Abstract
Depression is one of the most common neuropsychiatric disorders and is associated with dysfunction of the neuroendocrine system and alterations in specific brain proteins. Boophone disticha (BD) is an indigenous psychoactive bulb that belongs to the Amaryllidacae family, which is widely used in Southern Africa to treat depression, with scientific evidence of potent antidepressant-like effects. The present study examined the antidepressant effects of BD and its mechanisms of action by measuring some behavioural parameters in the elevated plus maze, brain content of corticosterone, brain derived neurotropic factor (BDNF), and neuroblast differentiation in the hippocampus of Balb/c mice exposed to the five day repeated forced swim stress (5d-RFSS). Male Balb/c mice were subjected to the 5d-RFSS protocol to induce depressive-like behaviour (decreased swimming, increased floating, decreased open arm entry, decreased time spent in the open arms and decreased head dips in the elevated plus maze test) and treated with distilled water, fluoxetine and BD. BD treatment (10 mg/kg/p.o for 3 weeks) significantly attenuated the 5d-RFSS-induced behavioural abnormalities and the elevated serum corticosterone levels observed in stressed mice. Additionally, 5d-RFSS exposure significantly decreased the number of neuroblasts in the hippocampus and BDNF levels in the brain of Balb/c mice, while fluoxetine and BD treatment attenuated these changes. The antidepressant effects of BD were comparable to those of fluoxetine, but unlike fluoxetine, BD did not show any anxiogenic effects, suggesting better pharmacological functions. In conclusion, our study shows that BD exerted antidepressant-like effects in 5d-RFSS mice, mediated in part by normalizing brain corticosterone and BDNF levels.
World Health Organization identifies depression as one of the leading causes of morbidity and mortality worldwide [1]. Depression is a complex disease that can occur because of multitude of factors, including biological, emotional, and environmental influences [2]. Diagnosis of depression is mainly based on symptomatic behavioural criteria such as depressed mood, low self-esteem and recurrent thoughts of death and suicide. The heterogeneity of depression suggests that multiple biological mechanisms may underlie its etiology [3]. It is estimated that 40% risk of developing depression is genetic, though the specific genes involved are partially understood [4]. The other 60% non-genetic risk remains poorly defined, with diverse theories implicating acute or chronic stress, childhood trauma, viral infections, and even random processes during brain development as possible causes [5].
The most prescribed antidepressant drugs include tricyclic antidepressants (TCAs) and selective serotonin reuptake inhibitors (SSRIs) [6]. Fluoxetine in particular has been shown not only to have antidepressant properties but to also have antioxidant activities [7]. However, these antidepressant drugs have undesirable side effects. For instance, acute administration of SSRIs such as fluoxetine has been reported to paradoxically increase symptoms of anxiety for some individuals thereby complicating the treatment objective [8, 9]. This increase in anxiety is related to increased serotonergic function of SSRIs, which is believed to provoke an anxiogenic effect in animal models [10]. Co-administration of benzodiazepines and SSRIs is believed to cause faster onset of anxiolytic effects of the SSRI [9]. However, research has shown that non-sedating anti-depressants in combination with benzodiazepines could result in driving impairment [11]. Furthermore, the conventional antidepressant drugs such as fluoxetine are highly expensive and beyond the reach of the poor and the needy. Consequently, the need for alternative antidepressant drugs becomes a great necessity and have resulted in the search for affordable, cost effective and easily accessible natural products and herbal medicines with potential anxiolytic and antidepressant properties such as Boophone disticha (BD).
BD (tumbleweed/sore-eye flower) is an indigenous bulb that is widely used by traditional healers in Southern Africa for the treatment of a variety of ailments including anxiety [12]. BD belongs to the Amaryllidaceae family, Boophone genus, found throughout Southern and Tropical Africa [13], and has been reported to reduce mean arterial pressure of maternally separated mice [14] and in normal non-stressed mice [15].
Furthermore, crude leaf extracts of BD have also been shown to have a high affinity for the serotonin transporter (SERT) [16], with its two alkaloids buphanadrine and buphanamine, being responsible for binding to the SSRI site of the serotonin P transporter [17]. These mechanisms may partially explain why BD is used traditionally for the management of anxiety. One of the proposed mechanisms of action of many antidepressants is through regulation of adult hippocampal neurogenesis (AHN). AHN is a complex multistep process involving precursor cell proliferation in the subgranular zone (SGZ) of the hippocampal dentate gyrus, differentiation into neuroblasts, migration and subsequent integration into the granule cell layer [18]. SSRIs have been shown to enhance AHN, which might contribute to their antidepressant properties [19]. Conversely, stress and major depression have been shown to reduce volume, cell proliferation and neurogenesis in the adult mammalian hippocampus [20].
Given that BD possibly acts through a similar mechanism as SSRIs, we hypothesized that it would have a regulatory effect on AHN. Therefore, the aim of this study was to evaluate the potential of a crude hydroethanolic extract of BD to attenuate the five day repeated forced swim (5d-RFS) induced stress, associated effects and possible AHN impairment in male Balb/c mice. The 5d-RFS has been shown to result in progressive increases in immobility time in rodents usually persisting for at least 4 weeks and often considered the onset and persistence of a depression-like state [21]. Additionally, the 5d-RFS has been reported to decrease sucrose preference for at least 4 weeks after the last swimming session, suggesting anhedonia, which is also observed in human depression [21, 22]. The progressive increase in immobility time and persistence of anhedonia has been shown to last for at least 4 weeks, giving a window of opportunity to evaluate therapeutic interventions [21].
Twenty (n=20) 6 week old male Balb/c mice were used in the current study. Animals were purchased and housed at Wits Research Animal Facility (WRAF) and were handled by professional personnel of the facility. Before the start of the experiment, animals were acclimatized for one week. Consequently, at the start of the experimental procedure, the animals were seven weeks old. Animals were individually housed in perspex cages with wood shavings as a bedding. A 12 hours light dark cycle was maintained throughout the experimental period. Food and water was provided ad libitum. Initial body weights were taken at the start of the experiment, and then once every week. Terminal weights were also recorded and compared to initial weights. All animal experiments were carried out according to the protocols approved by the Animal Ethics Screening Committee (AESC) of the University of the Witwatersrand (AESC number: 2017/011/78/D).
Bulbs of BD were purchased from Random Harvesters in Muldersdrift Johannesburg, South Africa and were authenticated at the Department of Botany, University of Johannesburg, South Africa.
A table knife was used to chop the fresh bulbs of BD into small pieces and sun dried for seven days. The dried plant material was ground into a fine powder using a blender. The powder was then mixed with 70% ethanol in a ratio 5:1 (70% ethanol: BD powder) and placed on an automatic shaker for 24 hours at room temperature. After 24 hours, plant debris were filtered using Whatman No. 1 filter paper. The resultant extract was concentrated using a rotary evaporator at 70°C. The final product was a dark brown solid paste which was air dried, crushed into powder and stored at room temperature. The administered dose of the powder was weighted in grams according to each animal’s body weight and dissolved in 2 ml distilled water and administered through orogastric gavage.
A Thermoscientific ultimate 3000 Ultra High-Performance Liquid Chromatography (UHPLC) in conjunction with Bruker Compact Q-TOF high resolution mass spectrophotometer (HRMS) was used to analyse 20 μl of BD extract dissolved in methanol. Gradient elusion techniques was applied to elute 20 μl of the sample that was injected into the UHPLC in a period of 14 minutes. Solvent A with a concentration ranging from 95%–5% of 0.1% formic acid in water (v/v) and a solvent B with a concentration ranging from 5%–95% of 0.1% formic acid in acetonitrile (v/v) was used through Raptor ARC C18 Column (2.7 μm 2.1×100 mm). Analyses of the sample was done using the UHPLC-HRMS analyses, while Bruker Daltonics analyses was used for mass spectrum analyses.
The twenty mice were randomly divided into two main groups: A (treatment group: n=15) and B (control: n=5). Group A was exposed to 5d-RFSS and was further divided into three treatment subgroups (Fluoxetine, BD and Distilled water groups) with 5 mice each. Fluoxetine group received a dose of 10 mg/kg body weight of fluoxetine [23, 24] via oral gavage for 21 days, BD group received 10 mg/kg body weight of BD extract via oral gavage for 21 days [14], and Distilled water group received 0.2 ml distilled water, the vehicle for the treatment drugs for 21 days. Group B (Control) consisted of five normal mice that were not exposed to 5d-RFSS and received distilled water for 21 days.
Forced swimming stress model was first introduced by Porsolt et al. [25], in 1977. The procedure for this model is to place the mouse for 6 minutes into a glass-polycarbonate cylinder (25 cm high×10 cm wide) filled to a depth of 10cm with water maintained at 24°C. Immediately following the 6 minutes exposure into the water cylinder, the animals are removed from the cylinder and dried using a water absorbent pad. In the current study, we used a recently described five day repeated forced swim stress (5d-RFSS) protocol [22], in which mice were forced to swim in an open cylindrical container (diameter, 10 cm; height, 25 cm) containing 10 cm deep water (25°C) for five consecutive days. The five days procedure constituted the induction phase of the stress model. This protocol had been shown to produce the depressive symptoms that last for at least four weeks, allowing for a time window to test and study therapeutic interventions [22]. After the five days induction period, animals were treated for 21 days, while five minutes behavioural tests were conducted and video recorded at intervals on days 7, 14 and 21, after 5d-RFSS. The period/duration of swimming and immobility during the 6 minutes test was measured from the video recordings and recorded using a timer. While the FST has received extensive criticism regarding its validity and reproducibility, a metanalysis of 73 behavioral studies that used FST in mice demonstrated external validity and reproducibility of the FST where different types of antidepressant drugs reduced immobility in the test [26].
The issue of ethics regarding the use of the FST has been raised due to its perceived suffering to animals [27]. However, there is currently no regulation against the use of the FST. The fact sheet published by British Association for Psychopharmacology in conjunction with, the Laboratory Animal Science Association and Understanding Animal Research advocated for the continued use of FST as a valuable behavioral screening tool [28]. A recent commentary suggested the use of none-behavioral neurochemical measures like brain derived neurotropic factor (BDNF) as an alternative to the FST [29]. The current study included the testing of the BDNF levels in the brain and showed that the changes in the BDNF are in solidarity with the reduction in immobility time in the FST paving a way for a shift away from the FST.
On the test day, mice were brought into the test room for at least one hour before the test. All testing was performed between 9:00 am and 15:00 pm by the experimenter. Behavioral tests were done randomly (Elevated plus maize and forced swimming test). All behavioral activities were video recorded via an overhead video camera (Canon Legria, HF R806, China) and later analyzed by the independent observer blinded to the experimental groups.
The maze is plus-shaped with four identical 50 cm (length)×10 cm (width) arms, elevated 70 cm above the floor. Two opposite arms are surrounded on three sides by 30 cm tall opaque walls, and the other two arms are open with no protecting walls on the sides. Each animal was introduced in the centre area (10×10 cm) facing an open arm and allowed to explore freely for 5 minutes. The number of arm entries and time spent in each arm was scored. An arm entry was scored when all four paws of the animals entered an arm and time in arm was counted only if all four paws of the animal are within the arm [25]. Behaviours in this task (i.e., activity in the open and closed arms) reflect a conflict between the rodent’s preference for protected areas (e.g., closed arms) and their innate motivation to explore novel environments (e.g., open arms). Anti-anxiety behaviour (increased open arm time and/or open arm entries) can be determined simultaneously with a measure of spontaneous motor activity (total and/or closed arm entries [30, 31].
