Abstract
Objective
We aimed to evaluate the cell viability and antimicrobial effects of orthodontic bands coated with silver or zinc oxide nanoparticles (nano-Ag and nano-ZnO, respectively).
Methods
In this experimental study, 30 orthodontic bands were divided into three groups (n = 10 each) control (uncoated band), Ag (silver-coated band), and ZnO (zinc oxide-coated band). The electrostatic spray-assisted vapor deposition method was used to coat orthodontic bands with nano-Ag or nano-ZnO. The biofilm inhibition test was used to assess the antimicrobial effectiveness of nano-Ag and nano-ZnO against Streptococcus mutans, Lactobacillus acidophilus, and Candida albicans. Biocompatibility tests were conducted using the 3-(4, 5-dimethylthiazol-2-yl)-2, 5-diphenyltetrazolium bromide assay. The groups were compared using one-way analysis of variance with a post-hoc test.
Results
The Ag group showed a significantly higher reduction in the number of L. acidophilus, C. albicans, and S. mutans colonies than the ZnO group (p = 0.015, 0.003, and 0.005, respectively). Compared with the control group, the Ag group showed a 2-log10 reduction in all the microorganisms' replication ability, but only S. mutants showed a 2-log10 reduction in replication ability in the ZnO group. The lowest mean cell viability was observed in the Ag group, but the difference between the groups was insignificant (p > 0.05).
Oral hygiene is greatly complicated following fixed orthodontic appliance placement.1 In many patients receiving fixed orthodontic therapy, molar bands are used throughout the treatment period, which normally lasts 1.5 to 2 years.2
The oral microbiological ecosystem may be altered by the insertion of bands through an increase in the number of cariogenic microorganisms (Lactobacillus acidophilus and Streptococcus mutans), accumulation of plaque, improvement of Candida albicans colonization, and reduced plaque pH.3 Clinically, such ecological alterations cause an increased occurrence of oral soft tissue disease and white spot lesions.4 White spots can form around orthodontic attachments as early as within a month of treatment initiation.5
Routinely used measures for dental biofilm removal include mechanical biofilm removal, tooth brushing, and antimicrobial or antiplaque agent use.6 However, these approaches may not entirely remove microorganisms, and biofilm formation may not be prevented. The persistence of drug-resistant microorganisms might disrupt the natural bacterial flora.7
White spot lesions during orthodontic treatment have a documented etiology. Briefly, the accumulation of plaque and food around attachments such as brackets, bands, and wires decreases the pH and increases S. mutans colonization, potentially leading to clinical demineralization.8-10
Therefore, methods with minimum side effects and maximum benefits are preferred in clinical practice. These methods include treating the surface of the metal appliance, such as coating it with nanoparticles, and they have been used to decrease or prevent bacterial aggregation around the teeth.11 Because of their small size, high surface-to-volume ratio, and a large amount of contact with the external environment, metal nanoparticles have many antimicrobial properties.12
Silver (Ag) nanoparticles (nano-Ag) have been reported to show greater antimicrobial activity than other metal nanoparticles.13 Studies have evaluated the cytotoxicity of nano-Ag against fungi, protozoa, and gram-negative and gram-positive bacteria such as S. mutans and L. acidophilus. As nano-Ag have been confirmed to possess antimicrobial properties, especially against S. mutans, they have been used as an antimicrobial additive in dental materials.14,15
Zinc oxide (ZnO) has significant antimicrobial properties against gram-positive and gram-negative bacteria and is an essential mineral for humans. ZnO nanoparticles (nano-ZnO) have been found to have antimicrobial properties, to be safe for humans, and to be non-polluting to the environment and are therefore used as an antimicrobial agent.16
Although coating orthodontic bands with nano-ZnO and nano-Ag does not reduce the number of bacteria in the oral cavity, it may reduce the colonization and plaque formation on the bands. Since the bands are in contact with the oral mucosa and fluids for a long time, they must be biocompatible.
The antimicrobial properties and biocompatibility of orthodontic brackets and wires coated with nano-Ag or nano-ZnO have been studied.17 However, studies on orthodontic bands and comparisons between the two nanoparticles are lacking.
This study aimed to evaluate and compare the cell viability and antimicrobial effects of orthodontic bands coated with nano-Ag and those coated with nano-ZnO because no study has simultaneously assessed the antimicrobial properties and biocompatibility of nano-Ag and nano-ZnO.
The nanoparticles were supplied by Pishgaman Iranian Nanomaterials Company (Mashhad, Iran). X-ray diffraction (TESCAN MIRA3; TESCAN, Paterson, Australia) was used to confirm the nature of the nanoparticles. Nanoparticle size and shape were confirmed using field emission scanning electron microscopy (FESEM, TESCAN MIRA3; accelerating voltage: 15 kV) and transmission electron microscopy (TESCAN MIRA3).
Of the 30 stainless steel orthodontic bands (American Orthodontics, Sheboygan, WI, USA) used in this study, 10 were not coated (control group), 10 were coated with nano-Ag (Ag group), and 10 were coated with nano-ZnO (ZnO group). The electrostatic spray-assisted vapor deposition method was used to coat the bands with nano-Ag or nano-ZnO. The ZnO suspension was prepared by mixing 0.1 g of ZnO powder with 3 mL of acetone; the Ag suspension was prepared similarly. The suspension was pumped at a flow rate of 10 mL/hr using a syringe pump at a distance of 3 cm from the bands. An input voltage of 8 kV was applied at the nozzle tip and counter electrode. FESEM and energy-dispersive X-ray spectroscopy were used to confirm the coating of the nanoparticles on the surface of the bands.
To evaluate the adhesion of the coating to the surface of the band, routine oral hygiene procedures such as brushing were simulated. After the presence of the nanoparticles on the surface of the bands was confirmed using FESEM, the coated band was immersed in 2 mL of artificial saliva for 30 days. The bands were brushed with a soft toothbrush using distilled water for 2 minutes, twice daily, for 1 month. Subsequently, FESEM was used to re-examine the presence of nanoparticles on the surface of the bands.
The ATCC 35668, ATCC 314, and ATCC 14053 strains of S. mutans, L. acidophilus, and C. albicans, respectively, were obtained from the Pasteur Institute of Iran (Tehran, Iran). S. mutans and L. acidophilus were incubated in tryptic soy broth (Merck, Darmstadt, Germany) in the presence of 5% CO2, and C. albicans has incubated in brain heart infusion (BHI) broth (Merck) under aerobic conditions for 48 hours at 37°C.
We added the bands to tubes containing the microbial suspensions with a concentration of 0.5 McFarland (1.5 × 108 colony forming units [CFU]/mL for S. mutans and L. acidophilus; 1.5 × 105 CFU/mL for C. albicans) and incubated them at 37°C according to the growth conditions of each microorganism for microbial biofilm formation.
After 48 hours of incubation, the bands were washed under aseptic conditions in 1 mL of sterile normal saline to remove loosely bonded microorganisms and those in the planktonic phase. The bands were then placed in tubes containing 1 mL of sterile BHI broth and vortexed at high speed for 1 minute to separate the microbial biofilm from the surface of the bands. The obtained microbial suspensions were serially diluted, and 10-μL aliquots were inoculated into BHI agar (Merck). The plates containing S. mutants and L. acidophilus were incubated at 37°C in the presence of 5% CO2, and those containing C. albicans were aerobically incubated. After 24 hours, the CFU/mL of each sample was calculated.18 The experiment was repeated three times.
The MTT assay was performed as outlined in ISO 10993-5.19 Human gingival fibroblasts (HGF; IBRCC 10459) were seeded in 96-well plates using Dulbecco’s modification of Eagles culture medium (Idehzist, Tehran, Iran) containing 10% fetal bovine serum (Capricorn, Düsseldorf, Germany) and 1% penicillin/streptomycin (Biosera, Nuaille, France). The cell density was 10 × 106 cells/well. The cells were incubated for 24 hours at 37°C in a humidified atmosphere with 5% CO2.
To evaluate the indirect cytotoxicity of the coated bands, extracts were prepared by incubating the samples in a medium containing serum at an extraction ratio of 0.75 cm2/mL for 24 hours (Figure 1). The experiment was repeated three times.
After 24 hours of incubation, the eluents were removed from each well, and 40 µL of MTT solution (5 mg/mL MTT [Sigma, Merck, Germany] in phosphate saline) was added followed by re-incubation for 3–4 hours at 37°C and 5% CO2. Finally, the MTT solution was removed, 60 µL of dimethylsulfoxide solution was added to each well, and the absorbance was determined at 570 nm using Microplate Reader (BioTek, Santa Clara, CA, USA).
To evaluate the morphological changes in the HGF, we considered the morphology of the cells that were not in contact with the band as normal and compared it with the morphology of the cells in contact with the band.
The data were analyzed using SPSS version 29.0 (IBM Corp., Armonk, NY, USA). Descriptive statistics were used to describe the data and one-way analysis of variance, repeated-measures analysis of variance, and the post-hoc Games–Howell and Tukey tests were used to analyze the data. The level of significance was set at p < 0.05.
The morphological characteristics of the nano-Ag and nano-ZnO have been shown in Figures 2 and 3, respectively. As demonstrated in Figures 2A and 3A, the nanoparticles were predominantly spherical and uniform in shape, and the distribution of the nanoparticles was visually acceptable. FESEM revealed that the particles were smaller than 60 nm (Figures 2B and 3B); this was confirmed by the X-ray diffraction graphs (Figures 2C and 3C).
FESEM indicated the presence of nano-Ag and nano-ZnO on the respective band surfaces (Figures 4A and 5A). Energy-dispersive X-ray spectroscopy of the bands coated with nano-Ag and nano-ZnO showed the presence of Ag and Zn ions, respectively, in addition to the normal constituents of the band (Figures 4B and 5B). The estimated values by weight of Ag and Zn were 62.53% and 64.02%, respectively. Post-brushing FESEM confirmed the presence of the nanoparticles on the band surface.
