Abstract
Cases of associations between random spinal congenital defects have previously been reported, yet several questions remain unanswered. Firstly, why are associations between what seems to be random combinations of vertebral malformations observed? Secondly, is there a common event or pattern that connects the associated defects? Therefore, this study aimed to identify congenital defects in the vertebral column and also to determine whether any associations, if present, between vertebral malformations exist. This article consequently discusses the possible embryological disruptions that may lead to the formation of various defects in the vertebral column. A random skeletal sample (n=187) was selected from the Pretoria Bone Collection housed in the Department of Anatomy, University of Pretoria (Ethics 678/2018). The sample was evaluated to determine the frequencies of spinal congenital defects in each set of remains. Identifiable congenital malformations were observed in 48.1% (n=90/187) of the sample. The results demonstrated a high probability of association between the different defects observed in the vertebral column. Findings are of value as they provide a reasonable explanation to why seemingly random cases of associations have been reported by several authors. This study is clinically relevant as severe spinal defects have been shown to have high morbidity in patients and mortality in infants.
The central nervous system starts to develop in the third week of gestation [1-3]. Vertebrae develop from somites, and they are formed rhythmically and sequentially through a process called somitogenesis. A factor that influences the morphology of the vertebral column is the timing of segmentation. During segmentation in humans, the paraxial mesoderm is divided into 42–44 pairs of somites, the precursor of vertebral segments [4-6]. The pairs of somites that are formed divide the paraxial mesoderm into various primordial vertebral regions, namely: occipital, cervical, thoracic, lumbar, sacral and coccygeal [5, 7, 8].
Ossification of vertebrae usually starts during the eighth week of embryonic development and continues during adolescence [4, 9-11]. During development, the secondary ossification centres are located in the spinous process, the transverse processes, and the ring apophysis [6, 9]. Some authors state that three ossification centres develop in each vertebra [4, 6]. Other authors suggest that as many as six ossification centres may be present [9].
Normally, each vertebra is temporarily divided into four unfused segments separating the neural arch from the vertebral body, and dividing the midline. There is a coronal cleft in the vertebral body during the first six to 12 months of infancy that is completely fused by two to three years of age. The ossified vertebral junction of the body is separated from the vertebral arch. There are neurocentral synchondroses at the junction of the neural arch and vertebral body. The primary ossification centres of the neural arch are present, however, laminae are not yet fused [4, 12-16] (Fig. 1).
Defects of the vertebral column, due to developmentally delayed fusion of vertebral elements, include: neural tube defects (NTDs), spondylolysis, and craniocaudal border shifts [10, 17].
Abnormalities resulting from incomplete closure of the anterior or posterior neuropore are known as NTDs. The most well-known NTD in the spine is spina bifida (Fig. 2). NTDs are often debilitating, with many surviving individuals subsequently suffering from chronic pain, biomechanical instability, sexual or bowel dysfunction, and even paralysis [4, 18-20].
Congenital spondylolysis (Fig. 3) is a common congenital defect associated with incomplete fusion of the pars interarticularis, separating the vertebral body and the neural arch [10, 21]. This defect is a frequent cause of lower back pain in adults [22, 23] and left untreated, the misaligned vertebrae can lead to isthmic spondylolisthesis. Causes of this condition have been linked to gene expression, environmental factors, or trauma [24, 25].
Meristic transformations in the vertebral column are anomalies that alter the total number of vertebrae in a region and homeotic transformations shift a vertebra from on region to another. Variability in the vertebral column is caused when there is a shift from the typical distribution of vertebral segments in a region. This may change the total number of vertebrae typically seen. Bateson states that changes in vertebral counts are homeotic when ‘one of the component parts of the axial skeleton assumes the morphological appearance and function of its neighbour either immediately preceding or immediately following it… in distinction from meristic variations characterised by changes in total number of component parts’ [4, 6, 12, 26, 27].
Overlapping of developing somites caused by cranial-caudal border shifts can also results in the formation of transitional vertebrae [4, 11, 28, 29]. These vertebral segments are located at a regional junction in the vertebral column and have retained features from the respective adjacent regions [12]. Transitional vertebrae (TV) have been commonly reported in the lumbosacral region and to a lesser extend in the thoracolumbar region. A type of transitional vertebra located between L5 and first sacral (S1) vertebrae is known as a lumbosacral transitional vertebra (LSTV). It is a common cause of back pain and biomechanical instability in patients [30-33]. Transitional vertebrae located between the thoracic and lumbar regions are known as thoracolumbar transitional vertebrae (TLTV). The classification of TLTV was recently broadened according to the overlapping morphology [34] of the vertebrae in question and quantitative morphometries [35].
