Abstract
The biocompatibility and durability of implant fixtures are major concerns for dentists and patients. Mechanical complications of the implant include abutment screw loosening, screw fracture, loss of implant prostheses, and implant fracture. This case report aims to describe management of a case of fixture damage that occurred after screw fracture in a tissue level, internal connection implant and microscopic evaluation of the fractured fixture. A trephine bur was used to remove the fixture, and the socket was grafted using allogeneic bone material. The failed implant was examined by scanning electron microscopy (SEM) and energy dispersive X-ray spectroscopy (EDS), which revealed a fractured fixture with both normal and irregular bone patterns. The SEM and EDS results give an enlightenment of the failed fixture surface micromorphology with microfracture and contaminated chemical compositions. Noticeably, the significantly high level of gold (Au) on the implant surface and the trace amounts of Au and titanium (Ti) in the bone tissue were recorded, which might have resulted from instability and micro-movement of the implant-abutment connection over an extended period of time. Further study with larger number of patient and different types of implants is needed for further conclusion.
Dental implants have been a reliable treatment option for rehabilitation of completely and partially edentulous patients. In addition to biocompatibility, the durability of implant fixtures is a major concern for dentists and patients. Mechanical complications of the implant include abutment screw loosening, screw fracture, loss of implant prostheses, and implant fracture. Although the success rate of implants is greater than 90%1, the incidence of implant fractures has been reported to range from 0.16% to 1.5% of cases2. One of the major causes of implant fracture is biomechanical overloading, which occurs due to various parafunctional activities like bruxism, inadequate occlusion, and distal extensions or cantilevers3-5. Other causes are peri-implant vertical bone loss due to peri-implantitis and occlusal trauma, with galvanic corrosion as an additional causative factor contributing to implant fractures6. Management of cases of screw and implant fractures can pose a challenge to clinicians because of its surgical implications. In addition, bone preservation at the implant socket site and rehabilitation after implant removal are essential.
Wee and Lee7 reported a case of implant fracture after screw fracture in a tissue level implant and suggested that screw fracture could be a precursor to fixture fracture. Reports of cases of simultaneous screw fracture and implant damage are rare. Recent studies have also questioned the importance of implant surface chemical composition. However, the available information on the release of elements from the fractured implant retrieved from the human body was very little. It is hypothesized that surface contamination might initiate the inflammatory response and possibly provoke the dissolution of titanium8. The screw loosening and the micro-movement might enhance this dissolving process and alter the implant surface and finally compromise the osseointegration. Studying the presence of dissolving ions on the fixture surface and peri-implant tissue might reveal the corrosion level during micro movement, and give the suggestion for further study of the multifactor corrosion phenomenon in implants that are failed due to fracture and other various etiologies.
Investigators have reported different techniques to determine the presence of elemental composition of the implant surface and integrated bone. Some studies evaluated the failed implant surface using X-ray photoelectron spectroscopy and Auger electron spectroscopy9,10. In our study, the element composition analysis was performed using the energy dispersive X-ray spectroscopy (EDS), which has relatively high energy and deep penetration into the sample surface11. With the EDS equipment, the compositional information can be achieved over a depth of 1 µm, which gives more information about the implant surface below the organic matter and titanium oxide layer.
This case report aims to describe the management of a case of fixture damage that occurred with screw fracture in a tissue level implant and microscopic evaluation of the failure implant segment using scanning electron microscopy (SEM) and EDS.
