Abstract
To maintain normal glucose homeostasis after a meal, it is essential to secrete an adequate amount of insulin from pancreatic β-cells. However, if pancreatic β-cells solely depended on the blood glucose level for insulin secretion, a surge in blood glucose levels would be inevitable after the ingestion of a large amount of carbohydrates. To avoid a deluge of glucose in the bloodstream after a large carbohydrate- rich meal, enteroendocrine cells detect the amount of nutrient absorption from the gut lumen and secrete incretin hormones at scale. Since insulin secretion in response to incretin hormones occurs only in a hyperglycemic milieu, pancreatic β-cells can secrete a “Goldilocks” amount of insulin (i.e., not too much and not too little) to keep the blood glucose level in the normal range. In this regard, pancreatic β-cell sensitivity to glucose and incretin hormones is crucial for maintaining normal glucose homeostasis. In this Namgok lecture 2022, we review the effects of current anti-diabetic medications on pancreatic β-cell sensitivity to glucose and incretin hormones.
Blood glucose levels are tightly regulated within a very narrow range in individuals with normal glucose tolerance, which is maintained by the coordinated action of islet and incretin hormones, as well as neural and humoral factors from different tissues and organs. Among them, the action of incretin hormones, known as the incretin effect, contributes to 50% to 70% of insulin secretion after the oral ingestion of glucose in healthy subjects [1]. To date, two incretin hormones have been discovered: glucagon-like peptide-1 (GLP-1) and glucose-dependent insulinotropic polypeptide (GIP) [2]. The amount of GLP-1 and GIP secretion is proportional to the amount of ingested carbohydrates [1,3], and these hormones send signals about the anticipated influx of glucose from the gut lumen so that the pancreatic β-cells secrete an appropriate amount of insulin to prevent excessive excursions of blood glucose levels. Both hormones augment insulin secretion in a glucose-dependent manner [4], which is a fail-safe mechanism that minimizes the risk of hypoglycemia even in a situation with excessive secretion of incretin hormones. Various pharmaceutical agents based on incretin hormones have been developed for the treatment of type 2 diabetes [5,6]. Considering the unique nature of glucose-dependent insulin secretion in response to incretin hormones, it would be timely to review the pancreatic β-cell response to glucose and incretin hormones and how it is modulated by current anti-diabetic medications.
Increased blood glucose levels and incretin hormones are the major stimuli of insulin secretion. Thus, in a simplified model as shown in the following equation, the insulin secretion rate is determined by β-cell glucose sensitivity and the blood glucose level, β-cell GLP-1 sensitivity and the blood GLP-1 level, and β-cell GIP sensitivity and the blood GIP level [7].
I, insulin; k1, β-cell glucose sensitivity; BG, blood glucose level; kglp-1, β-cell GLP-1 sensitivity; GLP-1,blood GLP-1 level; kgip, β-cell GIP sensitivity; GIP, blood GIP level
In vivo measurements of β-cell glucose sensitivity are made by calculating the slope of the insulin secretion rate versus the blood glucose level during a graded glucose infusion study, which gradually raises blood glucose levels, typically from 5 to 12 mmol/L (Fig. 1A) [8]. As illustrated in Fig. 1B, β-cell glucose sensitivity is markedly lower in patients with type 2 diabetes than in individuals with normal glucose tolerance [8].
Meanwhile, β-cell incretin sensitivity is measured in vivo by performing a hyperglycemic clamp study with GLP-1 and/or GIP infusion (Fig. 2). The increment of insulin secretion in response to GLP-1 or GIP infusion during the hyperglycemic clamp study indicates the β-cell incretin sensitivity [9-11]. In one study, the infusion of GLP-1 and GIP increased plasma insulin levels by 4.1 and 3.6 times, respectively, compared to a saline infusion in healthy subjects during a hyperglycemic clamp procedure [10]. In the same study, β-cell incretin sensitivity was markedly lower in patients with type 2 diabetes [10].
Defective insulin secretion is the cornerstone of the pathophysiology of type 2 diabetes. As shown in Equation 1, pancreatic β-cell sensitivity to glucose, incretin, or both is critical for insulin secretion. Therefore, restoring pancreatic β-cell sensitivity to glucose and/or incretin hormones is essential for improving glycemic control in patients with type 2 diabetes. Since chronic hyperglycemia itself impairs β-cell function [12], pharmacologic interventions to reduce hyperglycemia may improve β-cell function and restore β-cell glucose sensitivity [13].
The effect of a GLP-1 receptor agonist, liraglutide, on β-cell glucose sensitivity was examined in a placebo-controlled crossover study involving 10 patients with well-controlled type 2 diabetes (mean hemoglobin A1c [HbA1c], 6.5%) and 10 healthy subjects [8]. Remarkably, a single injection of liraglutide restored β-cell glucose sensitivity, which became similar to that of healthy subjects [8]. In another study, 45 patients with poorly controlled type 2 diabetes (mean HbA1c, 8.4%) were randomized to canagliflozin, liraglutide, or both on top of their existing treatment regimen [14], and β-cell glucose sensitivity was calculated by a mathematical model using data from oral glucose tolerance tests with frequent sampling for insulin and C-peptide. Both canagliflozin and liraglutide significantly decreased HbA1c (by 0.89% and 1.43%, respectively) and increased β-cell glucose sensitivity (from 29±5 to 55±11 and from 33±6 to 101± 16, respectively). No meaningful additive effect was found in participants who received both canagliflozin and liraglutide. The magnitude of HbA1c reduction was closely correlated with the increase in β-cell glucose sensitivity, suggesting that alleviating glucose toxicity would be important for improving β-cell glucose sensitivity. However, it is noteworthy that incretin hormones may exert direct effects on pancreatic β-cell function by reducing endoplasmic reticulum stress, enhancing autophagy, and preventing apoptosis [15,16].
