Abstract
This study aims to explore the impact of Rehmannioside D (RD) on ovarian functions of rats with diminished ovarian reserve (DOR) and its underlying mechanisms of action. A single injection of cyclophosphamide was performed to establish a DOR rat model, and fourteen days after the injection, the rats were intragastrically administrated with RD for two weeks. Rat estrus cycles were tested using vaginal smears. Ovarian tissues were histologically evaluated, the number of primordial, mature, and atretic follicles was calculated, and the apoptotic rate of granulosa cells. Follicle-stimulating hormone (FSH), luteinizing hormone (LH), and estradiol (E2) levels were determined by ELISA assays. Protein levels of Forkhead Box O1 (FOXO1), KLOTHO, Bcl-2, and Bax were investigated in ovarian tissues of DOR rats. The binding between FOXO1 and KLOTHO was verified by ChIP assay. High-dose administration of RD into DOR rats improved their estrus cycles, increased ovarian index, enhanced the number of primordial and mature follicles, reduced the number of atretic follicle number, and ovarian granulosa cell apoptosis in addition to inhibiting FSH and LH levels and upregulating E2 expression. FOXO1 and KLOTHO were significantly suppressed in DOR rats. FOXO1 knockdown partially suppressed the protective effects of RD on DOR rats, and KLOTHO overexpression could restore RD-induced blockade of DOR development despite knocking down FOXO1. FOXO1 antibody enriched KLOTHO promoter, and the binding between them was reduced in DOR group compared to that in sham group. RD improved ovarian functions in DOR rats and diminished granulosa cell apoptosis via the FOXO1/KLOTHO axis.
Diminished ovarian reserve (DOR) refers to the progressive loss of the number or quality of oocytes with advanced age [1], which essentially reflects female reproductive aging [2]. Pathologic DOR (DOR occurs early or is accelerated) is a threat for female reproductive and general health [3]. In clinical practice, diagnosis of DOR is based upon increased follicle-stimulating hormone (FSH) and luteinizing hormone (LH), low levels of estradiol (E2) and anti-Mullerian hormone (AMH), and decreased antral follicle count [4]. A follicle is comprised of oocytes and two sorts of somatic cells surrounding the oocytes, granulosa and theca cells [5]. Granulosa cell apoptosis has been shown to be disordered in DOR, which has significant consequences on the generation of empty follicles, oocyte retrieval, and the quality of oocytes and embryos [6]. Currently, hormone replacement therapy (HRT) is a commonly used treatment for pathologic DOR, but the long-term outcome of HRT is poor [7]. Although there have been a number of studies on DOR, the optimal treatment for pathologic DOR is still undetermined.
Forkhead Box O (FOXO) family is a cluster of transcription factors that mediate downstream target genes involved in cellular differentiation, growth, survival, and cell cycle [8]. Depletion of FOXO was associated with impaired oocyte maturation and blocked ovarian development [9]. FOXO1 was reported to regulate most of the FSH responsive genes in ovarian granulosa cells [10]. Increased expression of FOXO1 played a positive role in relieving follicle death [11]. KLOTHO is a type of β-glucuronidase engaged in the development and progression of reproductive diseases with anti-aging and antioxidant properties [12]. Decreased KLOTHO expression was found in women with DOR [13], and overexpression of KLOTHO was related to higher blastocyst formation frequency in porcine cell lines [14]. Our bioinformatics prediction with Jasper database indicated that FOXO1 bound to KLOTHO promoter. Previously, KLOTHO enhanced FOXO1-dependent antioxidant activity and thereby inhibited apoptosis in podocytes [15]. However, the function of the FOXO1-KLOTHO axis in DOR is largely elusive.
Rehmanniae radix preparata is an extract processed by wine-steaming the Rehmannia root, and possesses antioxidant, anti-senescence, anti-inflammatory, and neuroprotective features [16]. Kuntai capsule, a type of herb formulas consisting of six traditional Chinese herbs including Rehmanniae radix preparata, demonstrated to exert a protective role in ovarian reserve and fertility in premature ovarian failure [17]. Rehmannioside D (RD) is an iridoid compound that can be isolated from the extracts of Rehmannia glutinosa [18]. Reportedly, RD was implicated in restraining the migration of human umbilical vein endothelial cells, suggesting that it might possess an anti-angiogenesis property [19]. In addition, Zuogui Pill, in which RD is the effective component of its monarch drug rehmannia glutinosa, could significantly reduce the levels of FSH and LH, and promote AMH and endometrial repair in patients with DOR [20]. Above evidence suggests that Rehmanniae radix preparata can serve as a candidate drug for treating DOR. However, the influences of RD on DOR are little to be known. Moreover, a previous study found that catalpol, a prevalent chemical component in rehmannia glutinosa, extends lifespan and increases stress tolerance of Caenorhabditis elegans, partly depending on DAF-16, a FOXO family member [21]. Herein, we sought to investigate the relationship between RD and FOXO1/KLOTHO axis in relieving the progression of DOR.
Specific pathogen-free (SPF) female Sprague–Dawley (SD) rats (8 weeks old) in estrus were purchased. All animal experiments were ratified by the Ethics Committee of Maternal and Child Health Hospital of Jiangxi Province and performed in accordance with guide for the use and care of laboratory animals and related regulations. After acclimation, vaginal smears were prepared using cells exfoliated from rat vagina. Next, a total of 90 female rats with an estrus cycle of 4–5 days were selected and randomly divided into sham, DOR, DOR + RD (19 mg/kg), DOR + RD (38 mg/kg), DOR + RD (76 mg/kg), DOR + RD + sh-NC, DOR + RD + sh-FOXO1, DOR + RD + sh-FOXO1 + LV-NC, DOR + RD + sh-FOXO1 + LV-KLOTHO groups (n = 10 per group).
Rats in the sham group underwent intraperitoneal injection of normal saline (l ml) once. Rats in the model group were induced with DOR by intraperitoneal injection of 90 mg/kg cyclophosphamide (CTX; Beyotime) once [22]. Fourteen days after CTX administration, the DOR rats were gavaged with dimethyl sulfoxide (DMSO; Beyotime) or different doses of RD dissolved in DMSO (low: 19 mg/kg; medium: 38 mg/kg; high: 76 mg/kg) once a day for consecutive 2 weeks [18], during which lentiviral vectors of sh-FOXO1, sh-NC, LV-NC, or LV-KLOTHO (20 μl, virus titer: 109 TU/ml) were additionally injected into both ovaries of the DOR rats according to experimental design [23,24]. Fourteen days after injection of normal saline, sham-operated rats were intragastrically administrated with DMSO.
The survival of rats in each group was observed daily, and the changes in appearance signs, behaviors and activities, mental state, eating, and urine and feces were recorded.
After the last intragastric administration, all rats were fasted (without water deprivation). In the next morning, the rats were anesthetized by intraperitoneal injection of 40 mg/kg sodium pentobarbital, and weighted. Rat blood was collected through cardiac puncture. Both ovaries of the rats were extracted rapidly after cervical dislocation, and adipose tissues around the ovaries was dissected under a microscope. The ovaries were rinsed with normal saline, dried, and weighed. Part of the left ovary was immediately preserved at −80°C for biochemical analysis. The right ovaries were at once fixed in 4% paraformaldehyde solution for histological analysis.
Ovarian index was calculated by the following formula: ovarian index (%) = ovarian wet weight (g) / body weight before euthanasia (g) × 100.
Vaginal smears were collected daily at 9:00 a.m. Glass slides were numbered in advance, and 20 ml of normal saline was pipetted, gently injected into rat vagina, and repeatedly sucked for 3–5 times. The collected solution was then evenly smeared on the glass slides. After drying, the slides were fixed with 95% alcohol for 30 min. After staining with Wright's-Giemsa stain, the slides were washed and dried. The morphology and number of exfoliated cells on each glass slide were visualized under an optical microscope.
Enzyme-linked immunosorbent assay (ELISA) was implemented to measure FSH, LH, and E2 levels. Rat serum samples and ELISA kits for FSH, LH, and E2 were taken out from a refrigerator and equilibrated for 30 min, and the levels of FSH, LH, and E2 were tested according to the manufacturer’s instructions.