A 68-year-old Korean male was referred from the local clinic to the Prosthodontic Department, Seoul National University Dental Hospital (SNUDH) due to fracture of the #37i fixture. The 4.8 mm×10 mm tissue level, internal connection Straumann implant (Straumann, Basel, Switzerland) was placed at the local clinic in August 2010. After the implant was in function for almost 10 years, in January 2020, the patient visited the local clinic because the crown had detached from the fixture. During re-attachment of the crown, the screw was fractured. The local clinic dentist attempted to remove the screw but failed. Therefore, the dentist referred the patient to the SNUDH. From the Prosthodontic Department, the patient was referred to the Oral and Maxillofacial Surgery Department since removal of the fixture was too difficult to perform through a non-surgical approach. The panoramic radiograph revealed that the fixture of the implant was fractured at the alveolar bone level, with radiolucency at the coronal portion of the fixture.(Fig. 1. A) Furthermore, the computed tomography (CT) revealed a crevice on the mesiolingual aspect of the fixture, indicating the location of the fracture.(Fig. 1. B)
Informed consent was obtained from the patient. Under local anesthesia using lidocaine 2% with 1:100,000 epinephrine, the implant removal procedure was performed. A trephine bur (Shinhung, Seoul, Korea) with inner diameter 5.0 mm and length 15.0 mm was selected based on the fixture diameter so that the inner diameter of the trephine bur slightly exceeded the outer diameter of the fixture. Following this selection, the trephine bur was placed over the fixture and sunk into the bone to the exact depth using low speed at 80 rpm along with copious irrigation to avoid overheating. After the trephine bur reached a depth of 80% of the fixture body, the drilling was stopped, and the trephine bur and fixture were luxated out of the socket.(Fig. 1. C) The socket was grafted using 0.5 g allogeneic particulate graft Oragraft (LifeNet Health, Virginia Beach, VA, USA) and sutured. Five months following the surgery, a 5.0 mm×10.0 mm TSIII Osstem fixture (Osstem Implant, Busan, Korea) was installed in the grafted socket site followed by prosthetic delivery five months later.
After removal, the implant fixtures and surrounding tissue collected were immediately placed in a 2.5% glutaraldehyde solution. SEM examination was performed at 10 kV, and 65×, 500×, 1,000×, 2,500×, 5,000×, 10,000×, and 20,000× micrographs were acquired (JSM-7800F Prime; JEOL, Tokyo, Japan). The EDS instrument connected to the microscope was an XFlash6 (Bruker, Berlin, Germany) detector, and ESPRIT analysis software (Bruker) was used to analyze the data. The EDS points were designed as U: implant surface on the upper 1/3rd of the fixture, M: implant surface at the middle 1/3rd of the fixture, B: attached bone tissue on the fixture in the middle region of the fixture, A: implant surface at the apical 1/3rd of the fixture.(Fig. 2) Chemical composition was analyzed under a magnification of 10,000×. The mass concentration (C, wt%) ranges of element concentration achieved from the EDS were classified as: Major: C>0.1 mass fraction (greater than 10 wt%), Minor: 0.01≤C≤0.1 mass fraction (1 to 10 wt%), and Trace: C<0.01 mass fraction (less than 1 wt%).
The SEM-EDS analysis suggested mechanical failure of the implant.(Fig. 3, Table 1) In the area near the broken region of the fixture, microfracture lines were detected.(Fig. 2; U) EDS analysis of this region showed a homogenous oxidized titanium surface (titanium [Ti]: 82.41%, oxygen [O]: 12.04%). Minor levels of carbon (C: 3.98%) and silicon (Si: 1.57%) were recorded. Particles containing C and Si were observed in the elemental distribution map. There was no detection of other metal and non-metal elements in this area.
Morphologic SEM analysis of the layer of integrated bone tissue on the implant surface revealed a normal pattern with detection of bone lacunae.(Fig. 3; M, A) Both regions exhibited high levels of Ca and P (in 0102-M: Ca=23.23%, P=12.77%, in 0104-A: Ca=31.08%, P=15.70%). The Ca/P ratio of these bone regions was in the normal range: 1.82 at M and 1.98 at A. At the M point, a significantly high signal of gold (Au) was detected (10.99%). In addition, minor signs of Si and fluorine (F) and traces of sodium (Na) and aluminum (Al) were recorded. In A, the same high level of gold was detected (10.94%). Minor signs of Na and traces of Si also were recorded. Some irregular bone structures with a high percentage of organic matter was observed.(Fig. 3; 0102-B) High levels of C (30.10%), O (24.00%), and N (10.68%), and a low level of Ca confirmed the presence of organic matter over the bone surface. Noticeably, Au was detected at a pretty high level in this attached bone on the middle of the fixture (16.12%).