After behavioural tests, animals were euthanized by an overdose of 5% isoflurane at a flow of 2 l/min, followed by intra cardiac perfusion with buffered saline. Following perfusion, brains were rapidly removed from the skull and separated into two hemispheres. The left hemisphere was immediately snap frozen in dry ice before being stored at –80°C for biochemical analyses. The Elabscience ELISA kit (Biocom Africa, South Africa, catalogue No. E-EL-M0203, BDNF and E-OSEL-M0001, Corticosterone) for BDNF and corticosterone was used according to the product specifications. The brain stem and cerebellum was removed from the brains, after which the tissues were weighed and then homogenized in ice cold phosphatebuffered saline (PBS; 0.01 M, pH=7.4) in a volume ratio of 1:9 tissue to PBS with a kitchen blender in 1.5 ml PCR tubes dipped in ice. The homogenates were then centrifuged for 5–10 minutes at 5,000×g at 2°C–8°C to get the supernatant. A 100 μl each dilution of standard, blank and sample was added into the appropriate plates. Plates were then covered and incubated for 90 minutes at 37°C. After decanting the solution, 100 μl of Biotinylated Detection Ab working solution was added to each well, followed by 1 hour incubation at 37°C. The solution was decanted and 350 μl of wash buffer was added to each well, and pat dried after 1 minute. Washes were repeated 3 times. 100 μl of HRP conjugate working solution was then added to each well followed by 30 minutes incubation at 37°C. Wells were washed 5 times in a buffered solution and then 90 μl of substrate Reagent was added to each well, followed by 15 minutes incubation at 37°C. A 50 μl of stop Solution was added to each well and readings were done at 450 nm using a preheated microplate reader. The mean absorbance was calculated. The right hemisphere was immersion fixed in 4% buffered paraformaldehyde overnight before being transferred into 30% sucrose for Nissl staining and immunohistochemistry.
The right cerebral hemispheres were sectioned in a coronal plane at 50 μm, using a Leica croyostat at –25°C. One in 5 serial sections were collected free-floating into 24 well culture plates with 0.1 M PB. The first set of serial sections were mounted in positively charged slides and air dried, followed Cresyl violet staining. These sections were used to orientate the sections, measure the volumes of the DG, granule cell (GC) layer and hippocampus proper. The second set of the serial sections were stained with doublecortin (DCX) for migrating cells using immunohistochemical techniques. The remainder of the sections were stored for further analyses.
For anatomical orientation, and brain tissue architecture, Cresyl violet or Nissl staining was used. Decreasing concentrations of 5 minutes alcohol baths (100%, 95%, 70%, and 50%) were used to rehydrate the slide mounted tissues, followed by 1 minute deionization in water. Slides were than immersed for 1 minute in 1% Cresyl violet staining solution, transferred into distilled water for 1 minute. Five minutes baths of increasing concentrations of alcohol (50% to 100%) were used to dehydrate the slides. Dehydration was interrupted with a differentiation in 70% alcohol/acetic. Slides were dipped in the above solution until the desired intensity of the Cresyl violet stain was achieved. After differentiation, dehydration was continued, and slides were than cleared in xylene and cover slipped with DPX.
A protocol modified by Nkomozepi et al. [18], was used for DCX immunohistochemistry stains. Brain tissue sections were incubated in endogenous peroxidase inhibitor solution containing 49.2% 0.1 M PB, 49.2% methanol and 1.66% H2O2, and then the sections were washed in three ten minutes washes in 0.1 M PB. Washes were followed by a 2 hours incubation with gentle shaking at room temperature in a blocking buffer solution containing 0.25 % Triton X-100 in 0.1 M PB, 2% bovine serum albumin and 3% normal goat serum. The sections were then incubated with gentle shaking for 48 hours in primary antibody solution of rabbit anti-DCX (1:2,000, AB18723; Abcam, Cambridge, UK) in a blocking buffer solution, followed by three ten minutes washes in 0.1 M PB. Sections were than incubated under gentle shaking for 2 hours in 1,000 dilution of biotinylated anti-rabbit IgG (BA-5000; Vector Laboratories, Burlingame, CA, USA) secondary antibody solution in blocking buffer solution, followed by three 10 minute washes in 0.1 M PB. Sections were then incubated for one hour in an avidin-biotin solution [1:125 A and 1:125 B (Vector Laboratories) in 0.1 M PB] under gentle shaking, followed by three ten minutes washes of 0.1 M PB. The sections were then transferred into a solution containing 0.05% 3, 3-di-amino-benzidine tetrachloride in 0.1 M PB for five minutes. 3.3 μl of 30% H2O2 was added to each 1 ml of the above solution, and the reaction was monitored by viewing the tissues under the stereomicroscope. To stop the reaction, sections were transferred to a 0.1 M PB, and then washed for 10 minutes in another fresh solution of 0.1 M PB. Stained sections were mounted on positively charged slides and air dried overnight, followed by dehydration in increasing concentrations of alcohol. Air dried slides were cleared in xylene and cover slipped with DPX. Random control sections were stained following the above procedure but omitting either the secondary or primary antibody to eliminate the confounding effects of none specific staining. The control sections did not show DCX stained cells.
Six brain sections that were 250 μm apart were quantified for DCX in the SGZ of hippocampus in each animal. Cells were manually counted by means of a cell counter of ImageJ [32] on photo micrographs taken by a Leica ICC50 HD microscope mounted camera. Adobe photoshop version 7 software (Adobe Systems, Mountain View, CA, USA) was used to prepare the sample of micrographs of the DG of the hippocampus. The originality of the micrographs was not interfered with.
Statistical analysis was done using GraphPad Prism 7.0 (GraphPad Prism Software Inc., San Diego, CA, USA). All tests were parametric, and P≤0.05 was considered significant. ANOVA followed by Bonferroni’s post-hoc tests was used for comparisons of significant differences among the treatment groups. Comparisons of all scored behaviours were expressed as means±standard error and compared across treatments groups.
The spectrum view at 5.92 minutes had parental peaks as indicated by the black squares in Fig. 1 with the circle dots showing the fragments of the compounds. With the aid of a metabolite mass spectral database, the parental peaks in Fig. 1 were identified according to their specific m/z as follows: 246.1492 is Tropococaine; 274.1439 is Galanthamine; 286.1439 is Narwedine; 302.1388 is Buphanamine, and 316.1537 is Buphanidrine. All the phytochemicals obtain in this spectrum view are alkaloids and they are the most abundantly isolated phytochemicals, probably due to the use ethanol solvent.
Of the alkaloids detected in the current study (Table 1), buphanamine, lycorine, nerbowdine, crinine and distichamine have also been detected in a previous study conducted by Neergaard et al. [34], 2009. The above study also detected undulatine, acetylnerbowdine, crinamidine, buphacetine and buphanisine which were not detected in the current study. Of importance in the results of the current study, is the detection of buphanidrine and buphanamine, the two alkaloids which are known to be responsible for binding to the SERT site (Table 1).
Table 2 shows the changes in body weights of the animals in this study. Initial body weights were taken at the start of the experiment, and then once every week. Terminal weights were also recorded and compared to initial weights as shown in Table 2. There was no statistically significant difference in weight gain across the experimental groups (P=0.449). However, the percentage weight gain of the control group was significantly higher than the experimental groups (P=0.01). It is possible that the significant weight gain noted above is due to the significantly low (P=0.029) average initial weight of the control group as all groups ended with a similar weight.
During the induction phase, mice were forced to swim for 6 minutes daily for 5 days. On day one, the swimming and immobility times were 141.06±29.55 and 218.93±29.55 respectively. These two indices significantly changed to 76.37±29.77 and 283.62±29.77 respectively on day five (P=0.000). From day six, the animals were assigned to three different treatment groups as described in the methods above and were retested again after days 7, 14 and 21 of the treatment period post 5d-RFSS.
There were significant differences in swimming times across all the groups after 21 days of treatment (F=8.647, P=0.004; Fig. 2A). Bonferroni’s post-hoc analyses showed that the swimming time for both fluoxetine and BD treated groups was significantly higher than that of the distilled water treated group (P<0.05). A time dependant effect of treatments recorded in behavioural tests that were conducted in days 14 and 21 was performed. The post-hoc analyses showed that by treatment day 14, the swimming time for the fluoxetine treated animals was significantly higher (P=0.0012) compared to the distilled water and BD treated animals, while BD treated animals only showed a significant increase in swimming time by day 21 (P=0.004; Fig. 2B), whereby the fluoxetine treated group had reached a plateau of increase in swimming time.
On day 14 of the treatment period, immobility times were significantly different across the treatment groups as demonstrated in ANOVA test (F=4.237, P=0.029). Furthermore, Bonferroni’s post-hoc analysis revealed that the immobility times were significant reduced in the fluoxetine treated group compared to both the distilled water and BD treated groups (P= 0.01). On the contrary, no significant differences were found between the distilled water and BD treated groups in post-hoc test (P=0.185).
In day 21, ANOVA test detected significant differences in immobility times across the treatment groups (F=8.597, P=0.004, Fig. 2C). Bonferroni’s post-hoc analysis revealed that immobility times were significantly reduced in both the fluoxetine (0.001) and BD (0.004) groups compared to the distilled water treated group.
When compared to the control, distilled water treated group had significantly high immobility times by treatment day 21 (P=0.001). However, no differences were found between the control and both the fluoxetine (P=0.067) and BD (P=1.00) treated groups.
A time dependant steady reduction in immobility time from day 5 to day 21 was observed in the BD treated animals, whereas a steady reduction was observed in a fluoxetine treated group from day 5 to day 14 and plateaued between day 14 and 21. The immobility time for distilled water treated group remained high throughout the treatment period (P=0.001, Fig 2D).
Fig. 3 shows the results of the elevated plus maze. The ANOVA test showed that the time spent in the open arms of the elevated plus maze was significantly different among the groups (F=44.41, P=0.0001, Fig. 3A). Bonferroni’s post-hoc test revealed that the time spent in the open arms was significantly higher in the control group compared to experimental groups (P<0.05). Furthermore, BD treated group recorded significantly high time in the open arms compared to both distilled water and fluoxetine treated groups (P<0.05). The time spent in the open arms for fluoxetine and distilled water treated groups was not significantly different (P>0.05).
The time spent in closed arms was not significantly different across the groups as shown in ANOVA test (F=1.835, P=0.195). The control group recorded the lowest time in closed arms, though non-significant, when compared with the treatment groups. However, both fluoxetine and BD recorded low time in the closed arms as compared to the distilled water group (Fig. 3B).
The number of head dips was significantly different across the groups as shown by ANOVA test (F=6.122, P=0.0105, Fig. 3C). Bonferroni’s post-hoc test showed that the control group had significantly high number of head dips compared to distilled water and fluoxetine treated groups, both of which scored the lowest number of head dips (P<0.05). Furthermore, Bonferroni’s post-hoc test showed that the head dips of the BD treated group were not significantly different from those of the control group (P>0.05). In addition, the post-hoc test showed that both the control and BD treated groups recorded significantly high frequency of open arm entries compared to both distilled water and fluoxetine treated groups (P<0.05, Fig. 3D). Furthermore, BD treated group had significantly low frequency of closed arm entries compared to distilled water and fluoxetine treated groups (P<0.05, Fig. 3E).
