Abstract
The poor outcome of advanced ovarian cancer under conventional therapy necessitates new strategies to improve therapeutic efficacy. β-glucosidase (encoded by GBA) is a lysosomal enzyme and is involved in sphingolipids metabolism. Recent studies revealed that β-glucosidase plays a role in cancer development and chemoresistance. In this work, we systematically evaluated the expression and role of GBA in ovarian cancer. Our work demonstrates that inhibition of β-glucosidase has therapeutic potential for ovarian cancer. Gene Expression Profiling Interactive Analysis database, western blot and immunohistochemistry analyses of patient samples demonstrated that GBA mRNA and protein expression levels were significantly increased in ovarian cancer compared to normal tissues. Functional studies using gain-of-function and loss-of-function approaches demonstrated that GBA overexpression did not affect growth and migration but alleviated cisplatin’s efficacy in ovarian cancer cells. In addition, GBA depletion resulted in growth inhibition, apoptosis induction, and enhancement of cisplatin’s efficacy. Of note, we found that GBA inhibition specifically decreased receptor tyrosine kinase AXL level, leading to the suppression of AXL-mediated signaling pathways. Our data suggest that GBA represents a promising target to inhibit AXL signaling and overcome cisplatin resistance in ovarian cancer.
Ovarian cancer represents the fifth leading cause of cause-related deaths and the incidence is gradually increasing worldwide [1]. The treatment options for ovarian cancer are limited: cytoreductive surgery and combination of platinum (e.g., cisplatin and carboplatin)-based and taxane (paclitaxel and docetaxel)-based chemotherapy [2]. Unfortunately, approximately 70% of patients eventually develop resistance and experience cycles of relapse [3,4]. The molecular mechanisms leading to ovarian cancer progression and resistance are complex and may involve alterations in dysregulation of cellular metabolism, DNA damage repair, alternation in epigenetics and oncogenic activation, and epithelial-mesenchymal transition [5]. Identification of molecules involved in ovarian cancer development is important to develop targeted therapeutic strategies to improve clinical management of ovarian cancer.
β-glucosidase is a lysosomal enzyme encoded by GBA gene. It is involved in the salvage pathway of sphingolipids metabolism via cleaving the glycosidic linkage of glucosylceramide to generate ceramide and release glucose [6,7]. Defects in β-glucosidase activity results in the aberrant accumulation of lipid substrates in macrophages and this is associated with Gaucher’s disease and Parkinson’s disease [8-12]. It has been shown that β-glucosidase-ceramide axis inhibits interleukin-6 (IL-6) biosynthesis which plays essential role in inflammation and cancer [13-15]. The role of β-glucosidase in cancer remains to be revealed and there are only few relevant studies. β-glucosidase upregulation contributes to the development of chemoresistance in hepatocellular carcinoma [16]. GBA inhibition is active against breast and gastric cancer cellular activities, and further sensitizes them to chemotherapy [17,18].
In this study, we systematically evaluated the expression and role of GBA in ovarian cancer through 1) analysing the expression level of GBA in ovarian cancer and matching normal tissues from patient samples; 2) performing functional studies using genetic approach; 3) determining the therapeutic potential of GBA inhibition; and 4) analysing the molecular mechanism of GBA inhibition, in ovarian cancer cells.
Two human ovary adenocarcinoma cell lines SK-OV-3 and Caov3 were obtained from Guangzhou Jennio Biotechnology and authenticated using human 9-Marker short-tandem repeat (STR) profile analysis (Beyotime Biotechnology, Jiangsu, China). Cells were cultured using RPMI 1640 medium supplemented with 10% fetal bovine serum (FBS) and 2 mM L-glutamine (Invitrogen, Carlsbad, CA, USA). Cisplatin was obtained from Selleckchem. Cell proliferation was assessed using BrdU Proliferation Assay Kit (Abcam, Cambridge, MA, USA) as per manufacture’s protocol.
A total of 31 ovarian cancer tissues and adjacent normal tissue samples (> 5 cm away from the tumor edge) were obtained from ovarian cancer patients undergoing surgical resection at the Wuhan Third Hospital. Written informed consent was obtained from all patients under Institutional Review Board approved protocols. This study was performed according to guidelines approved by the ethics committee of Wuhan Third Hospital-Tongren Hospital of Wuhan University (approval ID: 201806JK and approval date: year 2018). The samples were stored in –80°C for protein extraction or immediately proceeded for fixation using 4% paraformaldehyde, paraffin embedding and tissue sectioning. Immunohistochemistry of tissue sections were performed using the standard protocol [19]. Briefly, slides were deparaffinised, hydrated, antigen retrieved and stained with anti-β-glucosidase antibody (sc-166407; Santa Cruz Biotechnology, Dallas, TX, USA). Slides were then stained with 3,3’-diaminobenzidine and hematoxylin. IHC staining was scored under microscope and quantified by ImageJ software (NIH, Bethesda, MD, USA).
Cells were stained using the Annexin V-fluorescein isothiocyanate/7-aminoactinomycin D (V-FITC/7-AAD) apoptosis kit (Abnova, Walnut, CA, USA) as per manufacturer’s instructions. The quantification of Annexin V was determined using flow cytometry (MACSQuant; Miltenyi Biotec, Gaithersburg, MD, USA).
For our experiments, 1 g of frozen normal and tumor tissues were homogenized using a polytron homogenizer or or 106 cells were trypsinzed, and were resuspended in ice-cold RIRA buffer containing phosphatase and protease inhibitors (Roche, Basel, Switzerland) for total proteins extraction. Proteins were loaded onto denaturing sodium dodecyl sulfate–polyacrylamide gel and resolved via electrophoresis, and then transferred to polyvinylidene difluoride (PVDF) membrane (Thermo Fisher Scientific, Waltham, MA, USA). Western blot was performed using standard protocol using antibodies from Cell Signaling. Visualization of protein density was achieved by using Amersham ECL Western blotting detection kit (GE HealthCare, Waukesha, WI, USA). The density was quantified using Image J software. GBA protein level was also measured using the Human Glucosylceramidase (GBA) ELISA kit (MyBioSource, San Diego, CA, USA) as per manufacture’s protocol. Briefly, tissues samples were adjusted to the concentration of 5 mg/ml using PBS. Blank was used as background control and standards were used to generate standard concentration curve. The optical density of each well was determined on a microplate reader at 450 nm.