Several studies have reported cases of defects in the spine that are randomly simultaneous, labelling them as an ‘association’ [36-38]. For example a case report published by George et al. [37] associated a single case of spina bifida occulta to LSTV. The data from those studies, however, do not support an association between the defects, but rather a chance of overlap. Therefore, the literature clearly identifies the need to evaluate whether there is an association between defects in the spine. In addition, it is crucial to understand the developmental disruptions that result in vertebral defects, as these defects can have a significant detrimental effect on the quality of lives of patients.
A random sample of skeletal remains (n=187) from the Pretoria Bone Collection (N=±2000) were examined (ethics number: 678/2018). This sample is representative of the current Pretoria population in Gauteng, South Africa and the sample size is considered sufficient for a 95% confidence interval and a margin of error of 7% for this population. The parameters of this study fall with the requirements set out in the National Health Act 61 of 2003.
The sample included a distribution of adults ranging between the ages of 18 and 88 (mean=58±15.4) years; and represented 155 males and 32 females. Remains were included in the study if the bones were not damaged in any discernible manner and the vertebral column could be fully sequenced for assessment. Sex and ancestry were not considered exclusion factors, however the sample randomly constituted approximately 69% black, 39% white and 2% coloured individuals. Any skeletal remains that demonstrated severe post-mortem damage and/or post-mortem loss of relevant bones or signs of surgical intervention were excluded from the study.
This was an observational study that recorded characteristics from numerous sets of skeletal remains. For this study all possible congenital anomalies, specific to the spine, were considered and overlapping relative frequencies compared. Remains were categorized according to the defects observed. All possible congenital defects of the spine were considered.
Statistical analyses were performed using the following program: Microsoft Excel XLStat extension pack (Addinsoft SARL 2010, Paris, France). Frequency and relative frequency were used to evaluate the distribution of data. This information was cross referenced in table format to observe any patterns in overlap among the various defects including the number and types of defects in every set of remains.
From the initial sample selected (n=223), only 187 met the inclusion criteria. A total of 45 (n=45/223) remains were excluded from the study due to, significant number of key vertebrae missing (n=39/45) and severe post-mortem damage (n=6/45). Malformations in the vertebral column were not observed in (51.9%; n=97/187) of the sample. These remains were classified as ‘normal’, consequently forming the baseline for the sample.
Defects and/or identifiable congenital malformations were observed in 48.1% (n=90/187) of the sample. In this selection the following defects were recorded: sacrococcygeal fusion (73.3%; n=25/90), spondylolysis (6.7%; n=6/90), and TLTV (73.3%; n=66/90). Numeric variations observed were also observed and were classified as homeotic (13.3%; n=12/90) or meristic (18.9%; n=17/90) transformations. Lastly, LSTV were classified as lumbarization (3.3%; n=3/90) or sacralization (31.1%; n=28/90). This study considered other defects such as Kippel-feil syndrome and hemi-vertebrae, however, no cases were found in the sample. Within the selection with notable defects (n=90/187), the frequency of multiple defects in one set of remains was recorded as well (Table 1).
Cross-referencing the relative frequencies of simultaneous defects within the selection was a method utilized to assess the associations. Sacrococcygeal fusion (n=25) had the highest frequency (56%) of cases that did not overlap with any other defect. The results indicate that there is a 12.0% (n=3/25) overlap with meristic transformation and 40.0% (n=10/25) overlap with TLTV. Lumbarization was the least frequent defect observed and, when present, was associated with TLTV in 100% of cases observed (n=3). Spondylolysis (n=6) was the second least frequent defect observed. It overlapped with NTDs in one case and with TLTV in all 6 cases. The results indicated that sacralization overlapped with NTDs in 17.9% (n=5/28) of the cases in the selection; 14.3% (n=4/28) with homeotic transformations; 60.7% (n=17/28) with TLTV and 10.7% (n=3/28) overlap with meristic transformations. The results also show that the majority of NTDs (88.9%; n=16/18) were associated with TLTV. Meristic transformations (n=17) that included the sacrum indicated a 76.5% (n=13/17) association with TLTV. Alternatively, meristic transformation that excludes the sacral region, indicated a 100% association with TLTV. Lastly, all homeotic transformations were associated with TLTV (Table 2).
The most significant finding of this study is the association between TLTV and other noted congenital malformations. The results from this study indicated a 35% prevalence of TLTV. Lumbarization, spondylolysis, and homeotic transformation were associated with TLTV in 100% of cases. In addition, 100% of meristic transformations that excluded the sacrum, were associated with TLTV. This specifically refers to super numeracy in the thoracic or lumbar regions. The results indicate that 89% of cases with TLTV can be associated with NTDs.