Ovarian tissues were fixed with 4% paraformaldehyde solution for 24 h, after which the fixative solution on the tissues was washed away with distilled water. The ovarian tissues underwent conventional dehydration in alcohol gradient (70%, 80%, 90%, 95%, and 100%) for 1 min per time, permeabilization by xylene twice (5 min per time), and paraffin embedding. Paraffin-embedded tissues were sliced into serial sections (5 μm). The sections were heated in an oven at 80°C for 1 h, hydrated by conventional alcohol gradient, permeabilized by xylene, and washed. Next, hematoxylin staining was performed for 4 min. After washing, the sections were immersed in hydrochloric acid alcohol for 10 sec and washed for 5 min. Eosin solution was appended for 2 min of staining. Histopathological changes in the tissues were observed under an optical microscope after the sections were sealed with neutral balsam. The number of primordial, mature, and atretic follicles in the ovaries was calculated.
Paraffin sections (5 μm) of ovarian tissues were dewaxed in xylene and hydrated in alcohol gradient. The sections were stained with a fluorescein-conjugated TUNEL in situ cell death assay kit, and fluorescence images were obtained under an Olympus IX-73 fluorescence microscope (Olympus).
Total RNA from ovarian tissues was extracted using TRIzol (15596026; Invitrogen). A PrimeScript RT reagent kit (RR047A; Takara) was used to convert total RNA into cDNA. A real-time qPCR instrument (ABI7500; ABI) was used for qRT-PCR analyses. The cDNA templates (2 μl) were added with SYBR Mix (9 μl), forward primer (0.5 μl), reverse primer (0.5 μl), and RNase Free dH2O (8 μl), and then amplified (95°C for 10 min, 95°C for 15 sec, and 60°C for 1 min; in a total of 40 cycles). Each sample had three duplicates. Ct value of each well was recorded, and GAPDH served as the internal control. Relative gene expression was calculated by the 2–ΔΔCt method. ΔΔCt = (average Ct target gene in experimental group – average Ct housekeeping gene in experimental group) − (average Ct target gene in control group – average Ct housekeeping gene in control group). Each test was repeated thrice (see in Table 1 for primer sequences).
Ovarian tissues were washed thrice with pre-cooled PBS buffer and added with RIPA lysis buffer (Beyotime). A BCA protein assay kit (Beyotime) was used to evaluate protein concentration, after which the corresponding volume of protein was mixed with loading buffer (Beyotime) and heated in a boiling-water bath for 3 min. Electrophoresis was performed at 80 V for 30 min, and turned to 120 V for additional 1–2 h after bromophenol blue entered separation gels. The protein was blotted onto membranes in an ice-bath at a current of 300 mA for 60 min, after which the membranes were rinsed with washing buffer for 1–2 min and blocked in blocking buffer at room temperature for 60 min or at 4°C overnight. Incubation with anti-KLOTHO (PA5-99961, 1:1,000; Thermo Fisher Scientific), anti-FOXO1 (ab179450, 1:1,000; Abcam), anti-Bcl-2 (ab196495, 1:1,500; Abcam), or anti-Bax (ab32503, 1:1,000; Abcam) was performed on a shaker at room temperature for 1 h. The membrane was washed thrice with washing buffer for 10 min each, incubated with goat anti-rabbit immunoglobulin G (IgG) for 1 h at room temperature, and washed thrice for 10 min each. A chemiluminescence imaging system (Bio-Rad) was used to visualize the immunoblots after developer was added dropwise onto the membranes. All experiments were repeated in triplicate.
ChIP assay was implemented with a SimpleChIP Plus sonication chromatin IP Kit (Cell Signaling Technology) according to the manufacturer's protocol. Rat ovarian tissues were fixed in 1% formaldehyde for DNA-protein crosslinking. Microson Ultrasonic Cell DisruptorXL (Misonix Inc.) was used to sonicate chromatin and fragmented chromatin was incubated with FOXO1 antibody or IgG antibody (negative control) at 4°C overnight. Thereafter, the immunoprecipitate was bound to protein G magnetic beads, and the crosslinking of protein and DNA was reversed and eluted DNA was purified DNA. The enrichment of DNA fragments was measured using qPCR.
GraphPad Prism software version 8.0 (Graph Pad Software Inc.) was used for the statistical analysis. Measuring data were expressed as mean ± standard deviation. For samples with normal distribution, t-test was used for comparisons between two groups; one-way analysis of variance was implemented to compare data among multiple groups, and post-hoc analysis was conducted by Tukey's multiple comparisons test. In terms of samples not conforming to normal distribution, Mann–Whitney U test was used for two independent samples, and statistical analysis for multiple independent samples was performed by Kruskal–Wallis. p < 0.05 was considered to have significant differences.
The DOR rat model was established with CTX, and the rats were gavaged with different doses of RD (low: 19 mg/kg; medium: 38 mg/kg; high: 76 mg/kg) for 2 weeks. None of the rats died at the end of intragastric administration. Rats in the sham group showed normal mental state, physical appearance, behavior and activity, food intake, respiration, as well as shape and color of urine and feces. Poor spirit, lusterless and thin fur, and decreased activities appeared in rats in the DOR group. After 2 weeks of RD treatment, the mental and physical states of the rats were recovered, but the mental state of rats treated with high dose of RD was better than those treated with low and medium doses of RD. Compared to the sham-operated rats, the estrous cycles of rats in the DOR group was disordered and notably prolonged, and rats in the DOR + RD (76 mg/kg) group had shorter and relatively regular estrous cycles compared to rats in the DOR group (Fig. 1A). Two weeks later, the ovarian index of rats with DOR was remarkably increased by treatment with high dose of RD (Fig. 1B). Results of H&E staining revealed that the structures of ovarian cortex and medulla were clear, and the morphology and structure of follicles were intact in the ovaries of sham-operated rats; rat ovaries in the DOR, DOR + RD (19 mg/kg), and DOR + RD (38 mg/kg) groups showed medullary atrophy, cortical hyperplasia, interstitial fibrosis-like changes, reduced thickness of granulosa cell layer, less primordial and mature follicles, and more ovarian atretic follicles. Compared with those in the DOR group, the structures of ovarian tissues gradually recovered, the thickness of granulosa cell layer increased, the number of atresia follicles decreased, and the number of primordial and mature follicles increased in the DOR + RD (76 mg/kg) group after 2 weeks of RD treatment (Fig. 1C). The levels of FSH and LH were obviously higher, but the level of E2 was distinctly lower in the DOR group compared to those in the sham group. On the contrary, FSH and LH were clearly restrained, and E2 was upregulated in the DOR + RD (76 mg/kg) group compared to those in DOR group (Fig. 1D–F). TUNEL staining demonstrated that CTX induced apoptosis of granulosa cells, and high-dose administration of RD inhibited the damage of CTX to ovarian granulosa cells (Fig. 1G). Immunoblots displayed that Bcl-2 evidently decreased while Bax significantly strengthened in ovarian tissues of the DOR group than those in the sham group, and high-dose administration of RD facilitated the expression of Bcl-2, but suppressed the expression of Bax (Fig. 1H). Taken together, rat DOR models were successfully induced by CTX, and treatment with a high dose of RD blocked the decline in ovarian function and granulosa cell apoptosis in CTX-induced rats.
Shown by qRT-PCR and western blotting, FOXO1 expressed at a low level in the DOR group, but it expression was notably strengthened by a high-dose administration of RD for two weeks (Fig. 2A, B). A FOXO1-deficiency DOR rat model was established by ovarian injection with sh-FOXO1 or its negative control, and these rats were administrated with a high dose of RD for 2 weeks. Results of qRT-PCR and western blotting indicated that ovarian FOXO1 expression was dramatically weakened in the DOR + RD + sh-FOXO1 group compared to that in the DOR + RD + sh-NC group (Fig. 2C, D). Rats in the DOR + RD + sh-FOXO1 group showed languid mental state and lackluster fur, as well as prolonged and irregular estrous cycles, compared to those in the DOR + RD + sh-NC group (Fig. 2E). The ovarian index of rats was evidently decreased in the DOR + RD + sh-FOXO1 group than that in the DOR + RD + sh-NC group (Fig. 2F). Results of H&E staining suggested slight medullary atrophy and mild granulosa cell loss in the DOR + RD + sh-NC group (Fig. 2G). Also, the histological staining showed aggravated medullary atrophy in ovarian tissues, thinned granulosa cell layer, increased number of atretic follicles, and less primordial and mature follicles in the DOR + RD + sh-FOXO1 group (Fig. 2G). The expression of FSH and LH was significantly increased and the expression of E2 was remarkably reduced in the DOR + RD + sh-FOXO1 group compared with those in the DOR + RD + sh-NC group (Fig. 2H–J). Ovarian TUNLE staining illustrated that the apoptotic rate in granulosa cells was distinctly higher in the DOR + RD + sh-FOXO1 group than that in the DOR + RD + sh-NC group (Fig. 2K). The results of immunoblots revealed decreased ovarian Bcl-2 expression and increased ovarian Bax level in the DOR + RD + sh-FOXO1 group (Fig. 2L). Collectively, FOXO1 was downregulated in the ovaries of rats with DOR, and RD intervention upregulated the expression of FOXO1 to improve ovarian functions and inhibit granulosa cell apoptosis.