The effect of a dipeptidyl peptidase-4 (DPP-4) inhibitor on β-cell glucose sensitivity was calculated by mathematical modeling using data from a mixed meal test and a corresponding isoglycemic intravenous glucose infusion that exactly copied the blood glucose changes during the mixed meal test [17]. In that study, which involved 50 patients with type 2 diabetes (mean HbA1c, 7.4%), sitagliptin or placebo was given for 6 weeks. Compared to the placebo, sitagliptin significantly improved β-cell glucose sensitivity during the mixed meal test and the corresponding isoglycemic procedure, but β-cell glucose sensitivity failed to reach the level of control subjects without diabetes [17].
Insulin secretion from pancreatic β-cells in response to incretin hormones is determined by the tissue levels of incretin hormones and signal transduction through incretin receptors. Although there have been mixed results with regard to the amount of incretin secretion, a meta-analysis showed that the secretion of GLP-1 and GIP is not reduced in patients with type 2 diabetes compared to their counterparts without diabetes [18,19]. Therefore, the secretion of GLP-1 and GIP does not play a critical role in impaired insulin secretion in patients with type 2 diabetes in general. However, in special situations with exposure to a massive amount of GLP-1 receptor agonists, such as in GLP-1 analog treatment or post-gastric bypass surgery, the tissue level of GLP-1 or its analog is important for determining pancreatic β-cell function. Otherwise, the expression level of the receptors for GLP-1 and/or GIP (hereafter, GLP-1R and GIPR, respectively) in pancreatic β-cells is a critical factor in determining the action of the incretin hormones [20].
Both genetic and environmental factors contribute to the expression level of GLP-1R and GIPR. Decreased GLP-1R and GIPR expression was observed in islets isolated from carriers of the type 2 diabetes risk allele of transcription factor 7 like 2 (TCF7L2) (rs7903146) [21]. In addition, individuals harboring the type 2 diabetes risk allele of Wolfram syndrome 1 gene (WFS1) (rs10010131) displayed reduced β-cell GLP-1 sensitivity as assessed by a hyperglycemic clamp with GLP-1 infusion [22]. It is also noteworthy that hyperglycemia itself may reduce incretin hormone receptor expression by mechanisms including glucose toxicity [23]. Several medications have the potential to restore β-cell sensitivity to incretin hormones (Table 1). In addition, downstream of the GLP-1 receptor, β-arrestin is an important modulator of the action of GLP-1 in pancreatic β-cells by regulating the internalization of GLP-1R [24]. A recent large genome-wide association study of type 2 diabetes patients who were treated with GLP-1R agonists reported that low-frequency variants of ARRB1 encoding β-arrestin were associated with better responses to GLP-1R agonists [25]. These variants may explain the pharmacogenomic differences in the efficacy of GLP-1R agonists among different ethnicities.
In the current armamentarium for the treatment of type 2 diabetes, insulin is the most effective drug to rapidly correct hyperglycemia. In a small study including eight obese patients with poorly controlled type 2 diabetes, intensive insulin therapy was provided for 4 weeks, targeting a pre-meal blood glucose level of 5 mmol/L [10]. After the treatment, HbA1c decreased from 8.6% to 7.4%. A hyperglycemic clamp with GIP and GLP-1 infusion was performed before and after intensive insulin treatment. Insulin secretion in response to the GLP-1 and GIP infusion increased by 3.4- and 5.6-fold from the baseline values after intensive insulin therapy. The basal insulin secretion without GLP-1 and GIP infusion also increased, suggesting that intensive insulin therapy improved β-cell function in general and β-cell incretin sensitivity in particular. Since chronic hyperglycemia imposes deleterious effects on β-cell function by building up glucose toxicity [26], ameliorating glucose toxicity with intensive insulin therapy may restore β-cell function [27,28] and improve β-cell incretin sensitivity through a similar mechanism.
Sulfonylureas stimulate insulin secretion in a non-glucose-dependent manner by closing the ATP-sensitive potassium channel (KATP) channel. These medications potentiate β-cell responses not only to glucose but also to non-glucose stimuli [29]. A small Canadian study administered glyburide for a month to five newly diagnosed type 2 diabetes patients, and glyburide decreased their fasting blood glucose levels from 12.8±1.8 to 8.5±0.8 mmol/L [9]. A hyperglycemic clamp with GIP infusion performed before and after glyburide treatment showed improvements in β-cell glucose sensitivity by 58% and β-cell GIP sensitivity by 130% [9]. Like insulin, sulfonylureas also improve both β-cell glucose sensitivity and GIP sensitivity by ameliorating hyperglycemia in patients with poorly controlled type 2 diabetes.
The mechanism of action of metformin in glycemic control is very complex and involves multiple pathways. With regard to the incretin system, metformin is both an enhancer of GLP-1 secretion and a sensitizer of GLP-1 and GIP action [30]. Metformin stimulates GLP-1 secretion from the intestine and increases plasma levels of GLP-1 both in mice and humans [31,32]. The underlying mechanism of GLP-1 secretion in response to metformin is poorly understood. However, a possibility is that metformin may inhibit the apical sodium-dependent bile acid transporter, which in turn increases the concentration of bile acids in the intestinal lumen [33]. Then, the increased bile acids in the lumen may activate the G-protein-coupled receptor, Takeda-G-protein receptor 5 (TGR5) signaling, which stimulates GLP-1 secretion from enteroendocrine L-cells [34]. In addition, metformin may directly stimulate GLP-1 secretion by modulating AMP-activated kinase (AMPK) activity based on an ex vivo experiment using human ileal and colonic tissue [35]. Of note, metformin and a DPP-4 inhibitor additively increase active GLP-1 levels in the plasma [32], which confers clinical benefits in the management of type 2 diabetes.
Intriguingly, some clues suggest a GLP-1 sensitizing action of metformin in pancreatic β-cells. It was reported that metformin directly increased GLP-1R expression in the INS-1 β-cell line [31]. Metformin also increased both GLP-1R and GIPR levels in mice in a peroxisome proliferator-activated receptor α (PPAR-α)-dependent manner [31]. In our own study, 4-week metformin treatment also increased GLP-1R expression in pancreatic β-cells in Goto-Kakizaki rats, a non-obese type 2 diabetes animal model [36]. However, we did not observe any increase in GLP-1R expression after fenofibrate, a PPAR-α agonist, treatment. The discrepancy between these two studies might be explained by the nature of endogenous or pharmacologic ligands for PPAR-α and different animal species and experimental settings.