Transfections of siRNA were performed with Lipofectamine TM 2000 and OptiMEM (Invitrogen) using the same protocol as per manufacture’s instruction. GBA-overexpressing plasmid was generated by cloning GBA into pCMV3-C-his vector (Sino Biological, Waukesha, PA, USA). The sequences of GBA siRNA and NC siRNA were indicated in Supplementary Table 1. Protein expression analysis was performed at 48 h post-transfection.
We assessed the expression pattern of GBA in ovarian cancer and normal counterpart based on the data obtained from the RNA-Seq datasets GEPIA (Gene Expression Profiling Interactive Analysis) [20]. GBA mRNA level from 424 ovarian cancer samples and 88 normal samples were shown in Fig. 1A. A significant increase of GBA expression level was observed in ovarian cancer compared to normal. To verify this finding, we performed the IHC analysis of β-glucosidase in ovarian cancer tissues and their matching normal tissues from 31 patients. Among these patients, 20 are serous, 5 are mucinous and 6 are endometroid, and their clinicopathological information were presented in Supplementary Table 2. Representative IHC images of ovarian cancer patients with all three subtypes showed an increased β-glucosidase staining on tumor compared to normal (Fig. 1B, Supplementary Fig. 1). Quantification of β-glucosidase IHC staining showed that the average expression of β-glucosidase was significantly higher in tumor than normal (n = 31, Fig. 1C). Consistent with IHC analysis, we observed the same expression pattern of β-glucosidase in tumor and normal tissues by western blotting and ELISA analysis (n = 3, Fig. 1D and n = 25, Fig. 1E). Collectively, the consistent upregulation of GBA on its transcriptional and protein level suggests that GBA is likely to play an important role in the development of ovarian cancer.
We applied well-established gain-of-function approach to study the role of GBA in ovarian cancer. We overexpressed GBA in ovarian cancer cells: SK-OV-3 and Caov3, which are frequently used to model ovarian cancer for preclinical studies [21]. In addition, SK-OV-3 displays stem cell properties which are closely associated with ovarian cancer recurrence [22]. GBA overexpression in SK-OV-3 and Caov3 were confirmed as both cell lines showed up to 3-fold increase in β-glucosidase activity (Fig. 2A, B). We did not observe any significant difference on the growth and migration between control and GBA overexpressing-cells (Fig. 2C, D). We next exposed GBA-overexpressing cells to first-line chemotherapeutic agent cisplatin and assessed cell growth and survival. We found that there was a significantly more proliferation in GBA-overexpressing cells than control cells in the presence of cisplatin (Fig. 2E). In addition, a significantly less apoptosis induced by cisplatin was observed in GBA-overexpressing cells compared to control (Fig. 2F). These results demonstrate that GBA overexpression alleviates cytotoxic effects of cisplatin in ovarian cancer cells.
We further applied the loss-of-function approach to determine the therapeutic value of targeting GBA in ovarian cancer. Two siRNAs targeting different region of GBA were used. GBA knockdown in SK-OV-3 and Caov3 were confirmed as both cell lines displayed remarkable reduction of β-glucosidase protein level and activity (Fig. 3A, B). Compared to control cells transfected with scramble siRNA, GBA-depleted cells demonstrated reduced proliferation and increased apoptosis (Fig. 3C, D). In particular, GBA knockdown inhibited growth by 40%–50% and induced apoptosis by 30%–40% in SK-OV-3 and Caov3 cells. A significant further reduction of growth and a further induction of apoptosis were found in GBA-depleted cells in the presence of cisplatin compared with those subjects to cisplatin or GBA inhibition alone (Fig. 3C, D). These results demonstrate that 1) GBA inhibition is active against ovarian cancer; 2) GBA inhibition significantly enhances the inhibitory effects of cisplatin; 3) cisplatin augment the inhibitory effects of GBA inhibition.
To understand the molecular signaling pathways involved in GBA inhibition, we performed immunoblotting analysis of molecules which play role in ovarian cancer growth and survival. We found that GBA depletion resulted in reduction of both phosphorylated AXL and total AXL in SK-OV-3 cells (Fig. 4A, B). Interestingly, GBA overexpression did not affect levels of AXL nor p-AXL in ovarian cancer cells (Supplementary Fig. 2). PI3K/Akt/mTOR and Ras/ERK are the primary downstream signaling pathways of AXL [23]. We found that GBA depletion significantly decreased phosphorylation but not total Akt, mTOR and 4EBP1 (Fig. 4A, C). In addition, we observed a decreased p-ERK and p-90RSK but not ERK and 90RSK in GBA-depleted cells (Fig. 4A, D). It is noted that GBA inhibition decreased phosphorylation without affecting total level of these molecules, suggesting that reduction of total AXL level is the specific effect of GBA depletion. We attempted to rescue the inhibitory effects of GBA depletion using AKT activator SC79 which enhances phosphorylation of all three Akt isoforms [24]. We showed that SC79 partially but significantly reversed the pro-apoptotic and anti-proliferative effects of GBA depletion (Fig. 5). These results demonstrate that GBA depletion results in a reduction of AXL expression level and a subsequent disruption of AXL-mediated signaling pathways in ovarian cancer. In contrast, we did not observe the difference on AXL and p-AXL levels between cisplatin-treated and control ovarian cancer cells (Supplementary Fig. 3). In addition, cisplatin did not affect levels of p-Akt and p-mTOR (Supplementary Fig. 3). This finding is consistent with the fact that cisplatin acts on cancer cells via inhibiting DNA synthesis and inducing DNA damage [25].