The ANOVA test did not detect any significant differences in the volume of the dentate gyrus (F=0.3083, P=0.8190, Fig. 4A), whole hippocampus (F=3.110, P=0.1101, Fig. 4B), and granule cell layer of the dentate gyrus (F=1.501, P=0.2955, Fig. 4C) across all the groups. However, there was a slight reduction of the dentate gyrus volume in the experimental groups compared to the control group. Similarly, a slight reduction in the hippocampal volume of the experimental groups was observed compared to the control group. The same observation was also noted in the volume of the granule cell layer of the experimental groups compared to the control group.
There were statistically significant differences in the DCX expression across the groups as detected by the ANOVA test (F=10.23, P=0.0005). Bonferroni’s post-hoc test showed that the DCX expression was significantly lower in the distilled water treated group compared to both fluoxetine and BD treated groups (P<0.05, Figs. 5, 6A). The DCX expression in fluoxetine and BD treated animals was similar and comparable to the control group. Furthermore, the DCX immunoreactive cells of the control, BD and fluoxetine treated groups had well developed dendritic spines, while those of the distilled water treated group had poorly developed dendritic spines (Fig. 5).
The expression of BDNF was significantly different across the groups in ANOVA test (F=9.051, P=0.0026, Fig. 6B). The expression of BDNF was significantly lower in the distilled water group compared to the control, fluoxetine and BD treated groups in the post-hoc test (P<0.05). Furthermore, the post-hoc test showed that fluoxetine and BD treatment significantly increased the expression of BDNF compared to the distilled water group (P<0.05). There were no significant differences when comparing the control group to both fluoxetine and BD treated groups (P>0.05).
The expression of corticosterone was significantly different across the groups (F=53.55, P=0.001, Fig. 6C). The post-hoc test showed that corticosterone levels remained significantly high in the distilled water treated group when compared to the control group (P<0.05). On the contrary, treatment with either fluoxetine or BD significantly reduced corticosterone levels (P<0.05). The levels of corticosterone in the fluoxetine and BD treated groups were not significantly different from that of the control group (P>0.05).
In the current study, we investigated the therapeutic efficacy of the hydroethanolic extract of BD in attenuating the effects of five days forced swimming stress in male Balb/c mice. In the induction phase of the stress model, mice were forced to swim for 6 minutes, each day, in a glass cylinder for five consecutive days followed by 21 days treatment interventions mixed with behavioral tests in-between. The results indicate that the five days forced swimming significantly increased the immobility time in day five compared to day one, thus confirming a successful stress induction. The immobility time was high in control group compared to either fluoxetine or BD treated groups throughout the 21 days treatment period. The persistent high immobility time observed in distilled water treated group corroborates with previous reports indicating that the 5 days forced swimming induced stress persists for a period of four weeks [21, 22]. Treatment with fluoxetine caused significant reduction in immobility time by day 14. By day 21, immobility time for fluoxetine treated group remained similar to day 14 but BD treated group showed significant decline in immobility time compared distilled water group.
The absence of change in immobility time in fluoxetine treated group between day 14 and 21 suggest fast onset and peak of action by fluoxetine, followed by plateaued effect. Previous studies reported similar reduction in immobility time following 10 mg/kg fluoxetine treatment for 24 days in Balb/c mice but did not include the weekly intervals of behavioural test assessments as in the present study [35]. However, the result is consistent with other previous reports of continuous reduction in immobility time between week one and week two of fluoxetine treatment [36]. Therefore, the distinction between the present study results and the previous report [36] is the third week behavioural test assessment which showed a plateauing in the action of fluoxetine.
The present study has therefore revealed lack of prolonged fluoxetine action relative to prolonged continuous potency of BD against the effects of the stress [35].
The time dependent increase in the efficacy of BD with the same dose could be an advantage in maintaining depression patients without increasing the dosage of the drug. A previous study showed that two alkaloids of BD, buphanidrine and distichamine have the affinity for the SERT, a property which is believed to be responsible for the antidepressant effects of BD [12, 34]. Overall, the effects of both fluoxetine and BD on the swimming behavior of animals are in agreement with the known principle that serotonergic compounds like fluoxetine are sensitive to swimming behavior while TCAs are sensitive to climbing behavior of animals [37, 38].
Fluoxetine treated group performed poorly in the elevated plus maze with regards to the number of head dips and time spent in the open arms when compared BD treated group. The lower fluoxetine scores in the open arms and head dips is in agreement with the reports by the previous authors who recorded that acute administration of selective serotonin reuptake inhibiters paradoxically increase symptoms of anxiety for some individuals whereby anxiolytic effects only become observable after chronic treatment [8, 9]. A sharp increase in serotonergic function of SSRIs provoke an anxiogenic effects in animal models [10]. The same effect has been observed in humans where anxiety is exacerbated at the initiation of SSRIs treatment [39]. It is possible that the slow action of BD shown in this study gives an advantage of a slow increase in serotonergic function as opposed to a sharp increase caused by SSRIs which has been suspected to cause anxiogenic symptoms in patients taking these drugs. The number of head dips, time spent in open arms and the frequency of open arm entries in the BD treated group was comparable to that of the control group, suggesting that BD attenuated the effects of stress. These findings are in agreement with a previous study where 10, 25, and 40 mg/kg doses of BD produced high scores of head dips and frequency of unprotected arm entries [15]. We hypothesize that the observed time dependant increase in the efficacy of BD is the reason for anxiolytic effects of BD treated animals as demonstrated in the elevated plus maze test.
Not much has been reported about how acute stress affect the volumes of the hippocampus. Previous studies have reported a 3% reduction in hippocampal volume in rats after exposure to chronic stress [40-45]. The neuronal loss caused by stress in the hippocampus could be one of the explanations for the reduction in the volume of the hippocampus [46, 47]. A previous study done do demonstrate the effects of prenatal stress on the volume of the hippocampus found that the reduction in volume specifically affected DG, subiculum, molecular layer, all subfields of the cornu armonis and GC [48]. The above hippocampal subfields are identified as sensitive to stress and glucocorticoids [48]. Furthermore, animal studies have shown that changes in hippocampal volume are interlinked to changes in cell proliferation, dendritic structure and neurogenesis [49, 50].
In the current study, the chronic stress significantly reduced the expression of DCX immunoreactive cells. This reduction in expression of DCX immunireactive cells was attenuated by both fluoxetine and BD treatments. The above results are consistent with the previous findings that provided evidence that acceleration of neurogenesis is one of the cellular mechanisms underpinning SSRIs antidepressant efficacy [51-54]. Chronic administration of SSRIs including fluoxetine has long been shown to play a role in promoting proliferation of progenitor cell early phase maturation and cell survival stages of neurogenesis [24, 55, 56]. In human patients, it has also been shown that the number of mature granule cells in the dentate gyrus of major depressive disorder patients treated with SSRIs is higher than in untreated patients [57]. On the hand, a previous study in 5-HT1A receptor knockout mice showed that proliferation and early maturation of cells in the DG was lacking after treatment with fluoxetine [58]. The above results suggest that the effects of SSRIs on granule cell layer proliferation is mediated through 5-HT1A receptors [59]. The involvement of 5-HT1A receptors in SSRIs mediated neurogenesis is also confirmed by the studies that used a 5-HT1A receptor agonist, 8-OH-DPAT, where the cell proliferation in the DG was seen to increase after short-term administration in rodents [60, 61]. In addition, 5-HT4 receptor activation has also been shown to facilitate proliferation and maturation of new-born neurones [62]. In the current study, BD treated animals showed results comparable to fluoxetine in terms of expression of DCX immunoreactive cells. Previous studies have demonstrated that two alkaloids of BD, buphanidrine and buphanamine have the affinity for the 5-HT1A receptors [11]. The above is thought to be the explanation for the antidepressant effects of BD. While previous studies did not look at the effect of BD on the expression of the DCX immunoreactive cells of the dentate gyrus, the observations of the current study suggest that BD causes antidepressant effects with the mechanism similar to that of fluoxetine in rectifying the decline of DCX imminoreactive cell expression.
A significant increase in the brain corticosterone was observed in stressed control animals of the current study, in agreement with previous authors [63-68]. In the current study, treatment with either fluoxetine or BD normalised the levels of brain corticosterone. Similarly, other studies have recorded reduction in corticorsterone levels in depressed human patients and animals after treatment with SSRIs [69-72]. Furthermore, our observations confirm the findings by previous research that SSRIs are able to downregulate the HPA axis activity in both rats and human subjects [73, 74]. The reduction of corticosterone levels seen after SSRIs treatment has been associated with improvement in depressive symptoms including memory impairment [75].
We recorded significantly reduced levels of BDNF in the control group of the current study. These findings are in agreement with previous authors who reported low levels of BDNF in animals exposed to stress and in post mortem brains of depressed patients [76-82]. Evidence has been previously provided that the reduction in BDNF levels are linked to depressive symptoms in mice [83, 84]. Similar to corticosterone, treatment with either fluoxetine or BD restored the levels of BDNF to the levels comparable to that of the control group in the current study. Early studies established that part of the mechanism of action of antidepressant drugs is enhancement of BDNF and TrkB mRNA expression in the hippocampus and cortical regions [85, 86]. Fluoxetine in particular has been shown to promote BDNF expression in the visual cortex and hippocampus through BDNF signalling [87]. Furthermore, the increased levels of BNDF coupled with increase in DCX immunoreactive cells recorded in the current study further support the theory that the activity of the antidepressant drugs is related to neuronal plasticity [88, 89]. It is therefore possible that BD exerts its antidepressant effects on the BDNF levels through the same mechanism described for fluoxetine and SSRIs in general.
In conclusion, we hypothesize that the hydroethanolic extract of BD exerts its antidepressant effects with a mechanism similar to that of fluoxetine. Both the above treatments caused a reduction of corticosterone and an increase in BDNF levels. The Increase in BDNF levels is believed to be responsible for the improvement in the count of DCX immunoreactice cell counts in the treated animals when compared to the control group. Mean while both BD and fluoxetine demonstrated anxiolytic effects in the forced swimming test, animals treated with fluoxetine showed anxiogenic like behaviour in the open field test where the animals spent more time in the protected arms and had fewer count of head dips, while animals treated with BD showed good anxiolytic activities where the above parameters were significantly higher. In the current study, we have also shown that the potency of BD has a time dependant increase in the forced swimming test. Fluoxetine treatment reached its maximum potency by day 14 of the treatment period as shown by the swimming time of the animals, while in BD treated animals swimming time continued to increase steadily, and by day 21 it was comparable to that of fluoxetine treated animals. These results suggest that BD has a potential for better outcomes as an antidepressant medication when compared to the current conventional medication.
Notes
References
1. Vigo D, Thornicroft G, Atun R. 2016; Estimating the true global burden of mental illness. Lancet Psychiatry. 3:171–8. DOI: 10.1016/S2215-0366(15)00505-2. PMID: 26851330.