qRT-PCR and western blotting demonstrated that the expression of KLOTHO was significantly downregulated in the DOR group, but remarkably increased after 2 weeks of intervention with a high dose of RD (Fig. 3A, B). Jaspar database predicted that a transcription factor FOXO1 bound to the KLOTHO promoter (Fig. 3C). For further verification, ChIP assay was conducted and displayed that FOXO1 antibody evidently enriched the KLOTHO promoter, and the binding of FOXO1 to the KLOTHO promoter was reduced in the DOR group compared to that in the sham group (Fig. 3D). Results of qRT-PCR and western blotting manifested that KLOTHO level was remarkably decreased in the DOR + RD + sh-FOXO1 group in contrast to that in the DOR + RD + sh-NC group (Fig. 3E, F). All results above illustrated that FOXO1 bound to the KLOTHO promoter to promote KLOTHO transcription.
The impact of KLOTHO on DOR progression was further investigated by injection with sh-FOXO1 and LV-KLOTHO into rat ovaries. qRT-PCR and western blotting indicated that KLOTHO level was notably increased in the DOR + RD + sh-FOXO1 + LV-KLOTHO group than that in the DOR + RD + sh-FOXO1 + LV-NC group (Fig. 4A, B). When compared to the DOR + RD + sh-FOXO1 + LV-NC group, rats of the DOR + RD + sh-FOXO1 + LV-KLOTHO group showed a better mental state, as well as shorter and more regular estrus cycles (Fig. 4C). The ovarian index of rats was dramatically enhanced in the DOR + RD + sh-FOXO1 + LV-KLOTHO group (Fig. 4D). H&E staining demonstrated that the medullary atrophy in ovarian tissue was alleviated, the number of atretic follicles was reduced, and the number of primordial and mature follicles was increased in the DOR + RD + sh-FOXO1 + LV-KLOTHO group in contrast with those in the DOR + RD + sh-FOXO1 + LV-NC group (Fig. 4E). The DOR + RD + sh-FOXO1 + LV-KLOTHO group had downregulated expression of FSH and LH and increased E2 level in rat serum (Fig. 4F–H). Ovarian TUNEL staining manifested that apoptosis of granulosa cells was significantly blocked in the DOR + RD + sh-FOXO1 + LV-KLOTHO group than that in the DOR + RD + sh-FOXO1 + LV-NC group (Fig. 4I). Western blotting displayed that Bcl-2 expression was evidently enhanced while Bax expression was distinctly weakened in the DOR + RD + sh-FOXO1 + LV-KLOTHO group (Fig. 4J). Above results suggested that RD administration meliorated ovarian functions in DOR rats by the FOXO1/KLOTHO axis.
DOR is clinically manifested as decreased quality of ovarian follicular reserve as a consequence of ovarian dysfunction, which is caused by various factors involving endocrine disorders and ovarian injury and leads to reduced fertility [25]. Current research has investigated the clinical therapeutics for DOR, including dehydroepiandrosterone supplementation, ovulation induction therapy, and HRT [26]. Nevertheless, the true benefit of HRT is under active debate as it has some side effects of overall increased risks of breast cancer, heart disease, stroke, and venous thromboembolism [27]. The complex pathophysiology and etiology of DOR urge more efficient treatment strategies. In the present study, the impact of RD treatment on moderating disease progression of DOR was unraveled in CTX-induced rats. In terms of specific mechanisms, RD administration suppressed apoptosis of granulosa cells and improved ovarian functions in DOR rats via the FOXO1/KLOTHO axis, ultimately mitigating DOR progression.
Western medicine believes that DOR can be attributed to advanced age and non-physiological parameters such as genetic background, surgical interventions, and therapies for cancer treatment [28]. In accordance with the theories of traditional Chinese medicine, oocytes derive from kidney essence and the basic pathogenesis of DOR is related to a deficiency of kidney essence [29]. Herein, a DOR rat model was established by CTX injection which has been widely used for DOR modelling [22]. Rehmannia is effective in cooling blood and hemostasis and tonifying the yin and the kidney [30]. Rehmannia glutinosa leaves were disclosed to restore the function of intestinal flora, mediate glycolipid level, and reduce early kidney damage in diabetes [31]. A more recent work reported that Rehmannioside A, derived from Rehmannia glutinosa Libosch, has a neuroprotective effect in cerebral ischemia-induced cognitive impairment by inhibiting ferroptosis and activating the PI3K/AKT pathway [32]. Our experimental results revealed that high-dose administration of RD inhibited the damage caused by CTX in ovarian reserve and granulosa cells. Of note, as a major cause of DOR, the apoptosis of ovarian granulosa cells has been a consistent research focus concerning the etiology of DOR [33]. FOXO1 expression was found to be downregulated in granulosa cells from patients with poor ovarian reserve [34]. Liu et al. [35] found an interesting association between abnormal follicular development and significantly reduced FOXO1 mRNA expression in mice. In DOR rats, FOXO1 expressed at a low level, but notably increased after 2 weeks of intervention with a high dose of RD, suggesting that RD played a positive role in ameliorating ovarian functions and reducing apoptosis of granulosa cells by upregulating FOXO1.
KLOTHO is an aging-suppressor gene predominantly found in the kidney and choroid plexus [36]. CTX-dependent microRNA-15 reduced the autophagy and the scavenging of reactive oxygen species in mouse ovarian granulosa cells by inhibiting α-KLOTHO expression, thereby promoting premature ovarian failure [37]. Besides, increasing β-KLOTHO expression contributed to inhibiting age-related malfunction in bovine granulosa cells [38]. In addition to the association between KLOTHO expression and granulosa cell behaviors, the relationship of KLOTHO and FOXO1 has been reported. Previously, KLOTHO and FOXO1 dephosphorylation were suppressed in tacrolimus-induced renal injury, and KLOTHO relieved renal injury by the PI3K/AKT/FOXO pathway [39]. Activated PPARγ-KLOTHO-FOXO1 signaling pathway was participated in attenuating podocyte apoptosis [15]. Herein, FOXO1 was disclosed to facilitate KLOTHO transcription by binding to the KLOTHO promoter. To explore the effects of KLOTHO in DOR, we injected with KLOTHO overexpression lentiviruses into rat ovaries, and found the efficacy of those lentiviruses lasted for four weeks. However, considering the difficulty of blocking DOR development by KLOTHO overexpression alone, we intended to investigate the improvement of ovarian functions in DOR rats by RD treatment in relation to the FOXO1/KLOTHO axis. In DOR rats, RD treatment-promoted ovarian functions were improved by KLOTHO upregulation despite of FOXO1 silencing. FOXOs are deemed as downstream targets of PI3K/AKT, and FOXO phosphorylation is induced by phosphorylated AKT, which leads to suppression of FOXO transcription [40]. There has been convincing evidence manifesting that Zihuai recipe alleviated CTX-DOR by suppressing PI3K/AKT pathway-mediated ovarian cell apoptosis [7]. Likewise, Dingkun Pill enhanced ovarian reserve by suppressing the PI3K/AKT/mTOR signaling pathway in DOR rats [41]. The available findings suggested that the PI3K/AKT signaling pathway may be implicated in RD treatment enhancing the FOXO1/KLOTHO axis and protecting against granulosa cell apoptosis in DOR, but extensive experiments are required to validate this notion.
Collectively, this study suggested the role of RD in blocking DOR progression via the FOXO1/KLOTHO signaling pathway. To the best of our knowledge, the present study provided the first evidence for the beneficial effects of RD on alleviating DOR progression, paving a way for possible clinical trials of RD in the future. However, our finding still needs more clinical supports, and more attention could be paid on the downstream signaling pathways involved in RD-mediated protective effect in DOR, as well as the toxic effects of RD in DOR.
REFERENCES
1. Zhang QL, Lei YL, Deng Y, Ma RL, Ding XS, Xue W, Sun AJ. 2022; Treatment progress in diminished ovarian reserve: Western and Chinese medicine. Chin J Integr Med. doi: 10.1007/s11655-021-3353-2. [Epub ahead of print]. DOI: 10.1007/s11655-021-3353-2. PMID: 35015221. PMID: https://www.scopus.com/inward/record.uri?partnerID=HzOxMe3b&scp=85122748390&origin=inward.