It is well known that β-glucosidase (GBA) plays a role in metabolism of ceramide and sphingolipids and its abnormal expression/activity is associated with neurodegenerative disorders [8-12,26,27]. Although substantial evidence has highlighted that targeting sphingolipid metabolism has therapeutic value because sphinogolipids have key roles in the regulation of cancer cell signaling to control cancer growth, survival and response to treatment [28], the role of GBA in cancer remains largely unknown. Tumor/normal differential expression analysis based on the RNA sequencing expression data of up to 10,000 tumors and normal samples from The Cancer Genome Atlas (TCGA) and the Genetype-Tissue Expression projects demonstrates that GBA mRNA expression is significantly increased in many cancers, including breast cancer, glioma, ovarian cancer, leukemia, pancreatic cancer, hepatocellular carcinoma, melanoma and gastric adenocarcinoma [20]. This data suggests that GBA is highly likely to play a positive role in those cancers which display aberrant upregulation of GBA expression/activity. In support to this speculation, functional studies demonstrate that GBA is critically involved in the growth and survival of breast cancer and gastric cancer [16,17]. Using patient samples and multiple cell lines, our work adds ovarian cancer to the increasing list of cancers that their proliferation and survival are regulated by GBA. Experiments with immunohistochemistry staining, ELISA and western blot on patient samples show that there is a up to 3-fold increase of GBA protein level in ovarian cancer compared to normal counterparts. Our functional studies show that although GBA overexpression does not affect ovarian cancer cells, GBA inhibition remarkably suppresses growth and induces apoptosis.
Our work further shows that GBA overexpression alleviates cisplatin’s efficacy and GBA depletion augments cisplatin’s efficacy. These findings suggest that GBA might be involved in ovarian cancer chemoresistance. This speculation is supported by a recent study that GBA expression and activity in gastric cancer are regulated by prolonged exposure of chemotherapy, and GBA inhibition sensitizes gastric cancer to chemotherapy [18]. Based on our findings and previous work, it would be interesting to explore the role of GBA in the development of ovarian cancer chemoresistance. We show that in GBA-overexpressing cells, cisplatin significantly reverses the stimulatory effect of GBA overexpression on cell growth. We also show that cisplatin is able to increase apoptosis in GBA-overexpressing cells. This demonstrates that chemotherapy is still effective in inhibiting GBA-overexpressing ovarian cancer cells.
A significant finding of our work is that GBA inhibition decreases AXL expression and suppresses AXL-mediated signaling pathways in ovarian cancer. Receptor tyrosine kinase AXL is overexpressed in several malignancies, including leukemia, gastric cancer, breast cancer and non-small cell lung cancer, and its upregulation is associated with therapy-resistance and metastasis [29]. It regulates multiple oncogenic signaling pathways including PI3K/AKT/mTOR, JAK/STAT, NF-κB, and RAS/RAF/MEK/ERK [30]. Consistent with the previous work that GBA inhibition suppresses phosphorylation of Akt and mTOR in breast cancer cells [17], GBA inhibition also suppresses Akt/mTOR signaling in ovarian cancer. In addition, our work further extends the previous finding by showing that the suppression of Akt/mTOR by GBA inhibition is the consequence of remarkable reduction of AXL level. We speculate that the inhibitory effect of GBA on AXL in ovarian cancer is associated with the role of GBA in sphingolipid metabolism. Glycolipids are the intermediates of sphingolipid metabolism regulated by GBA and their role is to maintain the stability of thecell membrane [31]. AXL is a transmembrane protein and its function is affected by plasma membrane organization. Thus, GBA depletion might disrupt the stability of the cell membrane, leading to inhibition of AXL. It is worthy of pursing the exact mechanisms that lead to Axl reduction in GBA-depleted cells. Aberrant AXL activity is detected in ovarian cancers, particularly in cisplatin-resistant ovarian cancer, and targeting AXL improves sensitivity to platinum and taxane in ovarian cancer [32,33]. This is consistent with our findings that GBA inhibition augments pro-apoptotic and anti-proliferative effects of cisplatin in ovarian cancer cells. Our findings suggest that the inhibition of AXL-mediated signaling pathways by GBA inhibition further enhance anti-proliferative and pro-apoptotic effects by cisplatin in ovarian cancer. Although AXL might not be the exclusive downstream target of GBA, our findings show that targeting GBA represents a promising strategy to inhibit AXL and its mediated signaling pathways to overcome cisplatin chemoresistance.
In conclusion, we reveal that GBA is upregulated in ovarian cancer and supports cell growth and survival. We also demonstrate the therapeutic value of GBA inhibition in ovarian cancer and describes a path to AXL in ovarian cancer cells that proceeds via GBA.
Supplementary data including two tables and three figures can be found with this article online at https://doi.org/10.4196/kjpp.2023.27.1.21.
Notes
REFERENCES
1. Bray F, Ferlay J, Soerjomataram I, Siegel RL, Torre LA, Jemal A. 2018; Global cancer statistics 2018: GLOBOCAN estimates of incidence and mortality worldwide for 36 cancers in 185 countries. CA Cancer J Clin. 68:394–424. Erratum in: CA Cancer J Clin. 2020;70:313. DOI: 10.3322/caac.21492. PMID: 30207593. PMID: https://www.scopus.com/inward/record.uri?partnerID=HzOxMe3b&scp=85053395052&origin=inward.
2. Raja FA, Chopra N, Ledermann JA. 2012; Optimal first-line treatment in ovarian cancer. Ann Oncol. 23 Suppl 10:x118–x127. DOI: 10.1093/annonc/mds315. PMID: 22987945. PMID: https://www.scopus.com/inward/record.uri?partnerID=HzOxMe3b&scp=84866749053&origin=inward.