If present, an individual will most likely have between one to three different malformations present, with two being the most probable. This is likely due to the cascading effect disruption in development has on the formation of more than one structure and location [4]. Some exceptions to this trend were evident and interestingly, all of the exceptions were defects that were exclusively located in the sacrum. It may be inferred that the high association between TLTV and specific defects exclude the non-mobile segments of the vertebral column relative to the mobile segments, specifically the cervical, thoracic and lumbar regions.
The results showed that 24% of meristic transformations did not overlap with TLTV. In all these cases, the transformation was located in the sacrum. Specifically, an additional sacral segment was present without any other regions in the vertebral column being affected. This is most likely because the formation of an additional sacral vertebral segment does not necessarily involve a craniocaudal border shift at the thoracolumbar junction [6, 18, 39]. Without a border shift at the thoracolumbar junction, TLTV cannot form as the somites do not overlap and the ossification centres are not disrupted.
The results indicated that 60% of the cases with sacrococcygeal fusion were not associated with TLTV. The reported prevalence in literature of sacrococcygeal fusion ranges between 0 to 71.7% [40]. Thus, the result is not surprising, as sacrococcygeal fusion specifically refers to the ankylosing of the coccyx to the sacrum [41, 42]. There are several mechanisms in which the coccyx could potentially fuse to the sacrum. Trauma to the region, such as a fracture, may result in bone remodelling that could fuse the segments [42]. Possible pathology such as degenerative joint disease results in sclerotic lesions that could ankylose the segments.
The research from this study found that the mutual finding between specific defects in the vertebral column is the high association with the presence of TLTV. By cross referencing findings from published literature, events that result in the various defects can likely be explained.
For a defect in the vertebral column to form, disruption of secondary osseous centres in vertebrae must occur [9]. The thoracolumbar junction is the starting point of ossification during development and continues bidirectionally through the vertebral column [4, 5]. As a border shift at the thoracolumbar junction must have occurred to disrupt vertebral morphology to form TLTV, it could be possible that the border shift also disrupts the ossification centres leading to malformations in the vertebral column. This is inferred by the results of this study indicating a high probability of association between TLTV and other congenital malformations [4] (Fig. 4).
Based on the results, it can be inferred that NTDs in the vertebral column result from disruption of secondary ossification centres ‘A’ or ‘F’, as illustrated in Fig. 5. This study observed that NTDs can have bifurcation of the neural arch, which results from inadequate ossification of the spinous process. Alternatively, NTDs may present complete aplasia of the spinous processes resulting in a cleft neural arch.
Dysplastic spondylopathic conditions involve congenital malformation of the pars interarticularis [43]. Congenital spondylolysis has a high association with TLTV, as demonstrated by the results in this study. It can, therefore, be inferred that the disruption of secondary ossification centre ‘E’, as illustrated in Fig. 5, will result in spondylolysis.
Disruption of the ossification centre ‘B’ (Fig. 5) results in aplasia or hypoplasia of the transverse process, a characteristic observed in TLTV. Craniocaudal shifts of the vertebral column can be systemic or regional [40]. The direction, magnitude, and segments involved in the shift determines the type and severity of the defect in the vertebral column.
Individuals with one defect or congenital malformation in the spine are more likely to have at least one other associated defect of the spine. Other defects can occur simultaneously resulting in up to three or, even less likely, four defects. This can be ascribed to the cascading effect that disruptions in embryological development demonstrate. It is evident that TLTV has a high probability to be associated with spondylolysis, NTDs, homeotic transformations, meristic transformations, that exclude the sacrum. In conclusion results suggest that the common event that associates the above specified spinal anomalies is a craniocaudal border shift, suggested by the presence of TLTV, which has features that resemble both the thoracic and lumbar regions caused by overlapping somites. The findings from this study enlighten the pattern of mutual events that causes an association among the various defects in the spine.
Notes
References
1. Greene ND, Stanier P, Copp AJ. 2009; Genetics of human neural tube defects. Hum Mol Genet. 18(R2):R113–29. DOI: 10.1093/hmg/ddp347. PMID: 19808787. PMCID: PMC2758708.
2. Puvirajesinghe TM, Borg JP. 2015; Neural tube defects: from a proteomic standpoint. Metabolites. 5:164–83. DOI: 10.3390/metabo5010164. PMID: 25789708. PMCID: PMC4381295.
3. Farruggia SP, Han C, Watson L, Moss TP, Bottoms BL. 2018; Noncognitive factors and college student success. J Coll Stud Retent Res Theory Pract. 20:308–27. DOI: 10.1177/1521025116666539.