As shown in Figure 6 and Table 1, the mean numbers of L. acidophilus colonies cultured from the nano-Ag–coated, nano-ZnO–coated, and uncoated bands were 4.3 ± 0.55 × 107 CFU/mL, 31.0 ± 4.35 × 107 CFU/mL, and 656.6 ± 61.1 × 107 CFU/mL, respectively. The mean numbers of C. albicans colonies cultured from the nano-Ag–coated, nano-ZnO–coated, and uncoated bands were 2.96 ± 0.71 × 104 CFU/mL, 111.0 ± 8.18 × 104 CFU/mL, and 460.0 ± 55.7 × 104 CFU/mL, respectively. The mean numbers of S. mutans colonies cultured from the nano-Ag–coated, nano-ZnO–coated, and uncoated bands were 2.6 ± 0.7 × 106 CFU/mL, 46.3 ± 4.51 × 106 CFU/mL, and 6,166.7 ± 1,106.04 × 106 CFU/mL, respectively (Table 1). The differences among all the groups were significant (p < 0.05).
Compared with the control group, the Ag and ZnO groups showed 3.4- and 2.14-log10 reductions in the number of S. mutans, 2.18- and 1.36-log10 reductions in the number of L. acidophilus, and 2.2- and 0.6-log10 reductions in the number of C. albicans, respectively.
Figure 7 shows the mean cell viability in each group. The lowest mean cell viability was observed in the Ag group (0.42 ± 0.02), followed by the ZnO group (0.45 ± 0.02) and the control group (0.48 ± 0.04), in that order. The relative percentages of viable cells in control, ZnO, and Ag groups were 98%, 91.8%, and 85.7%, respectively, in comparison with an untreated (100%) control. The mean cell viability of the Ag group was lower than that of the other two groups, but the differences among the groups were not significant (p > 0.05).
Oral hygiene maintenance has long been an important challenge in patients receiving fixed orthodontic treatment, leading clinicians to search for methods that are minimally dependent on patient cooperation. The use of fluoride-releasing materials is appropriate for caries-prone patients, but they are primarily used in dental offices and their use is limited.20
Studies have assessed the antimicrobial properties of nanoparticles.21-24 Adding nanoparticles to orthodontic adhesives can be problematic and can have a negative effect on their mechanical properties.25,26 The use of Ag ions containing nano-ZnO in orthodontic composites decreases the shear bond strength.27 Further, the discoloration of composite resins caused by nano-Ag (1 mM) is problematic for dental applications.28 Therefore, nanoparticles have only recently been added to metal orthodontic materials. However, various properties of nanoparticles (such as size, shape, consistency, and surfactant type) can affect their antimicrobial effect;29,30 therefore, their properties should be re-evaluated when they are combined with other materials.
Orthodontic bands, a type of fixed metal orthodontic appliance, remain in the mouth throughout treatment. Due to the large area of the bands compared with the bonded attachments and due to food becoming trapped around the bands, using an orthodontic band with antimicrobial properties makes it possible to prevent tooth decay and oral lesions.
In this study, we evaluated the cell viability and antimicrobial effect against S. mutans, L. acidophilus, and C. albicans associated with stainless steel orthodontic bands coated with nano-ZnO or nano-Ag. The results showed that nano-Ag–coated bands showed lower biocompatibility than nano-ZnO–coated bands, although the difference was not significant (0.42 ± 0.02 vs. 0.45 ± 0.02, p > 0.05). Furthermore, nano-Ag–coated bands (0.1 g nano-Ag in 3 mL acetone) showed a significantly higher reduction in the number of colonies of all three microorganisms (S. mutans, L. acidophilus, and C. albicans) than nano-ZnO–coated (nano-ZnO in 3 mL acetone) or uncoated bands. This result was consistent with that of Hernández-Sierra et al.,31 who evaluated the effects of nano-Ag (25 nm), and nano-ZnO (125 nm) on S. mutans and reported that the antimicrobial activity of nano-Ag is much higher than that of nano-ZnO and gold nanoparticles.31
The reduction in the number of colonies of all three microorganisms induced by each of the nanoparticles was statistically significant when compared with that in the control group. However, to use the term “antimicrobial” clinically, dental materials must show at least a 2-log reduction in bacterial ability compared with a control material.32 Accordingly, this term can be used for nano-Ag–coated bands because it showed the necessary reduction in the number of all three microorganisms’ colonies. For nano-ZnO–coated bands, the term “antimicrobial” can only be used for S. mutans because the reduction of L. acidophilus and C. albicans was less than 2-log. Nevertheless, these small reductions may also be clinically relevant.
The results of some previous studies33-35 contradict our results. Kasraei et al.33 concluded that ZnO had a more significant antimicrobial effect than Ag against S. mutans, but there was no difference between the two nanoparticles’ antimicrobial effect against L. acidophilus.33 However, we observed a significant difference in the mean number of S. mutans and L. acidophilus colonies between the Ag and ZnO groups. This difference in results may be due to differences in nanoparticle size and concentration; Kasraei et al.33 used 1% solutions of nano-ZnO and nano-Ag, with an average particle size of 50 nm and 20 nm, respectively. Reducing the nanoparticle size increases the specific surface area. Hence, the interaction of the nanoparticles with the environment increases. Besides, cell wall penetration is facilitated by small particle sizes. Therefore, the antimicrobial effect of substances like Ag and ZnO, which are naturally antimicrobial, is enhanced by increasing the surface/volume ratio.
Hailan and Al-Khatieeb34 demonstrated a reduction in the number of S. mutans colonies around primer discs containing nano-ZnO and nano-Ag, which is consistent with our results. However, in their study, the antimicrobial effect of nano-ZnO against S. mutans was significantly higher than that of nano-Ag, which is inconsistent with the results of our study.34 These differences might be attributed to the size of the nanoparticles used; in their study, the nano-ZnO (50 nm) was smaller than the nano-Ag (80 nm), while in our study, the average size of both nanoparticles was 20 nm. The concentration of both nanoparticles in the primer in the study of Hailan and Al-Khatieeb34 was 1%. In our study, the bands were coated with 0.1 g of Ag or 0.1 g of ZnO in 3 mL of acetone.
Prabha et al.36 demonstrated the antimicrobial effect of nano-Ag against gram-positive pathogens. They used the thermal evaporation method to coat orthodontic bands (vacuum of 5 × 10−5 millibar at 961°C for 10 minutes) and showed the biocompatibility of bands coated with nanoparticles. In our study, the antimicrobial effect and biocompatibility of nano-Ag and nano-ZnO were compared, although our results differed from theirs. The difference in the FESEM findings of the two studies was due to the method of coating the bands. In our study, the bands were coated using the electrostatic spray-assisted vapor deposition method (distance: 3 cm, rate: 200 rpm, and voltage: 8 kV), which is cost-effective and suitable for coating alloys and metal objects.37
Several in vitro studies have been performed on the mechanisms underlying the toxicity of nano-ZnO and nano-Ag in mammalian cells.38-40 These studies revealed that the intrinsic toxicity of the metals and solubility of the nanoparticles dictated by the metals’ chemical features, uptake, and potential to induce oxidative stress were the main mechanisms underlying the cytotoxicity.
Clinical trials are the most reliable way to determine biocompatibility. Nonetheless, ethical considerations limit their use. It is essential to assess the toxicity and biocompatibility of dental materials before they are used in clinical practice. The use of biocompatible dental materials presents no or minimal danger.41 The MTT analysis indicated that the orthodontic bands coated with nano-Ag or nano-ZnO had no major or significant cytotoxic effects. From our results, it is evident that all coated bands significantly prevent the growth of the primary bacteria responsible for dental caries (S. mutans, Lactobacillus spp, and C. albicans) and reduce the likelihood of plaque accumulation and dental caries in the long orthodontic treatment duration. Nano-ZnO–coated bands are considered to be more useful than nano-Ag–coated bands because they are less toxic and have antimicrobial properties that are comparable to those of nano-Ag coated bands.
After comprehensively searching the dental literature, we believe this is the first study to compare the antimicrobial properties of nano-Ag–coated bands and nano-ZnO–coated bands. One strength of this study is the investigation and comparison of the antimicrobial effects of these nanoparticles against the main microorganisms implicated in the formation of dental caries and the biocompatibility of these nanoparticles. However, certain limitations exist. First, we did not use a brushing machine to equalize the speed and force of brushing when checking whether the coating adhered to the surface. Second, we only investigated the antimicrobial effect on a single species, and multispecies biofilms were not evaluated. Third, although we assessed the impact of nanoparticle coatings on cariogenic microorganisms, peri-pathogenic bacteria remain to be investigated in a future study.
Further studies are needed on the durability of the ions released from nanoparticle-coated bands and the changes in the physical properties of the material, such as its long-term stability in the oral environment and the retention of nanoparticles during clinical application.
We found that the antimicrobial properties of coated orthodontic bands were significantly higher than those of uncoated bands. The antimicrobial properties of nano-Ag–coated bands were significantly higher than those of nano-ZnO–coated bands. The lowest cell viability rate was observed in the nano-Ag–coated bands, and the highest cell viability rate was observed in the uncoated bands, but this difference was not statistically significant.
ACKNOWLEDGEMENT
This study was part of a specialty in orthodontics thesis supported by Tehran University of Medical Sciences (Grant no. 9811114002). This study was also funded and supported by Tehran University of Medical Sciences (Grant no. 1400-2-133-54440).
Notes
REFERENCES
1. Antonio-Zancajo L, Montero J, Albaladejo A, Oteo-Calatayud MD, Alvarado-Lorenzo A. 2020; Pain and oral-health-related quality of life in orthodontic patients during initial therapy with conventional, low-friction, and lingual brackets and aligners (Invisalign): a prospective clinical study. J Clin Med. 9:2088. DOI: 10.3390/jcm9072088. PMID: 32635196. PMCID: PMC7408790. PMID: ae922d85ec61459999b0394a5d12238d.