The main action of DPP-4 inhibitors is augmenting active GLP-1 and GIP levels in the plasma by inhibiting their enzymatic degradation. In addition to this, DPP-4 inhibitors may increase the action of incretin hormones. In a study involving 24 patients with inadequately controlled type 2 diabetes using metformin at baseline (mean HbA1c, 7.8%), sitagliptin or placebo was given on top of metformin for 12 weeks [37]. The GIP or GLP-1 sensitivity of β-cells was estimated by performing a hyperglycemic clamp with the infusion of saline, GIP, or GLP-1. The baseline GLP-1 sensitivity was 3- to 4-fold higher than that of GIP. Interestingly, sitagliptin treatment improved only β-cell GIP sensitivity by 35% compared to the placebo [37]. It was not clear why β-cell GLP-1 sensitivity did not improve in response to sitagliptin in that study. However, the baseline GLP-1 sensitivity was not exactly evaluated because the baseline hyperglycemic clamp was performed 2 to 7 days after treatment initiation [37].
Phlorizin (a non-specific sodium-glucose co-transporter [SGLT] 1/SGLT2 inhibitor) increased both GLP-1R and GIPR expression in the pancreatic islets of 90% pancreatectomized hyperglycemic rats [38]. Zucker diabetic fatty rats showed decreased GIPR expression in the pancreatic islets, which was restored by phlorizin treatment [39]. A pancreatic perfusion study revealed that insulin secretion improved during GIP infusion in phlorizin-treated rats as compared with vehicle-treated rats [39]. The effects of phlorizin on the incretin sensitivity of pancreatic β-cells suggest that selective SGLT2 inhibitors may also have such salutary effects.
We previously measured the incretin sensitivity of pancreatic β-cells in 10 subjects with normal glucose tolerance and 19 subjects with type 2 diabetes using a hyperglycemic clamp with the sequential addition of GLP-1 and GIP infusions [11]. Eight-week dapagliflozin treatment in patients with type 2 diabetes reduced the mean HbA1c from 7.9% to 7.1%. Dapagliflozin treatment significantly increased insulin secretion upon sequential addition of GLP-1 and GIP during the hyperglycemic clamp study (Fig. 2). The improvements in β-cell sensitivity to glucose, GLP-1, and GIP were closely correlated with the improvement of HbA1c, suggesting that the amelioration of glucose toxicity could be an important factor for improving pancreatic β-cell sensitivity to glucose, GLP-1, and GIP in patients with type 2 diabetes.
As discussed above, metformin has the unique property of being both an incretin enhancer and an incretin sensitizer. Therefore, a combination of metformin and incretin-based drugs would be a good therapeutic option for the treatment of type 2 diabetes. Indeed, the combination of a GLP-1R agonist or DPP-4 inhibitor with metformin provided additive glycemic improvements in patients with inadequately controlled type 2 diabetes [40-42].
Combining incretin-based drugs and sulfonylureas is another commonly used treatment option for type 2 diabetes. Although DPP-4 inhibitors are known to have a very low risk for hypoglycemia based on their mechanism of action, the concomitant use of a sulfonylurea increases the hypoglycemia risk [43]. In Japan, during the first 6 months after the launch of sitagliptin, cases of severe hypoglycemia increased when sitagliptin was added onto a sulfonylurea, especially in elderly patients with renal insufficiency [44]. Interestingly, there is cross-talk between sulfonylurea and incretin signaling involving the activation of the exchange protein activated by cyclic-AMP 2A (Epac2A)-Ras-like small GTPase 1 (RAP1) pathway, which may augment insulin secretion from pancreatic β-cells upon exposure to the combination of both drugs [45,46]. Therefore, caution should be exercised to prevent hypoglycemia when adding incretin-based therapy to pre-existing sulfonylurea therapy in patients with type 2 diabetes.
Regarding the positive effect of SGLT2 inhibitors on β-cell sensitivity to incretin hormones, the combination of incretin-based therapies and SGLT2 inhibitors could be a very effective option for anti-diabetic treatment. In our systematic review and meta-analysis evaluating the clinical efficacy and safety of combinations of DPP-4 inhibitors and SGLT2 inhibitors in patients with inadequately controlled type 2 diabetes, the combination of an SGLT2 inhibitor and a DPP-4 inhibitor showed a greater reduction in HbA1c (weighted mean difference, −0.6%), fasting plasma glucose, and 2-hour postprandial plasma glucose levels than the combination of a placebo with a DPP-4 inhibitor [47]. However, unlike our expectations, a synergistic effect of SGLT2 inhibitor and DPP-4 inhibitor use was not observed in these clinical trials, which could be attributed, at least partially, to the opposite action on glucagon secretion by the two classes of drugs.
For normal postprandial glucose metabolism, it is crucial to maintain adequate β-cell glucose, GLP-1, and GIP sensitivity. Several anti-diabetic medications have shown the potential to improve β-cell incretin sensitivity (Table 1). Evidence indicates that sulfonylureas, DPP-4 inhibitors, SGLT2 inhibitors, and insulin improve β-cell incretin sensitivity in humans. In addition, experimental evidence for metformin and phlorizin has been reported in animal studies. The improvement of β-cell incretin sensitivity by these different classes of anti-diabetic medications suggests a shared mechanism: the amelioration of glucose toxicity (Fig. 3), which provides a mechanistic insight into the use of combination treatment including incretin-based therapy for type 2 diabetes.
Notes
The Namgok Award is the highest scientific award of the Korean Endocrine Society, and is given to honor an individual who has made excellent contributions to progress in the field of endocrinology and metabolism. The Namgok Award is named after the pen name of Professor Hun Ki Min, who founded the Korean Endocrine Society in 1982. Professor Young Min Cho received the Namgok Award at the 10th Seoul International Congress of Endocrinology and Metabolism of the Korean Endocrine Society in October 2022.
REFERENCES
1. Nauck MA, Homberger E, Siegel EG, Allen RC, Eaton RP, Ebert R, et al. Incretin effects of increasing glucose loads in man calculated from venous insulin and C-peptide responses. J Clin Endocrinol Metab. 1986; 63:492–8.