4. Dias MS. 2007; Normal and abnormal development of the spine. Neurosurg Clin N Am. 18:415–29. DOI: 10.1016/j.nec.2007.05.003. PMID: 17678747.
5. Rawls A, Fisher RE. Kusumi K, Dunwoodie SL, editors. 2010. Development and functional anatomy of the spine. The Genetics and Development of Scoliosis. Springer-Verlag;New York: p. 21–46. DOI: 10.1007/978-1-4419-1406-4_2.
6. Thawait GK, Chhabra A, Carrino JA. 2012; Spine segmentation and enumeration and normal variants. Radiol Clin North Am. 50:587–98. DOI: 10.1016/j.rcl.2012.04.003. PMID: 22643386.
7. Greene ND, Copp AJ. 2009; Development of the vertebrate central nervous system: formation of the neural tube. Prenat Diagn. 29:303–11. DOI: 10.1002/pd.2206. PMID: 19206138.
8. Copp AJ, Stanier P, Greene ND. 2013; Neural tube defects: recent advances, unsolved questions, and controversies. Lancet Neurol. 12:799–810. DOI: 10.1016/S1474-4422(13)70110-8. PMID: 23790957. PMCID: PMC4023229.
9. Byrd SE, Comiskey EM. 2007; Postnatal maturation and radiology of the growing spine. Neurosurg Clin N Am. 18:431–61. DOI: 10.1016/j.nec.2007.05.002. PMID: 17678748.
10. Masnicová S, Beňuš R. 2003; Developmental anomalies in skeletal remains from the Great Moravia and Middle Ages cemeteries at Devín (Slovakia). Int J Osteoarchaeol. 13:266–74. DOI: 10.1002/oa.684.
11. Savage C. 2005. Lumbosacral transitional vertebrae: classification of variation and association with low back pain [thesis]. University of Missouri;Columbia:
12. Oostra RJ, Hennekam RC, de Rooij L, Moorman AF. 2005; Malformations of the axial skeleton in Museum Vrolik I: homeotic transformations and numerical anomalies. Am J Med Genet A. 134:268–81. DOI: 10.1002/ajmg.a.30639. PMID: 15732082.
13. Krishnamurthy A, Adibatti M. 2016; Study on incidence of sacralisation of fifth lumbar vertebra in South Indian population. Ital J Anat Embryol. 121:60–5. PMID: 28872798.
14. Moore KL, Agur AMR, Dalley AF. 2012. Essential clinical anatomy. 4th ed. Lippincott Williams & Wilkins;Philadelphia:
15. Drake R, Vogl W, Mitchell A. 2012. Gray's anatomy for students. 2nd ed. Churchill Livingstone;Philadelphia: p. 160.
16. Khairnar KB, Rajale MB. 2013; Sacralization of lumbar vertebra. Indian J Basic Appl Med Res. 2:510–4.
17. Wilson RD, Wilson RD, Audibert F, Brock JA, Carroll J, Cartier L, Gagnon A, Johnson JA, Langlois S, Murphy-Kaulbeck L, Okun N, Pastuck M, Deb-Rinker P, Dodds L, Leon JA, Lowel HL, Luo W, MacFarlane A, McMillan R, Moore A, Mundle W, O'Connor D, Ray J, Van den Hof M. 2015; Pre-conception folic acid and multivitamin supplementation for the primary and secondary prevention of neural tube defects and other folic acid-sensitive congenital anomalies. J Obstet Gynaecol Can. 37:534–52. DOI: 10.1016/S1701-2163(15)30230-9. PMID: 26334606.
18. Kaplan KM, Spivak JM, Bendo JA. 2005; Embryology of the spine and associated congenital abnormalities. Spine J. 5:564–76. DOI: 10.1016/j.spinee.2004.10.044. PMID: 16153587.
19. Kumar A, Tubbs RS. 2011; Spina bifida: a diagnostic dilemma in paleopathology. Clin Anat. 24:19–33. DOI: 10.1002/ca.21058. PMID: 20949487.
20. Greene ND, Copp AJ. 2014; Neural tube defects. Annu Rev Neurosci. 37:221–42. DOI: 10.1146/annurev-neuro-062012-170354. PMID: 25032496. PMCID: PMC4486472.
21. Gillis CC, Eichholz K, Thoman WJ, Fessler RG. 2015; A minimally invasive approach to defects of the pars interarticularis: restoring function in competitive athletes. Clin Neurol Neurosurg. 139:29–34. DOI: 10.1016/j.clineuro.2015.08.024. PMID: 26363364.
22. Wright J, Balaji V, Montgomery AS. 2013; (ii) Spondylolysis & spondylolisthesis. Orthop Trauma. 27:195–200. DOI: 10.1016/j.mporth.2013.07.002.