2. Erbe C, Hornikel S, Schmidtmann I, Wehrbein H. 2011; Quantity and distribution of plaque in orthodontic patients treated with molar bands. J Orofac Orthop. 72:13–20. DOI: 10.1007/s00056-010-0001-4. PMID: 21484542.


3. Anhoury P, Nathanson D, Hughes CV, Socransky S, Feres M, Chou LL. 2002; Microbial profile on metallic and ceramic bracket materials. Angle Orthod. 72:338–43. DOI: 10.1043/0003-3219(2002)072<0338:MPOMAC>2.0.CO;2. PMID: 12169034.
4. Manuelli M, Marcolina M, Nardi N, Bertossi D, De Santis D, Ricciardi G, et al. 2019; Oral mucosal complications in orthodontic treatment. Minerva Stomatol. 68:84–8. DOI: 10.23736/S0026-4970.18.04127-4. PMID: 30854838.


5. Bishara SE, Ostby AW. 2008; White spot lesions: formation, prevention, and treatment. Semin Orthod. 14:174–82. DOI: 10.1053/j.sodo.2008.03.002.


6. Walsh LJ, Healey DL. 2019; Prevention and caries risk management in teenage and orthodontic patients. Aust Dent J. 64 Suppl 1:S37–45. DOI: 10.1111/adj.12671. PMID: 31144319.


7. Lacerda Rangel Esper MÂ, Junqueira JC, Uchoa AF, Bresciani E, Navarro RS, Nara de Souza Rastelli A, et al. 2019; Photodynamic inactivation of planktonic cultures and Streptococcus mutans biofilms for prevention of white spot lesions during orthodontic treatment: an in vitro investigation. Am J Orthod Dentofacial Orthop. 155:243–53. Erratum in: Am J Orthod Dentofacial Orthop 2019;155:458. DOI: 10.1016/j.ajodo.2018.03.027. PMID: 30712696.
8. Beyth N, Houri-Haddad Y, Baraness-Hadar L, Yudovin-Farber I, Domb AJ, Weiss EI. 2008; Surface antimicrobial activity and biocompatibility of incorporated polyethylenimine nanoparticles. Biomaterials. 29:4157–63. DOI: 10.1016/j.biomaterials.2008.07.003. PMID: 18678404.


