Abstract
Background/Aims
Methods
Results
ACKNOWLEDGEMENTS
Notes
FINANCIAL SUPPORT: This study was supported in part by Health and Labour Sciences Research Grants for research on intractable diseases from the Japanese Ministry of Health.
AUTHOR CONTRIBUTION: Mori K, Naganuma M, Mizuno S, Suzuki H, Matsuoka K, Hisamatsu T and Kanai T participated in the design of the study; Kitazume M, Shimamura K and Sugita A collected samples of this study; FACS staining and PCR analysis was performed by Mori K, Suzuki H and Chiba S; Statistical analysis was done by Mori K and Hisamatsu T; Naganuma M, Mizuno S and Hisamatsu T drafted the first version of the manuscript; all authors were involved in the writing process, read, and approved the final manuscript.
References




























SUPPLEMENTARY MATERIALS
Supplementary Fig. 1.
Supplementary Fig. 2.
Fig. 1
Differentiated Mγ-Mφs express CD206 and CD209. Cell surface staining. Peripheral blood mononuclear cells were gated on the monocyte gate forward scatter (FSC)/CD14+ before (A) and after (B) using CD14+ MACS. The numbers are the ratio of CD14+ cells in all monocytes. (C) Cell surface molecule of M-Mφs or Mγ-Mφs. The open histograms show the isotype control. The filled histograms show the cells. M-Mφs, macrophages differentiated in vitro by macrophage colony-stimulating factor alone; Mγ-Mφs, macrophages differentiated in vitro by macrophage colony-stimulating factor and interferon-γ.
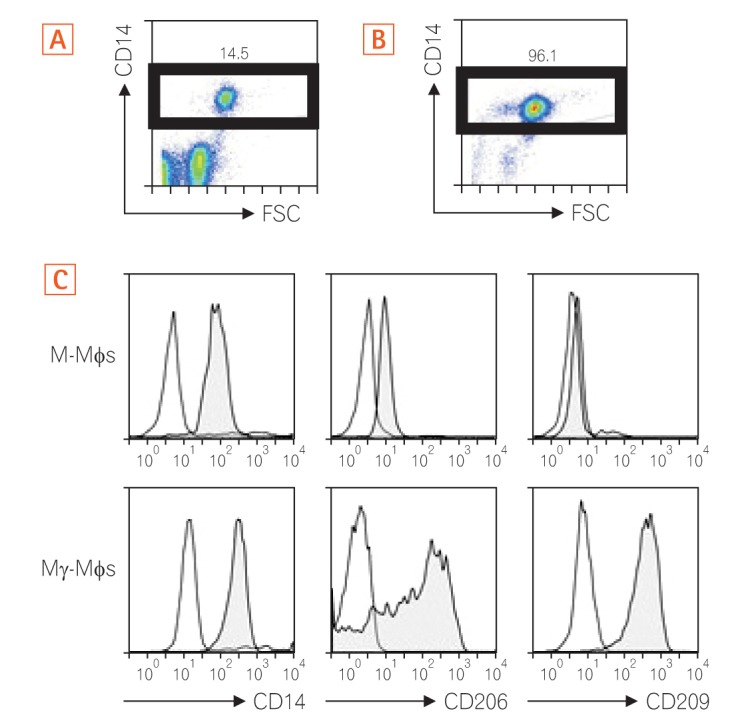
Fig. 2
Mγ-Mφs produce a large amount of inflammatory cytokines in response to β-(1,3)-glucan. Cytokine production (A, TNF-α; B, IL-6; C, IL-1β) by macrophages derived from peripheral blood cells from healthy controls (n=6) in response to β-(1,3)-glucan (A, 20 µg/mL; B and C, 100 µg/mL) was analyzed using a cytometric bead array kit. CD14+ monocytes were differentiated in the presence of M-CSF (M-macrophages, M-Mφs) or M-CSF and IFN-γ (M-gamma macrophages, Mγ-Mφs). M-Mφs and Mγ-Mφs (1×106 cells/mL) were stimulated with β-(1,3)-glucan for 24 hours. Statistical analysis was performed using the Mann-Whitney U-test. a P<0.001. TNF, tumor necrosis factor; IL, interleukin; M-CSF, macrophage colony-stimulating factor; IFN, interferon.
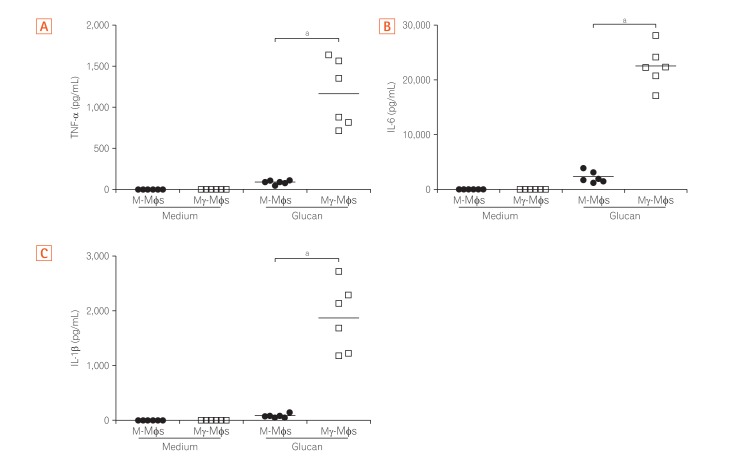
Fig. 3
Mγ-Mφs induction of proinflammatory cytokine via Dectin-1. (A) Expression of Dectin-1 mRNA in M-Mφs and Mγ-Mφs was examined using quantitative RT-PCR. Statistical analysis was performed using the Mann-Whitney U-test (a P<0.01). (B) Expression of Dectin-1, the receptor for β-(1,3)-glucan, was examined by flow cytometry. (C) Expression of Dectin-1 was also investigated by western blotting using CD14+ monocytes, M-Mφs, and Mγ-Mφs. Some isotypes of Dectin-1, Dectin-1A and 1B, comprise the 15 kDa band. (D) Mγ-Mφs from healthy controls (n=4) were cultured in the presence of isotype, 10 µg/mL anti-Dectin-1 antibody or 10 µg/mL anti-Dectin-2 antibody for 1 hour. Tumor necrosis factor-α (TNF-α) production by Mγ-Mφs in response to heat-killed Candida albicans was analyzed using a cytometric bead array kit. Statistical analysis was performed using the Mann-Whitney U-test (b P<0.05). M-Mφs, macrophages differentiated in vitro by macrophage colony-stimulating factor alone; Mγ-Mφs, macrophages differentiated in vitro by macrophage colony-stimulating factor and interferon-γ.