2. Park SU, Walsh L, Berkowitz KM. 2021; Mechanisms of ovarian aging. Reproduction. 162:R19–R33. DOI: 10.1530/REP-21-0022. PMID: 33999842. PMCID: PMC9354567. PMID: https://www.scopus.com/inward/record.uri?partnerID=HzOxMe3b&scp=85110943330&origin=inward.


3. Buigues A, Marchante M, Herraiz S, Pellicer A. 2020; Diminished ovarian reserve chemotherapy-induced mouse model: a tool for the preclinical assessment of new therapies for ovarian damage. Reprod Sci. 27:1609–1619. Erratum in: Reprod Sci. 2021;28:615. DOI: 10.1007/s43032-020-00191-w. PMID: 32430713. PMID: https://www.scopus.com/inward/record.uri?partnerID=HzOxMe3b&scp=85084993228&origin=inward.


4. Pastore LM, Christianson MS, Stelling J, Kearns WG, Segars JH. 2018; Reproductive ovarian testing and the alphabet soup of diagnoses: DOR, POI, POF, POR, and FOR. J Assist Reprod Genet. 35:17–23. DOI: 10.1007/s10815-017-1058-4. PMID: 28971280. PMCID: PMC5758472. PMID: https://www.scopus.com/inward/record.uri?partnerID=HzOxMe3b&scp=85030471235&origin=inward.


5. Murase T, Iwase A, Komatsu K, Bayasula , Nakamura T, Osuka S, Takikawa S, Goto M, Kotani T, Kikkawa F. 2018; Follicle dynamics: visualization and analysis of follicle growth and maturation using murine ovarian tissue culture. J Assist Reprod Genet. 35:339–343. DOI: 10.1007/s10815-017-1073-5. PMID: 29080194. PMCID: PMC5845041. PMID: https://www.scopus.com/inward/record.uri?partnerID=HzOxMe3b&scp=85032469760&origin=inward.


6. Fan Y, Chang Y, Wei L, Chen J, Li J, Goldsmith S, Silber S, Liang X. 2019; Apoptosis of mural granulosa cells is increased in women with diminished ovarian reserve. J Assist Reprod Genet. 36:1225–1235. DOI: 10.1007/s10815-019-01446-5. PMID: 30980221. PMCID: PMC6602993. PMID: https://www.scopus.com/inward/record.uri?partnerID=HzOxMe3b&scp=85064352322&origin=inward.


7. Liu W, Chen Q, Liu Z, Weng Z, Nguyen TN, Feng J, Zhou S. 2021; Zihuai recipe alleviates cyclophosphamide-induced diminished ovarian reserve via suppressing PI3K/AKT-mediated apoptosis. J Ethnopharmacol. 277:113789. DOI: 10.1016/j.jep.2021.113789. PMID: 33422655. PMID: https://www.scopus.com/inward/record.uri?partnerID=HzOxMe3b&scp=85106926813&origin=inward.
8. Lim SW, Jin L, Luo K, Jin J, Shin YJ, Hong SY, Yang CW. 2017; Klotho enhances FoxO3-mediated manganese superoxide dismutase expression by negatively regulating PI3K/AKT pathway during tacrolimus-induced oxidative stress. Cell Death Dis. 8:e2972. DOI: 10.1038/cddis.2017.365. PMID: 28771227. PMCID: PMC5596554. PMID: https://www.scopus.com/inward/record.uri?partnerID=HzOxMe3b&scp=85026836651&origin=inward.


9. Wu Z, He Q, Zeng B, Zhou H, Zhou S. 2020; Juvenile hormone acts through FoxO to promote Cdc2 and Orc5 transcription for polyploidy-dependent vitellogenesis. Development. 147:dev188813. DOI: 10.1242/dev.188813. PMID: 32907849. PMID: https://www.scopus.com/inward/record.uri?partnerID=HzOxMe3b&scp=85091585479&origin=inward.
10. Herndon MK, Law NC, Donaubauer EM, Kyriss B, Hunzicker-Dunn M. 2016; Forkhead box O member FOXO1 regulates the majority of follicle-stimulating hormone responsive genes in ovarian granulosa cells. Mol Cell Endocrinol. 434:116–126. DOI: 10.1016/j.mce.2016.06.020. PMID: 27328024. PMCID: PMC4983523. PMID: https://www.scopus.com/inward/record.uri?partnerID=HzOxMe3b&scp=84976493060&origin=inward.


11. Masciangelo R, Hossay C, Chiti MC, Manavella DD, Amorim CA, Donnez J, Dolmans MM. 2020; Role of the PI3K and Hippo pathways in follicle activation after grafting of human ovarian tissue. J Assist Reprod Genet. 37:101–108. DOI: 10.1007/s10815-019-01628-1. PMID: 31732846. PMCID: PMC7000614. PMID: https://www.scopus.com/inward/record.uri?partnerID=HzOxMe3b&scp=85075338703&origin=inward.