3. Grabowski JP, Sehouli J. 2015; Current management of ovarian cancer. Minerva Med. 106:151–156. PMID: 25900837. PMID: https://www.scopus.com/inward/record.uri?partnerID=HzOxMe3b&scp=84964525466&origin=inward.
4. Agarwal R, Kaye SB. 2003; Ovarian cancer: strategies for overcoming resistance to chemotherapy. Nat Rev Cancer. 3:502–516. DOI: 10.1038/nrc1123. PMID: 12835670. PMID: https://www.scopus.com/inward/record.uri?partnerID=HzOxMe3b&scp=0043240182&origin=inward.
5. Norouzi-Barough L, Sarookhani MR, Sharifi M, Moghbelinejad S, Jangjoo S, Salehi R. 2018; Molecular mechanisms of drug resistance in ovarian cancer. J Cell Physiol. 233:4546–4562. DOI: 10.1002/jcp.26289. PMID: 29152737. PMID: https://www.scopus.com/inward/record.uri?partnerID=HzOxMe3b&scp=85042552250&origin=inward.
6. Grabowski GA, Osiecki-Newman K, Dinur T, Fabbro D, Legler G, Gatt S, Desnick RJ. 1986; Human acid beta-glucosidase. Use of conduritol B epoxide derivatives to investigate the catalytically active normal and Gaucher disease enzymes. J Biol Chem. 261:8263–8269. DOI: 10.1016/S0021-9258(19)83905-4. PMID: 3087971.
7. Aureli M, Masilamani AP, Illuzzi G, Loberto N, Scandroglio F, Prinetti A, Chigorno V, Sonnino S. 2009; Activity of plasma membrane beta-galactosidase and beta-glucosidase. FEBS Lett. 583:2469–2473. DOI: 10.1016/j.febslet.2009.06.048. PMID: 19577566. PMID: https://www.scopus.com/inward/record.uri?partnerID=HzOxMe3b&scp=67849109791&origin=inward.
8. Futerman AH, Sussman JL, Horowitz M, Silman I, Zimran A. 2004; New directions in the treatment of Gaucher disease. Trends Pharmacol Sci. 25:147–151. DOI: 10.1016/j.tips.2004.01.004. PMID: 15019270. PMID: https://www.scopus.com/inward/record.uri?partnerID=HzOxMe3b&scp=1342306744&origin=inward.
9. Li Y, Sekine T, Funayama M, Li L, Yoshino H, Nishioka K, Tomiyama H, Hattori N. 2014; Clinicogenetic study of GBA mutations in patients with familial Parkinson's disease. Neurobiol Aging. 35:935.e3–e8. DOI: 10.1016/j.neurobiolaging.2013.09.019. PMID: 24126159. PMID: https://www.scopus.com/inward/record.uri?partnerID=HzOxMe3b&scp=85058205837&origin=inward.
10. Chahine LM, Qiang J, Ashbridge E, Minger J, Yearout D, Horn S, Colcher A, Hurtig HI, Lee VM, Van Deerlin VM, Leverenz JB, Siderowf AD, Trojanowski JQ, Zabetian CP, Chen-Plotkin A. 2013; Clinical and biochemical differences in patients having Parkinson disease with vs without GBA mutations. JAMA Neurol. 70:852–858. DOI: 10.1001/jamaneurol.2013.1274. PMID: 23699752. PMCID: PMC3762458. PMID: https://www.scopus.com/inward/record.uri?partnerID=HzOxMe3b&scp=84880296731&origin=inward.
11. Futerman AH, van Meer G. 2004; The cell biology of lysosomal storage disorders. Nat Rev Mol Cell Biol. 5:554–565. DOI: 10.1038/nrm1423. PMID: 15232573. PMID: https://www.scopus.com/inward/record.uri?partnerID=HzOxMe3b&scp=2942687937&origin=inward.
12. Sun Y, Zhang W, Xu YH, Quinn B, Dasgupta N, Liou B, Setchell KD, Grabowski GA. 2013; Substrate compositional variation with tissue/region and Gba1 mutations in mouse models--implications for Gaucher disease. PLoS One. 8:e57560. DOI: 10.1371/journal.pone.0057560. PMID: 23520473. PMCID: PMC3592923. PMID: https://www.scopus.com/inward/record.uri?partnerID=HzOxMe3b&scp=84874761253&origin=inward.
13. Kitatani K, Sheldon K, Anelli V, Jenkins RW, Sun Y, Grabowski GA, Obeid LM, Hannun YA. 2009; Acid beta-glucosidase 1 counteracts p38delta-dependent induction of interleukin-6: possible role for ceramide as an anti-inflammatory lipid. J Biol Chem. 284:12979–12988. DOI: 10.1074/jbc.M809500200. PMID: 19279008. PMCID: PMC2676030. PMID: https://www.scopus.com/inward/record.uri?partnerID=HzOxMe3b&scp=67649752327&origin=inward.
14. Arafa HM. 2009; Possible contribution of beta-glucosidase and caspases in the cytotoxicity of glufosfamide in colon cancer cells. Eur J Pharmacol. 616:58–63. DOI: 10.1016/j.ejphar.2009.06.024. PMID: 19545561. PMID: https://www.scopus.com/inward/record.uri?partnerID=HzOxMe3b&scp=67651108804&origin=inward.
15. Hirano T. 2021; IL-6 in inflammation, autoimmunity and cancer. Int Immunol. 33:127–148. DOI: 10.1093/intimm/dxaa078. PMID: 33337480. PMCID: PMC7799025. PMID: https://www.scopus.com/inward/record.uri?partnerID=HzOxMe3b&scp=85102658220&origin=inward.
16. Zhang Y, Zhu K, Miao X, Hu X, Wang T. 2016; Identification of β-glucosidase 1 as a biomarker and its high expression in hepatocellular carcinoma is associated with resistance to chemotherapy drugs. Biomarkers. 21:249–256. DOI: 10.3109/1354750X.2015.1134662. PMID: 26849828. PMID: https://www.scopus.com/inward/record.uri?partnerID=HzOxMe3b&scp=84958049578&origin=inward.