3. Vilsboll T, Krarup T, Sonne J, Madsbad S, Volund A, Juul AG, et al. Incretin secretion in relation to meal size and body weight in healthy subjects and people with type 1 and type 2 diabetes mellitus. J Clin Endocrinol Metab. 2003; 88:2706–13.


4. Cho YM. Incretin physiology and pathophysiology from an Asian perspective. J Diabetes Investig. 2015; 6:495–507.
5. Cho YM, Fujita Y, Kieffer TJ. Glucagon-like peptide-1: glucose homeostasis and beyond. Annu Rev Physiol. 2014; 76:535–59.


6. Nauck MA, D’Alessio DA. Tirzepatide, a dual GIP/GLP-1 receptor co-agonist for the treatment of type 2 diabetes with unmatched effectiveness regrading glycaemic control and body weight reduction. Cardiovasc Diabetol. 2022; 21:169.


7. Kim M, Oh TJ, Lee JC, Choi K, Kim MY, Kim HC, et al. Simulation of oral glucose tolerance tests and the corresponding isoglycemic intravenous glucose infusion studies for calculation of the incretin effect. J Korean Med Sci. 2014; 29:378–85.


8. Chang AM, Jakobsen G, Sturis J, Smith MJ, Bloem CJ, An B, et al. The GLP-1 derivative NN2211 restores beta-cell sensitivity to glucose in type 2 diabetic patients after a single dose. Diabetes. 2003; 52:1786–91.
9. Meneilly GS, Bryer-Ash M, Elahi D. The effect of glyburide on beta-cell sensitivity to glucose-dependent insulinotropic polypeptide. Diabetes Care. 1993; 16:110–4.
10. Hojberg PV, Vilsboll T, Rabol R, Knop FK, Bache M, Krarup T, et al. Four weeks of near-normalisation of blood glucose improves the insulin response to glucagon-like peptide-1 and glucose-dependent insulinotropic polypeptide in patients with type 2 diabetes. Diabetologia. 2009; 52:199–207.


11. Ahn CH, Oh TJ, Kwak SH, Cho YM. Sodium-glucose cotransporter-2 inhibition improves incretin sensitivity of pancreatic β-cells in people with type 2 diabetes. Diabetes Obes Metab. 2018; 20:370–7.