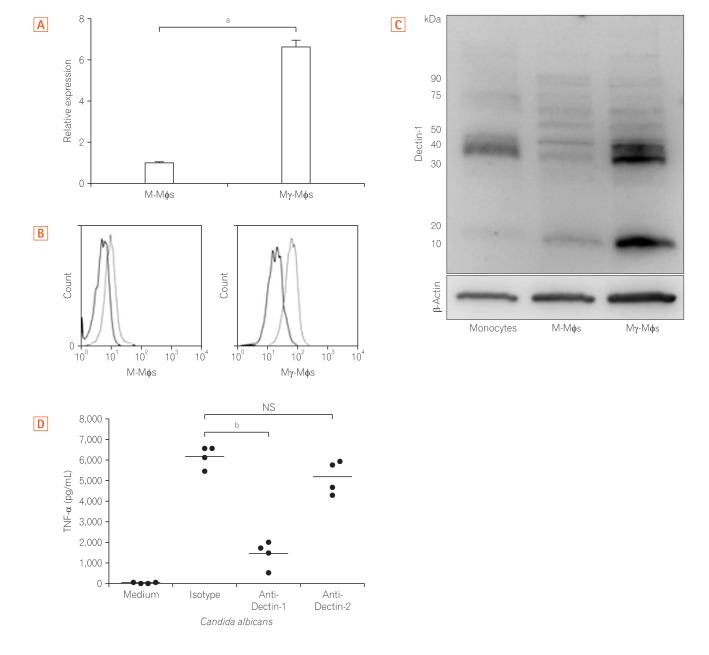
Fig. 4
Dectin-1 induces tumor necrosis factor-α (TNF-α) production by Mγ-Mφs and lamina propria macrophages in a Syk and Raf-1-dependent manner. (A) Mγ-Mφs were cultured in the presence of isotype or 10 µg/mL anti-Dectin-1 antibody for 1 hour, and TNF-α production by Mγ-Mφs in response to β-(1,3)-glucan or lipopolysaccharide (LPS) was analyzed by flow cytometry (n=3). Statistical analysis was performed using the Mann-Whitney U-test (a P<0.05). (B) Mγ-Mφs were cultured in the presence of isotype, 10 µM Raf-1 inhibitor or 20 µM Syk inhibitor for 1 hour, and TNF-α production by Mγ-Mφs in response to β-(1,3)-glucan was analyzed by flow cytometry (n=4). Statistical analysis was performed using the Mann-Whitney U-test (a P<0.05). (C) CD3–CD14+ monocytes were isolated from lamina propria mononuclear cells from patients with CD (n=4) and cultured in the presence of isotype or 10 µg/mL anti-Dectin-1 antibody for 1 hour. TNF-α production in response to heat-killed Candida albicans or Enterococcus faecalis was analyzed using a cytometric bead array kit. Statistical analysis was performed using the Mann-Whitney U-test (b P<0.01). M-Mφs, macrophages differentiated in vitro by macrophage colony-stimulating factor alone; Mγ-Mφs, macrophages differentiated in vitro by macrophage colony-stimulating factor and interferon-γ; CA, Candida albicans; EF, Enterococcus faecalis.
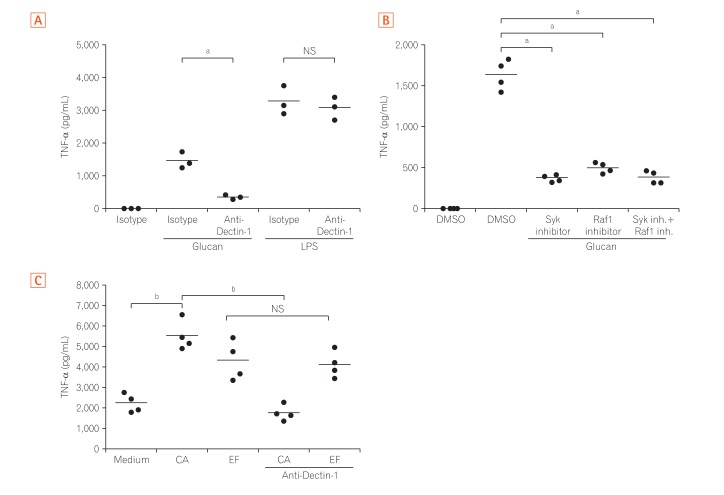