17. Zhou X, Huang Z, Yang H, Jiang Y, Wei W, Li Q, Mo Q, Liu J. 2017; β-Glucosidase inhibition sensitizes breast cancer to chemotherapy. Biomed Pharmacother. 91:504–509. DOI: 10.1016/j.biopha.2017.04.113. PMID: 28478274. PMID: https://www.scopus.com/inward/record.uri?partnerID=HzOxMe3b&scp=85018776558&origin=inward.
18. Li Z, Xu D, Tong X, Shan C. 2021; Inhibition of β-glucosidase overcomes gastric cancer chemoresistance through inducing lysosomal dysfunction. Clin Res Hepatol Gastroenterol. 45:101456. DOI: 10.1016/j.clinre.2020.04.020. PMID: 32507687. PMID: https://www.scopus.com/inward/record.uri?partnerID=HzOxMe3b&scp=85085930739&origin=inward.
19. Shi SR, Liu C, Young L, Taylor C. 2007; Development of an optimal antigen retrieval protocol for immunohistochemistry of retinoblastoma protein (pRB) in formalin fixed, paraffin sections based on comparison of different methods. Biotech Histochem. 82:301–309. DOI: 10.1080/10520290701791763. PMID: 18097796. PMID: https://www.scopus.com/inward/record.uri?partnerID=HzOxMe3b&scp=38449121657&origin=inward.
20. Tang Z, Li C, Kang B, Gao G, Li C, Zhang Z. 2017; GEPIA: a web server for cancer and normal gene expression profiling and interactive analyses. Nucleic Acids Res. 45:W98–W102. DOI: 10.1093/nar/gkx247. PMID: 28407145. PMCID: PMC5570223. PMID: https://www.scopus.com/inward/record.uri?partnerID=HzOxMe3b&scp=85023171204&origin=inward.
21. Hernandez L, Kim MK, Lyle LT, Bunch KP, House CD, Ning F, Noonan AM, Annunziata CM. 2016; Characterization of ovarian cancer cell lines as in vivo models for preclinical studies. Gynecol Oncol. 142:332–340. DOI: 10.1016/j.ygyno.2016.05.028. PMID: 27235858. PMCID: PMC4961516. PMID: https://www.scopus.com/inward/record.uri?partnerID=HzOxMe3b&scp=84970028733&origin=inward.
22. Vathipadiekal V, Saxena D, Mok SC, Hauschka PV, Ozbun L, Birrer MJ. 2012; Identification of a potential ovarian cancer stem cell gene expression profile from advanced stage papillary serous ovarian cancer. PLoS One. 7:e29079. DOI: 10.1371/journal.pone.0029079. PMID: 22272227. PMCID: PMC3260150. PMID: https://www.scopus.com/inward/record.uri?partnerID=HzOxMe3b&scp=84855857047&origin=inward.
23. Elkabets M, Pazarentzos E, Juric D, Sheng Q, Pelossof RA, Brook S, Benzaken AO, Rodon J, Morse N, Yan JJ, Liu M, Das R, Chen Y, Tam A, Wang H, Liang J, Gurski JM, Kerr DA, Rosell R, Teixidó C, et al. 2015; AXL mediates resistance to PI3Kα inhibition by activating the EGFR/PKC/mTOR axis in head and neck and esophageal squamous cell carcinomas. Cancer Cell. 27:533–546. DOI: 10.1016/j.ccell.2015.03.010. PMID: 25873175. PMCID: PMC4398915. PMID: https://www.scopus.com/inward/record.uri?partnerID=HzOxMe3b&scp=84928015512&origin=inward.
24. Jo H, Mondal S, Tan D, Nagata E, Takizawa S, Sharma AK, Hou Q, Shanmugasundaram K, Prasad A, Tung JK, Tejeda AO, Man H, Rigby AC, Luo HR. 2012; Small molecule-induced cytosolic activation of protein kinase Akt rescues ischemia-elicited neuronal death. Proc Natl Acad Sci U S A. 109:10581–10586. DOI: 10.1073/pnas.1202810109. PMID: 22689977. PMCID: PMC3387065. PMID: https://www.scopus.com/inward/record.uri?partnerID=HzOxMe3b&scp=84862998910&origin=inward.
25. Tchounwou PB, Dasari S, Noubissi FK, Ray P, Kumar S. 2021; Advances in our understanding of the molecular mechanisms of action of cisplatin in cancer therapy. J Exp Pharmacol. 13:303–328. DOI: 10.2147/JEP.S267383. PMID: 33776489. PMCID: PMC7987268. PMID: https://www.scopus.com/inward/record.uri?partnerID=HzOxMe3b&scp=85103132176&origin=inward.
26. Kitatani K, Sheldon K, Rajagopalan V, Anelli V, Jenkins RW, Sun Y, Grabowski GA, Obeid LM, Hannun YA. 2009; Involvement of acid beta-glucosidase 1 in the salvage pathway of ceramide formation. J Biol Chem. 284:12972–12978. DOI: 10.1074/jbc.M802790200. PMID: 19279011. PMCID: PMC2676029. PMID: https://www.scopus.com/inward/record.uri?partnerID=HzOxMe3b&scp=67649710875&origin=inward.
27. Dinur T, Osiecki KM, Legler G, Gatt S, Desnick RJ, Grabowski GA. 1986; Human acid beta-glucosidase: isolation and amino acid sequence of a peptide containing the catalytic site. Proc Natl Acad Sci U S A. 83:1660–1664. DOI: 10.1073/pnas.83.6.1660. PMID: 3456607. PMCID: PMC323143. PMID: https://www.scopus.com/inward/record.uri?partnerID=HzOxMe3b&scp=0343646886&origin=inward.