9. Lim BS, Lee SJ, Lee JW, Ahn SJ. 2008; Quantitative analysis of adhesion of cariogenic streptococci to orthodontic raw materials. Am J Orthod Dentofacial Orthop. 133:882–8. DOI: 10.1016/j.ajodo.2006.07.027. PMID: 18538253.


10. Shah AG, Shetty PC, Ramachandra CS, Bhat NS, Laxmikanth SM. 2011; In vitro assessment of photocatalytic titanium oxide surface modified stainless steel orthodontic brackets for antiadherent and antibacterial properties against Lactobacillus acidophilus. Angle Orthod. 81:1028–35. DOI: 10.2319/021111-101.1. PMID: 22007663. PMCID: PMC8903869.
11. De Stefani A, Bruno G, Preo G, Gracco A. 2020; Application of nanotechnology in orthodontic materials: a state-of-the-art review. Dent J (Basel). 8:126. DOI: 10.3390/dj8040126. PMID: 33182424. PMCID: PMC7712537. PMID: edbfccbb4482466e9c6ea6eba21d3ea4.


12. Doudi M, Naghsh N, Heiedarpour A. 2011; The effect of silver nanoparticles on gram-negative bacilli resistant to extended-spectrum β-lactamase enzymes. Med Lab J. 5:44–51.
13. Lloyd JR. 2003; Microbial reduction of metals and radionuclides. FEMS Microbiol Rev. 27:411–25. DOI: 10.1016/S0168-6445(03)00044-5. PMID: 12829277.


14. Bürgers R, Eidt A, Frankenberger R, Rosentritt M, Schweikl H, Handel G, et al. 2009; The anti-adherence activity and bactericidal effect of microparticulate silver additives in composite resin materials. Arch Oral Biol. 54:595–601. DOI: 10.1016/j.archoralbio.2009.03.004. PMID: 19375069.


15. Spacciapoli P, Buxton D, Rothstein D, Friden P. 2001; Antimicrobial activity of silver nitrate against periodontal pathogens. J Periodontal Res. 36:108–13. DOI: 10.1034/j.1600-0765.2001.360207.x. PMID: 11327077.


16. Ohira T, Yamamoto O, Iida Y, Nakagawa ZE. 2008; Antibacterial activity of ZnO powder with crystallographic orientation. J Mater Sci Mater Med. 19:1407–12. DOI: 10.1007/s10856-007-3246-8. PMID: 17914627.


17. Afonso Camargo SE, Mohiuddeen AS, Fares C, Partain JL, Carey PH 4th, Ren F, et al. 2020; Anti-bacterial properties and biocompatibility of novel SiC coating for dental ceramic. J Funct Biomater. 11:33. DOI: 10.3390/jfb11020033. PMID: 32443691. PMCID: PMC7353563. PMID: 114fa56d7f834778a4ccad9da19491fe.


18. Miles AA, Misra SS, Irwin JO. 1938; The estimation of the bactericidal power of the blood. J Hyg (Lond). 38:732–49. DOI: 10.1017/S002217240001158X. PMID: 20475467. PMCID: PMC2199673.


19. International Organization for Standardization (ISO). 2009. ISO 10993-5:2009. Biological evaluation of medical devices- part 5: tests for in vitro cytotoxicity. ISO Copyright Office;Geneva:
20. Tavassoli-Hojjati S, Haghgoo R, Mehran M, Niktash A. 2012; Evaluation of the effect of fluoride gel and varnish on the demineralization resistance of enamel: an in vitro. J Iran Dent Assoc. 24:28–34.
21. Farhadian N, Usefi Mashoof R, Khanizadeh S, Ghaderi E, Farhadian M, Miresmaeili A. 2016; Streptococcus mutans counts in patients wearing removable retainers with silver nanoparticles vs those wearing conventional retainers: a randomized clinical trial. Am J Orthod Dentofacial Orthop. 149:155–60. Erratum in: Am J Orthod Dentofacial Orthop 2017;151:11. DOI: 10.1016/j.ajodo.2015.07.031. PMID: 26827971.
22. Arash V, Keikhaee F, Rabiee SM, Rajabnia R, Khafri S, Tavanafar S. 2016; Evaluation of antibacterial effects of silver-coated stainless steel orthodontic brackets. J Dent (Tehran). 13:49–54. PMID: 27536328. PMCID: PMC4983565. PMID: 93db76e397d94eb4b16f3f304c3d33e2.
23. Ghorbanzadeh R, Pourakbari B, Bahador A. 2015; Effects of baseplates of orthodontic appliances with in situ generated silver nanoparticles on cariogenic bacteria: a randomized, double-blind cross-over clinical trial. J Contemp Dent Pract. 16:291–8. DOI: 10.5005/jp-journals-10024-1678. PMID: 26067732.


24. Poosti M, Ramazanzadeh B, Zebarjad M, Javadzadeh P, Naderinasab M, Shakeri MT. 2013; Shear bond strength and antibacterial effects of orthodontic composite containing TiO2 nanoparticles. Eur J Orthod. 35:676–9. DOI: 10.1093/ejo/cjs073. PMID: 23264617.


25. Jedrychowski JR, Caputo AA, Kerper S. 1983; Antibacterial and mechanical properties of restorative materials combined with chlorhexidines. J Oral Rehabil. 10:373–81. DOI: 10.1111/j.1365-2842.1983.tb00133.x. PMID: 6355413.


26. Bulut H, Türkün M, Türkün LS, Işiksal E. 2007; Evaluation of the shear bond strength of 3 curing bracket bonding systems combined with an antibacterial adhesive. Am J Orthod Dentofacial Orthop. 132:77–83. DOI: 10.1016/j.ajodo.2005.06.040. PMID: 17628254.


27. Kachoei M, Divband B, Rahbar M, Esmaeilzadeh M, Ghanizadeh M, Alam M. 2021; A novel developed bioactive composite resin containing silver/zinc oxide (Ag/ZnO) nanoparticles as an antimicrobial material against Streptococcus mutans, Lactobacillus, and Candida albicans. Evid Based Complement Alternat Med. 2021:4743411. DOI: 10.1155/2021/4743411. PMID: 34697547. PMCID: PMC8541865.
28. Garmasheva I, Kovalenko N, Voychuk S, Ostapchuk A, Livins'ka O, Oleschenko L. 2016; Lactobacillus species mediated synthesis of silver nanoparticles and their antibacterial activity against opportunistic pathogens in vitro. Bioimpacts. 6:219–23. DOI: 10.15171/bi.2016.29. PMID: 28265538. PMCID: PMC5326670.


29. Sharma VK, Yngard RA, Lin Y. 2009; Silver nanoparticles: green synthesis and their antimicrobial activities. Adv Colloid Interface Sci. 145:83–96. DOI: 10.1016/j.cis.2008.09.002. PMID: 18945421.


30. Chen X, Schluesener HJ. 2008; Nanosilver: a nanoproduct in medical application. Toxicol Lett. 176:1–12. DOI: 10.1016/j.toxlet.2007.10.004. PMID: 18022772.