12. Weir GC, Marselli L, Marchetti P, Katsuta H, Jung MH, Bonner-Weir S. Towards better understanding of the contributions of overwork and glucotoxicity to the beta-cell inadequacy of type 2 diabetes. Diabetes Obes Metab. 2009; 11 Suppl 4:82–90.
13. DeFronzo RA, Abdul-Ghani MA. Preservation of β-cell function: the key to diabetes prevention. J Clin Endocrinol Metab. 2011; 96:2354–66.


14. Ali AM, Mari A, Martinez R, Al-Jobori H, Adams J, Triplitt C, et al. Improved beta cell glucose sensitivity plays predominant role in the decrease in HbA1c with Cana and Lira in T2DM. J Clin Endocrinol Metab. 2020; 105:dgaa494.


15. Chon S, Gautier JF. An update on the effect of incretinbased therapies on β-cell function and mass. Diabetes Metab J. 2016; 40:99–114.


16. Holz GG, Kuhtreiber WM, Habener JF. Pancreatic betacells are rendered glucose-competent by the insulinotropic hormone glucagon-like peptide-1(7-37). Nature. 1993; 361:362–5.


17. Muscelli E, Casolaro A, Gastaldelli A, Mari A, Seghieri G, Astiarraga B, et al. Mechanisms for the antihyperglycemic effect of sitagliptin in patients with type 2 diabetes. J Clin Endocrinol Metab. 2012; 97:2818–26.


18. Calanna S, Christensen M, Holst JJ, Laferrere B, Gluud LL, Vilsboll T, et al. Secretion of glucagon-like peptide-1 in patients with type 2 diabetes mellitus: systematic review and meta-analyses of clinical studies. Diabetologia. 2013; 56:965–72.


19. Calanna S, Christensen M, Holst JJ, Laferrere B, Gluud LL, Vilsboll T, et al. Secretion of glucose-dependent insulinotropic polypeptide in patients with type 2 diabetes: systematic review and meta-analysis of clinical studies. Diabetes Care. 2013; 36:3346–52.
20. Cho YM, Merchant CE, Kieffer TJ. Targeting the glucagon receptor family for diabetes and obesity therapy. Pharmacol Ther. 2012; 135:247–78.


21. Shu L, Matveyenko AV, Kerr-Conte J, Cho JH, McIntosh CH, Maedler K. Decreased TCF7L2 protein levels in type 2 diabetes mellitus correlate with downregulation of GIP- and GLP-1 receptors and impaired beta-cell function. Hum Mol Genet. 2009; 18:2388–99.


22. Schafer SA, Mussig K, Staiger H, Machicao F, Stefan N, Gallwitz B, et al. A common genetic variant in WFS1 determines impaired glucagon-like peptide-1-induced insulin secretion. Diabetologia. 2009; 52:1075–82.


23. Rajan S, Dickson LM, Mathew E, Orr CM, Ellenbroek JH, Philipson LH, et al. Chronic hyperglycemia downregulates GLP-1 receptor signaling in pancreatic β-cells via protein kinase A. Mol Metab. 2015; 4:265–76.


24. Sonoda N, Imamura T, Yoshizaki T, Babendure JL, Lu JC, Olefsky JM. Beta-arrestin-1 mediates glucagon-like peptide-1 signaling to insulin secretion in cultured pancreatic beta cells. Proc Natl Acad Sci U S A. 2008; 105:6614–9.
25. Dawed AY, Mari A, Brown A, McDonald TJ, Li L, Wang S, et al. Pharmacogenomics of GLP-1 receptor agonists: a genome-wide analysis of observational data and large randomised controlled trials. Lancet Diabetes Endocrinol. 2023; 11:33–41.
26. Bensellam M, Laybutt DR, Jonas JC. The molecular mechanisms of pancreatic β-cell glucotoxicity: recent findings and future research directions. Mol Cell Endocrinol. 2012; 364:1–27.


27. Garvey WT, Olefsky JM, Griffin J, Hamman RF, Kolterman OG. The effect of insulin treatment on insulin secretion and insulin action in type II diabetes mellitus. Diabetes. 1985; 34:222–34.


28. Hidaka H, Nagulesparan M, Klimes I, Clark R, Sasaki H, Aronoff SL, et al. Improvement of insulin secretion but not insulin resistance after short term control of plasma glucose in obese type II diabetics. J Clin Endocrinol Metab. 1982; 54:217–22.


29. Pfeifer MA, Halter JB, Graf R, Porte D. Potentiation of insulin secretion to nonglucose stimuli in normal man by tolbutamide. Diabetes. 1980; 29:335–40.


30. Cho YM, Kieffer TJ. New aspects of an old drug: metformin as a glucagon-like peptide 1 (GLP-1) enhancer and sensitiser. Diabetologia. 2011; 54:219–22.


31. Maida A, Lamont BJ, Cao X, Drucker DJ. Metformin regulates the incretin receptor axis via a pathway dependent on peroxisome proliferator-activated receptor-α in mice. Diabetologia. 2011; 54:339–49.


32. Shin D, Cho YM, Lee S, Lim KS, Kim JA, Ahn JY, et al. Pharmacokinetic and pharmacodynamic interaction between gemigliptin and metformin in healthy subjects. Clin Drug Investig. 2014; 34:383–93.