28. Ogretmen B. 2018; Sphingolipid metabolism in cancer signalling and therapy. Nat Rev Cancer. 18:33–50. DOI: 10.1038/nrc.2017.96. PMID: 29147025. PMCID: PMC5818153. PMID: https://www.scopus.com/inward/record.uri?partnerID=HzOxMe3b&scp=85038830855&origin=inward.
29. Gay CM, Balaji K, Byers LA. 2017; Giving AXL the axe: targeting AXL in human malignancy. Br J Cancer. 116:415–423. DOI: 10.1038/bjc.2016.428. PMID: 28072762. PMCID: PMC5318970. PMID: https://www.scopus.com/inward/record.uri?partnerID=HzOxMe3b&scp=85009188501&origin=inward.
30. Zhu C, Wei Y, Wei X. 2019; AXL receptor tyrosine kinase as a promising anti-cancer approach: functions, molecular mechanisms and clinical applications. Mol Cancer. 18:153. DOI: 10.1186/s12943-019-1090-3. PMID: 31684958. PMCID: PMC6827209. PMID: 34f90097190e412d9db5558bc7397754. PMID: https://www.scopus.com/inward/record.uri?partnerID=HzOxMe3b&scp=85074548394&origin=inward.
31. Shorthouse D, Hedger G, Koldsø H, Sansom MS. 2016; Molecular simulations of glycolipids: towards mammalian cell membrane models. Biochimie. 120:105–109. DOI: 10.1016/j.biochi.2015.09.033. PMID: 26427555. PMCID: PMC4710579.
32. Macleod K, Mullen P, Sewell J, Rabiasz G, Lawrie S, Miller E, Smyth JF, Langdon SP. 2005; Altered ErbB receptor signaling and gene expression in cisplatin-resistant ovarian cancer. Cancer Res. 65:6789–6800. DOI: 10.1158/0008-5472.CAN-04-2684. PMID: 16061661. PMID: https://www.scopus.com/inward/record.uri?partnerID=HzOxMe3b&scp=23044504066&origin=inward.
33. Quinn JM, Greenwade MM, Palisoul ML, Opara G, Massad K, Guo L, Zhao P, Beck-Noia H, Hagemann IS, Hagemann AR, McCourt CK, Thaker PH, Powell MA, Mutch DG, Fuh KC. 2019; Therapeutic inhibition of the receptor tyrosine kinase AXL improves sensitivity to platinum and taxane in ovarian cancer. Mol Cancer Ther. 18:389–398. DOI: 10.1158/1535-7163.MCT-18-0537. PMID: 30478151. PMCID: PMC6363844. PMID: https://www.scopus.com/inward/record.uri?partnerID=HzOxMe3b&scp=85061038639&origin=inward.
Fig. 1
GBA was upregulated in ovarian cancer.
(A) Scatter plot showing the expressions of GBA mRNA in ovarian cancer and normal ovary tissues. (B) Representative IHC images of β-glucosidase for paired ovarian cancer (tumor) and adjacent normal tissue from 3 individual serous ovarian cancer patients. Magnification is ×40. (C) Quantification using Image J software showed the average GBA protein levels in paired ovarian tumor and adjacent normal tissues of 31 ovarian cancer patients. Result is shown as relative to control. (D) GBA protein level was significantly increased in ovarian cancer tissues as assessed by ELSIA assay. (E) Representative Western blot analysis of β-glucosidase on ovarian cancer (tumor) and adjacent normal from 3 individual serous ovarian cancer patients. IHC, immunohistochemistry. Values are presented as mean ± SD. *p < 0.05, compared to normal.

Fig. 2
GBA overexpressing ovarian cancer cells were resistant to cisplatin treatment.
(A) Western blot of GBA in GBA-overexpressing SK-OV-3 and Caov-3 cells. (B) GBA overexpression significantly increased β-glucosidase activity in SK-OV-3 and Caov-3 cells. GBA overexpression did not affect ovarian cancer cell growth (C) and migration (D). Cisplatin treatment resulted in less growth inhibition (E) and apoptosis (F) in GBA-overexpressing ovarian cancer cells compared to control cells. Cisplatin at 0.5 µM was used in proliferation and apoptosis assays. After 3 days drug treatment, proliferation and apoptosis were measured. Results were shown as relative to control. Values are presented as mean ± SD. *p < 0.05, compared to Vector or cisplatin.
