Abstract
Objective
This study aimed to evaluate the shear bond strength (SBS) of orthodontic brackets bonded to three-dimensionally (3D)-printed materials after various surface treatments and artificial aging compared with that bonded to computer-aided design/computer-aided manufacturing (CAD-CAM) polymethyl methacrylate (PMMA)-milled materials.
Methods
Eighty cylindrical specimens were 3D printed and divided into the following four subgroups (n = 20 each) according to the surface treatment and artificial aging procedure. Group A, sandblasted with 50 µm aluminum oxide particles (SA) and aging; group B, sandblasted with 30 µm silica-coated alumina particles (CO) and aging; group C, SA without aging; and group D, CO without aging. For the control group, 20 CAD-CAM PMMA-milled cylindrical specimens were sandblasted with SA and aged. The SBS was measured using a universal testing machine (0.25 mm/min), examined at ×2.5 magnification for failure mode classification, and statistically analyzed (p = 0.05).
Results
The retention obtained with the 3D-printed materials (groups A–D) was higher than that obtained with the PMMA-milled materials (control group). However, no significant difference was found between the study and control groups, except for group C (SA without aging), which showed significantly higher retention than the control group (PMMA-SA and thermocycling) (p = 0.037). Study groups A–D predominantly exhibited a cohesive specimen mode, indicating specimen fracture.
Adults comprise approximately 20% of the average orthodontist’s patient load, and their main motivation is to improve esthetic appearance and function.1 Orthodontic treatment is a part of a multidisciplinary treatment approach; teeth often have temporary crowns to help maintain proper function and esthetics. In such cases, definitive restorations prior to orthodontic treatment are not recommended because of changes in occlusal relationships and the risk of jeopardizing the surface.
Although provisional crowns are a better option than definitive restorations, clinicians are often confronted with the need to bond orthodontic brackets to them. Placing metal bands on teeth is also an option, but this is much less esthetically acceptable compared with conventional brackets, particularly on the anterior teeth. Bands are also less hygienic and accumulate more plaque, making them a less attractive alternative.2
Polymethyl methacrylate (PMMA) is the most popular choice among various materials available used to fabricate temporary crowns. PMMA-milled crowns are highly cross-linked, prefabricated PMMA resin blocks used in the computer-aided design/computer-aided manufacturing (CAD-CAM) technique. They have improved mechanical and esthetic properties, making them more suitable for long-term clinical applications.3
Three-dimensional (3D) printing has become a common trend in dentistry and is a manufacturing approach that creates objects one layer at a time. The CAD-CAM technology enables for easier recreation of any anatomical shape, which can be challenging in conventional methods. Moreover, it is more accurate and less time-consuming than milling. 3D printing allows clinicians to use several materials when fabricating crowns.4,5 3D printable composites are used to fabricate temporary crowns that have elastic moduli comparable with those of conventional crowns and bridge jet acrylic, but have lower peak stress than jet acrylic self-cured resin.5,6 A composite filling can be repaired using low-pressure depositing systems. Hannig et al.7 showed the best bond strength results using sandblasting with the Co-Jet system.
Orthodontic treatment requires a strong connection between the brackets and teeth or crowns. A weak bond leads to a high failure rate, with adverse consequences on the cost and efficiency of the treatment, which is likely to affect the patients’ overall comfort and satisfaction. Surface preparation techniques, such as etching, sandblasting, and roughening with a diamond bur, are used to increase the bond strength between a bracket and the crown’s surface.8 Newman et al.9 investigated the combinations of different types of bonding systems, orthodontic brackets, and various methods of surface treatment. However, the shear bond strength (SBS) of orthodontic brackets bonded to 3D-printed crowns after various surface treatments and artificial aging has not yet been investigated. Thus, this study aimed to evaluate the SBS of orthodontic brackets bonded to 3D-printed provisional crowns using composite cement after various surface treatments and artificial aging compared with that bonded to CAD-CAM PMMA-milled provisional crowns. We hypothesized no significant differences in SBS between the two provisional crowns or between the various surface treatments.
In the control group, 20 PMMA (Ceramill A-Temp; Amann Girrbach, Koblach, Austria) cylindrical (10 × 8 mm) specimens were milled in a CAD-CAM milling machine (Motion 2; Amann Girrbach). In the study group, 80 cylindrical (10 × 8 mm) specimens were made of a commercial 3D printing material (C&B MFH; NextDent, Soesterberg, Netherlands) by using CAD software (Exocad Plodiv v2.4; Exocad, Darmstadt, Germany) and a 3D printer (NextDent 5100; NextDent). Specimens were printed at a 0-degree angle, with a printing layer thickness of 50 µm, where supports were placed on the opposite side of the testing surface. For all printed specimens, supports were set to a density of 1 mm and a point size of 500 µm. The specimens in the control and study groups were visually examined for cracks and irregularities. Four specimens from the study group were reprinted.
All the specimens were cleaned in an ultrasonic bath (SD-120H; Mujigae Co., Seoul, Korea) containing 96% ethanol for 3 minutes, soaked in clean 96% ethanol for 2 minutes, and then dried. Subsequently, the samples were light cured for 30 minutes at 60°C (Form Cure; Formlabs Inc., Somerville, MA, USA) according to the manufacturer’s protocol. The chemical compositions of the materials are shown in Table 1.