In the current case, the failed implant was a tissue-level implant from the ITI system (Straumann implant; Institut Straumann AG, Basel, Switzerland). Tissue-level implants are designed to reduce marginal bone loss after implant placement by placing the platform 1.8 or 2.8 mm above bone level. Some studies have reported that marginal bone loss is associated with screw and fixture fractures12,13. With the thick wall design, which can withstand heavy occlusal forces, tissue level implants are expected to be resistant to fracture. However, there are no studies comparing the prevalence of fractures between tissue-level and bone-level implants. Risk factors for implant fractures include type of prosthetic structure (single crown), presence of parafunction, inappropriate occlusion, marginal bone resorption, and screw loosening.
The SEM-EDS results revealed an image of a fractured fixture with both normal and irregular bone patterns. Microfractures and scratching lines were observed in the adjacent area of the broken area of the fixture, indicating a fatigue condition of the fixture, which might have been caused by screw loosening and its movement during function over a long period of time. The elemental distribution map revealed a homogeneous oxidized Ti surface with particles composed of C and Si. Most of the integrated bone layer on the fixture surface was of normal bone structure with bone lacunae. However, irregular bone structure with the presence of organic matter also was detected. In addition to major elements, there was the detection of metal and non-metal elements including N, Na, F, Si, Al, and Au. The C, N, and Si were suggested to be absorbed during the manufacture. In addition, Si contamination might have originated from the glass storage vials. The rubber gloves could be a source of Si and C, but the possibility is not high11. The Al could be residual from sandblasting surface treatment, while Na and F could have been absorbed from the intraoral environment. Noticeable, the level of Au on the implant surface and peri-implant tissue was significantly high in the middle and apical region of the implant. The Au ions were suggested to be derived from the corrosion process of the prosthetic, however, this phenomenon needs to be further studied for confirmation.
Shemtov-Yona and Rittel14 performed an SEM-EDS study for chemical composition identification of commercial pure Ti (CP-Ti) and Ti-6Al-4V retrieved from fractured dental implants. The authors reported that the fracture surfaces were covered with different layers, including the Ti surface of the fixture, organic layer (containing mainly C), and inorganic layer (containing mainly Ca and P, suggestive of bone material). It is theorized that metal fatigue is the main failure mechanism of a fractured implant, and the intra-oral environment might cause a significant reduction in implant fatigue performance14. Furthermore, abrasion on the surfaces of the implant-abutment connection during masticatory tooth movement can create wear particles8. Due to damage of the titanium oxide layer, the exposed fresh titanium surface reacts immediately with the environment, corresponding to an anodic partial current and a subsequent increase of the corrosion rate due to the high chemical reactivity of the bare metal8. The mechanical instability of implant-abutment connections appears to trigger a chain of synergistic negative events involving issues such as micro-gap widening, micromovements of the contacting surfaces, wear, ion and debris release, peri-implant inflammatory reactions, and peri-implantitis15. In this study, the turnover bone structures were detected, and trace amounts of corrosion metals such as Au and Ti in the bone tissue were recorded, which might have resulted from instability and micro-movement of the implant-abutment connection over an extended period of time. Further, in vivo and in vitro evidence is needed for confirmation of this phenomenon.
It is worth noting that screw fracture occurred at the same time as fixture fracture in this case. In screw-retained implants, the stress produced by the micro-movement of screws can create constant tension on the implant fixture. Therefore, screw loosening often causes implant fracture and can be considered a warning signal. In this case, the patient did not have the recall check-up for a long period, and the continuous use of the prosthesis with loosening screw might be the main cause of the implant fracture. This implies the importance of periodic maintenance. The clinician also should instruct the patient to visit the clinic as soon as whenever there is any sign of mobility of the prosthesis. Once the fracture happens and the failure implant is removed, re-installation of an implant with wider diameter can be performed immediately after implant removal. However, in cases explantation using trephine bur, the socket should be preserved with an allogeneic bone graft and a wide implant can be used to maximize the potential initial stability. Long-term periodic follow-up is essential and the prosthesis, abutment screw, and peri-implant tissue should be carefully examined to detect and prevent any biomechanical complications.