31. Hernández-Sierra JF, Ruiz F, Pena DC, Martínez-Gutiérrez F, Martínez AE, Guillén Ade J, et al. 2008; The antimicrobial sensitivity of Streptococcus mutans to nanoparticles of silver, zinc oxide, and gold. Nanomedicine. 4:237–40. DOI: 10.1016/j.nano.2008.04.005. PMID: 18565800.


32. Cieplik F, Aparicio C, Kreth J, Schmalz G. 2022; Development of standard protocols for biofilm-biomaterial interface testing. JADA Found Sci. 1:100008. DOI: 10.1016/j.jfscie.2022.100008.


33. Kasraei S, Sami L, Hendi S, Alikhani MY, Rezaei-Soufi L, Khamverdi Z. 2014; Antibacterial properties of composite resins incorporating silver and zinc oxide nanoparticles on Streptococcus mutans and Lactobacillus. Restor Dent Endod. 39:109–14. DOI: 10.5395/rde.2014.39.2.109. PMID: 24790923. PMCID: PMC3978100.


34. Hailan SY, Al-Khatieeb MM. 2019; Antimicrobial efficacy of silver, zinc oxide, and titanium dioxide nanoparticles incorporated in orthodontic bonding agent. J Baghdad Coll Dent. 31:10–6. DOI: 10.26477/jbcd.v31i3.2693. PMID: 2adb939a2c1747d28c8cc28b100e6b21.


35. Ahrari F, Eslami N, Rajabi O, Ghazvini K, Barati S. 2015; The antimicrobial sensitivity of Streptococcus mutans and Streptococcus sangius to colloidal solutions of different nanoparticles applied as mouthwashes. Dent Res J (Isfahan). 12:44–9. DOI: 10.4103/1735-3327.150330. PMID: 25709674. PMCID: PMC4336971.


36. Prabha RD, Kandasamy R, Sivaraman US, Nandkumar MA, Nair PD. 2016; Antibacterial nanosilver coated orthodontic bands with potential implications in dentistry. Indian J Med Res. 144:580–6. DOI: 10.4103/0971-5916.200895. PMID: 28256467. PMCID: PMC5345305.
37. Halimi SU, Bakar NFA, Ismail SN, Hashib SA. 2014; Electrospray deposition of titanium dioxide (TiO2) nanoparticles. AIP Conf Proc. 1586:57. DOI: 10.1063/1.4866730.


38. Bondarenko O, Juganson K, Ivask A, Kasemets K, Mortimer M, Kahru A. 2013; Toxicity of Ag, CuO and ZnO nanoparticles to selected environmentally relevant test organisms and mammalian cells in vitro: a critical review. Arch Toxicol. 87:1181–200. DOI: 10.1007/s00204-013-1079-4. PMID: 23728526. PMCID: PMC3677982.


39. Kim S, Choi JE, Choi J, Chung KH, Park K, Yi J, et al. 2009; Oxidative stress-dependent toxicity of silver nanoparticles in human hepatoma cells. Toxicol In Vitro. 23:1076–84. DOI: 10.1016/j.tiv.2009.06.001. PMID: 19508889.


40. Lesniak A, Salvati A, Santos-Martinez MJ, Radomski MW, Dawson KA, Åberg C. 2013; Nanoparticle adhesion to the cell membrane and its effect on nanoparticle uptake efficiency. J Am Chem Soc. 135:1438–44. DOI: 10.1021/ja309812z. PMID: 23301582.


41. Shahi S, Özcan M, Maleki Dizaj S, Sharifi S, Al-Haj Husain N, Eftekhari A, et al. 2019; A review on potential toxicity of dental material and screening their biocompatibility. Toxicol Mech Methods. 29:368–77. DOI: 10.1080/15376516.2019.1566424. PMID: 30642212.


