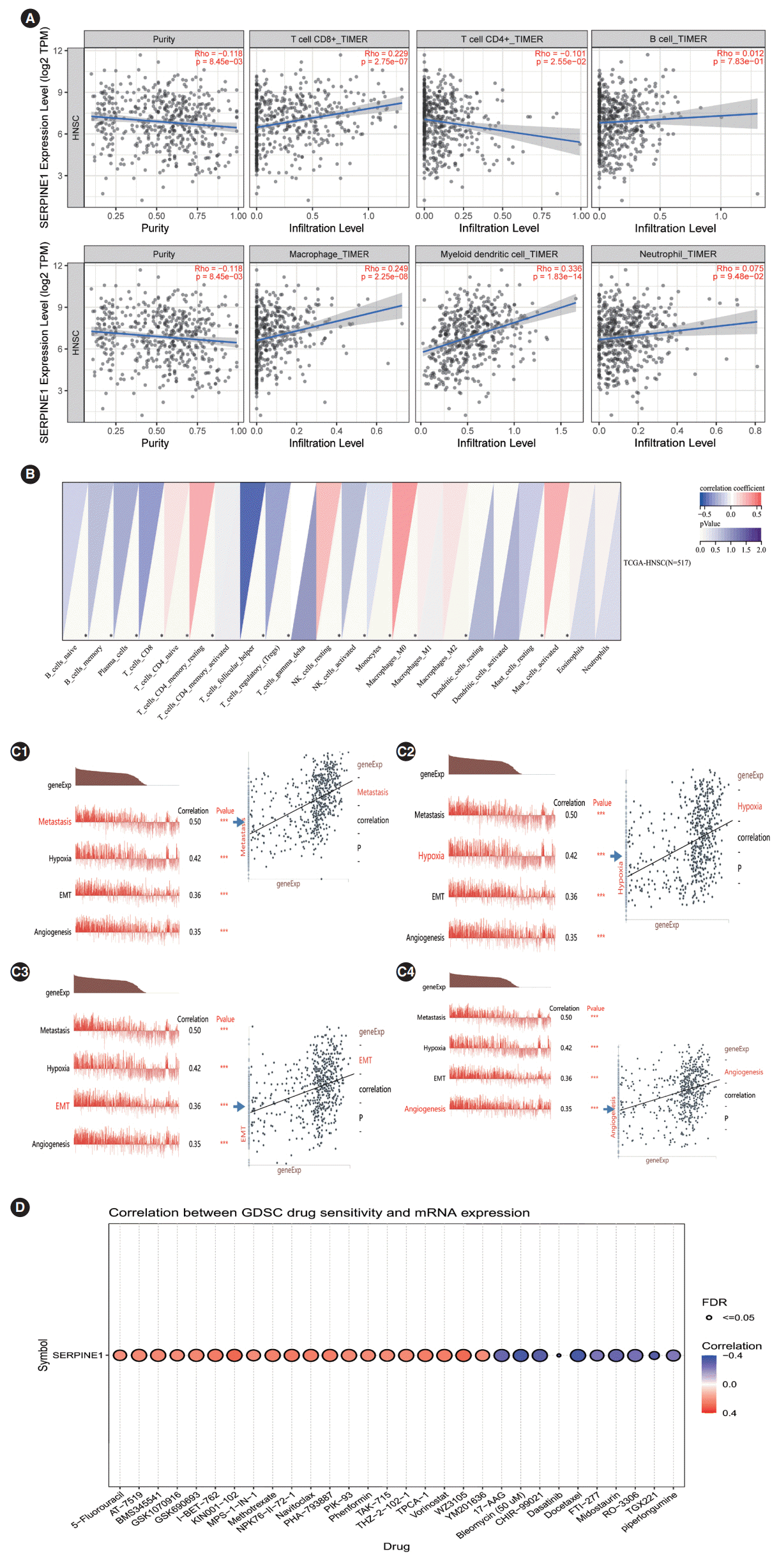
Fig. 4.
(A) The messenger RNA (mRNA) expression of SERPINE1 was higher in nicotine-treated oral cells (DOK/NIC) and oral cancer cell lines (Cal27, SAS, HSC-3) than that in DOK cells, respectively. (B) The immunohistochemistry scores of SERPINE1 protein expression in oral cancer tissues were markedly higher than in the normal controls. (C) The expression scores for SERPINE1 protein were higher in cancer samples with lymph node metastasis (LNM) than in those without LNM. (D) The scores of SERPINE1 expression were higher in the samples with high pathological stages than in those with low stages. No associations were presented concerning age (E), sex (F), and Tstage (G). IHC, immunohistochemistry; NS, not significant (P>0.05). *P<0.05.