The specimens were sandblasted with 50 µm aluminum oxide (SA, Zest Dental Solutions; Carlsbad, CA, USA). Phosphoric acid (37%) was applied to each specimen for 20 seconds to clean the sample surfaces, including possible oil or debris from the CAM and cutting methods. The specimens were then thoroughly washed with water for 10 seconds and dried. TransbondTM XT Primer (3M Unitek, Monrovia, CA, USA) was applied to the dried surface and light cured for 20 seconds. Subsequently, stainless steel (0.022-inch Victory Series; 3M Unitek) central incisor brackets were bonded to each specimen with light-cure bis-acrylic resin composite adhesive cement (Transbond XT; 3M Unitek).
Each bracket was positioned at the center of the disc using a bracket positioning gauge (3M Unitek), and all excess composite material was removed. The specimens were light cured using an LED curing lamp (Deep Cure-S; 3M, St. Paul, MN, USA) for 20 seconds. All the procedures were performed by the same physician. After bonding, the specimens were stored at 37°C under 100% humidity for 1 month, followed by 1,000 cycles of thermocycling between 5°C and 55°C with a 30-second dwell time.10,11
The specimens were randomly assigned to the following groups (2 × 40), according to the surface treatment methods before bonding the brackets: SA or sandblasting with 30 µm silica-coated alumina particles (CO, CoJet; 3M ESPE, Seefeld, Germany). After surface treatment with CO, a silane coupling agent (Ultradent silane; Ultradent, South Jordan, UT, USA) was applied with a brush, left undisturbed for 60 seconds, and then air dried. Sandblasting of all the specimens was performed by a single operator for 10 seconds at 1 bar and a distance of 10 mm. The brackets were then bonded as described in the control group. After bonding, all specimens were stored at 37°C under 100% humidity for 1 month. Subsequently, the specimens from each surface treatment group were divided into two subgroups (2 × 20) based on whether they were subjected to aging conditions (thermocycling for 1,000 cycles between 5°C and 55°C, with a 30-second dwell time). Therefore, the following four study groups were evaluated: group A, SA with aging; group B, CO with aging; group C, SA without aging; and group D, CO without aging (Table 1). The experimental design is illustrated in Figure 1.
All bracket-crown assemblies were subjected to dislodgment forces using a universal testing machine (Instron, Norwood, MA, USA). Each specimen was placed in a custom-made device, with an attached knife-edge ram (Figure 2).
The crosshead speed was set at 0.025 mm/min until failure, and the values were recorded in Newtons. The base surface area of the brackets was calculated by measuring the length and width using a digital caliper (Digimatic; Mitutoyo Co., Tokyo, Japan), and the bracket base area was 11.9 mm2.12,13 The SBS (MPa) was calculated by dividing the force (N) at dislodgment by the total surface area of each prepared sample.
The surfaces of the de-bonded specimens were examined at ×2.5 magnification to determine the type of failure (Table 2). A scanning electron microscope was used to inspect the surface samples of the study group before and after treatment.
The one-sample Kolmogorov–Smirnov test was performed to confirm whether the data followed a normal distribution. The SBS was evaluated using a two-way analysis of variance (ANOVA), with the dependent variable being SBS (MPa). Surface treatment (n = 2) and thermocycling (n = 2) were independent variables. One-way ANOVA was performed to analyze the differences between the study and control groups. The post hoc Dunnett’s t-test was used for multiple comparisons. Statistical significance was set at α = 0.05.
One-way ANOVA showed significant statistical differences between the control and study groups (p = 0.043), and a post hoc t-test was performed between each study group and the control group (Table 3). The retentive strengths (mean, standard deviation) of the various materials, surface treatments, and aging procedure combinations are presented in Table 3.
Box plots of the minimum, maximum, interquartile range, median, and outliers for each group are shown in Figure 3. Two-way ANOVA for the independent variables (thermocycling, type of sandblasting, and their combination) showed no effect on the SBS of the study groups (Table 4).
The retention obtained with the 3D-printed materials (groups A–D) was higher than that obtained with the PMMA (control group). However, no significant difference was found between the study and control groups, except for group C (SA without aging), which showed significantly higher retention than the control group (PMMA-SA with thermocycling) (p = 0.037).
Examination under magnification of the failure mode revealed complete adhesive cement–bracket failure for the control group (PMMA), indicating that the cement was mainly on the specimen surface (classification 2). Scanning electron microscopy (SEM) images of the specimen surface in the control group prior to surface treatment are shown in Figures 4A and 4B.
In the 3D-printed study groups, SEM images of the specimen surface prior to surface treatment are shown in Figures 4C and 4D, and images of SA and CO surface treatments prior to bracket bonding are shown in Figure 5.
Bracket bonding to provisional crowns must support orthodontic and masticatory forces during chewing cycles. The adhesive resistance of brackets bonded to temporary materials must be sufficiently strong to resist dental movement without debonding the orthodontic accessory. However, it should be weak enough to be removed without damaging the surface after completion of orthodontic treatment.
In this study, the SBS of orthodontic brackets bonded to 3D-printed materials using composite cement after various surface treatments and artificial aging was compared with that bonded to CAD-CAM PMMA-milled materials. Our null hypothesis was partially rejected, as no significant difference in SBS was found between the study groups and between the study and control groups, except for group C (SA without aging). Group C showed significantly higher SBS than the control group (p = 0.037).