In conclusion, dental implant fracture is an infrequent but important cause of implant therapy failure. The SEM and EDS results from in current study give an enlightenment of the failed fixture surface micromorphology with microfracture and contaminated chemical compositions. Noticeably, the significantly high level of Au on the implant surface and the trace amounts of corrosion metals such as Au and Ti in the bone tissue were recorded, which might have resulted from instability and micro-movement of the implant-abutment connection over an extended period of time. Further study with larger number of patient and different types of implants is needed for further conclusion.
Notes
Authors’ Contributions
All authors read and approved the final manuscript. T.T.H.N. and M.Y.E. collected the data and wrote the manuscript, K.R.M. prepared the patient data, M.H.S. and H.M. revised and corrected the manuscript, S.M.K. designed and wrote the manuscript.
References
1. Albrektsson T, Zarb G, Worthington P, Eriksson AR. 1986; The long-term efficacy of currently used dental implants: a review and proposed criteria of success. Int J Oral Maxillofac Implants. 1:11–25. PMID: 3527955.
2. Berglundh T, Persson L, Klinge B. 2002; A systematic review of the incidence of biological and technical complications in implant dentistry reported in prospective longitudinal studies of at least 5 years. J Clin Periodontol. 29 Suppl 3:197–212. discussion 232–3. https://doi.org/10.1034/j.1600-051x.29.s3.12.x. DOI: 10.1034/j.1600-051X.29.s3.12.x. PMID: 12787220.
3. Rangert B, Krogh PH, Langer B, Van Roekel N. 1995; Bending overload and implant fracture: a retrospective clinical analysis. Int J Oral Maxillofac Implants. 10:326–34. PMID: 7615329.
4. Balshi TJ. 1996; An analysis and management of fractured implants: a clinical report. Int J Oral Maxillofac Implants. 11:660–6. PMID: 8908866.
5. Singh A, Singh A, Vivek R, Chaturvedi TP, Chauhan P, Gupta S. 2013; SEM analysis and management of fracture dental implant. Case Rep Dent. 2013:270385. https://doi.org/10.1155/2013/270385. DOI: 10.1155/2013/270385. PMID: 23710372. PMCID: PMC3655462.
6. Sánchez-Pérez A, Moya-Villaescusa MJ, Jornet-Garcia A, Gomez S. 2010; Etiology, risk factors and management of implant fractures. Med Oral Patol Oral Cir Bucal. 15:e504–8. https://doi.org/10.4317/medoral.15.e504. DOI: 10.4317/medoral.15.e504. PMID: 20038899.
7. Wee K, Lee BA. 2019; Implant fracture after screw fracture in a tissue-level implant: a case report. Implantology. 23:229–34. https://doi.org/10.32542/implantology.2019020. DOI: 10.32542/implantology.2019020.
8. Delgado-Ruiz R, Romanos G. 2018; Potential causes of titanium particle and ion release in implant dentistry: a systematic review. Int J Mol Sci. 19:3585. https://doi.org/10.3390/ijms19113585. DOI: 10.3390/ijms19113585. PMID: 30428596. PMCID: PMC6274707.
9. Hellsing M. 1997; Comparative surface analysis of four dental implant systems. J Dent Assoc S Afr. 52:399–402. PMID: 9461954.
10. Sawase T, Hai K, Yoshida K, Baba K, Hatada R, Atsuta M. 1998; Spectroscopic studies of three osseointegrated implants. J Dent. 26:119–24. https://doi.org/10.1016/s0300-5712(96)00080-2. DOI: 10.1016/S0300-5712(96)00080-2. PMID: 9540308.
11. Shibli JA, Marcantonio E, dʼAvila S, Guastaldi AC, Marcantonio E Jr. 2005; Analysis of failed commercially pure titanium dental implants: a scanning electron microscopy and energy-dispersive spectrometer x-ray study. J Periodontol. 76:1092–9. https://doi.org/10.1902/jop.2005.76.7.1092. DOI: 10.1902/jop.2005.76.7.1092. PMID: 16018751.