23. Alton TB, Patel AM, Lee MJ, Chapman JR. 2014; Pediatric cervical spondylolysis and American football. Spine J. 14:e1–5. DOI: 10.1016/j.spinee.2013.09.037. PMID: 24345471.
24. Haukipuro K, Keränen N, Koivisto E, Lindholm R, Norio R, Punto L. 1978; Familial occurrence of lumbar spondylolysis and spondylolisthesis. Clin Genet. 13:471–6. DOI: 10.1111/j.1399-0004.1978.tb01200.x. PMID: 668183.
25. Kim HK, Laor T. 2010; Bilateral congenital cervical spondylolysis. Pediatr Radiol. 40:132. DOI: 10.1007/s00247-009-1349-8. PMID: 19629464.
26. Bots J, Wijnaendts LC, Delen S, Van Dongen S, Heikinheimo K, Galis F. 2011; Analysis of cervical ribs in a series of human fetuses. J Anat. 219:403–9. DOI: 10.1111/j.1469-7580.2011.01400.x. PMID: 21689099. PMCID: PMC3171776.
27. Bateson W. 2014; Materials for the study of variation treated with especial regard to discontinuity in the origin of species. Mater Study Var Treat with Espec Regard to Discontinuity Orig Species. 28:53–4. DOI: 10.5962/bhl.title.17250.
28. Deepa T, John MK. 2014; A study of lumbarisation of first sacral vertebra among the South Indians. Int J Med Res Health Sci. 3:1–4. DOI: 10.5958/j.2319-5886.3.1.001.
29. Jacob M, Agrawal N, Paul D. 2017; Study of additive effect of Dexmedetomidine added to epidural Ropivacaine for orthopedic lower limb procedures. Int J Biomed Res. 8:699–703.
30. Konin GP, Walz DM. 2010; Lumbosacral transitional vertebrae: classification, imaging findings, and clinical relevance. AJNR Am J Neuroradiol. 31:1778–86. DOI: 10.3174/ajnr.A2036. PMID: 20203111. PMCID: PMC7964015.
31. Mahato NK. 2011; Relationship of sacral articular surfaces and gender with occurrence of lumbosacral transitional vertebrae. Spine J. 11:961–5. DOI: 10.1016/j.spinee.2011.08.007. PMID: 21924685.
32. Uçar BY, Uçar DE, Bulut M, Azboy İ, Demirtaş A. 2013; Lumbosacral transitional vertebrae in low back pain population. J Spine. 2:1000125. DOI: 10.4172/2165-7939.1000125.
33. Sekharappa V, Amritanand R, Krishnan V, David KS. 2014; Lumbosacral transition vertebra: prevalence and its significance. Asian Spine J. 8:51–8. DOI: 10.4184/asj.2014.8.1.51. PMID: 24596605. PMCID: PMC3939369.
34. Du Plessis A, Greyling L, Page B. 2018; Differentiation and classification of thoracolumbar transitional vertebrae. J Anat. 232:850–6. DOI: 10.1111/joa.12781. PMID: 29363131. PMCID: PMC5879990.
35. Du Plessis A, Van Schoor A, Wessels Q, Murphy P, Van Schouwenburg F, Ihuhua P, Kehrmann J, Scholtz M, Keough N. 2022; Vertebrae at the thoracolumbar junction: a quantitative assessment using CT scans. J Anat. 240:1179–86. DOI: 10.1111/joa.13619. PMID: 34958488. PMCID: PMC9119610.
36. Vasava PKG, Chaudhary SM. 2017; Lumbarization of first sacral vertebra: a case report. J Anat Soc India. 66(Suppl 1):S109. DOI: 10.1016/j.jasi.2017.08.345.
37. George P, Maria T, Panagiotis K. 2013; Lumbosacral transitional vertebra associated with sacral spina bifida occulta: a case report. Acta Medica (Hradec Kralove). 56:126–9. DOI: 10.14712/18059694.2014.21. PMID: 24592751.
38. Chen L, Battié MC, Yuan Y, Yang G, Chen Z, Wang Y. 2020; Lumbar vertebral endplate defects on magnetic resonance images: prevalence, distribution patterns, and associations with back pain. Spine J. 20:352–60. DOI: 10.1016/j.spinee.2019.10.015. PMID: 31669615.
39. Carrino JA, Campbell PD Jr, Lin DC, Morrison WB, Schweitzer ME, Flanders AE, Eng J, Vaccaro AR. 2011; Effect of spinal segment variants on numbering vertebral levels at lumbar MR imaging. Radiology. 259:196–202. DOI: 10.1148/radiol.11081511. PMID: 21436097.