Figure 1
Cells after the addition of eluents but before 3-(4, 5-dimethylthiazol-2-yl)-2, 5-diphenyltetrazolium bromide assay. A, Uncontacted cells with the band. B, Contacted cells with the nano-Ag–coated band. C, Contacted cells with the nano-ZnO–coated band. D, Contacted cells with the uncoated band.
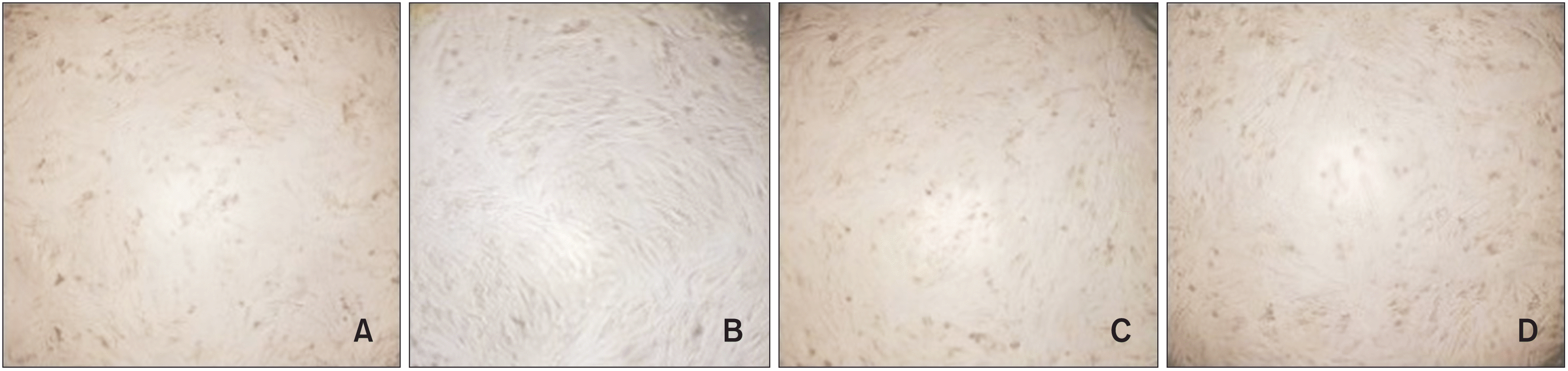
Figure 2
Characterization of synthesized Ag nanoparticles. A, Transmission electron microscopy image of Ag nanoparticles in optimal conditions (scale bar: 20 nm). B, Field emission scanning electron microscopy image of Ag nanoparticles in optimal conditions (scale bar: 200 nm). C, X-ray diffraction graph of Ag nanoparticles.
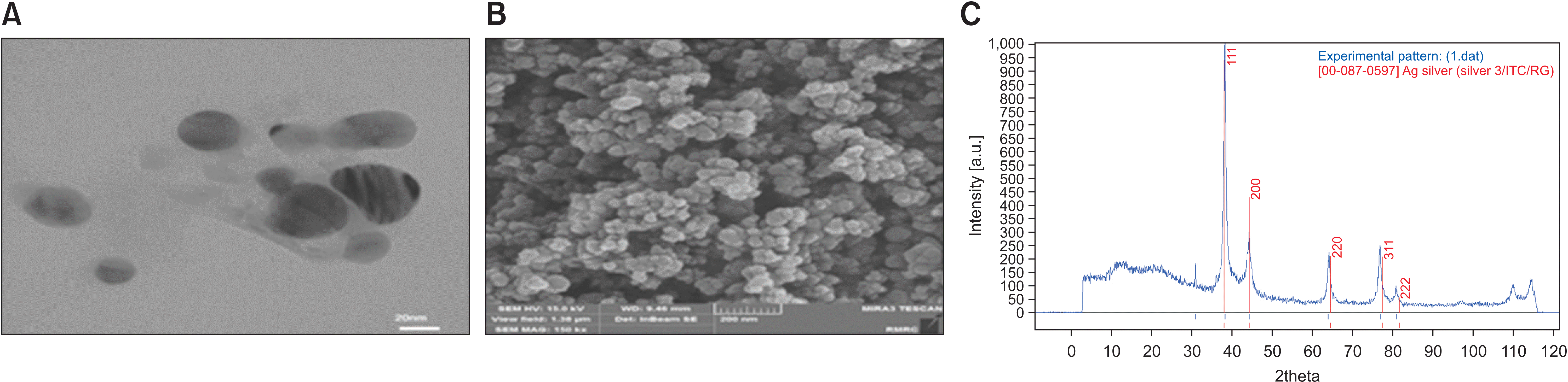
Figure 3
Characterization of synthesized ZnO nanoparticles. A, Transmission electron microscopy image of ZnO nanoparticles in optimal conditions (scale bar: 20 nm). B, Field emission scanning electron microscopy image of ZnO nanoparticles in optimal conditions (scale bar: 200 nm). C, X-ray diffraction graph of ZnO nanoparticles.