2. Zayka TO, Evdokimov DV, Sidorova YV, Abramets II, Nalotov SV. 2015; Antidepressant-like effects of substances with cerebroprotective activity. Biol Markers Guided Ther. 2:79–88. DOI: 10.12988/bmgt.2015.5117.


3. Anacker C. 2014; Fresh approaches to antidepressant drug discovery. Expert Opin Drug Discov. 9:407–21. DOI: 10.1517/17460441.2014.892071. PMID: 24588262.


4. Hornung OP, Heim CM. 2014; Gene-environment interactions and intermediate phenotypes: early trauma and depression. Front Endocrinol (Lausanne). 5:14. DOI: 10.3389/fendo.2014.00014. PMID: 24596569. PMCID: PMC3925849.


5. Kessler RC, Berglund P, Demler O, Jin R, Koretz D, Merikangas KR, Rush AJ, Walters EE, Wang PS. 2003; The epidemiology of major depressive disorder: results from the National Comorbidity Survey Replication (NCS-R). JAMA. 289:3095–105. DOI: 10.1001/jama.289.23.3095. PMID: 12813115.
6. Kharamin S, Razmeh S, Nabovvati M, Moradian K, Rahimi S, Orooji M, Taghavian L, Mand Maher MK. 2019; Evaluation of antidepressant-like effect Lavandulifolia stachys in the forced swimming test in comparison with imipramine and fluoxetine. Int J Plant Biol. 10:7458. DOI: 10.4081/pb.2019.7458.


7. Panwar R, Sivakumar M, Menon V, Vairappan B. 2020; Changes in the levels of comet parameters before and after fluoxetine therapy in major depression patients. Anat Cell Biol. 53:194–200. DOI: 10.5115/acb.19.217. PMID: 32647087. PMCID: PMC7343562.


8. Bagdy G, Graf M, Anheuer ZE, Modos EA, Kantor S. 2001; Anxiety-like effects induced by acute fluoxetine, sertraline or m-CPP treatment are reversed by pretreatment with the 5-HT2C receptor antagonist SB-242084 but not the 5-HT1A receptor antagonist WAY-100635. Int J Neuropsychopharmacol. 4:399–408. DOI: 10.1017/S1461145701002632. PMID: 11806866.


9. Nutt DJ. 2005; Overview of diagnosis and drug treatments of anxiety disorders. CNS Spectr. 10:49–56. DOI: 10.1017/S1092852900009901. PMID: 15618947.


10. Birkett MA, Shinday NM, Kessler EJ, Meyer JS, Ritchie S, Rowlett JK. 2011; Acute anxiogenic-like effects of selective serotonin reuptake inhibitors are attenuated by the benzodiazepine diazepam in BALB/c mice. Pharmacol Biochem Behav. 98:544–51. DOI: 10.1016/j.pbb.2011.03.006. PMID: 21397628. PMCID: PMC3085089.


11. Ramaekers JG. 2003; Antidepressants and driver impairment: empirical evidence from a standard on-the-road test. J Clin Psychiatry. 64:20–9. DOI: 10.4088/JCP.v64n0106. PMID: 12590619.
12. Sandager M, Nielsen ND, Stafford GI, van Staden J, Jäger AK. 2005; Alkaloids from Boophane disticha with affinity to the serotonin transporter in rat brain. J Ethnopharmacol. 98:367–70. DOI: 10.1016/j.jep.2005.01.037. PMID: 15814274.


13. Gadaga LL, Tagwireyi D, Dzangare J, Nhachi CF. 2011; Acute oral toxicity and neurobehavioural toxicological effects of hydroethanolic extract of Boophone disticha in rats. Hum Exp Toxicol. 30:972–80. DOI: 10.1177/0960327110384524. PMID: 20889580.


14. Pote W, Tagwireyi D, Chinyanga HM, Musara C, Pfukenyi DM, Nkomozepi P, Gadaga LL, Nyandoro G, Chifamba J. 2014; Long-term cardiovascular autonomic responses to aqueous ethanolic extract of Boophone disticha bulb in early maternally separated BALB/c mice. S Afr J Bot. 94:33–9. DOI: 10.1016/j.sajb.2014.04.012.


15. Pote W, Musarira S, Chuma D, Gadaga LL, Mwandiringana E, Tagwireyi D. 2018; Effects of a hydroethanolic extract of Boophone disticha bulb on anxiety-related behaviour in naive BALB/c mice. J Ethnopharmacol. 214:218–24. DOI: 10.1016/j.jep.2017.12.001. PMID: 29223391.


16. Pedersen ME, Szewczyk B, Stachowicz K, Wieronska J, Andersen J, Stafford GI, van Staden J, Pilc A, Jäger AK. 2008; Effects of South African traditional medicine in animal models for depression. J Ethnopharmacol. 119:542–8. DOI: 10.1016/j.jep.2008.08.030. PMID: 18809486.


17. Cheesman L, Nair JJ, van Staden J. 2012; Antibacterial activity of crinane alkaloids from Boophone disticha (Amaryllidaceae). J Ethnopharmacol. 140:405–8. DOI: 10.1016/j.jep.2012.01.037. PMID: 22322252.


18. Nkomozepi P, Mazengenya P, Ihunwo AO. 2019; Age-related changes in Ki-67 and DCX expression in the BALB/c mouse (Mus Musculus) brain. Int J Dev Neurosci. 72:36–47. DOI: 10.1016/j.ijdevneu.2018.11.005. PMID: 30472241.


19. Song NN, Huang Y, Yu X, Lang B, Ding YQ, Zhang L. 2017; Divergent roles of central serotonin in adult hippocampal neurogenesis. Front Cell Neurosci. 11:185. DOI: 10.3389/fncel.2017.00185. PMID: 28713247. PMCID: PMC5492328.


20. Malykhin NV, Coupland NJ. 2015; Hippocampal neuroplasticity in major depressive disorder. Neuroscience. 309:200–13. DOI: 10.1016/j.neuroscience.2015.04.047. PMID: 25934030.


21. Mul JD, Zheng J, Goodyear LJ. 2016; Validity assessment of 5 day repeated forced-swim stress to model human depression in young-adult C57BL/6J and BALB/cJ mice. eNeuro. 3:ENEURO.0201-16.2016. DOI: 10.1523/ENEURO.0201-16.2016. PMID: 28058270. PMCID: PMC5197406.


22. Serchov T, Clement HW, Schwarz MK, Iasevoli F, Tosh DK, Idzko M, Jacobson KA, de Bartolomeis A, Normann C, Biber K, van Calker D. 2015; Increased signaling via adenosine A1 receptors, sleep deprivation, imipramine, and ketamine inhibit depressive-like behavior via induction of homer1a. Neuron. 87:549–62. DOI: 10.1016/j.neuron.2015.07.010. PMID: 26247862. PMCID: PMC4803038.


23. Hodes GE, Hill-Smith TE, Lucki I. 2010; Fluoxetine treatment induces dose dependent alterations in depression associated behavior and neural plasticity in female mice. Neurosci Lett. 484:12–6. DOI: 10.1016/j.neulet.2010.07.084. PMID: 20692322. PMCID: PMC4623584.


24. Pawluski JL, van Donkelaar E, Abrams Z, Houbart V, Fillet M, Steinbusch HW, Charlier TD. 2014; Fluoxetine dose and administration method differentially affect hippocampal plasticity in adult female rats. Neural Plast. 2014:123026. DOI: 10.1155/2014/123026. PMID: 24757568. PMCID: PMC3976918.


25. Porsolt RD, Bertin A, Jalfre M. 1977; Behavioral despair in mice: a primary screening test for antidepressants. Arch Int Pharmacodyn Ther. 229:327–36. PMID: 596982.
26. Kara NZ, Stukalin Y, Einat H. 2018; Revisiting the validity of the mouse forced swim test: systematic review and meta-analysis of the effects of prototypic antidepressants. Neurosci Biobehav Rev. 84:1–11. DOI: 10.1016/j.neubiorev.2017.11.003. PMID: 29128579.


27. PETA. c2020. Victories! PETA is ending near-drowning experiments on animals [Internet]. People for the Ethical Treatment of Animals;Norfolk, VA: Available from: https://www.peta.org/features/peta-ends-near-drowning-tests-small-animals. cited 2022 Jul 18.
28. LASA. c2020. The forced swim test [Internet]. LASA;Hull: Available from: https://www.lasa.co.uk/the-forced-swim-test. cited 2022 Jul 22.
29. Sewell F, Waterson I, Jones D, Tricklebank MD, Ragan I. 2021; Preclinical screening for antidepressant activity - shifting focus away from the Forced Swim Test to the use of translational biomarkers. Regul Toxicol Pharmacol. 125:105002. DOI: 10.1016/j.yrtph.2021.105002. PMID: 34245825.


30. Nalloor R, Bunting K, Vazdarjanova A. 2011; Predicting impaired extinction of traumatic memory and elevated startle. PLoS One. 6:e19760. DOI: 10.1371/journal.pone.0019760. PMID: 21611173. PMCID: PMC3097191.


31. Walf AA, Frye CA. 2007; The use of the elevated plus maze as an assay of anxiety-related behavior in rodents. Nat Protoc. 2:322–8. DOI: 10.1038/nprot.2007.44. PMID: 17406592. PMCID: PMC3623971.


32. Schneider CA, Rasband WS, Eliceiri KW. 2012; NIH Image to ImageJ: 25 years of image analysis. Nat Methods. 9:671–5. DOI: 10.1038/nmeth.2089. PMID: 22930834. PMCID: PMC5554542.


33. Zhang L, Li J, Lin A. 2021; Assessment of neurodegeneration and neuronal loss in aged 5XFAD mice. STAR Protoc. 2:100915. DOI: 10.1016/j.xpro.2021.100915. PMID: 34755118. PMCID: PMC8556527.


34. Neergaard JS, Andersen J, Pedersen ME, Stafford GI, Staden JV, Jäger AK. 2009; Alkaloids from Boophone disticha with affinity to the serotonin transporter. S Afr J Bot. 75:371–4. DOI: 10.1016/j.sajb.2009.02.173.


35. Dulawa SC, Holick KA, Gundersen B, Hen R. 2004; Effects of chronic fluoxetine in animal models of anxiety and depression. Neuropsychopharmacology. 29:1321–30. DOI: 10.1038/sj.npp.1300433. PMID: 15085085.


36. Mezadri TJ, Batista GM, Portes AC, Marino-Neto J, Lino-de-Oliveira C. 2011; Repeated rat-forced swim test: reducing the number of animals to evaluate gradual effects of antidepressants. J Neurosci Methods. 195:200–5. DOI: 10.1016/j.jneumeth.2010.12.015. PMID: 21167866.


37. Detke MJ, Rickels M, Lucki I. 1995; Active behaviors in the rat forced swimming test differentially produced by serotonergic and noradrenergic antidepressants. Psychopharmacology (Berl). 121:66–72. DOI: 10.1007/BF02245592. PMID: 8539342.


38. Page ME, Detke MJ, Dalvi A, Kirby LG, Lucki I. 1999; Serotonergic mediation of the effects of fluoxetine, but not desipramine, in the rat forced swimming test. Psychopharmacology (Berl). 147:162–7. DOI: 10.1007/s002130051156. PMID: 10591883.


39. Handley SL, McBlane JW. 1993; An assessment of the elevated X-maze for studying anxiety and anxiety-modulating drugs. J Pharmacol Toxicol Methods. 29:129–38. DOI: 10.1016/1056-8719(93)90063-K. PMID: 8103377.


40. Rocher C, Spedding M, Munoz C, Jay TM. 2004; Acute stress-induced changes in hippocampal/prefrontal circuits in rats: effects of antidepressants. Cereb Cortex. 14:224–9. Erratum in: Cereb Cortex 2004;14:352. DOI: 10.1093/cercor/bhg122. PMID: 14704220.