Dentists depend on several surface treatments to improve the bond strength. Improving the bond strength between two materials involves increasing the surface roughness to enhance mechanical retention, followed by applying a bonding agent to promote better chemical bonding. Surface roughening using aluminum oxide minimizes bracket bonding failure by increasing the surface area and retentiveness. Sandblasting is a surface treatment that causes micro-retentive features.14
SA and CO were surface treatments performed in this study. Sandblasting aimed to roughen the surface and create micropores to enhance bonding. However, a study on different composite materials with various surface treatments found that surface treatment with 30 µm alumina particles coated with silica improves bond strength.15 This finding is attributed to the additional chemical effect contributed by silica particles, emphasizing that silica-coated alumina pretreatment is not only a mechanical but also a chemical roughening.
This study evaluated the differences in SBS between surfaces treated with SA and those with CO, but revealed no significant difference between them. This result is not in agreement with other studies that found that sandblasting treatment with 50 µm aluminum oxide particles exerts a beneficial effect on SBS.16 Cardoso et al.17 concluded that sandblasting with aluminum oxide is the most effective mechanical treatment to increase the bond strength of brackets to PMMA material. This may be explained by the occurrence of cohesive failure in all specimens in the study groups, without the expression of differences between the two types of surface treatment.
Subgroup C (3D printed: SA without aging) exhibited a significantly higher bonding strength than the control group (PMMA: SA and thermocylcing) (p = 0.037). However, this difference may be related to the type of material and not only to the effect of the thermocycling procedure. Moreover, thermocycling was not a significant factor in the study groups.
Given that orthodontic adhesives are routinely subjected to thermal changes in the oral cavity, determining whether such temperature variations introduce stresses in the adhesive that might affect bond strength is important. Therefore, thermocycling is important for simulating the conditions of temperature changes and a moist environment in a patient’s mouth. These changes weaken temporary materials to different degrees and affect some materials more than others.18
In our study, the storage duration was set to 1 day. The storage duration varies in different studies, ranging from 30 minutes to 12 months. However, previous studies reported no significant difference in bond strength between 1- and 30-day storage duration.19
For this reason, the specimens in the current study were underwent thermocycling to induce a mouth-like environment when measuring bond strength. We found no significant effect of thermocycling on SBS, although the PMMA control group (SA and thermocycling) showed the lowest SBS, which is in agreement with the results of Al Jabbari et al.20 The results may be due to the fact that PMMA materials are more prone to water softening during artificial aging. Chay et al.21 tested the SBS of brackets bonded to PMMA restorations and found a significant difference in bond strength between treatment groups of specimens aged for 1 week and those aged for 1 month. They concluded that aging influences bond strength, as specimens aged for 1 month have a lower bond strength than those aged for 1 week.
The crosshead speed was set at 0.025 mm/min, and different studies have used different crosshead speeds, varying from 0.25 to 2 mm/min. However, according to Klocke and Khal-Nieke,22 the crosshead speed does not affect the debonding value but only affects the time until debonding occurs. The SBS values reported in our study are eight times higher than those reported for polycarbonate provisional crowns.23 This suggests that using PMMA-milled or 3D-printed provisional crowns for combined orthodontic–prosthodontic treatment may allow better predictability.
The 3D printing material used in this study was classified as a microfilled hybrid material. Previous studies have shown that conventional resin should be the material of choice for repairing a 3D-printed restoration.24 This may be the reason for the higher bonding strength we found between the bracket and 3D-printed specimen, which may have caused cohesive failure owing to the lower mechanical properties of the material. However, PMMA has a higher flexural strength, which may indicate that the material itself held up well to the pressure applied and may explain the more frequent adhesive failures observed. The 3D-printed specimens had more cohesive failures, and the material broke. This indicates that the 3D-printed material itself is weak and not up to the masticatory forces in the mouth. Albahri et al.24 achieved similar conclusions. They compared the SBS of 3D-printed materials with PMMA and bis-acrylic composite resin and bis-GMA composite, and found that all failures in the 3D-printed materials were due to poor cohesion, which is in agreement with our findings.
This study had several limitations that should be considered when interpreting the results. The flat surface of the tested specimen did not resemble the normally contoured tooth-bonded surface. The study settings, particularly the SBS test, did not reflect the clinical setting for removing orthodontic brackets, which might have affected the failure mode. In addition, the oral cavity presents a different testing environment regarding temperature changes, saliva, and pH levels, which might have also affected the outcomes.
Within the limitations of this in vitro study, we can conclude that orthodontic brackets bonded to 3D-printed materials exhibit adequate bonding strength compared with those bonded to PMMA-milled provisional materials. However, 3D-printed materials are more prone to cohesive failure, which may result in material fracture.
Notes
REFERENCES
1. Pabari S, Moles DR, Cunningham SJ. 2011; Assessment of motivation and psychological characteristics of adult orthodontic patients. Am J Orthod Dentofacial Orthop. 140:e263–72. DOI: 10.1016/j.ajodo.2011.06.022. PMID: 22133960.