33. Scarpello JH, Hodgson E, Howlett HC. Effect of metformin on bile salt circulation and intestinal motility in type 2 diabetes mellitus. Diabet Med. 1998; 15:651–6.


34. Thomas C, Gioiello A, Noriega L, Strehle A, Oury J, Rizzo G, et al. TGR5-mediated bile acid sensing controls glucose homeostasis. Cell Metab. 2009; 10:167–77.


35. Bahne E, Sun EW, Young RL, Hansen M, Sonne DP, Hansen JS, et al. Metformin-induced glucagon-like peptide-1 secretion contributes to the actions of metformin in type 2 diabetes. JCI Insight. 2018; 3:e93936.


36. Oh TJ, Shin JY, Kang GH, Park KS, Cho YM. Effect of the combination of metformin and fenofibrate on glucose homeostasis in diabetic Goto-Kakizaki rats. Exp Mol Med. 2013; 45:e30.


37. Aaboe K, Akram S, Deacon CF, Holst JJ, Madsbad S, Krarup T. Restoration of the insulinotropic effect of glucose-dependent insulinotropic polypeptide contributes to the antidiabetic effect of dipeptidyl peptidase-4 inhibitors. Diabetes Obes Metab. 2015; 17:74–81.


38. Xu G, Kaneto H, Laybutt DR, Duvivier-Kali VF, Trivedi N, Suzuma K, et al. Downregulation of GLP-1 and GIP receptor expression by hyperglycemia: possible contribution to impaired incretin effects in diabetes. Diabetes. 2007; 56:1551–8.
39. Piteau S, Olver A, Kim SJ, Winter K, Pospisilik JA, Lynn F, et al. Reversal of islet GIP receptor down-regulation and resistance to GIP by reducing hyperglycemia in the Zucker rat. Biochem Biophys Res Commun. 2007; 362:1007–12.


40. Alanazi AS. Systematic review and meta-analysis of efficacy and safety of combinational therapy with metformin and dipeptidyl peptidase-4 inhibitors. Saudi Pharm J. 2015; 23:603–13.


41. Wu D, Li L, Liu C. Efficacy and safety of dipeptidyl peptidase-4 inhibitors and metformin as initial combination therapy and as monotherapy in patients with type 2 diabetes mellitus: a meta-analysis. Diabetes Obes Metab. 2014; 16:30–7.


42. Lyseng-Williamson KA. Glucagon-like peptide-1 receptor analogues in type 2 diabetes: their use and differential features. Clin Drug Investig. 2019; 39:805–19.


43. Salvo F, Moore N, Arnaud M, Robinson P, Raschi E, De Ponti F, et al. Addition of dipeptidyl peptidase-4 inhibitors to sulphonylureas and risk of hypoglycaemia: systematic review and meta-analysis. BMJ. 2016; 353:i2231.


44. Yabe D, Seino Y. Dipeptidyl peptidase-4 inhibitors and sulfonylureas for type 2 diabetes: friend or foe? J Diabetes Investig. 2014; 5:475–7.


45. Zhang CL, Katoh M, Shibasaki T, Minami K, Sunaga Y, Takahashi H, et al. The cAMP sensor Epac2 is a direct target of antidiabetic sulfonylurea drugs. Science. 2009; 325:607–10.