40. Tague RG. 2011; Sacralization is not associated with elongated cervical costal process and cervical rib. Clin Anat. 24:209–17. DOI: 10.1002/ca.21087. PMID: 21322043.
41. Tague RG. 2011; Fusion of coccyx to sacrum in humans: prevalence, correlates, and effect on pelvic size, with obstetrical and evolutionary implications. Am J Phys Anthropol. 145:426–37. DOI: 10.1002/ajpa.21518. PMID: 21541925.
42. Nathan ST, Fisher BE, Roberts CS. 2010; Coccydynia: a review of pathoanatomy, aetiology, treatment and outcome. J Bone Joint Surg Br. 92:1622–7. DOI: 10.1302/0301-620X.92B12.25486. PMID: 21119164.
43. Peer KS, Fascione JM. 2007; Spondylolysis: a review and treatment approach. Orthop Nurs. 26:104–11. quiz 112–3. DOI: 10.1097/01.NOR.0000265867.70479.cb. PMID: 17414379.
Fig. 1
The sequence of normal ossification of a vertebrae. Step 1, the ossification of the spinous process; Step 2, closure of the posterior neuropore; Step 3, fusion of the vertebral body; Step 4, fusion of the pedicles to the vertebral body and Step 5, the final fused vertebra.
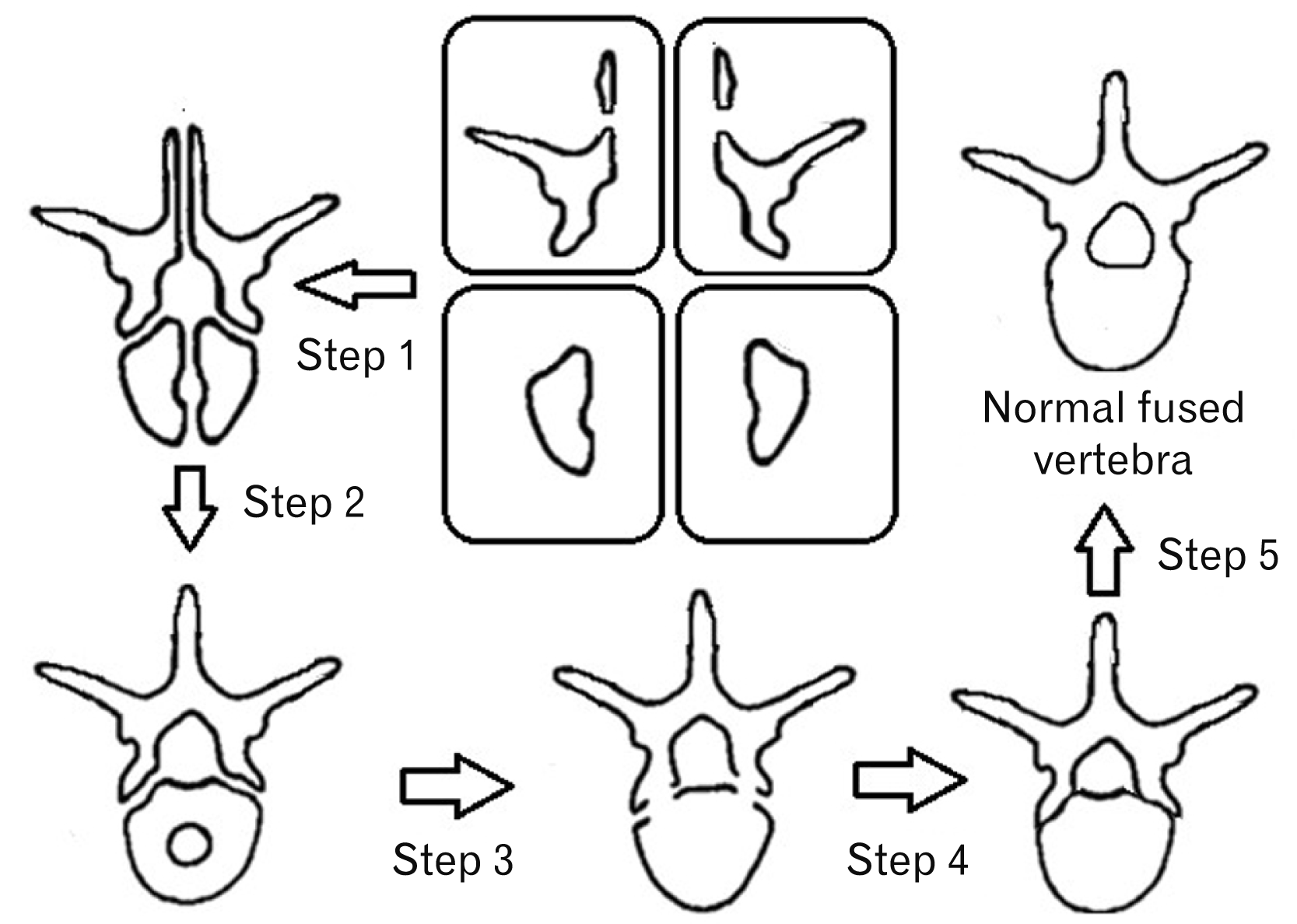
Fig. 2
An example of congenital spondylolysis in the fifth lumbar vertebra observed within the skeletal sample (arrows).