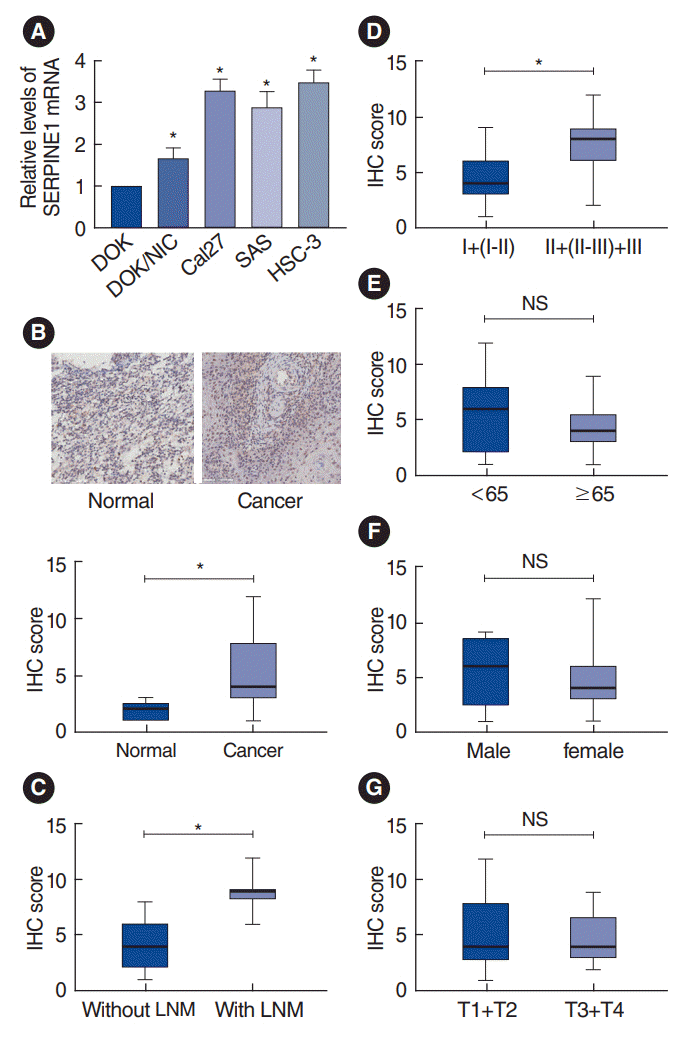
Fig. 5.
(A) The mRNA expression of SERPINE1 was significantly downregulated in the SERPINE1-silenced oral cancer cells (sh-SERPINE1-Cal27 or sh-SERPINE1-SAS) compared with that of the control cells (sh-NC-Cal27 and sh-NC-SAS). (B) The trend of SERPINE1 protein expression was in line with that of mRNA expression. (C) The cell proliferation abilities of the SERPINE1-silenced cancer cells were significantly lower than those of the control cells. (D) The number of colonies formed in the SERPINE1-silenced cells was significantly lower than that in the control cells. (E) The invasive abilities in the SERPINE1-silenced cells were significantly inhibited compared with those in the control cells. (F) The administration of Bleomycin or docetaxel resulted in a significant decrease in cell viability in SERPINE1-silenced cells compared with the control cells. mRNA, messenger RNA; GAPDH, glyceraldehyde-3-phosphate dehydrogenase; NC, negative control; OD, optical density. *P<0.05.