41. Lee T, Jarome T, Li SJ, Kim JJ, Helmstetter FJ. 2009; Chronic stress selectively reduces hippocampal volume in rats: a longitudinal magnetic resonance imaging study. Neuroreport. 20:1554–8. DOI: 10.1097/WNR.0b013e328332bb09. PMID: 19858767. PMCID: PMC2783199.


42. Malhi GS, Das P, Outhred T, Dobson-Stone C, Irwin L, Gessler D, Bryant R, Mannie Z. 2019; Effect of stress gene-by-environment interactions on hippocampal volumes and cortisol secretion in adolescent girls. Aust N Z J Psychiatry. 53:316–25. DOI: 10.1177/0004867419827649. PMID: 30754992.


43. Moica T, Gligor A, Moica S. 2016; The relationship between cortisol and the hippocampal volume in depressed patients - a MRI pilot study. Proced Technol. 22:1106–12. DOI: 10.1016/j.protcy.2016.01.156.


44. Rahman MM, Callaghan CK, Kerskens CM, Chattarji S, O'Mara SM. 2016; Early hippocampal volume loss as a marker of eventual memory deficits caused by repeated stress. Sci Rep. 6:29127. DOI: 10.1038/srep29127. PMID: 27374165. PMCID: PMC4931588.


45. Zimmerman ME, Ezzati A, Katz MJ, Lipton ML, Brickman AM, Sliwinski MJ, Lipton RB. 2016; Perceived stress is differentially related to hippocampal subfield volumes among older adults. PLoS One. 11:e0154530. DOI: 10.1371/journal.pone.0154530. PMID: 27144832. PMCID: PMC4856349.


46. Pham K, Nacher J, Hof PR, McEwen BS. 2003; Repeated restraint stress suppresses neurogenesis and induces biphasic PSA-NCAM expression in the adult rat dentate gyrus. Eur J Neurosci. 17:879–86. DOI: 10.1046/j.1460-9568.2003.02513.x. PMID: 12603278.


47. Mirescu C, Gould E. 2006; Stress and adult neurogenesis. Hippocampus. 16:233–8. DOI: 10.1002/hipo.20155. PMID: 16411244.


48. Marečková K, Mareček R, Bencurova P, Klánová J, Dušek L, Brázdil M. 2018; Perinatal stress and human hippocampal volume: findings from typically developing young adults. Sci Rep. 8:4696. DOI: 10.1038/s41598-018-23046-6. PMID: 29549289. PMCID: PMC5856850.


49. Maguire EA, Woollett K, Spiers HJ. 2006; London taxi drivers and bus drivers: a structural MRI and neuropsychological analysis. Hippocampus. 16:1091–101. DOI: 10.1002/hipo.20233. PMID: 17024677.


50. Leuner B, Gould E. 2010; Structural plasticity and hippocampal function. Annu Rev Psychol. 61:111–40. C1–3. DOI: 10.1146/annurev.psych.093008.100359. PMID: 19575621. PMCID: PMC3012424.


51. Kobayashi K, Ikeda Y, Sakai A, Yamasaki N, Haneda E, Miyakawa T, Suzuki H. 2010; Reversal of hippocampal neuronal maturation by serotonergic antidepressants. Proc Natl Acad Sci U S A. 107:8434–9. DOI: 10.1073/pnas.0912690107. PMID: 20404165. PMCID: PMC2889553.


52. Ohira K, Miyakawa T. 2011; Chronic treatment with fluoxetine for more than 6 weeks decreases neurogenesis in the subventricular zone of adult mice. Mol Brain. 4:10. DOI: 10.1186/1756-6606-4-10. PMID: 21385396. PMCID: PMC3060120.


53. Ohira K, Hagihara H, Miwa M, Nakamura K, Miyakawa T. 2019; Fluoxetine-induced dematuration of hippocampal neurons and adult cortical neurogenesis in the common marmoset. Mol Brain. 12:69. DOI: 10.1186/s13041-019-0489-5. PMID: 31383032. PMCID: PMC6683334. PMID: e232f729694340f5b197eb5d287f33cb.


54. Shuto T, Kuroiwa M, Sotogaku N, Kawahara Y, Oh YS, Jang JH, Shin CH, Ohnishi YN, Hanada Y, Miyakawa T, Kim Y, Greengard P, Nishi A. 2020; Obligatory roles of dopamine D1 receptors in the dentate gyrus in antidepressant actions of a selective serotonin reuptake inhibitor, fluoxetine. Mol Psychiatry. 25:1229–44. DOI: 10.1038/s41380-018-0316-x. PMID: 30531938. PMCID: PMC7244404.


55. Encinas JM, Vaahtokari A, Enikolopov G. 2006; Fluoxetine targets early progenitor cells in the adult brain. Proc Natl Acad Sci U S A. 103:8233–8. DOI: 10.1073/pnas.0601992103. PMID: 16702546. PMCID: PMC1461404.


56. Wang JW, David DJ, Monckton JE, Battaglia F, Hen R. 2008; Chronic fluoxetine stimulates maturation and synaptic plasticity of adult-born hippocampal granule cells. J Neurosci. 28:1374–84. DOI: 10.1523/JNEUROSCI.3632-07.2008. PMID: 18256257. PMCID: PMC6671574.


57. Boldrini M, Santiago AN, Hen R, Dwork AJ, Rosoklija GB, Tamir H, Arango V, John Mann J. 2013; Hippocampal granule neuron number and dentate gyrus volume in antidepressant-treated and untreated major depression. Neuropsychopharmacology. 38:1068–77. DOI: 10.1038/npp.2013.5. PMID: 23303074. PMCID: PMC3629406.


58. Samuels BA, Anacker C, Hu A, Levinstein MR, Pickenhagen A, Tsetsenis T, Madroñal N, Donaldson ZR, Drew LJ, Dranovsky A, Gross CT, Tanaka KF, Hen R. 2015; 5-HT1A receptors on mature dentate gyrus granule cells are critical for the antidepressant response. Nat Neurosci. 18:1606–16. DOI: 10.1038/nn.4116. PMID: 26389840. PMCID: PMC4624493.


59. Turcotte-Cardin V, Vahid-Ansari F, Luckhart C, Daigle M, Geddes SD, Tanaka KF, Hen R, James J, Merali Z, Béïque JC, Albert PR. 2019; Loss of adult 5-HT1A autoreceptors results in a paradoxical anxiogenic response to antidepressant treatment. J Neurosci. 39:1334–46. DOI: 10.1523/JNEUROSCI.0352-18.2018. PMID: 30552180. PMCID: PMC6381250.


60. Klempin F, Babu H, De Pietri Tonelli D, Alarcon E, Fabel K, Kempermann G. 2010; Oppositional effects of serotonin receptors 5-HT1a, 2, and 2c in the regulation of adult hippocampal neurogenesis. Front Mol Neurosci. 3:14. DOI: 10.3389/fnmol.2010.00014. PMID: 20721314. PMCID: PMC2922940.


61. Arnold SA, Hagg T. 2012; Serotonin 1A receptor agonist increases species- and region-selective adult CNS proliferation, but not through CNTF. Neuropharmacology. 63:1238–47. DOI: 10.1016/j.neuropharm.2012.07.047. PMID: 22884499. PMCID: PMC3438376.


62. Mendez-David I, David DJ, Darcet F, Wu MV, Kerdine-Römer S, Gardier AM, Hen R. 2014; Rapid anxiolytic effects of a 5-HT₄ receptor agonist are mediated by a neurogenesis-independent mechanism. Neuropsychopharmacology. 39:1366–78. DOI: 10.1038/npp.2013.332. PMID: 24287720. PMCID: PMC3988540.
63. Browne CA, Hanke J, Rose C, Walsh I, Foley T, Clarke G, Schwegler H, Cryan JF, Yilmazer-Hanke D. 2014; Effect of acute swim stress on plasma corticosterone and brain monoamine levels in bidirectionally selected DxH recombinant inbred mouse strains differing in fear recall and extinction. Stress. 17:471–83. DOI: 10.3109/10253890.2014.954104. PMID: 25117886. PMCID: PMC4527314.


64. Habr SF, Macrini DJ, Florio JC, Bernardi MM. 2014; Repeated forced swim stress has additive effects in anxiety behavior and in cathecolamine levels of adult rats exposed to deltamethrin. Neurotoxicol Teratol. 46:57–61. DOI: 10.1016/j.ntt.2014.10.001. PMID: 25444720.


65. Gong S, Miao YL, Jiao GZ, Sun MJ, Li H, Lin J, Luo MJ, Tan JH. 2015; Dynamics and correlation of serum cortisol and corticosterone under different physiological or stressful conditions in mice. PLoS One. 10:e0117503. DOI: 10.1371/journal.pone.0117503. PMID: 25699675. PMCID: PMC4336318. PMID: d26e6ee3abcc48ee981c0795b090ada3.


66. Wasinski F, Estrela GR, Arakaki AM, Bader M, Alenina N, Klempin F, Araújo RC. 2016; Maternal forced swimming reduces cell proliferation in the postnatal dentate gyrus of mouse offspring. Front Neurosci. 10:402. DOI: 10.3389/fnins.2016.00402. PMID: 27621701. PMCID: PMC5002407.


67. Emiliano S, Ana Cecilia L, Karen B, Guillermo H, Nancy R. 2019; Effect of prenatal stress and forced swimming acute stress on adult rat's skeletal muscle and liver MDA levels. MOJ Anat Physiol. 6:266–31. DOI: 10.15406/mojap.2019.06.00277.
68. Safari MA, Koushkie Jahromi M, Rezaei R, Aligholi H, Brand S. 2020; The effect of swimming on anxiety-like behaviors and corticosterone in stressed and unstressed rats. Int J Environ Res Public Health. 17:6675. DOI: 10.3390/ijerph17186675. PMID: 32937768. PMCID: PMC7558513. PMID: 7a7491c33ea34dc4ad2bb64f82c78a0a.


69. Aihara M, Ida I, Yuuki N, Oshima A, Kumano H, Takahashi K, Fukuda M, Oriuchi N, Endo K, Matsuda H, Mikuni M. 2007; HPA axis dysfunction in unmedicated major depressive disorder and its normalization by pharmacotherapy correlates with alteration of neural activity in prefrontal cortex and limbic/paralimbic regions. Psychiatry Res. 155:245–56. DOI: 10.1016/j.pscychresns.2006.11.002. PMID: 17587554.


70. Knorr U, Vinberg M, Gether U, Winkel P, Gluud C, Wetterslev J, Kessing LV. 2012; The effect of escitalopram versus placebo on perceived stress and salivary cortisol in healthy first-degree relatives of patients with depression-a randomised trial. Psychiatry Res. 200:354–60. DOI: 10.1016/j.psychres.2012.05.015. PMID: 22703642.


71. Hernandez ME, Mendieta D, Pérez-Tapia M, Bojalil R, Estrada-Garcia I, Estrada-Parra S, Pavón L. 2013; Effect of selective serotonin reuptake inhibitors and immunomodulator on cytokines levels: an alternative therapy for patients with major depressive disorder. Clin Dev Immunol. 2013:267871. DOI: 10.1155/2013/267871. PMID: 24348675. PMCID: PMC3855951.


72. Ruhé HG, Khoenkhoen SJ, Ottenhof KW, Koeter MW, Mocking RJ, Schene AH. 2015; Longitudinal effects of the SSRI paroxetine on salivary cortisol in Major Depressive Disorder. Psychoneuroendocrinology. 52:261–71. DOI: 10.1016/j.psyneuen.2014.10.024. PMID: 25544738.