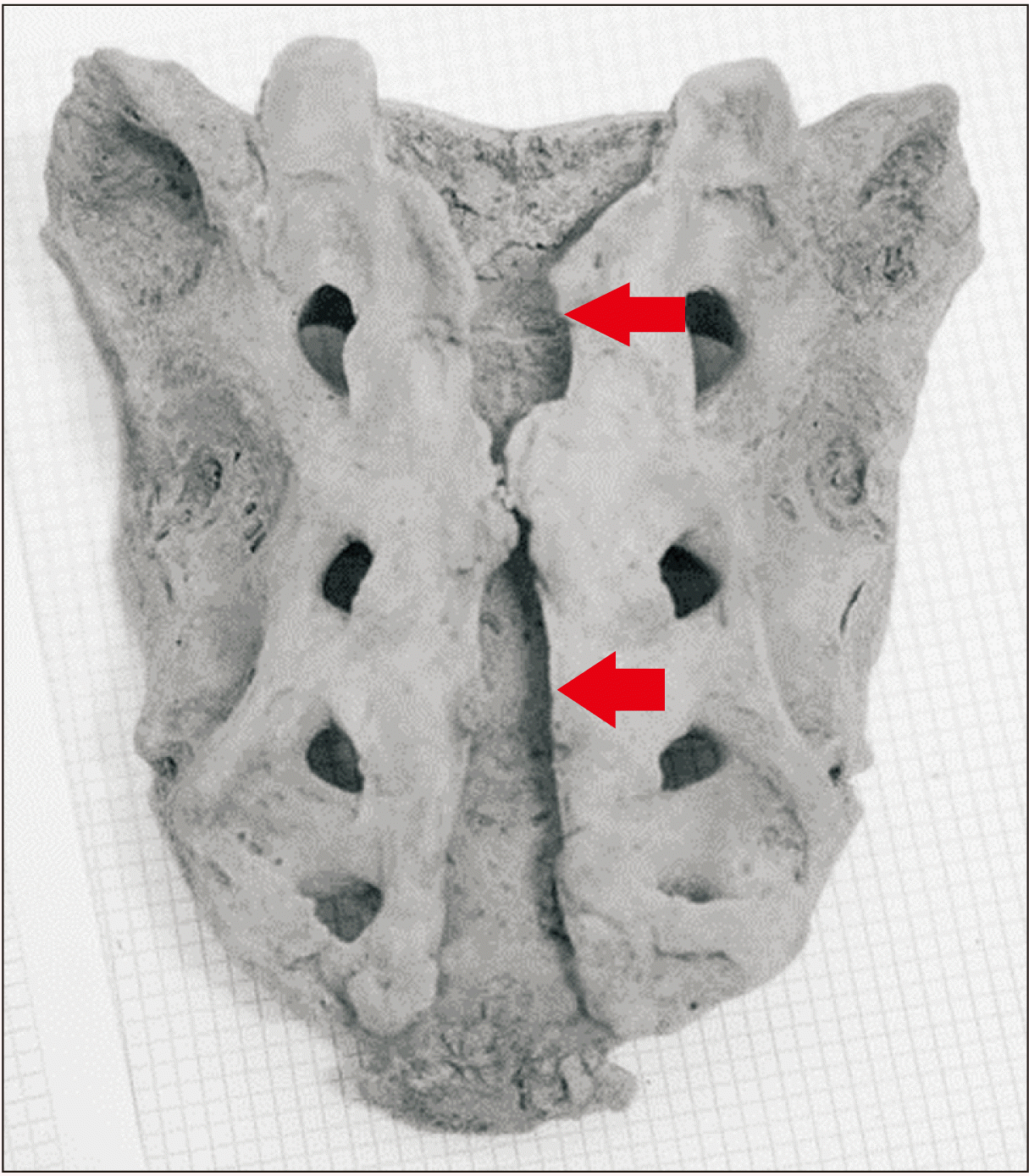
Fig. 4
Illustration of various examples resulting from ossification disruption. (A) The normal layout of the somites relative to the neural tube and T/L junction. (B) A unilateral (possibly bilateral) cranial shift resulting in a defect in the caudal regions. (C) A bilateral (possibly unilateral) caudal shift which results in malformations in the cranial regions. (D) Overlapping developing somites resulting in transitional vertebrae (possible with both cranial and caudal border shifts). T/L, thoracolumbar; X, defect.