Fig. 1.
In vivo assessment of β-cell glucose sensitivity using a graded insulin infusion protocol. (A) Estimation of β-cell glucose sensitivity by calculating the slope of the insulin secretion rate versus the blood glucose level. (B) Results showing that a single injection of liraglutide restored β-cell glucose sensitivity in patients with type 2 diabetes. Open triangles represent healthy control subjects. Open and closed rectangles represent type 2 diabetes subjects who received placebo or liraglutide, respectively. Adapted from Chang et al. [8], with permission from the American Diabetes Association. ISR, insulin secretion rate.
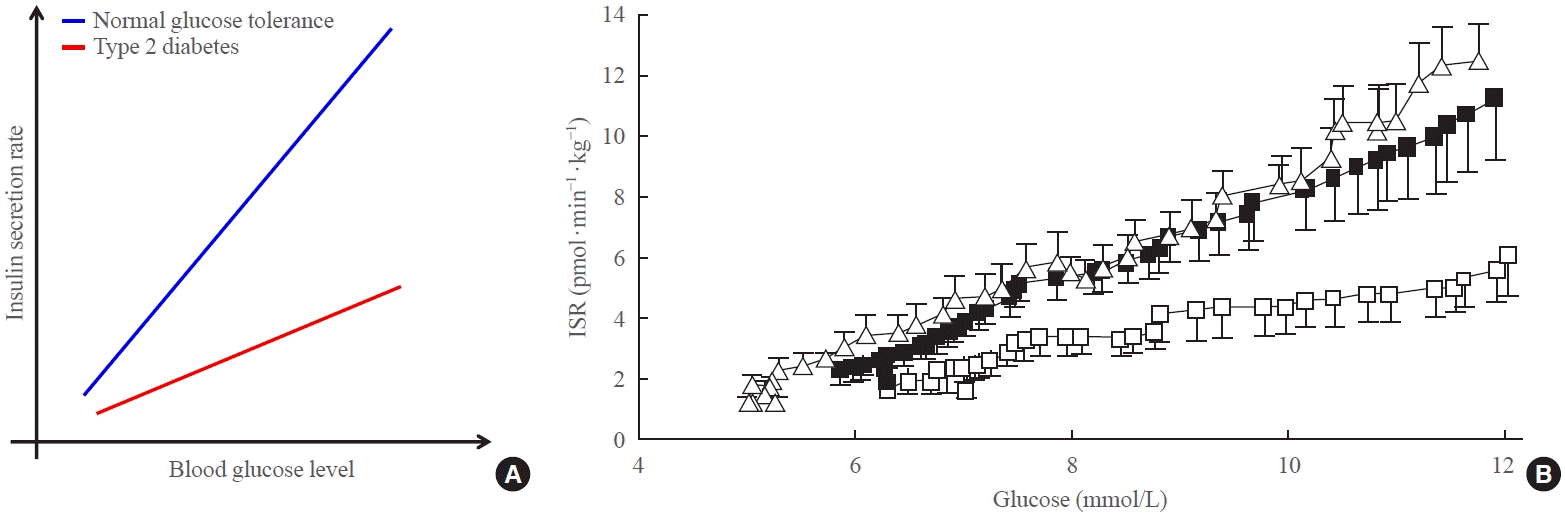
Fig. 2.
In vivo assessment of β-cell incretin sensitivity using a hyperglycemic clamp with glucagon-like peptide-1 (GLP-1) and glucose-dependent insulinotropic polypeptide (GIP) infusion. (A) C-peptide responses to hyperglycemia, GLP-1 infusion, and GIP infusion in subjects with normal glucose tolerance and type 2 diabetes. (B) C-peptide response to hyperglycemia, GLP-1 infusion, and GIP infusion in subjects with type 2 diabetes before and after dapagliflozin treatment (magnified from Fig. 2A). Adapted from Ahn et al. NGT, normal glucose tolerance. aP<0.05 for comparison between NGT and predapagliflozin studies; bP<0.05 for comparison between NGT and both pre- and postdapagliflozin studies.
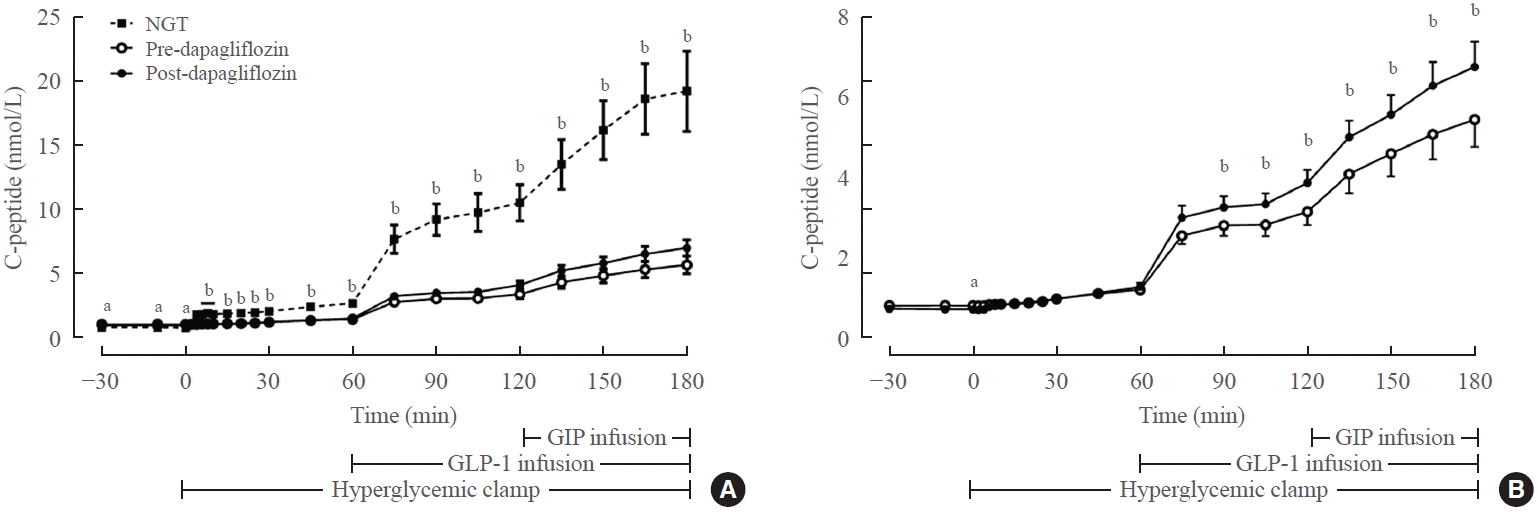
Fig. 3.
A proposed mechanism explaining how anti-diabetic medications improve β-cell incretin sensitivity. Anti-diabetic medications including insulin, sulfonylurea, metformin, dipeptidyl peptidase-4 (DPP-4) inhibitor and sodium-glucose co-transporter 2 (SGLT2) inhibitor restores pancreatic β-cell glucose and incretin sensitivity by ameliorating hyperglycemia. GIPR, glucose-dependent insulinotropic polypeptide receptor; GLP-1R, glucagon-like peptide-1 receptor; GIP, glucose-dependent insulinotropic polypeptide; GLP-1, glucagon-like peptide-1.
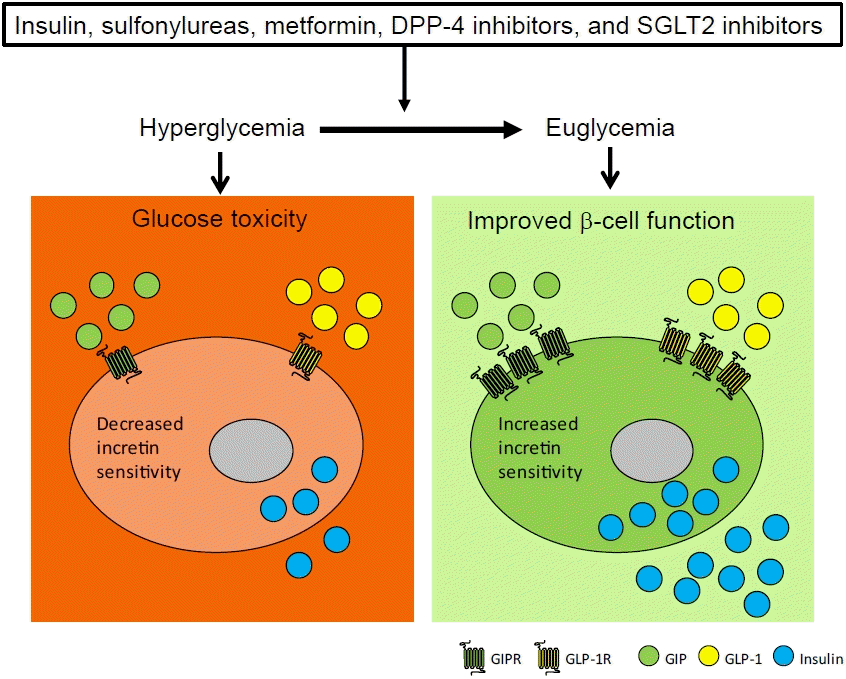
Table 1.
Effects of Pharmacotherapies on the β-Cell Incretin Sensitivity
Design | Intervention | Methods of measurement | No. | HbA1c | Duration of diabetes | Main results (control vs. intervention, if indicated) | |