12. Romeed SA, Malik R, Dunne SM. 2013; Marginal bone loss influence on the biomechanics of single implant crowns. J Craniofac Surg. 24:1459–65. https://doi.org/10.1097/SCS.0b013e3182902db0. DOI: 10.1097/SCS.0b013e3182902db0. PMID: 23851832.
13. Jimbo R, Halldin A, Janda M, Wennerberg A, Vandeweghe S. 2013; Vertical fracture and marginal bone loss of internal-connection implants: a finite element analysis. Int J Oral Maxillofac Implants. 28:e171–6. https://doi.org/10.11607/jomi.3052. DOI: 10.11607/jomi.3052. PMID: 23869374.
14. Shemtov-Yona K, Rittel D. 2014; Identification of failure mechanisms in retrieved fractured dental implants. Eng Fail Anal. 38:58–65. https://doi.org/10.1016/j.engfailanal.2014.01.002. DOI: 10.1016/j.engfailanal.2014.01.002.
15. Apaza-Bedoya K, Tarce M, Benfatti CAM, Henriques B, Mathew MT, Teughels W, et al. 2017; Synergistic interactions between corrosion and wear at titanium-based dental implant connections: a scoping review. J Periodontal Res. 52:946–54. https://doi.org/10.1111/jre.12469. DOI: 10.1111/jre.12469. PMID: 28612506.
Fig. 1
Abutment of the implant was fractured at the same height level as the surrounding alveolar bone. A. A radiolucency was observed on the coronal portion of the fixture, indicating that the fixture body also was fractured (arrow). B. Axial computed tomography showed the location of the fracture at the mesiolingual aspect of the fixture body (arrows). C. The #37i fixture was retrieved with bony attachments after removal using a trephine bur.
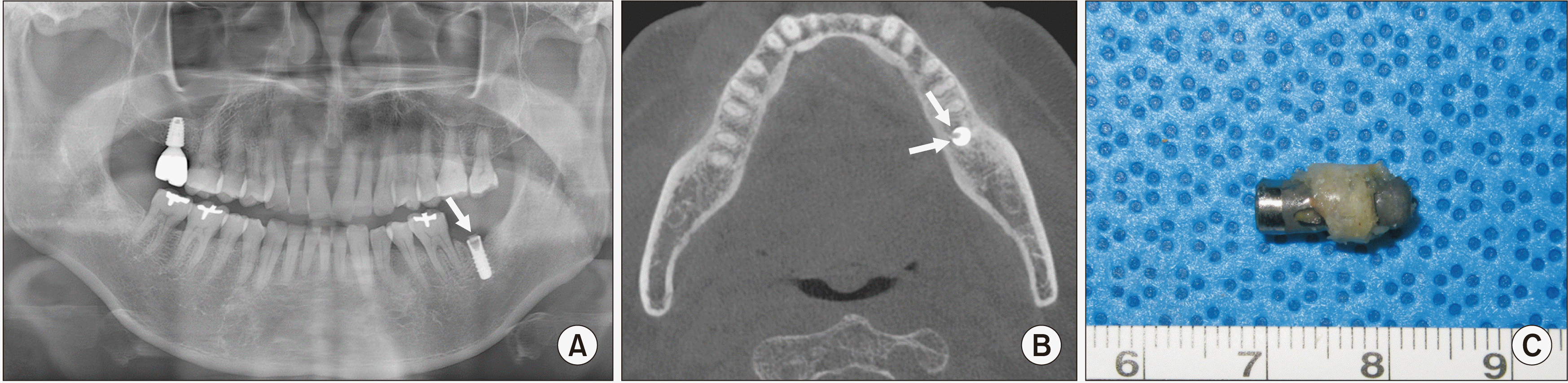
Fig. 2
Scanning electron microscopy photograph of the failed implant at ×65 magnification. The regions of interest examined by energy dispersive X-ray spectroscopy at ×10,000 magnifications are marked with red rectangles. U: surface in the upper area of the fixture, adjacent to the fracture region, M: implant surface at the middle of the implant fixture, B: attached bone tissue in the middle region of the fixture, A: implant surface in the apical region of the implant.