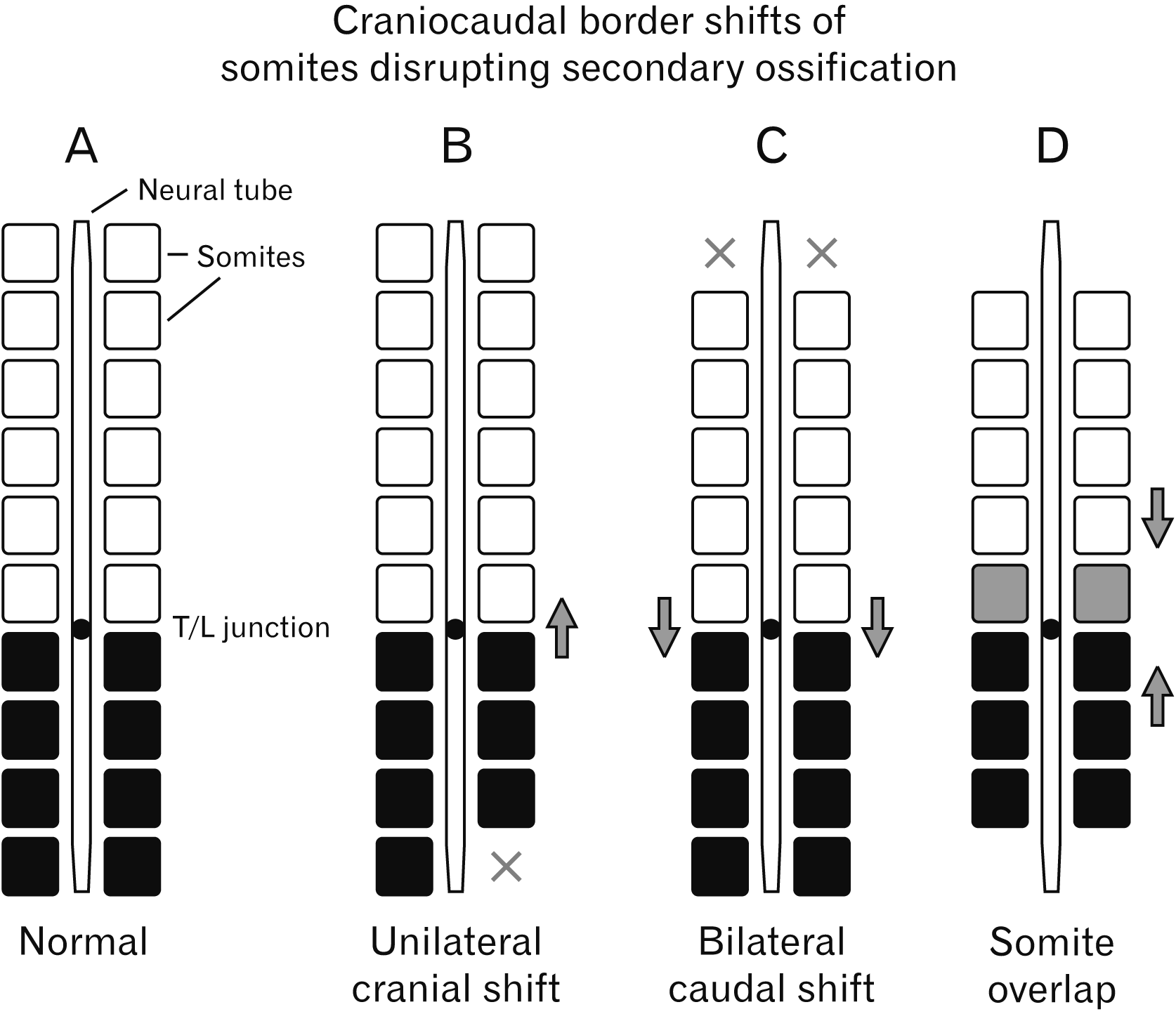
Table 1
A frequency chart demonstrating the number of defects observed as well as the number of remains with more than one defect in the vertebral column
Number of defects in one set of remains | Value (n=90) |
---|---|
1 | 22 (24.4) |
2 | 53 (58.9) |
3 | 13 (14.4) |
4 | 2 (2.2) |
Table 2
A cross-evaluation of the number of remains that demonstrated overlap among the different malformations observed