73. Inder WJ, Prickett TC, Mulder RT, Donald RA, Joyce PR. 2001; Reduction in basal afternoon plasma ACTH during early treatment of depression with fluoxetine. Psychopharmacology (Berl). 156:73–8. DOI: 10.1007/s002130100737. PMID: 11465636.


74. Paile-Hyvärinen M, Wahlbeck K, Eriksson JG. 2003; Quality of life and metabolic status in mildly depressed women with type 2 diabetes treated with paroxetine: a single-blind randomised placebo controlled trial. BMC Fam Pract. 4:7. DOI: 10.1186/1471-2296-4-7. PMID: 12747810. PMCID: PMC165418.


75. Hinkelmann K, Moritz S, Botzenhardt J, Muhtz C, Wiedemann K, Kellner M, Otte C. 2012; Changes in cortisol secretion during antidepressive treatment and cognitive improvement in patients with major depression: a longitudinal study. Psychoneuroendocrinology. 37:685–92. DOI: 10.1016/j.psyneuen.2011.08.012. PMID: 21944955.


76. Aydemir O, Deveci A, Taneli F. 2005; The effect of chronic antidepressant treatment on serum brain-derived neurotrophic factor levels in depressed patients: a preliminary study. Prog Neuropsychopharmacol Biol Psychiatry. 29:261–5. DOI: 10.1016/j.pnpbp.2004.11.009. PMID: 15694233.


77. Castrén E, Rantamäki T. 2010; The role of BDNF and its receptors in depression and antidepressant drug action: reactivation of developmental plasticity. Dev Neurobiol. 70:289–97. DOI: 10.1002/dneu.20758. PMID: 20186711.


78. Nagahara AH, Tuszynski MH. 2011; Potential therapeutic uses of BDNF in neurological and psychiatric disorders. Nat Rev Drug Discov. 10:209–19. DOI: 10.1038/nrd3366. PMID: 21358740.


79. Guilloux JP, Douillard-Guilloux G, Kota R, Wang X, Gardier AM, Martinowich K, Tseng GC, Lewis DA, Sibille E. 2012; Molecular evidence for BDNF- and GABA-related dysfunctions in the amygdala of female subjects with major depression. Mol Psychiatry. 17:1130–42. DOI: 10.1038/mp.2011.113. PMID: 21912391. PMCID: PMC3237836.


80. Tripp A, Oh H, Guilloux JP, Martinowich K, Lewis DA, Sibille E. 2012; Brain-derived neurotrophic factor signaling and subgenual anterior cingulate cortex dysfunction in major depressive disorder. Am J Psychiatry. 169:1194–202. DOI: 10.1176/appi.ajp.2012.12020248. PMID: 23128924. PMCID: PMC3638149.


81. Wu LM, Hu MH, Tong XH, Han H, Shen N, Jin RT, Wang W, Zhou GX, He GP, Liu YS. 2012; Chronic unpredictable stress decreases expression of brain-derived neurotrophic factor (BDNF) in mouse ovaries: relationship to oocytes developmental potential. PLoS One. 7:e52331. DOI: 10.1371/journal.pone.0052331. PMID: 23284991. PMCID: PMC3527516. PMID: 7615391004254dfba9d52ffa95c2ff6d.


82. Phillips C. 2017; Brain-derived neurotrophic factor, depression, and physical activity: making the neuroplastic connection. Neural Plast. 2017:7260130. DOI: 10.1155/2017/7260130. PMID: 28928987. PMCID: PMC5591905.


83. Monteggia LM, Luikart B, Barrot M, Theobold D, Malkovska I, Nef S, Parada LF, Nestler EJ. 2007; Brain-derived neurotrophic factor conditional knockouts show gender differences in depression-related behaviors. Biol Psychiatry. 61:187–97. DOI: 10.1016/j.biopsych.2006.03.021. PMID: 16697351.


84. Lindholm JS, Castrén E. 2014; Mice with altered BDNF signaling as models for mood disorders and antidepressant effects. Front Behav Neurosci. 8:143. DOI: 10.3389/fnbeh.2014.00143. PMID: 24817844. PMCID: PMC4012208.


85. Nibuya M, Morinobu S, Duman RS. 1995; Regulation of BDNF and trkB mRNA in rat brain by chronic electroconvulsive seizure and antidepressant drug treatments. J Neurosci. 15:7539–47. DOI: 10.1523/JNEUROSCI.15-11-07539.1995. PMID: 7472505. PMCID: PMC6578063.


86. Nibuya M, Nestler EJ, Duman RS. 1996; Chronic antidepressant administration increases the expression of cAMP response element binding protein (CREB) in rat hippocampus. J Neurosci. 16:2365–72. DOI: 10.1523/JNEUROSCI.16-07-02365.1996. PMID: 8601816. PMCID: PMC6578518.


87. Maya Vetencourt JF, Sale A, Viegi A, Baroncelli L, De Pasquale R, O'Leary OF, Castrén E, Maffei L. 2008; The antidepressant fluoxetine restores plasticity in the adult visual cortex. Science. 320:385–8. DOI: 10.1126/science.1150516. PMID: 18420937.


88. Castrén E. 2014; Neurotrophins and psychiatric disorders. Handb Exp Pharmacol. 220:461–79. DOI: 10.1007/978-3-642-45106-5_17. PMID: 24668483.


89. Castrén E, Kojima M. 2017; Brain-derived neurotrophic factor in mood disorders and antidepressant treatments. Neurobiol Dis. 97(Pt B):119–26. DOI: 10.1016/j.nbd.2016.07.010. PMID: 27425886.