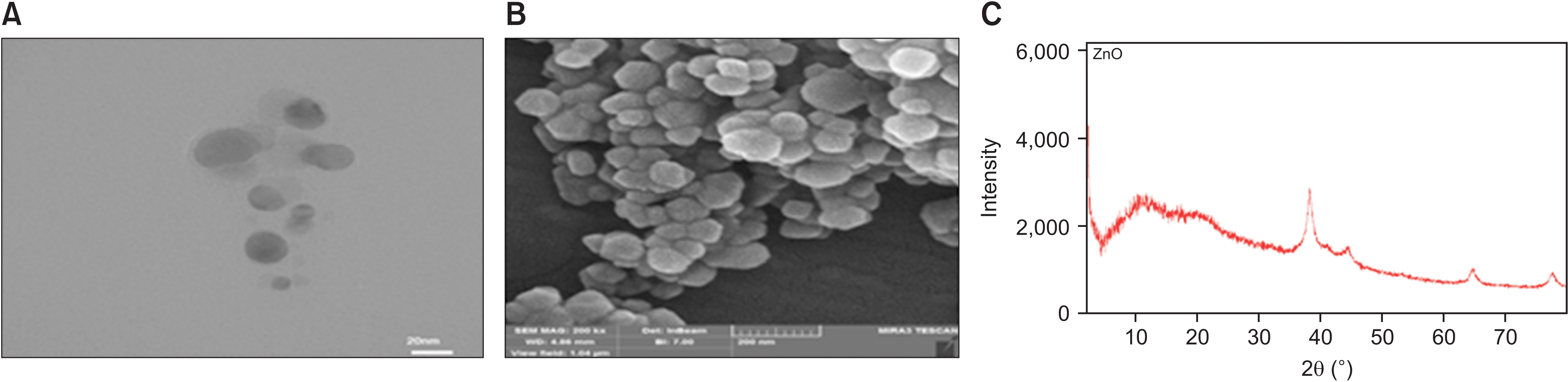
Figure 4
Band surface coated with ZnO nanoparticles. A, Field emission scanning electron microscopy image. B, Energy-dispersive X-ray spectroscopy analysis.
L, length. L1, L2 and L3 were randomly selected.
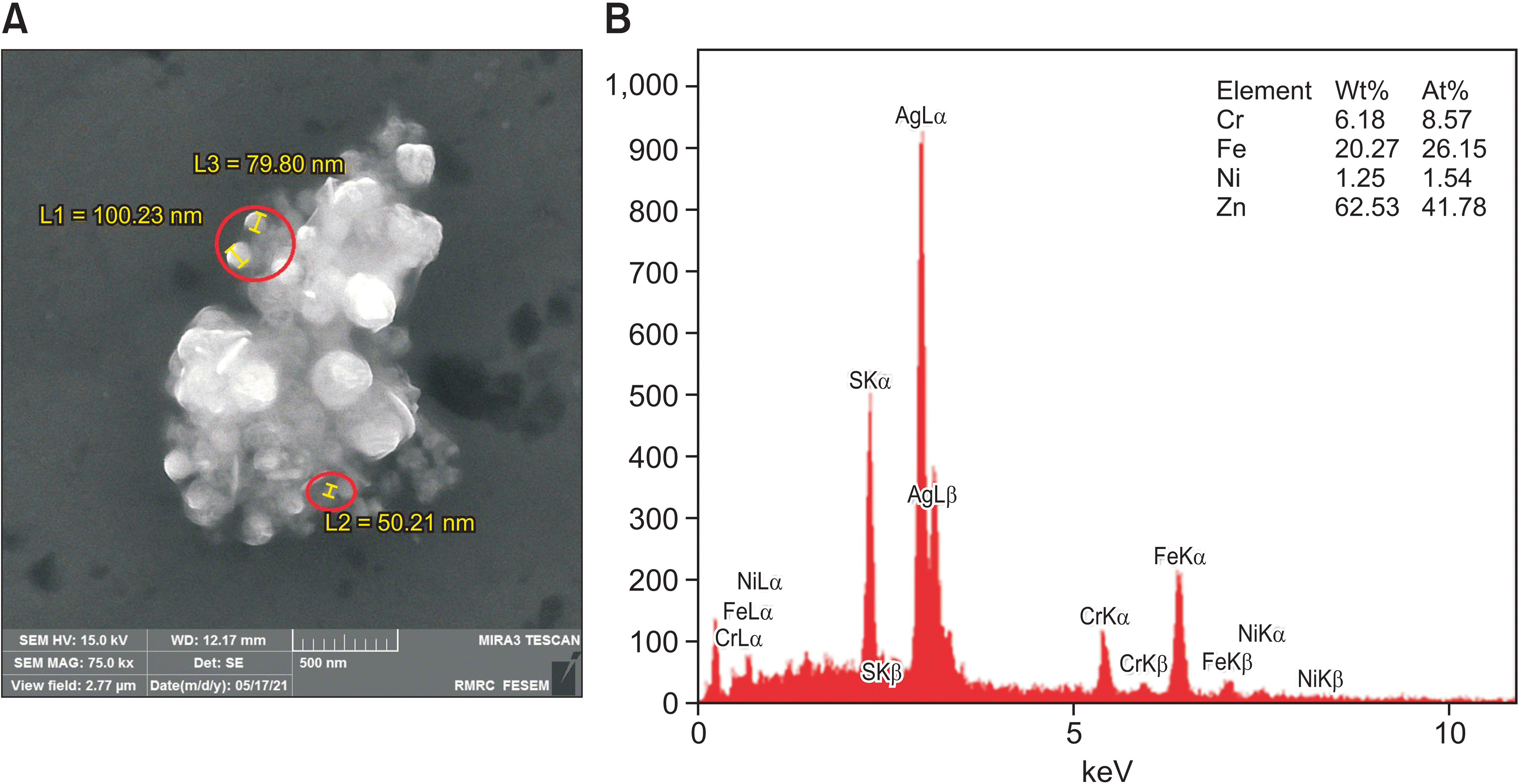
Figure 5
Band surface coated with Ag nanoparticles. A, Field emission scanning electron microscopy image. B, Energy-dispersive X-ray spectroscopy analysis.
L, length. L1, L2 and L3 were randomly selected.
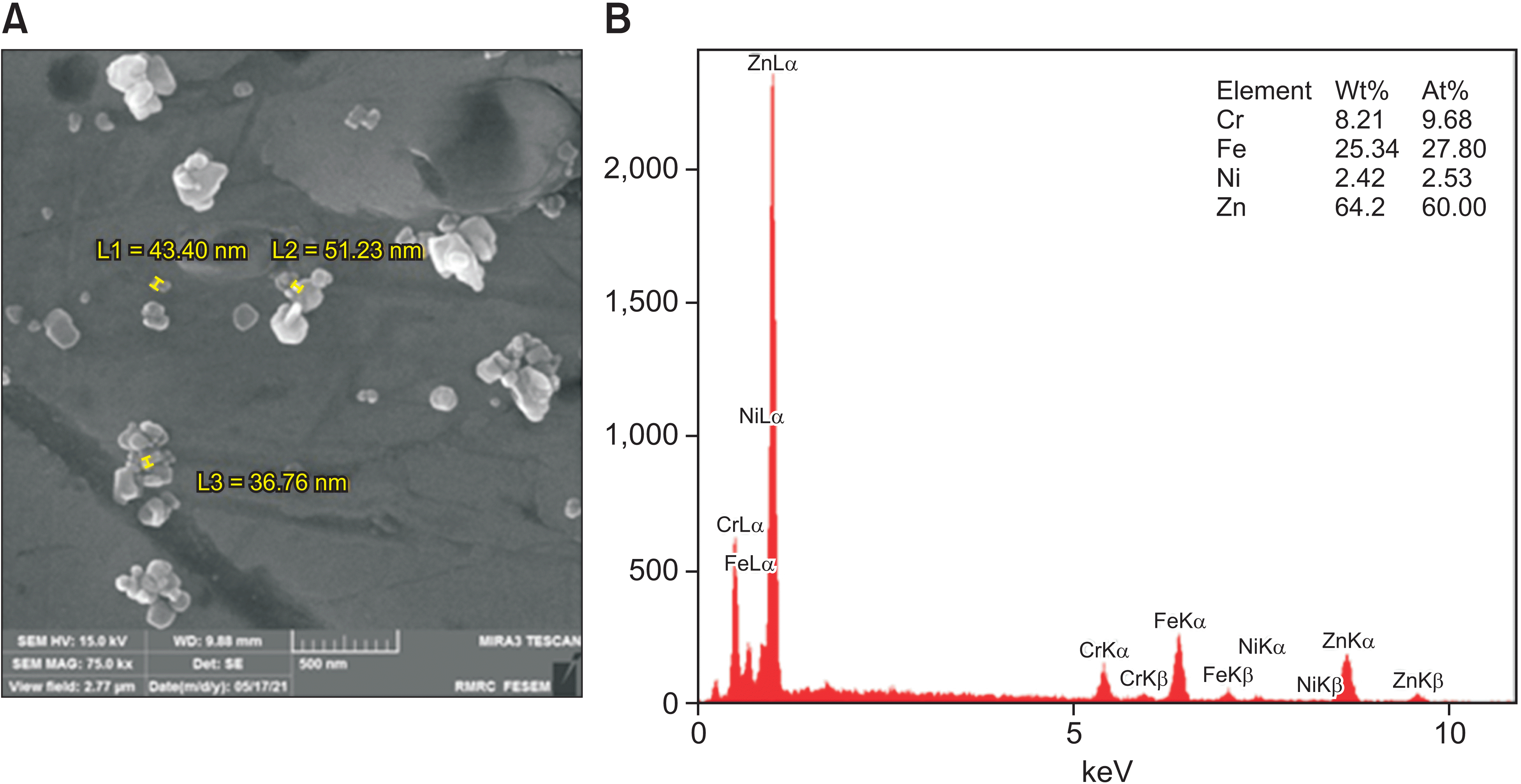
Figure 6
Colony count of the microorganisms for the three groups (colony forming units/mL).
SE, standard error.
*Significantly different with p < 0.05.
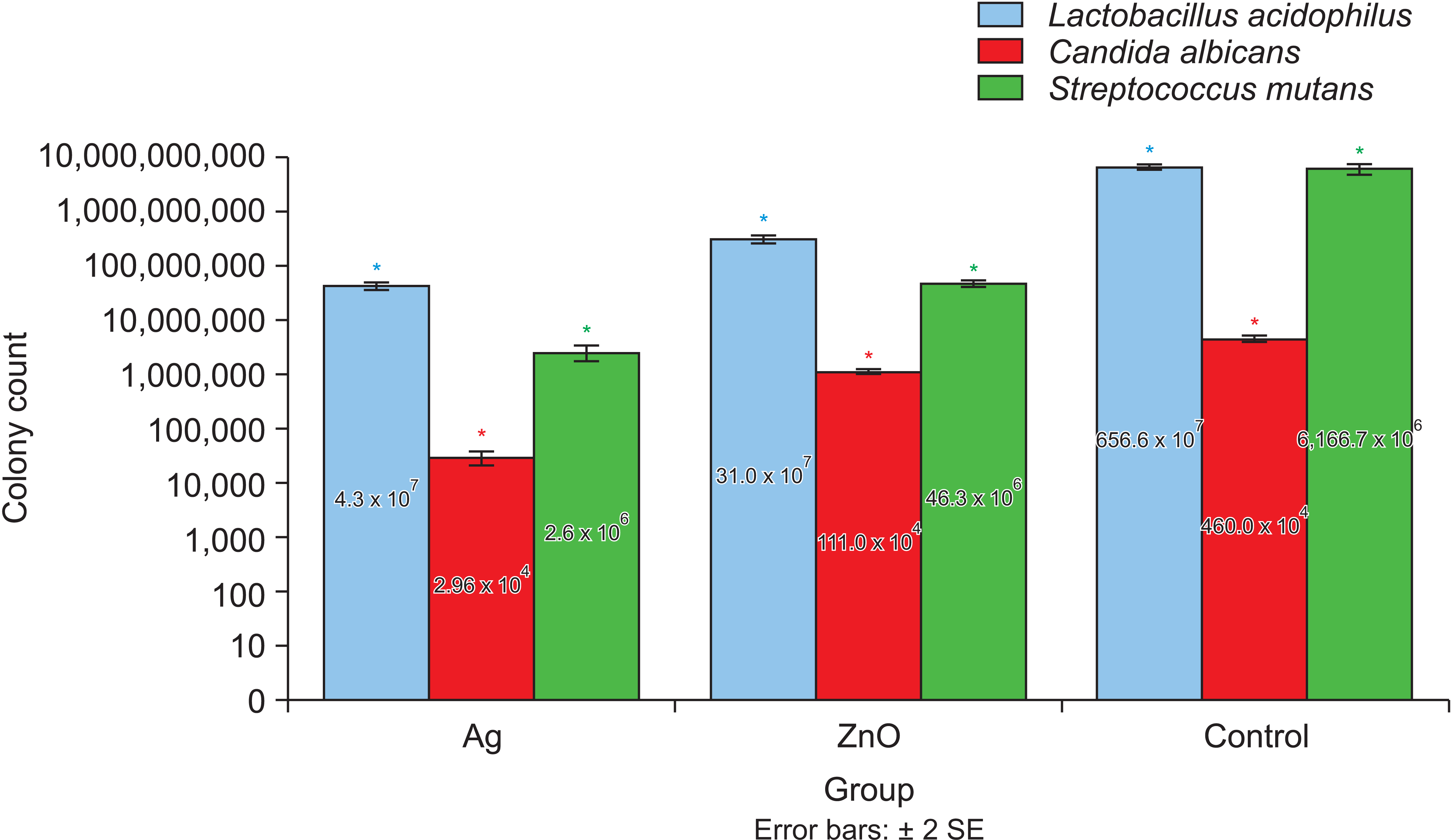
Figure 7
Mean cell viability in each group. A, Uncontacted cells with the band. B, Contacted cells with the nano-Ag–coated band. C, Contacted cells with the nano-ZnO–coated band. D, Contacted cells with the uncoated band.