12. Xie T, Ye W, Liu J, Zhou L, Song Y. 2021; The emerging key role of Klotho in the hypothalamus-pituitary-ovarian axis. Reprod Sci. 28:322–331. DOI: 10.1007/s43032-020-00277-5. PMID: 32783104. PMID: https://www.scopus.com/inward/record.uri?partnerID=HzOxMe3b&scp=85089312518&origin=inward.


13. Xu X, Hao Y, Zhong Q, Hang J, Zhao Y, Qiao J. 2020; Low KLOTHO level related to aging is associated with diminished ovarian reserve. Fertil Steril. 114:1250–1255. DOI: 10.1016/j.fertnstert.2020.06.035. PMID: 33153705. PMID: https://www.scopus.com/inward/record.uri?partnerID=HzOxMe3b&scp=85092198047&origin=inward.


14. Lee S, Moon JH, Song K, Taweechaipaisankul A, Jo YK, Oh HJ, Park SC, Lee BC. 2017; Establishment of transgenic porcine fibroblasts expressing a human Klotho gene and its effects on gene expression and preimplantation development of cloned embryos. DNA Cell Biol. 36:42–49. DOI: 10.1089/dna.2016.3482. PMID: 28004977. PMID: https://www.scopus.com/inward/record.uri?partnerID=HzOxMe3b&scp=85008689698&origin=inward.


15. Xing L, Fang J, Zhu B, Wang L, Chen J, Wang Y, Huang J, Wang H, Yao X. 2021; Astragaloside IV protects against podocyte apoptosis by inhibiting oxidative stress via activating PPARγ-Klotho-FoxO1 axis in diabetic nephropathy. Life Sci. 269:119068. DOI: 10.1016/j.lfs.2021.119068. PMID: 33476631. PMID: https://www.scopus.com/inward/record.uri?partnerID=HzOxMe3b&scp=85099664539&origin=inward.
16. Yuan H, Yang M, Han X, Ni X. 2018; The therapeutic effect of the Chinese herbal medicine, Rehmanniae radix preparata, in attention deficit hyperactivity disorder via reversal of structural abnormalities in the cortex. Evid Based Complement Alternat Med. 2018:3052058. DOI: 10.1155/2018/3052058. PMID: 30405737. PMCID: PMC6204205. PMID: https://www.scopus.com/inward/record.uri?partnerID=HzOxMe3b&scp=85062483291&origin=inward.
17. Journal of Ethnopharmacology. 2022; Retraction: Kuntai capsule attenuates premature ovarian failure through the PI3K/AKT/mTOR pathway. J Ethnopharmacol. 289:115091. Retraction of: Zhang H, Qin F, Liu A, Sun Q, Wang Q, Li Q, Lu S, Zhang D, Lu Z. J Ethnopharmacol. 2019;239:111885. DOI: 10.1016/j.jep.2022.115091. PMID: 35148932. PMID: https://www.scopus.com/inward/record.uri?partnerID=HzOxMe3b&scp=85124252647&origin=inward.
18. Yang H, Zhai B, Wang M, Fan Y, Wang J, Cheng J, Zou J, Zhang X, Shi Y, Guo D, Tang Z. 2022; The influence of rhein on the absorption of rehmaionoside D: in vivo, in situ, in vitro, and in silico studies. J Ethnopharmacol. 282:114650. DOI: 10.1016/j.jep.2021.114650. PMID: 34536515. PMID: https://www.scopus.com/inward/record.uri?partnerID=HzOxMe3b&scp=85115769712&origin=inward.
19. Chen L, Chen H, Lu Y, Han L, Wang S, Liu M, Li X, Zhao J, Lu C, Li S. 2020; Decoding active components in a formulation of multiple herbs for treatment of psoriasis based on three cell lines fishing and liquid chromatography-mass spectrometry analysis. J Pharm Biomed Anal. 186:113331. DOI: 10.1016/j.jpba.2020.113331. PMID: 32380350. PMID: https://www.scopus.com/inward/record.uri?partnerID=HzOxMe3b&scp=85084092130&origin=inward.


20. Zhou J, Pan XY, Lin J, Zhou Q, Lan LK, Zhu J, Duan R, Wang L, Sun Y, Wang L. 2022; Effects of Bushen Yiqi Huoxue Decoction in treatment of patients with diminished ovarian reserve: a randomized controlled trial. Chin J Integr Med. 28:195–201. DOI: 10.1007/s11655-020-3484-x. PMID: 33423188. PMID: https://www.scopus.com/inward/record.uri?partnerID=HzOxMe3b&scp=85099307929&origin=inward.


21. Seo HW, Cheon SM, Lee MH, Kim HJ, Jeon H, Cha DS. 2015; Catalpol modulates lifespan via DAF-16/FOXO and SKN-1/Nrf2 activation in Caenorhabditis elegans. Evid Based Complement Alternat Med. 2015:524878. DOI: 10.1155/2015/524878. PMID: 25821490. PMCID: PMC4363898. PMID: https://www.scopus.com/inward/record.uri?partnerID=HzOxMe3b&scp=84925310745&origin=inward.
22. Jiang M, Wang W, Zhang J, Wang C, Bi Y, Li P, Yang S, Li J, Xu YT, Wang T. 2020; Protective effects and possible mechanisms of actions of Bushen Cuyun Recipe on diminished ovarian reserve induced by cyclophosphamide in rats. Front Pharmacol. 11:546. DOI: 10.3389/fphar.2020.00546. PMID: 32477106. PMCID: PMC7237638. PMID: 9aed7ae01de042b28555b813bf739929. PMID: https://www.scopus.com/inward/record.uri?partnerID=HzOxMe3b&scp=85085371353&origin=inward.


23. Li X, Hu S, Zhu Q, Yao G, Yao J, Li J, Wang Y, Ding Y, Qi J, Xu R, Zhao H, Zhu Z, Du Y, Sun K, Sun Y. 2021; Addressing the role of 11β-hydroxysteroid dehydrogenase type 1 in the development of polycystic ovary syndrome and the putative therapeutic effects of its selective inhibition in a preclinical model. Metabolism. 119:154749. DOI: 10.1016/j.metabol.2021.154749. PMID: 33722534. PMID: https://www.scopus.com/inward/record.uri?partnerID=HzOxMe3b&scp=85103082312&origin=inward.


24. Sun XF, Li YP, Pan B, Wang YF, Li J, Shen W. 2018; Molecular regulation of miR-378 on the development of mouse follicle and the maturation of oocyte in vivo. Cell Cycle. 17:2230–2242. DOI: 10.1080/15384101.2018.1520557. PMID: 30244637. PMCID: PMC6226232. PMID: https://www.scopus.com/inward/record.uri?partnerID=HzOxMe3b&scp=85053657978&origin=inward.


25. Jaillard S, eenivasan R Sr, Beaumont M, Robevska G, Dubourg C, Knarston IM, Akloul L, van den Bergen J, Odent S, Croft B, Jouve G, Grover SR, Duros S, Pimentel C, Belaud-Rotureau MA, Ayers KL, Ravel C, Tucker EJ, Sinclair AH. 2020; Analysis of NR5A1 in 142 patients with premature ovarian insufficiency, diminished ovarian reserve, or unexplained infertility. Maturitas. 131:78–86. DOI: 10.1016/j.maturitas.2019.10.011. PMID: 31787151. PMID: https://www.scopus.com/inward/record.uri?partnerID=HzOxMe3b&scp=85075133320&origin=inward.