Fig. 1
Spectrum view of LC-MS chromatogram, acquired in the positive-ion mode, from an extract of the bulb of BD. LC-MS, liquid chromatography-mass spectrometry; BD, boophone disticha.
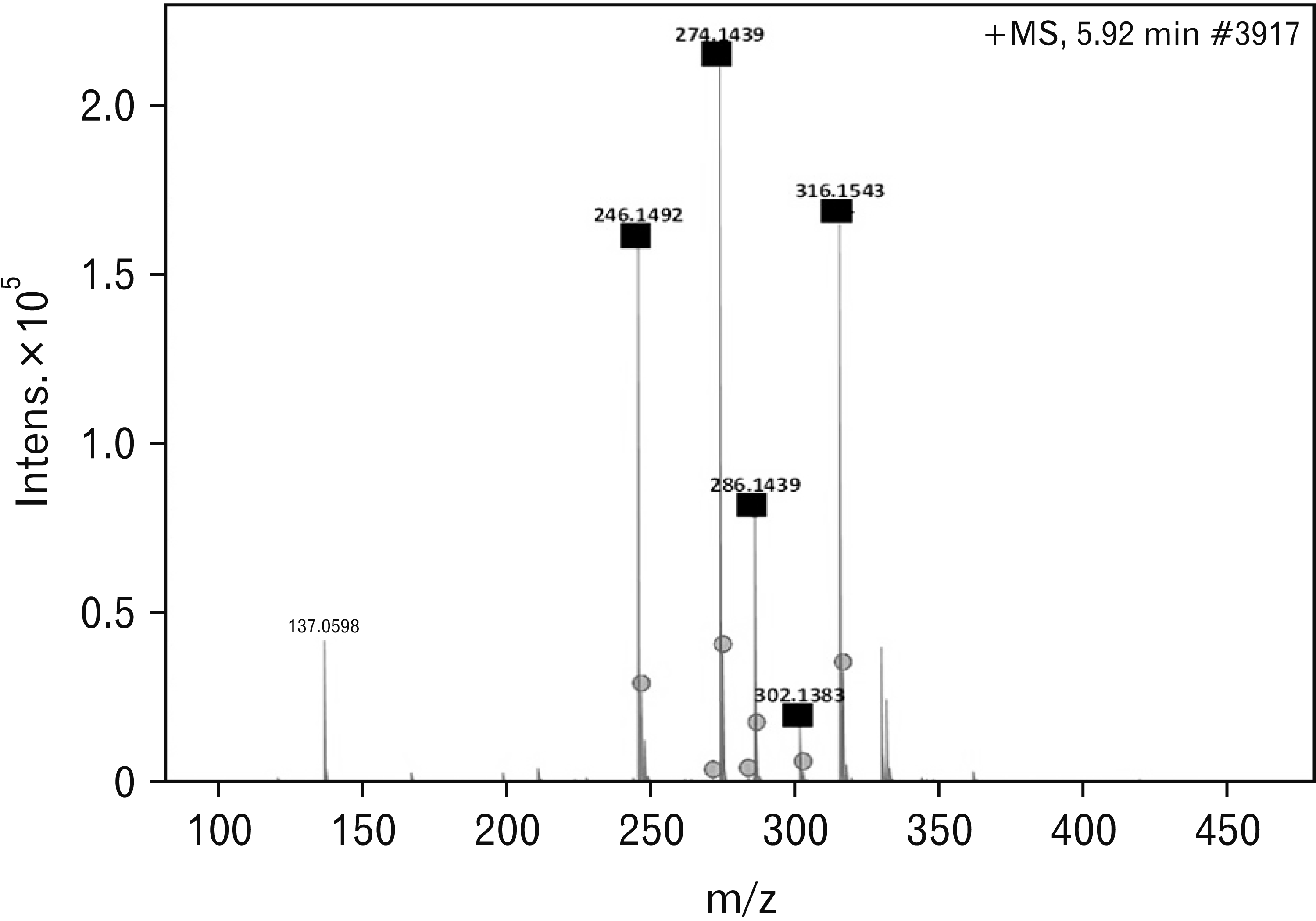
Fig. 2
Graphic representation of changes in swimming times after 21 days of treatment. (A) Significant differences in swimming time across different groups were observed in ANOVA test (P=0.04). (B) The post-hoc test showed that in day 14 of treatment, the swimming time for the fluoxetine treated animals was significantly higher (P=0.0012) compared to the distilled water and BD treated animals, while BD treated animals only showed a significant increase by day 21, whereby the fluoxetine group had reached a plateau of increase in swimming time. (C) In day 21, immobility times were significantly different across the treatment groups as demonstrated in ANOVA test (P=0.029). (D) A significant time dependant steady reduction in immobility time from day 5 to day 21 was observed in the BD treated animals (P=0.004), whereas a steady reduction was observed in fluoxetine treated group from day 5 to day 14 and plateaued between day 14 to day 21. The immobility time for distilled water treated group remained high throughout the treatment period (P=0.001). Error bars correspond to the standard error of the mean, **indicates significant changes due to treatments. BD, boophone disticha.
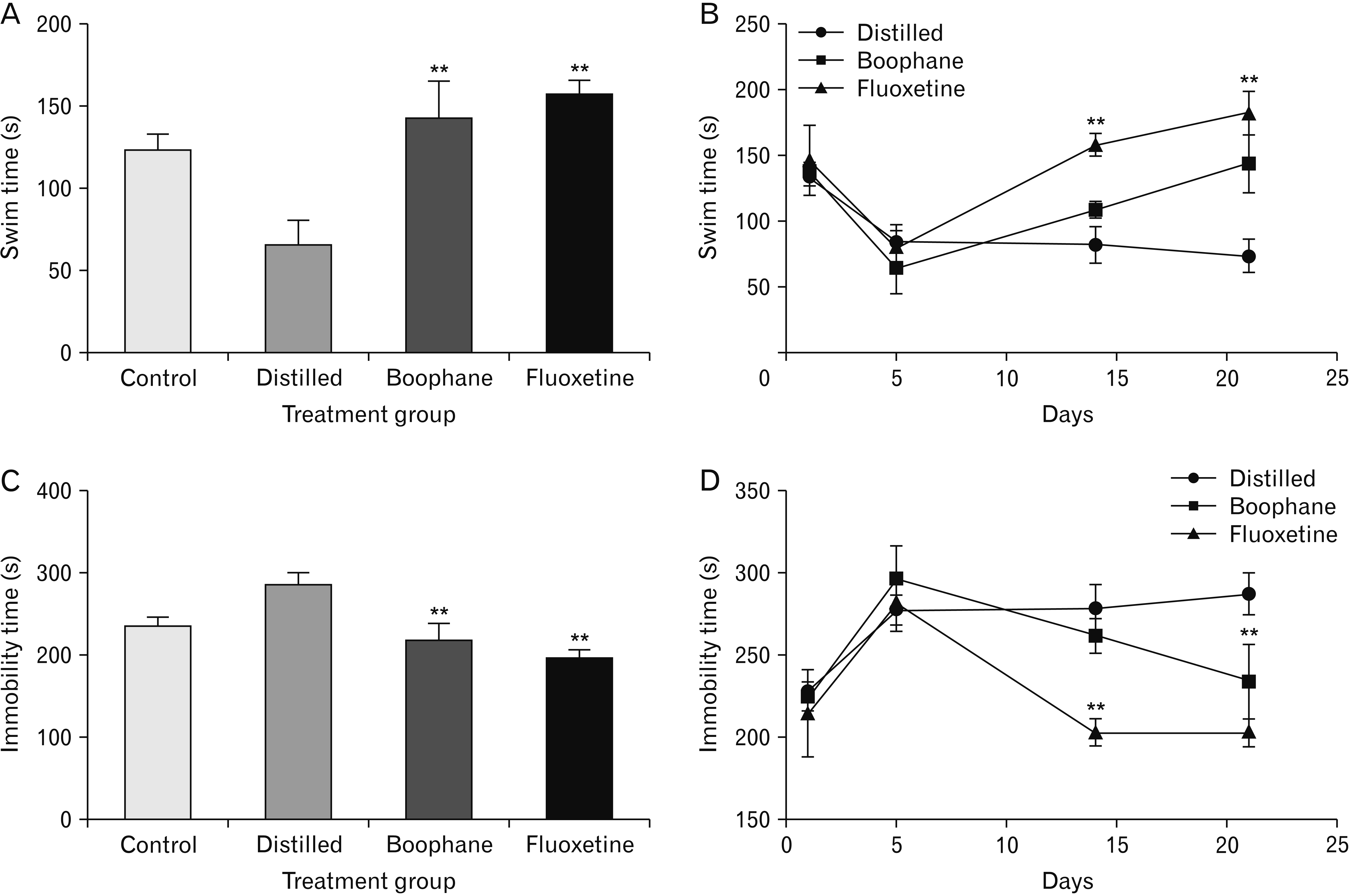
Fig. 3
Graphic representation of the elevated plus maze results. (A) The time spent in the open arms was significantly different across the groups in ANOVA test (P=0.0001). Bonferroni’s post-hoc test revealed that the time spent in the open arms was significantly higher in the control group compared to experimental groups (P<0.05). BD treated group recorded significantly high time in the open arms compared to both distilled water and fluoxetine groups (P<0.05). The time spent in the open arms for fluoxetine and distilled water group was not significantly different (P>0.05). (B) The time spent in the closed arms was not significantly different across the groups (P=0.195). The control group recorded the lowest time in closed arms though non-significant in the ANOVA test when compared with the treatment groups. Both fluoxetine and BD recorded low time in the closed arms as compared to the distilled water group. (C) The number of head dips was significantly different across the groups as shown by ANOVA test (F=6.122, P=0.0105). Bonferroni’s post-hoc test showed that the BD group had significantly high number of head dips compared to distilled water and fluoxetine treated groups both of which scored the lowest number of head dips (P<0.05). Both the control and BD treated groups recorded high frequency of open arm entries compared to both distilled water and fluoxetine treated groups (P<0.05). (D) Both the control and BD treated groups recorded high frequency of open arm entries when compared to both distilled water and fluoxetine treated groups (P<0.05). (E) BD treated group had significantly low frequency of closed arm entries compared to distilled water and fluoxetine treated groups (P<0.05). Error bars correspond to the standard error of the mean, **indicates significant changes due to treatments. BD, boophone disticha.
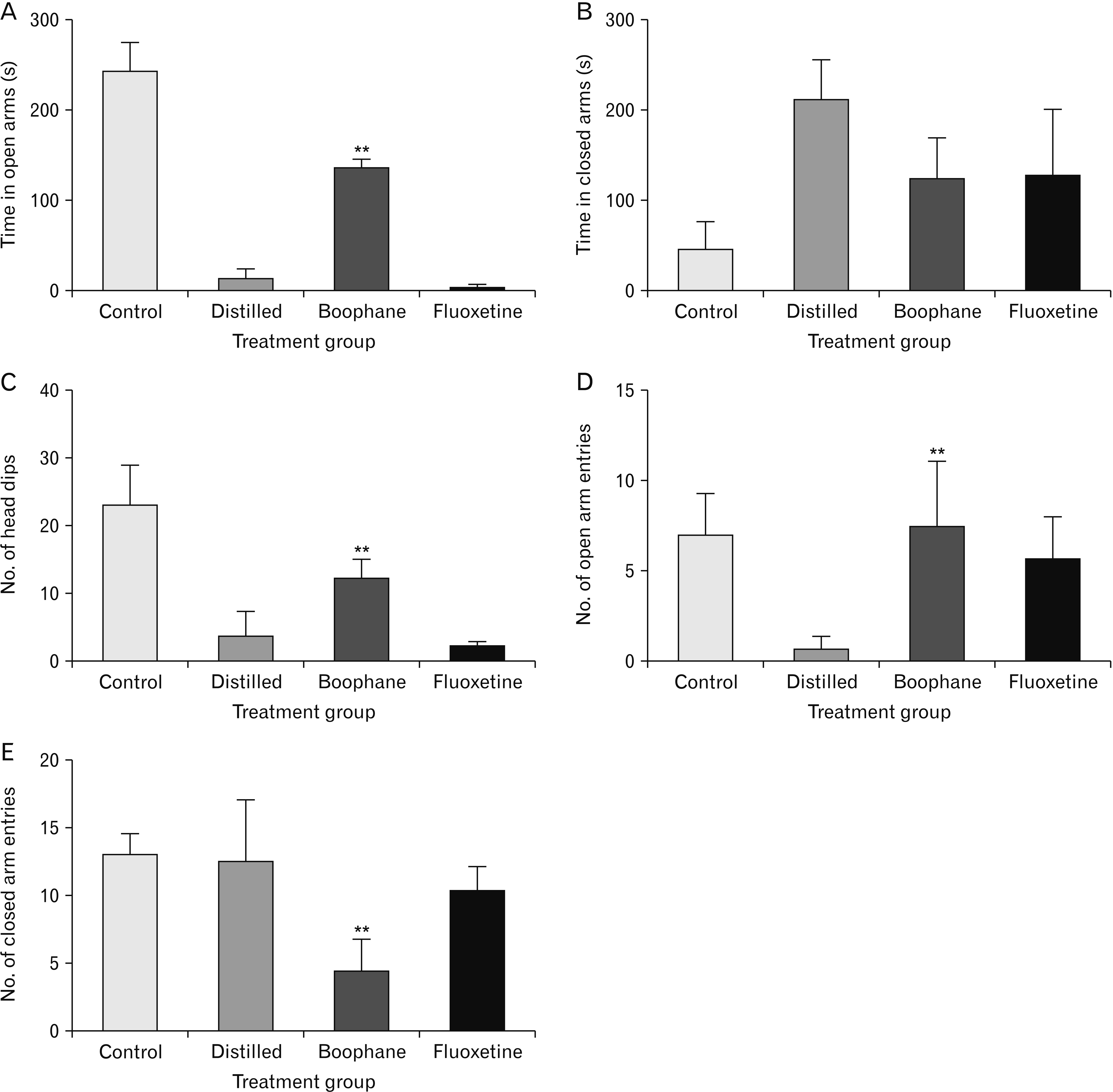
Fig. 4