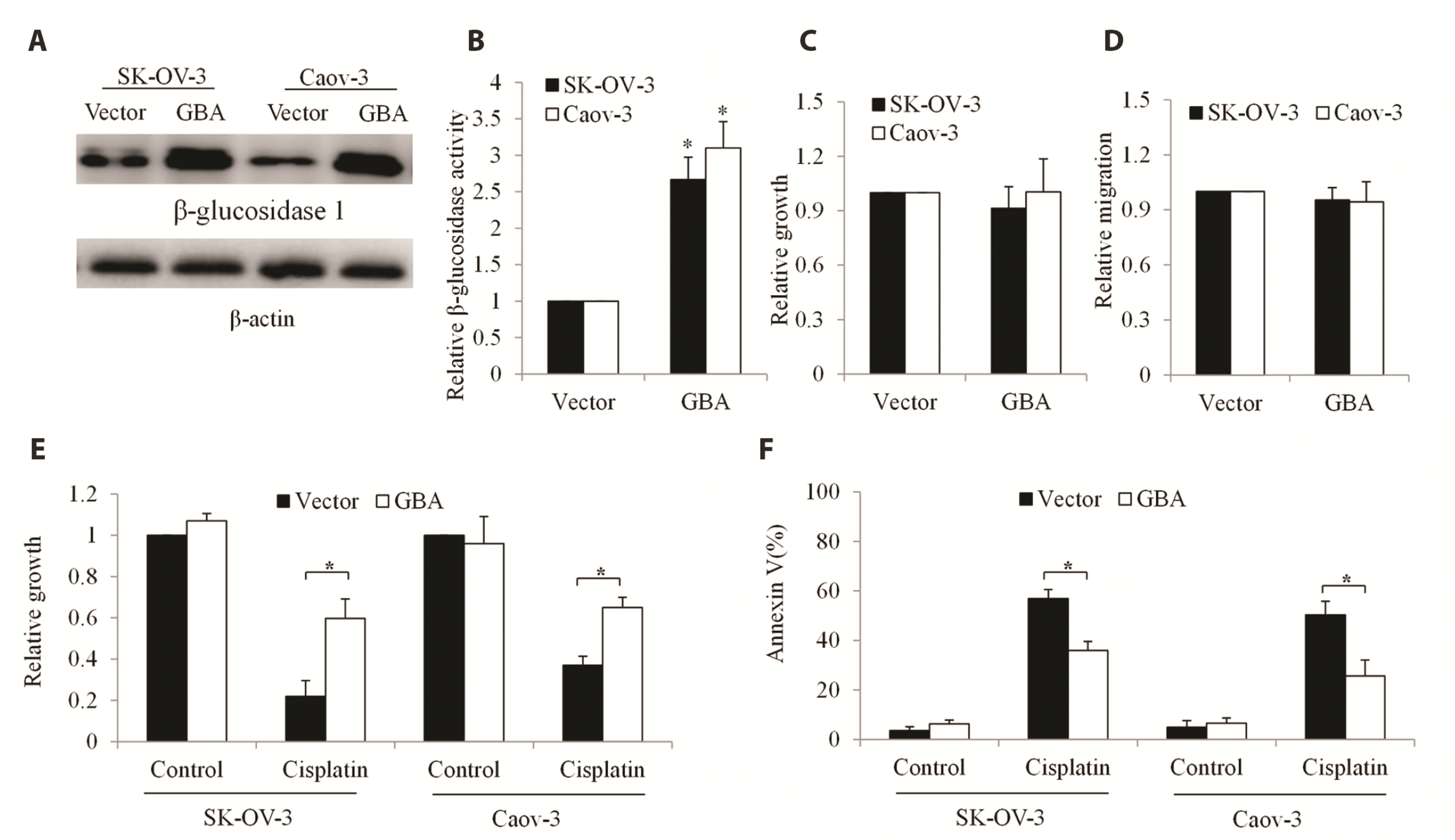
Fig. 3
GBA depletion inhibited growth and survival in ovarian cancer cells and enhanced cisplatin’s efficacy.
(A) Western blot analysis of β-glucosidase levels in SK-OV-3 and Caov-3 cells after transfecting with two specific independent GBA siRNA. (B) Minimal β-glucosidase activity in ovarian cancer cells after GBA depletion. Significant lesser growth (C) and more apoptosis (D) in GBA-depleting cells compared to control cells. GBA knockdown significantly enhanced anti-proliferative and pro-apoptotic effects of cisplatin (100 nM). After 3 days drug treatment, proliferation and apoptosis were measured. Results were shown as relative to control. Values are presented as mean ± SD. *p < 0.05, compared to Scr siRNA. #p < 0.05, compared to cisplatin.
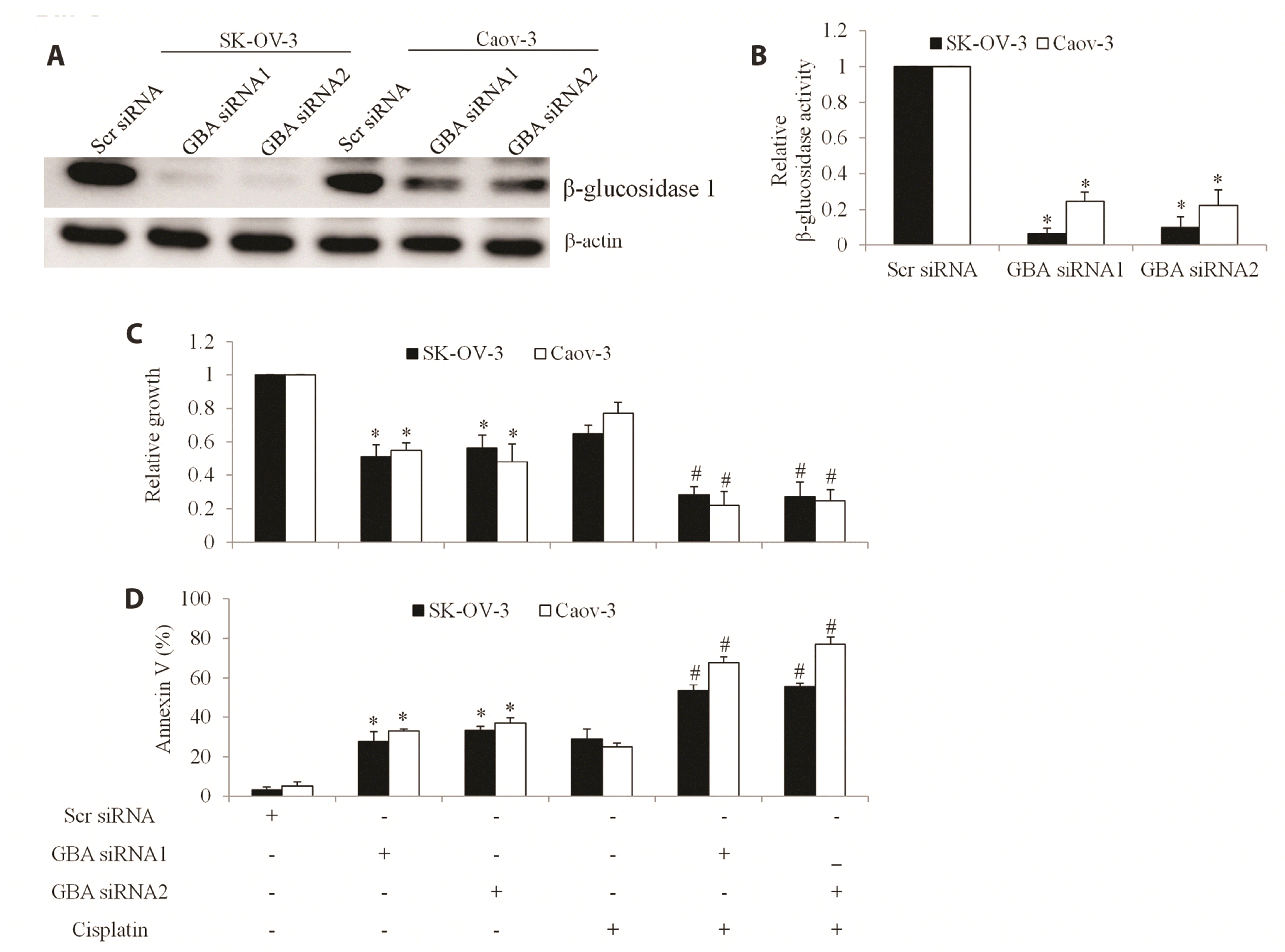
Fig. 4
GBA inhibition suppressed AXL and AXL-mediated signaling pathways in ovarian cancer.