2. Huser MC, Baehni PC, Lang R. 1990; Effects of orthodontic bands on microbiologic and clinical parameters. Am J Orthod Dentofacial Orthop. 97:213–8. DOI: 10.1016/S0889-5406(05)80054-X. PMID: 2309668.


3. Goracci C, Özcan M, Franchi L, Di Bello G, Louca C, Vichi A. 2019; Bracket bonding to polymethylmethacrylate-based materials for computer-aided design/manufacture of temporary restorations: influence of mechanical treatment and chemical treatment with universal adhesives. Korean J Orthod. 49:404–12. DOI: 10.4041/kjod.2019.49.6.404. PMID: 31815108. PMCID: PMC6883210.


4. Katreva I, Dikova T, Tonchev T. 2018; 3D Printing- an alternative of conventional crown fabrication: a case report. J IMAB. 24:2048–54. DOI: 10.5272/jimab.2018242.2048.
5. Tahayeri A, Morgan M, Fugolin AP, Bompolaki D, Athirasala A, Pfeifer CS, et al. 2018; 3D printed versus conventionally cured provisional crown and bridge dental materials. Dent Mater. 34:192–200. DOI: 10.1016/j.dental.2017.10.003. PMID: 29110921. PMCID: PMC5801146.


6. Scotti CK, Velo MMAC, Rizzante FAP, Nascimento TRL, Mondelli RFL, Bombonatti JFS. 2020; Physical and surface properties of a 3D-printed composite resin for a digital workflow. J Prosthet Dent. 124:614.e1–614.e5. DOI: 10.1016/j.prosdent.2020.03.029. PMID: 32636072.


7. Hannig C, Laubach S, Hahn P, Attin T. 2006; Shear bond strength of repaired adhesive filling materials using different repair procedures. J Adhes Dent. 8:35–40. PMID: 16536343.
8. Major PW, Koehler JR, Manning KE. 1995; 24-hour shear bond strength of metal orthodontic brackets bonded to porcelain using various adhesion promoters. Am J Orthod Dentofacial Orthop. 108:322–9. DOI: 10.1016/S0889-5406(95)70028-5. PMID: 7661151.


9. Newman GV, Newman RA, Sun BI, Ha JL, Ozsoylu SA. 1995; Adhesion promoters, their effect on the bond strength of metal brackets. Am J Orthod Dentofacial Orthop. 108:237–41. DOI: 10.1016/S0889-5406(95)70015-3. PMID: 7661138.


10. Gale MS, Darvell BW. 1999; Thermal cycling procedures for laboratory testing of dental restorations. J Dent. 27:89–99. DOI: 10.1016/S0300-5712(98)00037-2. PMID: 10071465.


11. Heller H, Arieli A, Beitlitum I, Pilo R, Levartovsky S. 2019; Load-bearing capacity of zirconia crowns screwed to multi-unit abutments with and without a titanium base: an in vitro pilot study. Materials (Basel). 12:3056. DOI: 10.3390/ma12193056. PMID: 31547045. PMCID: PMC6803877.


12. Elsaka SE. 2016; Influence of surface treatments on bond strength of metal and ceramic brackets to a novel CAD/CAM hybrid ceramic material. Odontology. 104:68–76. DOI: 10.1007/s10266-014-0188-8. PMID: 25585677.


13. Cozza P, Martucci L, De Toffol L, Penco SI. 2006; Shear bond strength of metal brackets on enamel. Angle Orthod. 76:851–6. DOI: 10.1043/0003-3219(2006)076[0851:SBSOMB]2.0.CO;2. PMID: 17029521.
14. de Pulido LG, Powers JM. 1983; Bond strength of orthodontic direct-bonding cement-plastic bracket systems in vitro. Am J Orthod. 83:124–30. DOI: 10.1016/S0002-9416(83)90297-X. PMID: 6337499.


15. Özcan M, Pekkan G. 2013; Effect of different adhesion strategies on bond strength of resin composite to composite-dentin complex. Oper Dent. 38:63–72. DOI: 10.2341/11-482-L. PMID: 22812912.


16. Brosh T, Pilo R, Bichacho N, Blutstein R. 1997; Effect of combinations of surface treatments and bonding agents on the bond strength of repaired composites. J Prosthet Dent. 77:122–6. DOI: 10.1016/S0022-3913(97)70224-5. PMID: 9051597.


17. Cardoso RM, Godinho J, Jardim L. 2021; Bond strength of orthodontic brackets to polymethylmethacrylate: effect of the surface treatment and adhesive system. Rev Port Estomatol Med Dent Cir Maxilofac. 62:16–22. DOI: 10.24873/j.rpemd.2021.01.818.


18. Fox NA, McCabe JF, Buckley JG. 1994; A critique of bond strength testing in orthodontics. Br J Orthod. 21:33–43. DOI: 10.1179/bjo.21.1.33. PMID: 8199163.


19. Oesterle LJ, Shellhart WC. 2008; Effect of aging on the shear bond strength of orthodontic brackets. Am J Orthod Dentofacial Orthop. 133:716–20. DOI: 10.1016/j.ajodo.2006.04.042. PMID: 18456145.


20. Al Jabbari YS, Al Taweel SM, Al Rifaiy M, Alqahtani MQ, Koutsoukis T, Zinelis S. 2014; Effects of surface treatment and artificial aging on the shear bond strength of orthodontic brackets bonded to four different provisional restorations. Angle Orthod. 84:649–55. DOI: 10.2319/090313-649.1. PMID: 24446920. PMCID: PMC8650449.