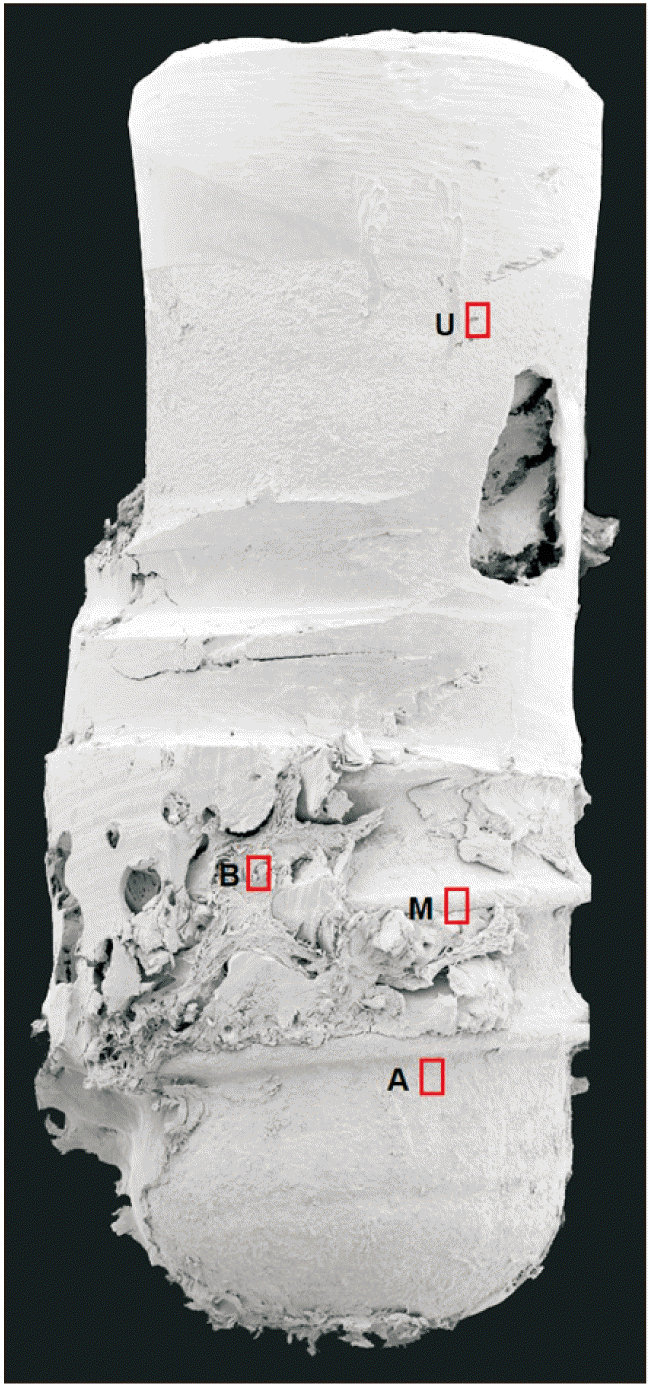
Fig. 3
Scanning electron microscopy-energy dispersive X-ray spectroscopy (SEM-EDS) analysis of the failed implant. SEM micrograph at ×500 and ×10,000 magnifications, EDS elemental distribution map, and spectrum of representative points on the failed implants. U: microfracture (white arrowheads) and scratching lines (black arrows) are observed. The elemental distribution map showed a homogeneous oxidized Ti surface with particles composed of C and Si. M: normal bone structure with bony lacunae covering the fixture surface (blue arrowheads). The Ti surface was detected on EDS in the region with a thin bone layer (black arrowheads). B: SEM images showing irregular bone structure with the presence of organic matter (blue arrowheads). EDS results reveal the presence of Na, F, N, Si, and Au. A: normal bone structure with lacunae covering the fixture surface (blue arrowheads). Trace amounts of Ti can be observed in the elemental distribution map (black arrowheads). (cps/eV: count per second per electron-volt)

Table 1
Energy dispersive X-ray spectroscopy (EDS) data and surface morphology of the scanning electron microscopy (SEM) results