Representative Western blot (A) and quantification of p-AXL and AXL (B); p-Akt, p-mTOR and p-4EBP1 (C); p-ERK and p-90RSK (D) in SK-OV-3 cells after GBA depletion. Values are presented as mean ± SD. *p < 0.05, compared to Scr siRNA.

Fig. 5
SC79 reversed the inhibitory effects of GBA depletion in ovarian cancer cells.
SC79 treatment significantly reversed the anti-proliferative (A) and pro-apoptotic effects (B) of GBA depletion. After 3 days drug treatment, proliferation and apoptosis were measured. Results were shown as relative to control. For this, 5 µg/ml SC79 was used. Values are presented as mean ± SD. *p < 0.05, compared to GBA siRNA.
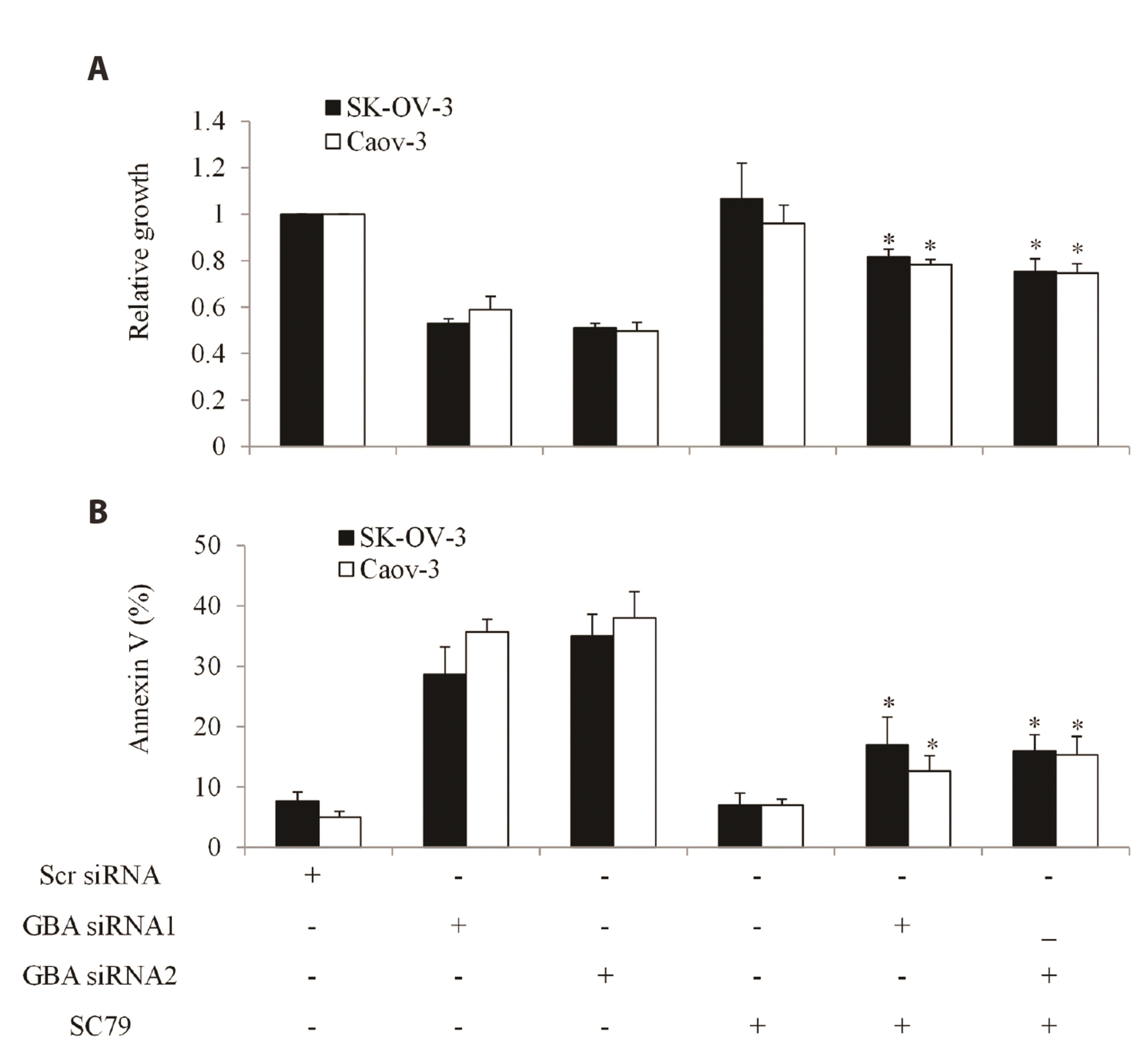