21. Chay SH, Wong SL, Mohamed N, Chia A, Yap AU. 2007; Effects of surface treatment and aging on the bond strength of orthodontic brackets to provisional materials. Am J Orthod Dentofacial Orthop. 132:577.e7–11. DOI: 10.1016/j.ajodo.2004.01.024. PMID: 18005828.


22. Klocke A, Kahl-Nieke B. 2005; Influence of cross-head speed in orthodontic bond strength testing. Dent Mater. 21:139–44. DOI: 10.1016/j.dental.2004.03.004. PMID: 15681012.


23. Blakey R, Mah J. 2010; Effects of surface conditioning on the shear bond strength of orthodontic brackets bonded to temporary polycarbonate crowns. Am J Orthod Dentofacial Orthop. 138:72–8. DOI: 10.1016/j.ajodo.2008.08.030. PMID: 20620836.


24. Albahri R, Yoon HI, Lee JD, Yoon S, Lee SJ. 2021; Shear bond strength of provisional repair materials bonded to 3D printed resin. J Dent Sci. 16:261–7. DOI: 10.1016/j.jds.2020.05.003. PMID: 33384807. PMCID: PMC7770250.


Figure 1
Experiment design.
Group A, sandblasted with 50 µm aluminum oxide particles (SA) and aging; Group B, sandblasted with 30 µm silica-coated alumina particles (CO) and aging; Group C, SA without aging; Group D, CO without aging; PMMA, polymethyl methacrylate; 3D, three-dimensional.
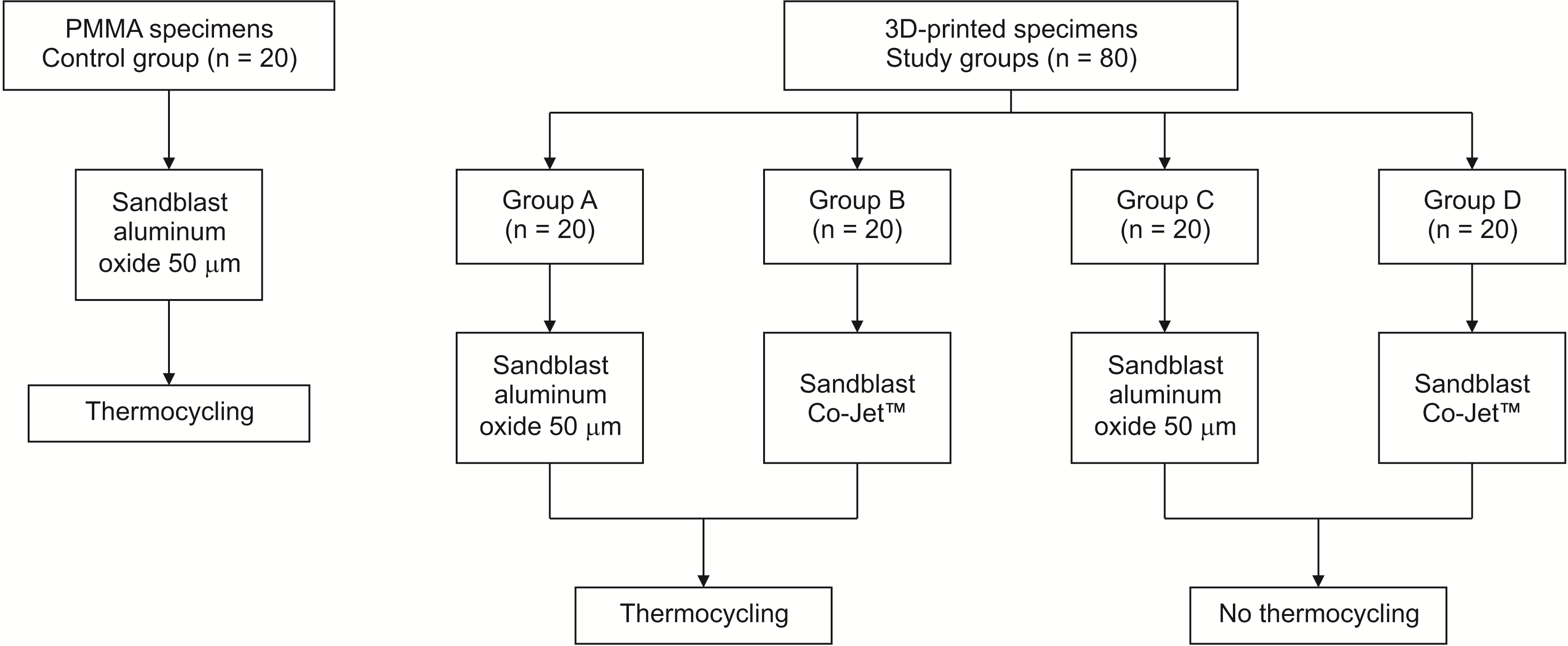
Figure 3
Minimum, maximum, interquartile range, median, and outliers of measurements for the control and study groups.
Group A, sandblasted with 50 µm aluminum oxide particles (SA) and aging; Group B, sandblasted with 30 µm silica-coated alumina particles (CO) and aging; Group C, SA without aging; Group D, CO without aging.