Graphic representation of the volumes of the dentate gyrus and hippocampus across the groups. (A) The ANOVA test did not detect any significant differences in the volume of the dentate gyrus (F=0.3083, P=0.8190), (B) whole hippocampus (F=3.110, P=0.1101), and (C) granule cell layer of the dentate gyrus (F=1.501, P=0.2955) across all the groups. There was a slight reduction of the dentate gyrus volume in the experimental groups compared to the control group. A slight reduction in the hippocampal volume of the experimental groups as compared to the control group was also observed. The same observation was also noted in the volume of the granule cell layer of the experimental groups compared to the control group. Error bars correspond to the standard error of the mean, **indicates significant changes due to treatments.
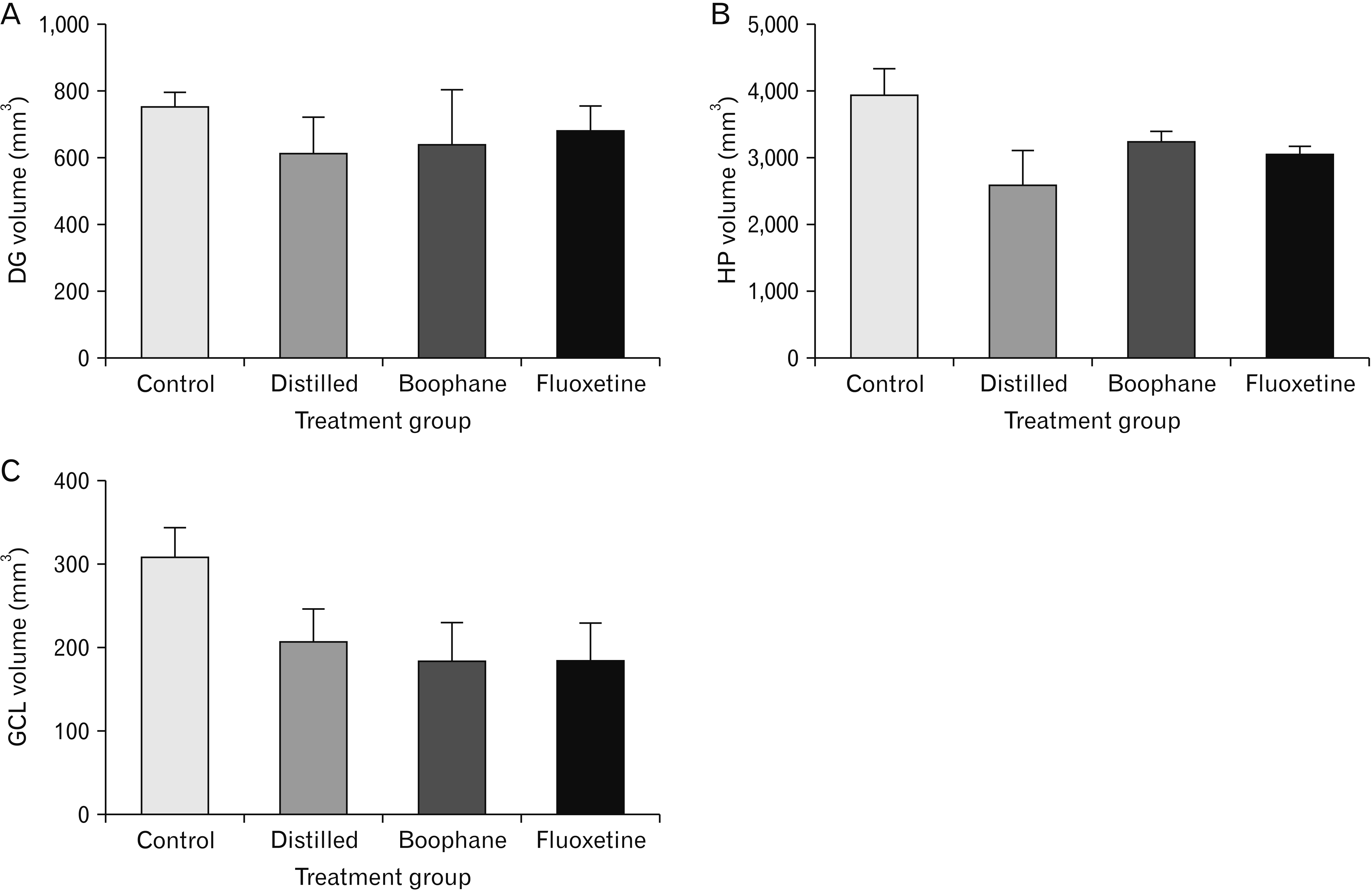
Fig. 5
Photomicrographs showing DCX immunoreactive cells in the SGZ of the dentate gyrus of the hippocampus of the normal (control) (A) and treated (B–D) groups. The black arrows are showing the cell bodies of the neuroblasts and the white arrows are showing the dendritic spines. Poorly developed dendritic spines can be seen in the distilled water treated group B. The oval circle in group B shows one of the patches in the lower limb of the dentate gyrus where DXC immunoreactive cells were absent leading to low cell count in this group. The magnified areas on the top right of each micrograph represent the areas indicated by the black rectangles. DCX, doublecortin; SGZ, subgranular zone; GCL, granule cell layer; ML, molecular layer.
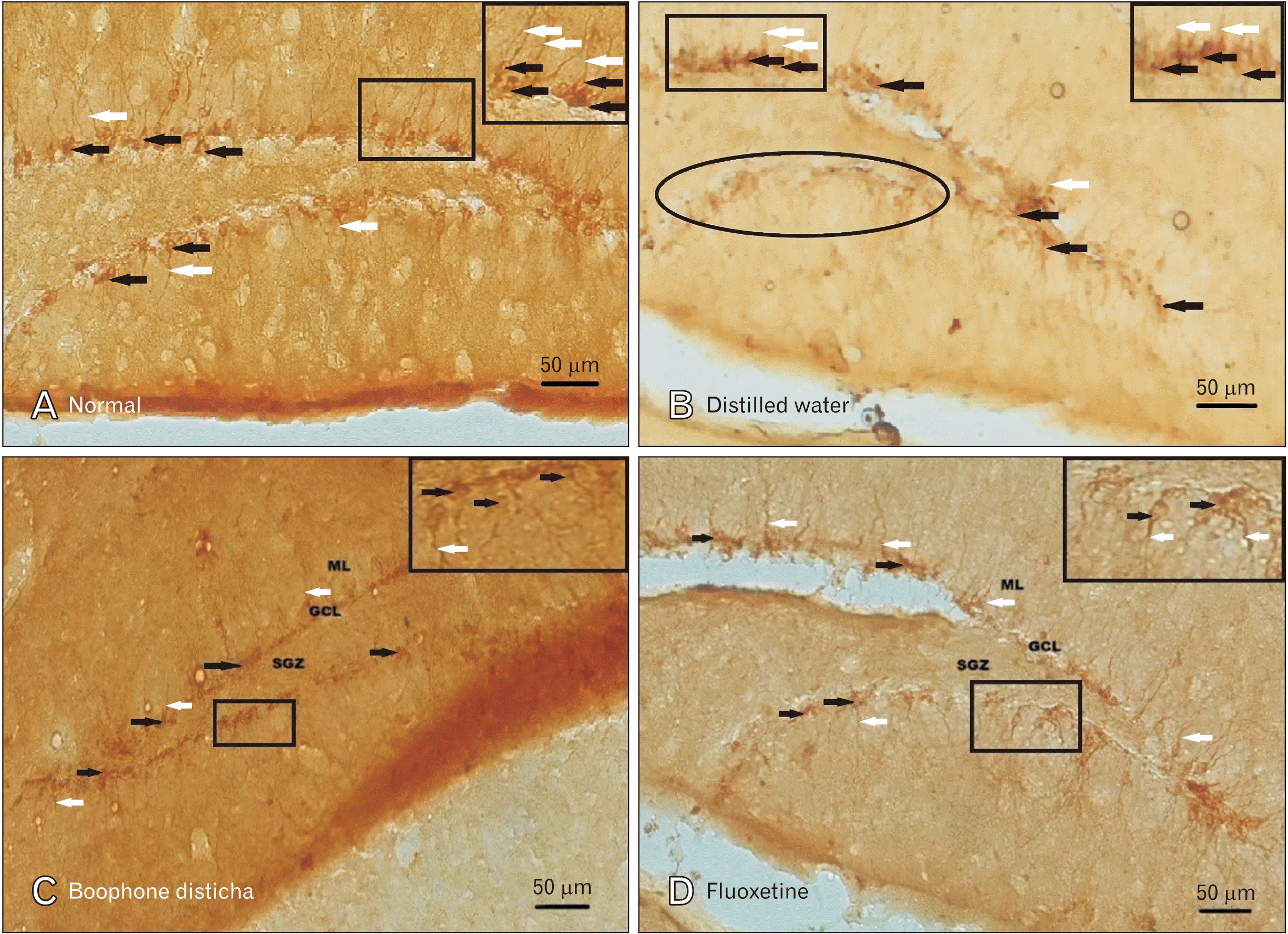
Fig. 6
Graphic representation of the expression of DCX-ir and biochemical analyses of BDNF and corticosterone. (A) The expression of DCX-ir was significantly different across the groups (P=0.0005). DCX-ir expression was significantly lower in the distilled water treated group when compared to both fluoxetine and BD treated groups as shown by the post-hoc test (P<0.05). Post-hoc analyses further showed that the counts of DCX-ir cells in both fluoxetine and BD groups were significantly higher than the distilled water group (P>0.05). (B) The expression of BDNF was significantly different across the groups (P=0.0026). BDNF expression was significantly lower in the distilled water treated group when compared to the control, fluoxetine and BD treated groups in the post-hoc test (P<0.05). The post-hoc test further showed that fluoxetine and BD treatment significantly increased the expression of BDNF when compared to the distilled water group (P<0.05). (C) The expression of corticosterone was significantly different across the groups (P=0.001). The post-hoc test showed that corticosterone levels remained significantly high in the distilled water treated group when compared to the control group (P<0.05). On the contrary, treatment with either fluoxetine or BD significantly reduced corticosterone levels (P<0.05). The levels of corticosterone in the fluoxetine and BD treated groups were not significantly different from that of the control group (P>0.05). Error bars correspond to the standard error of the mean, **indicates significant changes due to treatments. BDNF, brain derived neurotropic factor; DCX-ir, doublecortin immunoreactive cells; BD, boophone disticha.
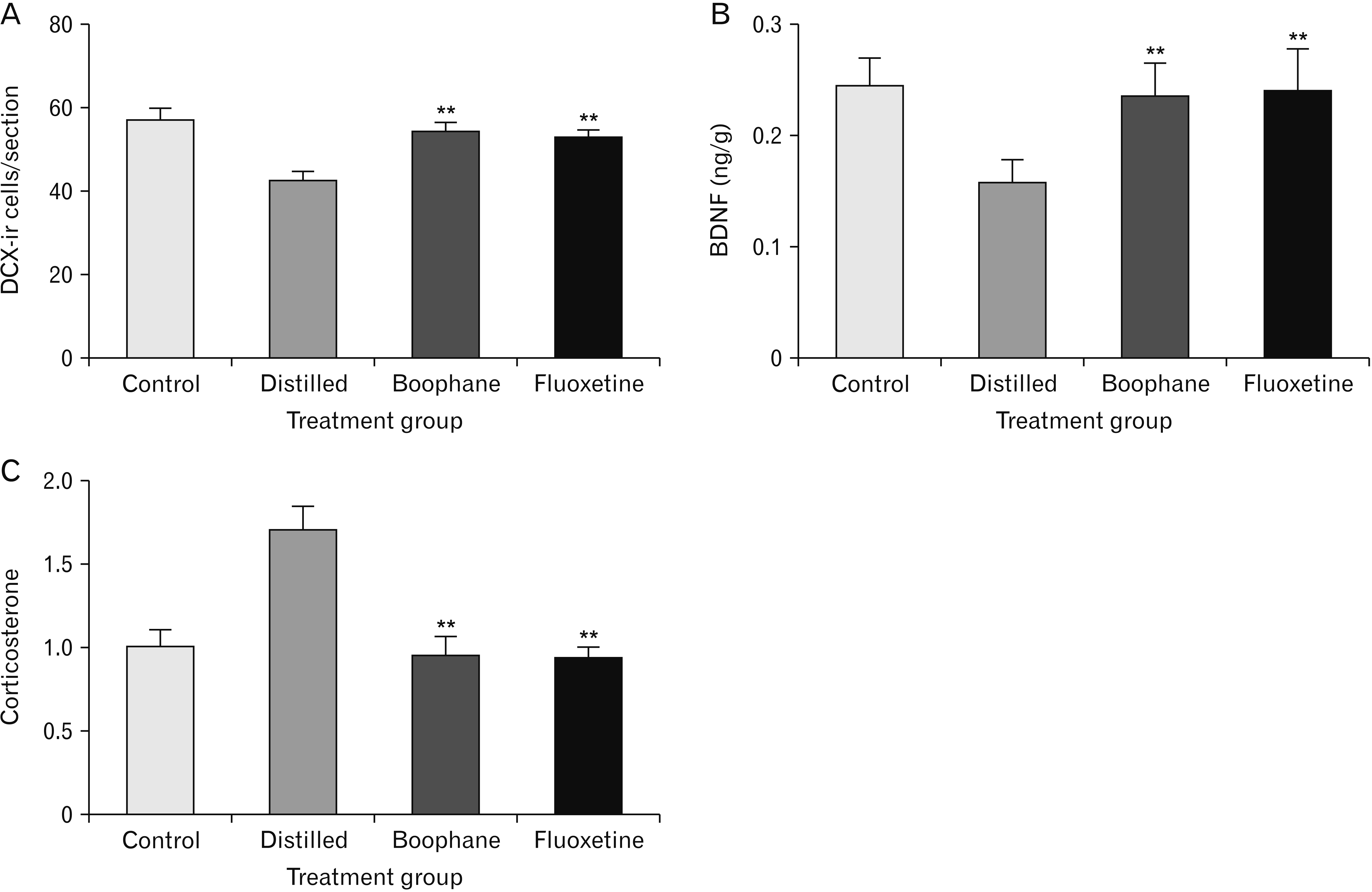
Table 1
Phytochemical profile of BD based on LC-MS data
Table 2
Body masses (g)