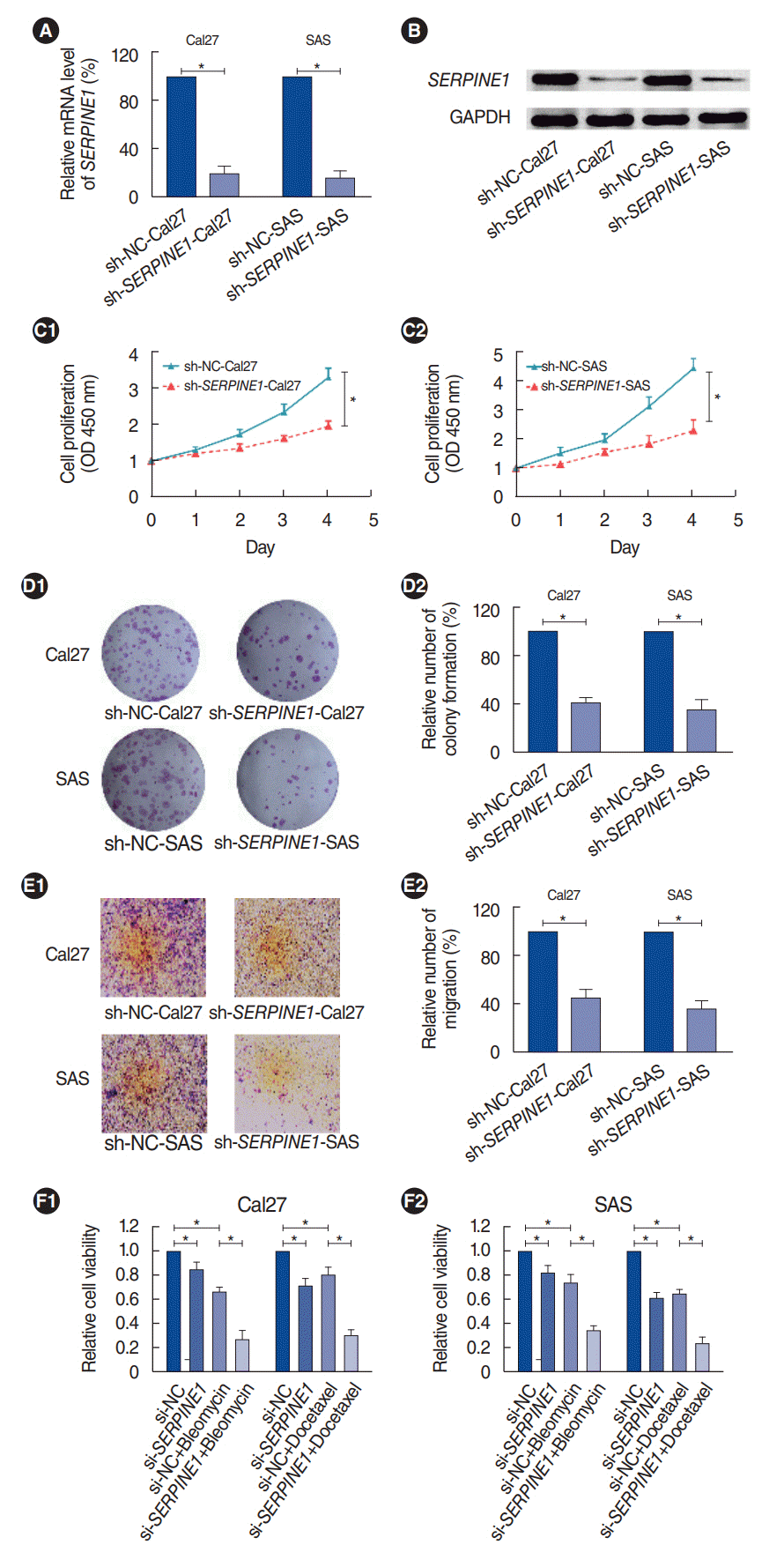
Table 1.
The hub genes in the intersection based on a Venn analysis
Node name | Degree | Closeness | Betweenness | Up/downa) | P (Exp)b) | P (HR)c) |
---|---|---|---|---|---|---|
EGFR | 92 | 92.36667 | 7997.67226 | UP | 1.84E-04 | 0.052 |
CDH1 | 72 | 83.28333 | 3446.40144 | UP | 6.53E-05 | 0.82 |
CXCL8 | 64 | 82.11667 | 2065.93923 | UP | 2.86E-08 | 0.23 |
SERPINE1 | 44 | 72.93333 | 778.08936 | UP | 9.78E-04 | 0.0025 |
S100A7 | 44 | 74.98333 | 2426.04387 | UP | 3.15E-03 | 0.24 |
EP300 | 40 | 72.65 | 1837.0896 | UP | 1.29E-03 | 0.41 |
LCN2 | 40 | 73.81667 | 801.31089 | Down | 1.01E-07 | 0.28 |
PI3 | 36 | 71.48333 | 1090.78971 | UP | 7.06E-07 | 0.63 |
Table 2.
Associations between SERPINE1 expression and clinicopathological factors in oral cancer (TCGA)