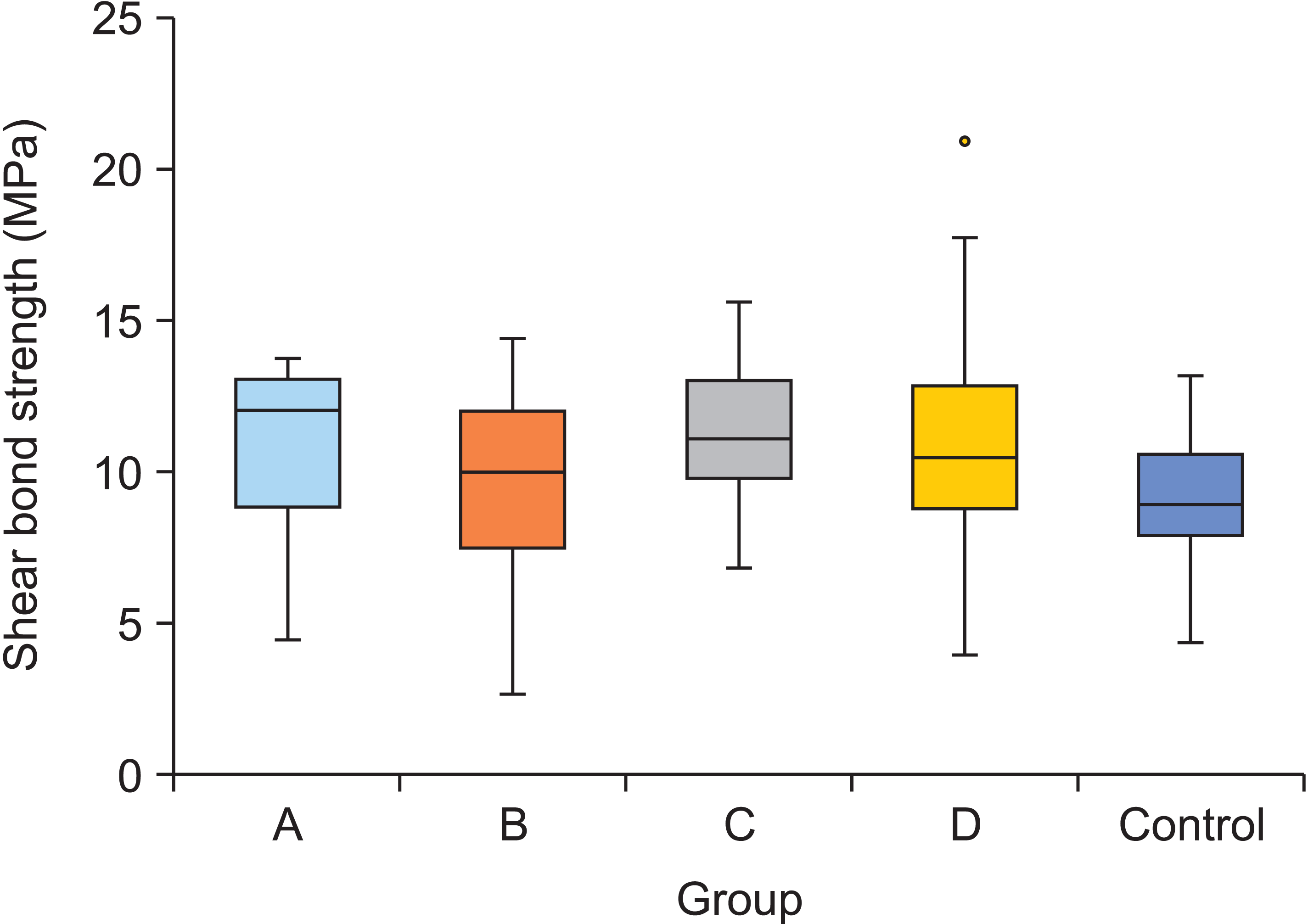
Figure 4
Scanning electron images of specimen surfaces prior to surface treatment. Control group: surface scanning at (A) ×200 magnification and (B) ×1,500 magnification. Three-dimensional printed groups: surface scanning at (C) ×200 magnification and (D) ×1,500 magnification. Several irregularities and porosities can be seen at the specimen surface at ×1,500 magnification.
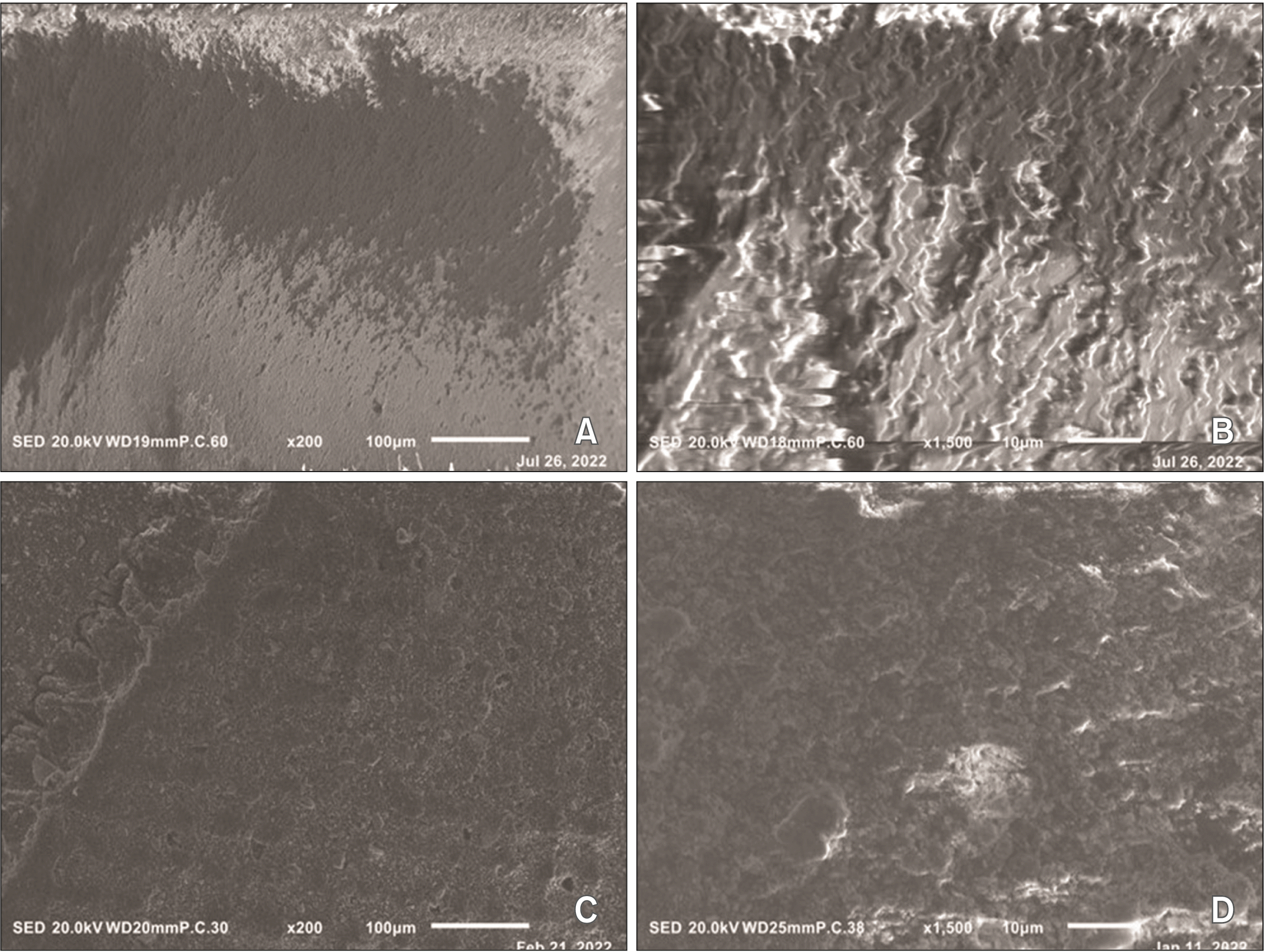
Figure 5
Scanning electron images of specimen surfaces prior to bracket bonding in the 3D-printed groups: surface after treatment with (A, B) 50 µm aluminum oxide particles (A, ×200 and B, ×1,500) and (C, D) 30 µm silica-coated-aluminum particles (C, ×200 and D, ×1,500). Specimen surfaces after treatment showed more irregularities