CI, confidence interval; SE, standard error.
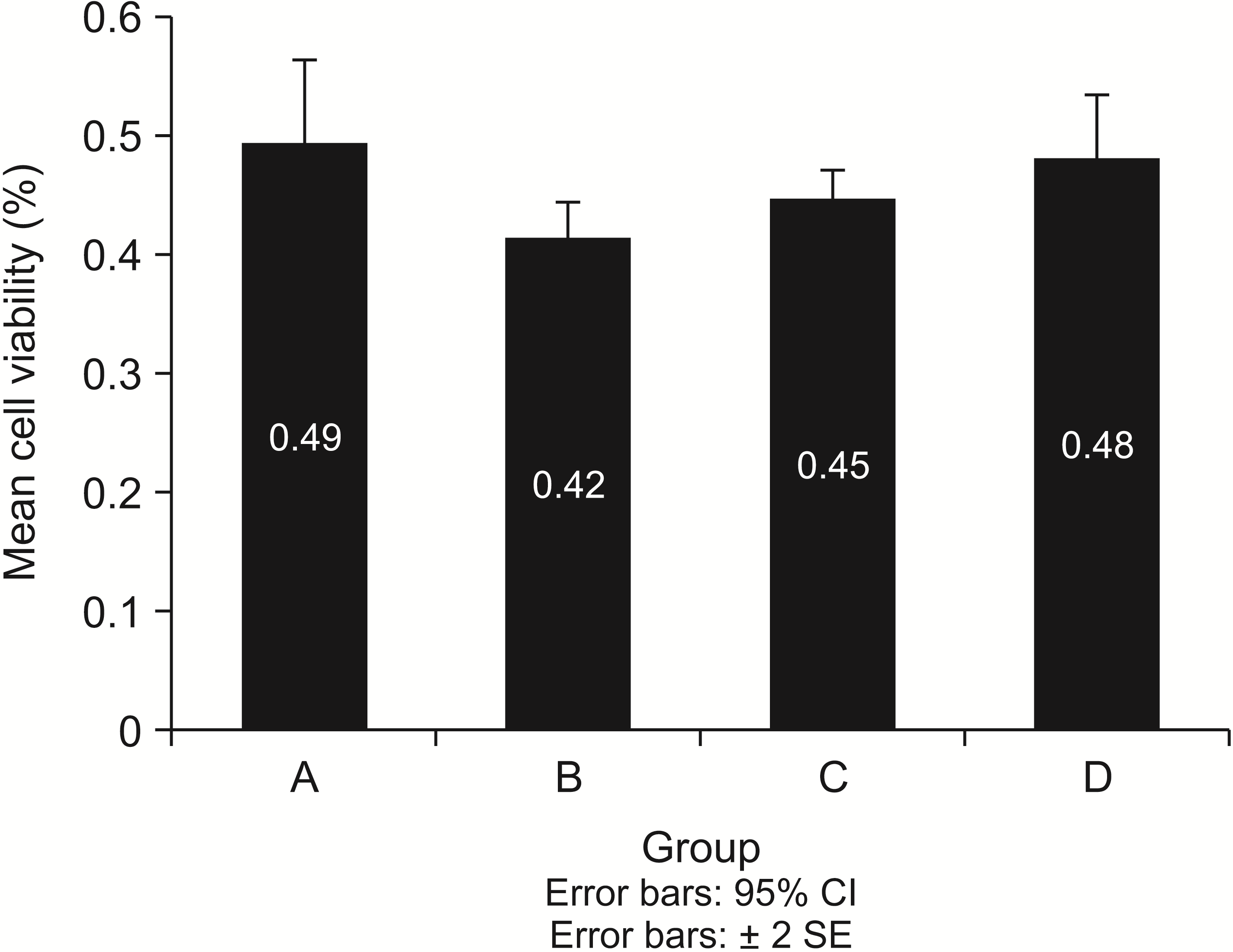
Figure 8
Monolayer culture of the 10459 human gingival fibroblast (HGF) cell line was used for indirect contact assay. A, The control sample consists of a confluent layer of fibroblast cells. Most of the cells are spindle-shaped, which is considered normal. B, The band coated with Ag nanoparticles showed no change in cell morphology following contact with the 10459 HGF confluent layer. C, The 10459 HGF cells in contact with the band coated with ZnO nanoparticles also showed normal morphology. D, The 10459 HGF cells in contact with the uncoated band are spindle-shaped. A, Uncontacted cells with the band; B, Contacted cells with the nano-Ag–coated band; C, Contacted cells with nano-ZnO–coated band; and D, Contacted cells with the uncoated band.
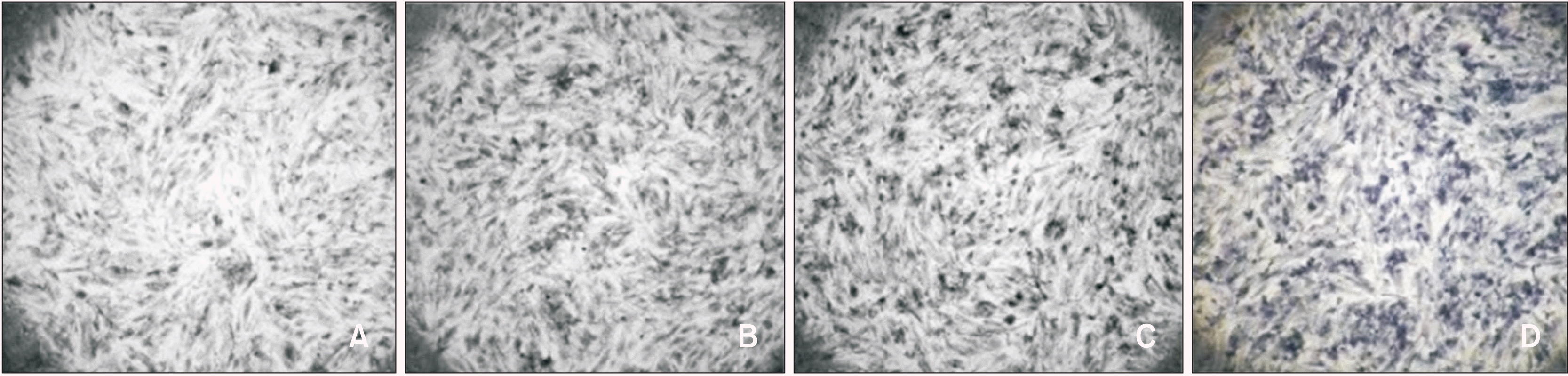
Table 1
Descriptive values of the colony count for each microbial strain in the three groups (colony forming units/mL)