---|---|---|---|---|---|---|---|
Insulin [10] | Single arm, non-controlled | Intensive insulin therapy, 4 wk | Hyperglycemic clamp (glucose level at 15 mM) with saline, GLP-1, or GIP infusion | 8 | 8.60% | 4.5 yr | Late-phase insulin response (nmol/L×min) |
Saline: 2.7±0.7 vs. 4.9±3.4 (P<0.05) | |||||||
GLP-1: 6.0±2.3 vs. 20.4±12.5 (P<0.05) | |||||||
GIP: 2.7±0.9 vs. 15.2±4.9 (P<0.05) | |||||||
Sulfonylurea [9] | Single arm, non-controlled | Glyburide, 1 mo | Hyperglycemic clamp (glucose level at 5.4 mM above fasting) with GIP infusion | 5 | NA | <3 mo | Insulin during hyperglycemic clamp: 99±29 vs. 156±41 pM (P<0.05) |
Insulin during GIP infusion: 123±37 vs. 283±80 pM (P<0.05) | |||||||
Metformin [36] | Animal study | Metformin, 4 wk | GLP-1 receptor expression in the pancreatic β-cells of Goto-Kakizaki rats | - | - | - | Expression level: 26.1%±2.1% vs. 45.4%±3.3%, P<0.001 |
DPP-4 inhibitor [37] | Randomized, double-blind, placebo-controlled | Sitagliptin, 12 wk | Hyperglycemic clamp (glucose level at 15 mM) with saline, GLP-1, or GIP infusion | 25 | 7.80% | 5.0 yr | AUC of insulin (pmol/L×120 min) |
Saline: 15.4±3.0 vs. 21.2±3.0 (nonsignificant) | |||||||
GIP: 20.7±3.8 vs. 31.2±6.4 (P<0.05) | |||||||
GLP-1: 52.5±11.1 vs. 95.3±27.0 (nonsignificant) | |||||||
Phlorizin [38] | Animal study | Phlorizin, 4 wk | GLP-1 and GIP receptor expression in the pancreatic β-cells of pancreatectomized hyperglycemic rats and Zucker diabetic fatty rats | - | - | - | Phlorizin treatment restored GLP-1 and GIP receptor expression in pancreatectomized hyperglycemic rat |
Phlorizin treatment restored GIP receptor expression in Zucker diabetic fatty rat | |||||||
SGLT2 inhibitor [11] | Single arm, non-controlled | Dapagliflozin, 8 wk | Hyperglycemic clamp (glucose level at 15.5 mM) with sequential GLP-1 and GIP infusion | 19 | 7.80% | 10.2 yr | C-peptide response (nmol/L×min) |
C-peptide response to hyperglycemia: 10.9±6.5 vs. 16.6±9.2, P=0.009 | |||||||
C-peptide response to GLP-1: 83.6±42.1 vs. 106.6±45.7, P=0.011 | |||||||
C-peptide response to GIP: 82.5±58.4 vs. 101.9±50.3, P=0.087 |