26. Zhang C, Xu X. 2016; Advancement in the treatment of diminished ovarian reserve by traditional Chinese and Western medicine. Exp Ther Med. 11:1173–1176. DOI: 10.3892/etm.2016.3025. PMID: 27073418. PMCID: PMC4812125. PMID: https://www.scopus.com/inward/record.uri?partnerID=HzOxMe3b&scp=84958755721&origin=inward.


27. Cagnacci A, Venier M. 2019; The controversial history of hormone replacement therapy. Medicina (Kaunas). 55:602. DOI: 10.3390/medicina55090602. PMID: 31540401. PMCID: PMC6780820. PMID: https://www.scopus.com/inward/record.uri?partnerID=HzOxMe3b&scp=85072516149&origin=inward.


28. Christodoulaki A, Boel A, Tang M, De Roo C, Stoop D, Heindryckx B. 2021; Prospects of germline nuclear transfer in women with diminished ovarian reserve. Front Endocrinol (Lausanne). 12:635370. DOI: 10.3389/fendo.2021.635370. PMID: 33692760. PMCID: PMC7937897. PMID: 423211a96efe4826b5e0e36efcca6f85. PMID: https://www.scopus.com/inward/record.uri?partnerID=HzOxMe3b&scp=85102366231&origin=inward.


29. Peng H, Zeng L, Zhu L, Luo S, Xu L, Zeng L, Li J, Liang Q, Geng H. 2019; Zuogui Pills inhibit mitochondria-dependent apoptosis of follicles in a rat model of premature ovarian failure. J Ethnopharmacol. 238:111855. DOI: 10.1016/j.jep.2019.111855. PMID: 30953821. PMID: https://www.scopus.com/inward/record.uri?partnerID=HzOxMe3b&scp=85064530336&origin=inward.


30. Yang H, Fan Y, Cheng J, Zou J, Zhang X, Shi Y, Guo D. 2020; Network pharmacology-based prediction of active ingredients and potential targets of ShengDiHuang Decoction for treatment of dysfunctional uterine bleeding. Evid Based Complement Alternat Med. 2020:7370304. DOI: 10.1155/2020/7370304. PMID: 32454870. PMCID: PMC7240676. PMID: https://www.scopus.com/inward/record.uri?partnerID=HzOxMe3b&scp=85085903084&origin=inward.


31. Xu Z, Dai XX, Zhang QY, Su SL, Yan H, Zhu Y, Shang EX, Qian DW, Duan JA. 2020; Protective effects and mechanisms of Rehmannia glutinosa leaves total glycoside on early kidney injury in db/db mice. Biomed Pharmacother. 125:109926. DOI: 10.1016/j.biopha.2020.109926. PMID: 32028239. PMID: https://www.scopus.com/inward/record.uri?partnerID=HzOxMe3b&scp=85078757862&origin=inward.


32. Fu C, Wu Y, Liu S, Luo C, Lu Y, Liu M, Wang L, Zhang Y, Liu X. 2022; Rehmannioside A improves cognitive impairment and alleviates ferroptosis via activating PI3K/AKT/Nrf2 and SLC7A11/GPX4 signaling pathway after ischemia. J Ethnopharmacol. 289:115021. DOI: 10.1016/j.jep.2022.115021. PMID: 35091012. PMID: https://www.scopus.com/inward/record.uri?partnerID=HzOxMe3b&scp=85123796044&origin=inward.
33. Wei C, Xiang S, Yu Y, Song J, Zheng M, Lian F. 2021; miR-221-3p regulates apoptosis of ovarian granulosa cells via targeting FOXO1 in older women with diminished ovarian reserve (DOR). Mol Reprod Dev. 88:251–260. DOI: 10.1002/mrd.23457. PMID: 33694202. PMCID: PMC8251591. PMID: https://www.scopus.com/inward/record.uri?partnerID=HzOxMe3b&scp=85102460770&origin=inward.


34. Rehnitz J, Capp E, Messmer B, Nguyen XP, Germeyer A, Freis A, Dietrich JE, Hinderhofer K, Strowitzki T, Vogt PH. 2021; FMR1 and AKT/mTOR signaling in human granulosa cells: functional interaction and impact on ovarian response. J Clin Med. 10:3892. DOI: 10.3390/jcm10173892. PMID: 34501340. PMCID: PMC8432207. PMID: 1eddecfc559842628b4ebe4b375ffc0e. PMID: https://www.scopus.com/inward/record.uri?partnerID=HzOxMe3b&scp=85113900484&origin=inward.


35. Liu T, Zhao H, Wang J, Shu X, Gao Y, Mu X, Gao F, Liu H. 2017; The role of fructose-1,6-bisphosphatase 1 in abnormal development of ovarian follicles caused by high testosterone concentration. Mol Med Rep. 16:6489–6498. DOI: 10.3892/mmr.2017.7463. PMID: 28901488. PMCID: PMC5865816. PMID: https://www.scopus.com/inward/record.uri?partnerID=HzOxMe3b&scp=85030121314&origin=inward.


36. Kuro-O M. 2019; The Klotho proteins in health and disease. Nat Rev Nephrol. 15:27–44. DOI: 10.1038/s41581-018-0078-3. PMID: 30455427. PMID: https://www.scopus.com/inward/record.uri?partnerID=HzOxMe3b&scp=85056791142&origin=inward.


37. Liu T, Liu Y, Huang Y, Chen J, Yu Z, Chen C, Lai L. 2019; miR-15b induces premature ovarian failure in mice via inhibition of α-Klotho expression in ovarian granulosa cells. Free Radic Biol Med. 141:383–392. DOI: 10.1016/j.freeradbiomed.2019.07.010. PMID: 31310795. PMID: https://www.scopus.com/inward/record.uri?partnerID=HzOxMe3b&scp=85068932302&origin=inward.


38. Xu L, Idrees M, Joo MD, Sidrat T, Wei Y, Song SH, Lee KL, Kong IK. 2021; Constitutive expression of TERT enhances β-Klotho expression and improves age-related deterioration in early bovine embryos. Int J Mol Sci. 22:5327. DOI: 10.3390/ijms22105327. PMID: 34070219. PMCID: PMC8158768. PMID: 26d10fb610cd491e89911976bb17aae4. PMID: https://www.scopus.com/inward/record.uri?partnerID=HzOxMe3b&scp=85105880535&origin=inward.


39. Jin J, Jin L, Lim SW, Yang CW. 2016; Klotho deficiency aggravates tacrolimus-induced renal injury via the phosphatidylinositol 3-kinase-Akt-Forkhead box protein O pathway. Am J Nephrol. 43:357–365. DOI: 10.1159/000446447. PMID: 27174564. PMID: https://www.scopus.com/inward/record.uri?partnerID=HzOxMe3b&scp=84967104385&origin=inward.


40. Gong Y, Luo S, Fan P, Zhu H, Li Y, Huang W. 2020; Growth hormone activates PI3K/Akt signaling and inhibits ROS accumulation and apoptosis in granulosa cells of patients with polycystic ovary syndrome. Reprod Biol Endocrinol. 18:121. DOI: 10.1186/s12958-020-00677-x. PMID: 33287836. PMCID: PMC7720521. PMID: 68321e5ae7da402a8d0ad9c414601cba. PMID: https://www.scopus.com/inward/record.uri?partnerID=HzOxMe3b&scp=85097218885&origin=inward.


41. Ma K, Chen Y, Fan X, Yuan Y, Wang K, Tian C, Li M. 2020; Dingkun Pill replenishes diminished ovarian reserve through the PI3K/AKT/mTOR signaling pathway in TWP-induced mice. J Ethnopharmacol. 262:112993. DOI: 10.1016/j.jep.2020.112993. PMID: 32473368. PMID: https://www.scopus.com/inward/record.uri?partnerID=HzOxMe3b&scp=85089796889&origin=inward.