and porosities. Surface after treatment with 50 µm aluminum oxide particles was more pronounced than that after treatment with 30 µm silica-coated aluminum particles.
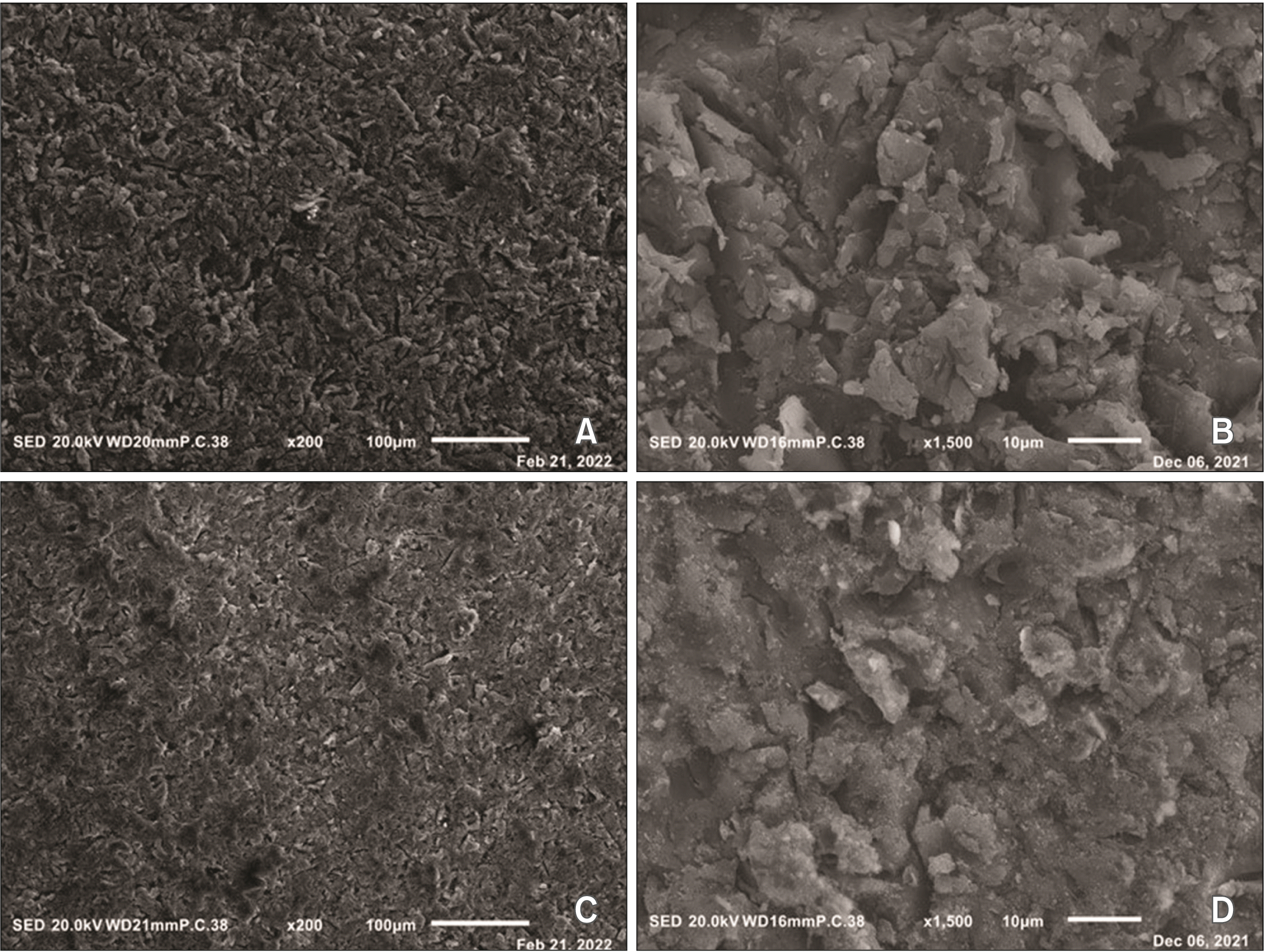
Figure 6
Scanning electron image of a fractured specimen in the study group, cohesive type (A, ×200 and B, ×1,500).
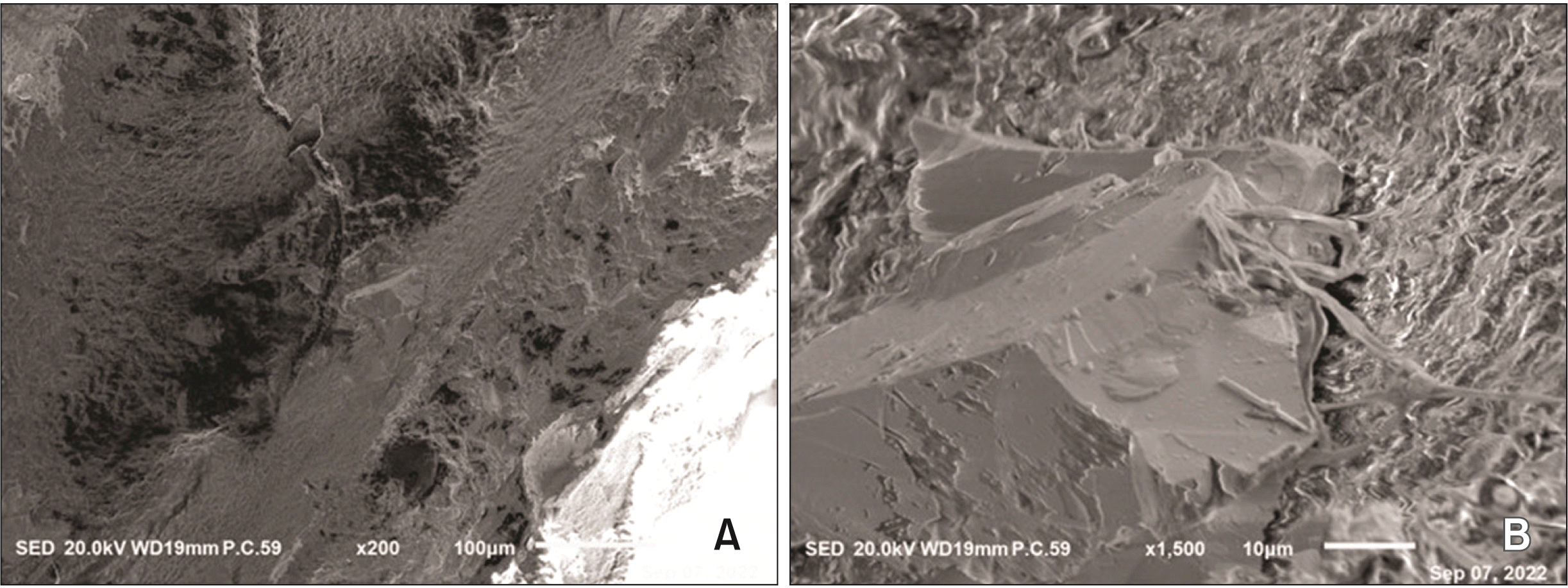
Table 1
Control and study groups
Table 2
Classification of failures
Table 3
Mean and standard deviation of retentive strength (MPa) of various materials in the control and study groups
Group |
Mean retentive value (MPa) |
Standard deviation | p-value* |
---|---|---|---|
Control | 9.11 | 2.21 | |
Study | |||
A | 10.95 | 2.42 | 0.136 |
B | 9.75 | 3.06 | 0.887 |
C | 11.46 | 2.21 | 0.037 |
D | 11.24 | 3.68 | 0.067 |