Fig. 1
High-dose administration of RD improved ovarian functions and decreased apoptosis of granulosa cells in DOR rats.
(A) Vaginal smear was used to investigate estrous cycles of rats. (B) Ovarian index. (C) H&E staining of ovary, and the number of primordial, mature, and atretic follicles. (D–F) ELISA assay was used to determine the levels of FSH (D), LH (E), and E2 (F). (G) Cell apoptosis in rat ovaries was tested by TUNEL staining. (H) The expression of Bcl-2 and Bax was measured by Western blotting. Values are presented as mean ± SD. DOR, diminished ovarian reserve; RD, Rehmannioside D; FSH, Follicle-stimulating hormone; LH, luteinizing hormone; E2, estradiol. n = 10. *p < 0.05.
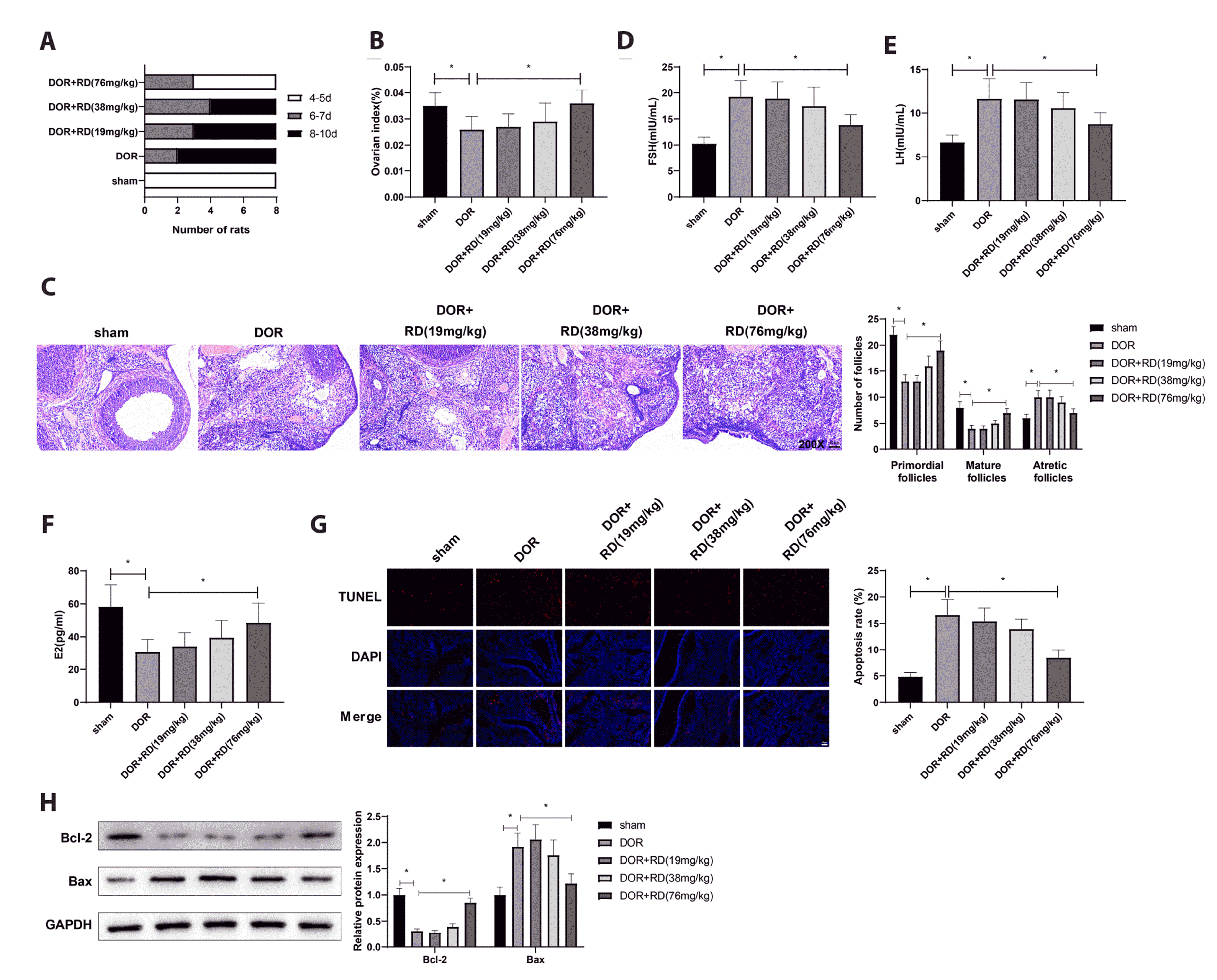
Fig. 2
RD treatment relieved DOR progression in rats by upregulating FOXO1.
(A, B) qRT-PCR (A) and Western blotting (B) were implemented to investigate the expression of FOXO1 in ovarian tissues. DOR rats were injected with sh-FOXO1 or its negative control and administrated with a high dose of RD for 2 weeks. (C, D) qRT-PCR (C) and Western blotting (D) were used to determine FOXO1 in ovarian tissues. (E) The estrous cycles of rats were measured using vaginal smears. (F) Ovarian index. (G) Ovarian H&E staining, and the number of primordial, mature, and atretic follicles. (H–J) The levels of FSH (H), LH (I), and E2 (J) were examined by ELISA assay. (K) Cell apoptosis in rat ovaries was investigated with TUNEL staining. (L) Western blotting was used to test the expression of Bcl-2 and Bax. Values are presented as mean ± SD. DOR, diminished ovarian reserve; RD, Rehmannioside D; FSH, Follicle-stimulating hormone; LH, luteinizing hormone; E2, estradiol; FOXO1, Forkhead Box O1. n = 10. *p < 0.05.

Fig. 3
FOXO1 enhanced KLOTHO transcription by binding to the KLOTHO promoter.
(A, B) The expression of KLOTHO in ovarian tissues was measured by qRT-PCR (A) and Western blotting (B). (C) The binding site of FOXO1 and the KLOTHO promoter was predicted by Jaspar database. (D) ChIP assay was used to detect the binding of FOXO1 to the KLOTHO promoter. (E, F) qRT-PCR (E) and Western blotting (F) were used to investigate the expression of KLOTHO in rat ovarian tissues. Values are presented as mean ± SD. DOR, diminished ovarian reserve; RD, Rehmannioside D; FOXO1, Forkhead Box O1; ChIP, Chromatin immunoprecipitation. n = 10. *p < 0.05.
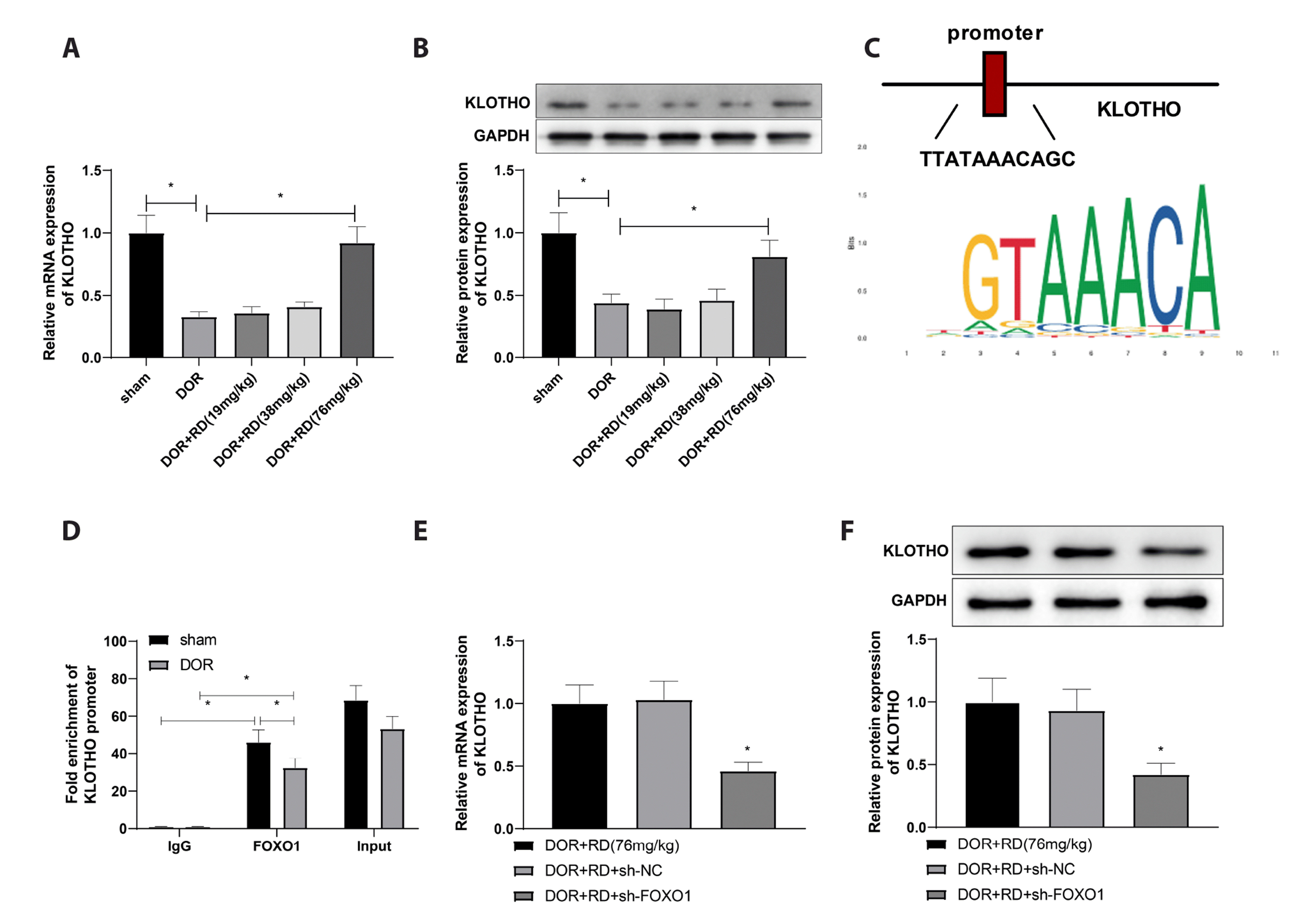
Fig. 4
RD restored ovarian functions in DOR rats through the FOXO1/KLOTHO axis.
DOR rats were injected with sh-FOXO1, LV-KLOTHO, and their negative controls, in addition to intervention with a high dose RD for 2 weeks. (A, B) The expression of KLOTHO in ovarian tissues was determined by qRT-PCR (A) and Western blotting (B). (C) Vaginal smear test was used to measure the estrous cycles of the rats. (D) Ovarian index. (E) Ovarian H&E staining, and quantitative histograms of primordial, mature, and atretic follicles. (F–H) The levels of FSH (F), LH (G), and E2 (H) were tested by ELISA assay. (I) Ovarian TUNEL staining was used to detect cell apoptosis. (J) The expression of Bcl-2 and Bax was investigated using Western blotting. Values are presented as mean ± SD. DOR, diminished ovarian reserve; RD, Rehmannioside D; FSH, Follicle-stimulating hormone; LH, luteinizing hormone; E2, estradiol; FOXO1, Forkhead Box O1. n = 10. *p < 0.05.
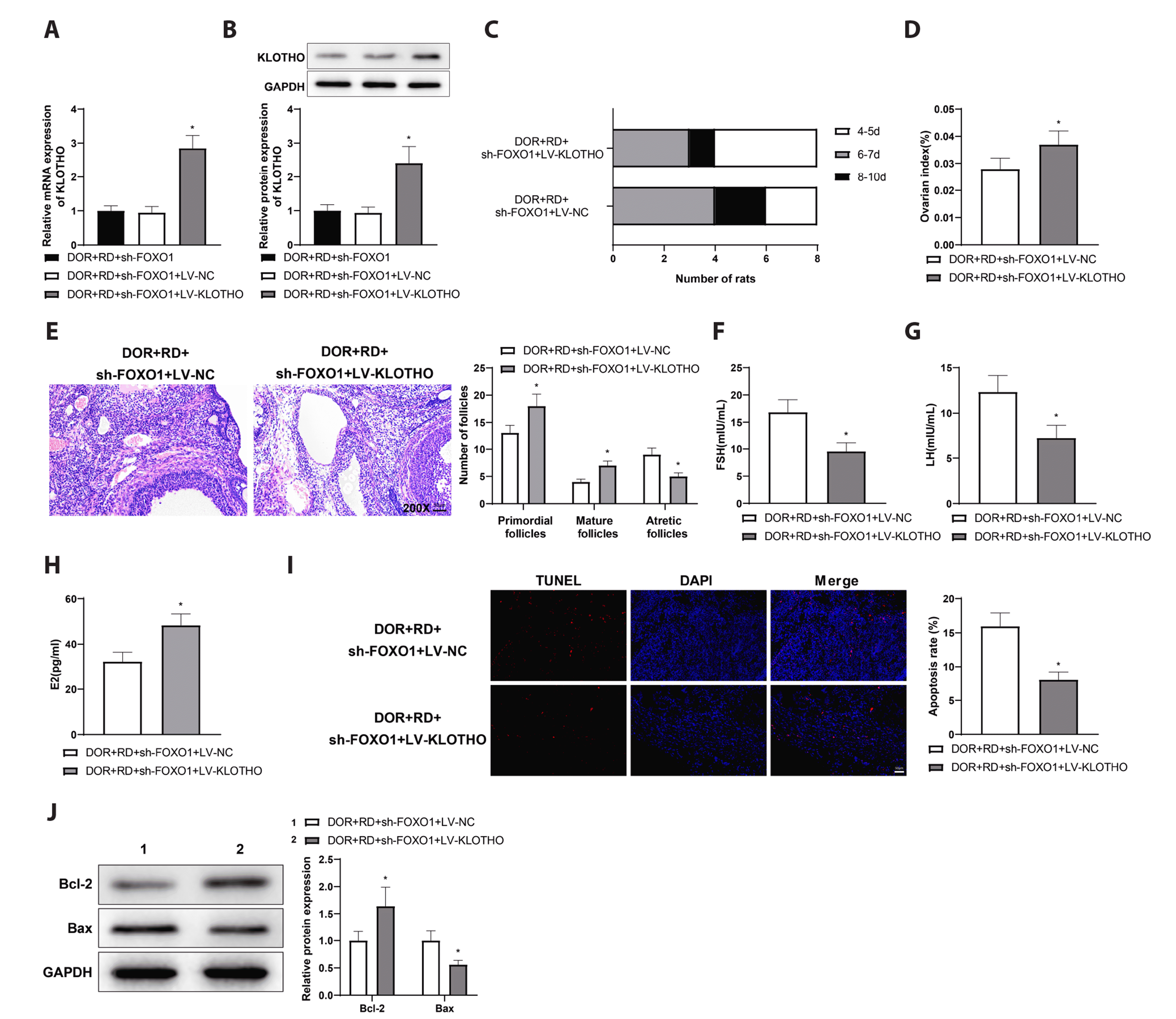